Optimisation of lactic acid production using cost effective agro residue for food applications†
Received
10th November 2023
, Accepted 23rd January 2024
First published on 4th March 2024
Abstract
Lactic acid (LA) (CH3CHOHCOOH) is utilised in a variety of industrial processes, including production of polymers, emulsifiers, pharmaceutical, and cosmetic formulations. Biological synthesis of useful products by fermentation of renewable resources has advantages over chemical methods. Focus on optimised usage of cost effective regional substrates is necessary to achieve environmentally sustainable practices and efficient biomass fermentation for production of organic acid such as lactic acid. The present study was carried out to optimize lactic acid production using Limosilactobacillus fermentum DFRM8 with cost effective regional carbon substrate (sugarcane bagasse). Experimental design used for the optimization of lactic acid production was 34 full factorial design using four factors such as sugarcane bagasse (%), inoculation level (%), incubation temperature (°C) and pH. The conditions optimized with sugarcane bagasse hydrolysate at 5.0% (v/v), inoculum level at 5.0% (v/v), and incubation temperature at 30 °C with an initial pH of 6.0 produced the maximum lactic acid (22.2 g L−1 on dry weight basis) after 48 h. Simple sugars when used as carbon source in fermentation medium produced (g L−1) LA in the range of 4.5 to 13.5 while agro residues such as mango peel, grape peel and banana peel produced (g L−1) LA at 18.0, 4.5 and 2.7 on dry weight basis. Lactic acid synthesised by the isolate L. fermentum DFRM8 is suitable for food and biomedical applications as the bacterium has GRAS status.
Sustainability spotlight
Our research contributes to the United Nations Sustainable Development Goals 12 (responsible consumption and production) and 13 (climate action) by production of lactic acid through environmentally conscious practices. In our study, we aimed to enhance the sustainability of lactic acid production by repurposing the regionally available agro residue sugarcane bagasse, as the cost-effective renewable carbon substrate. Utilization of regional resources contributes in creation of cost effective culture medium in lieu of conventional production medium containing dextrose. By optimizing production processes utilizing agro residues reduce the impact of wastes on the environmental and also pave way to promote responsible production practices for a more sustainable and resilient future.
|
1. Introduction
Lactic acid (LA) is a valuable chemical widely employed in diverse industries such as food, cosmetics, and pharmaceuticals due to its versatile properties. The relevance of LA spans various sectors, including its use as an alpha-hydroxy acid (AHA) in cosmetics for exfoliation and skin-softening, as well as a preservative in food products owing to its antimicrobial attributes. In the pharmaceutical industry, LA serves as an intermediate in drug synthesis and a chiral resolving agent. This compound is predominantly produced through the fermentation of carbohydrates by microorganisms, resulting in optically active isomers. The resulting LA can be either D- or L-isomer, depending on the microorganisms used.1 Microbial fermentation emerges as a sustainable alternative to chemical synthesis, utilizing cost-effective substrates and various microorganisms, such as bacteria, fungi, cyanobacteria, and algae.2,3 The most potent method for obtaining genetically stable and useable strains for industrial applications has been traditionally through isolation and screening of microorganisms from naturally occurring sources. LAB with commercial potential should be homofermentative, capable of producing only lactic acid to aid in quick purification from the cultivation medium. While synthetic methods exist4 our focus lies on sustainable microbial fermentation, particularly using Limosilactobacillus fermentum DFRM8 and sugarcane bagasse, a cost-effective regional carbon substrate. LAB were recovered from environmental samples on MRS (de Man–Rogosa–Sharpe) agar with one percent calcium carbonate, and incubated at 30 °C for 48 h anaerobically to separate the acid-producers from other bacteria based on clearing zones. Characterization of isolates based on biochemical parameters, Gram staining as well titratable acidity was reported.5
The raising market demand for naturally produced LA (about 130
000–150
000 tonnes per year) is being attributed to its broader applications in the field of food, cosmetics, pharma, and chemical industries.6 Ten percent of total LA synthesised used lactonitrile however, recycling industrial wastes such as lactose-rich whey water through microbial conversion was preferred due to its eco-friendly nature.7 Additionally, the use of renewable feed stocks and ecofriendly processes reduces the environmental impact by sustainable production of LA. Since about 90 percent of LA is produced microbiologically, the industrial production of the LA is primarily based on its fermentative route. Cost of raw materials is the most important factor and selection should be based on characteristics such as low cost, rapid rate of fermentation, lesser inherent contaminants, high LA yields, little or no by-product formation and availability throughout the year.8 LA production is estimated to be over 270
000 tons per year and the market demand is growing at 10 percent annually, which may be attributed to the use of LA in the production of the biocompostable polymer such as Poly Lactic Acid (PLA) which is used in manufacture of biocompostable containers, wraps, single-use trays, mulch films and packaging material. PLA is an environmentally friendly alternative to single use plastics, used in various applications, such as packaging materials and medical implants.9
Culture conditions such as carbon source, incubation temperature as well as pH of the medium influence microbial production of lactic acid, therefore statistical optimisation of factors such as media ingredients and culture conditions for increased LA production is essential. To cut down the cost of industrial production and to pave the way for circular bioeconomy, biotechnology based volarization of lignocellulosic residues obtained from food and agro industries are gaining importance in the present scenario.10 In the present study, LA production using cost effective agro residue (sugarcane bagasse) using Limosilactobacillus fermentum DFRM8 with potential LA production capability was carried out using a 34 factorial experimental design. The LA production was also confirmed by TLC and HPLC.
2. Materials and methods
All biochemicals and chemicals used were of analytical reagent (AR) grade procured from Merck (Mumbai, India), Hi-media (Mumbai, India), Sisco Research Laboratories (Mumbai, India), and Qiagen. Lactic acid was procured from Sisco Research Laboratories (Mumbai, India) and sugarcane bagasse was obtained from local market. Mango peel, banana peel, grapes peel and orange peel were obtained from local fruit juice vendors of Mysore, Karnataka, India.
2.1. Isolation, characterization and identification of Limosilactobacillus fermentum DFRM8 isolate
Samples of dairy products, dairy wastes, batter, and fruit wastes from various places in Mysore, Karnataka, India were collected in sterile airtight collection vials. The sampling area was located in the geographic coordinates 12°29′N and 76°63′E. The samples were collected carefully using 50 mL falcon tubes (Eppendorf) and surface sterilised using absolute ethanol, transported to laboratory in surface sterilised polythene bags and stored at 4 °C until use. The bacterial strain was isolated using de Man–Rogosa and Sharpe agar (MRS) comprising (g L−1): protease peptone 10.0; beef extract 10.0; yeast extract 5.0; dextrose 20.0; ammonium citrate 2.0; MgSO4·7H2O 0.1; agar 20.0 at an initial pH of 6.5 using serial dilution method. The sterile medium was poured in Petri plates, inoculated using quadrant streak plate method and incubated for 24 to 48 h at 37 °C. The single colonies were picked and streaked on fresh MRS agar plates to obtain sixty one bacterial isolates as pure culture. The isolates were screened for lactic acid production based on titratable acidity of the culture broth and were identified based on morphological, physiological, biochemical and molecular methods. DFRM8 exhibited maximum acid production (12.6 g L−1) was selected for further studies. The isolate was found to be catalase negative, Gram positive, and homofermentative in nature (ESI Fig. 1†). The isolate was identified by sequencing the 1.4 kb of 16S rRNA gene using universal eubacterial primers (forward primer: 5′ AGAGTTTGATCCTGGCTAG 3′ and reverse primer: 5′AAGGAGGTGATCCAGCC 3′). Based on the sequence analysis using NCBI-BLAST (https://www.ncbi.nml.nih.gov), it was identified as Limosilactobacillus fermentum, and the nucleotide sequence has been deposited with Genbank under the accession number OP804202. Inoculum was prepared from glycerol stock maintained at −20 °C.
2.2. Screening of microorganisms for lactic acid production
Sixty one bacterial cultures isolated at our laboratory from the present study and isolates obtained during earlier studies (Lactobacillus plantarum DFR4 and Pediococcus acidilactici DFR5) were propagated in 50 mL MRS broth and incubated at 37 °C for 24 h. The acid production was monitored using the cell free supernatant obtained using titratable acidity which was expressed as the percentage of organic acid present in the fermentation medium, which was determined by titration of a known amount of inoculated MRS broth with NaOH (0.1 mol L−1) using phenolphthalein as indicator (ESI Table 1†).
2.3. Inoculum preparation and lactic acid production using Limosilactobacillus fermentum DFRM8
MRS medium in form of broth was used as a seed medium for inoculum preparation. A loopful of inoculum of Limosilactobacillus fermentum DFRM8 was transferred into 30 mL of seed medium in a 100 mL Erlenmeyer flask and incubated for 24 h at 37 ± 2 °C without agitation. Erlenmeyer flasks of 250 mL capacity contained 100 mL of production medium comprising (g L−1): protease peptone 10.0; beef extract 10.0; yeast extract 5.0; dextrose 20.0; ammonium citrate 2.0 and MgSO4·7H2O 0.1. The production medium was inoculated using 2.0 percent (v/v) of 24 h old L. fermentum DFRM8 seed culture. The inoculated flasks were kept under static condition at 37 ± 2 °C for 48 h and lactic acid production was monitored.
2.4. Selection of carbon source and culture conditions for lactic acid production
Effect of various simple sugars such as sucrose, maltose, fructose and lactose were studied instead of dextrose in the media in one-at-a-time approach for production of lactic acid. Acid hydrolysates obtained from cost effective regionally available ingredients as substitutes for carbon source such as sugarcane cane bagasse, mango peel, banana peel, grapes peel and orange peel were also studied one-at-a-time to ascertain their effect on lactic acid production. Fruit peels were sterilized by autoclaving for 20 minutes at 121 °C, followed by boiling at 80 °C for 30 minutes on addition of sterile water at the concentration of 4.0 percent (w/v). The filtrate obtained after boiling was treated with 1.0 percent HCl (v/v) and autoclaved to obtain the acid hydrolysate and pH was adjusted using calcium oxide and CaSO4 formed as by-product was filtered out. The effect of inoculum concentration (1 to 5%), temperature (20, 25, 30,35 and 40 °C) and pH (4 to 8) of the initial media were also studied using one at a time approach on their effect of LA production during submerged fermentation.
2.5. Optimization of lactic acid production using factorial experiments
Factors for the LA media optimization using the selected strain DFRM8 were designed as per 34 factorial with three levels of four different factors, playing major role in LA production. Three levels of four factors such as carbon source (%), inoculum concentration (%), temperature (°C) and pH was chosen for factorial experiments. For 27 runs of 3 replicates, 3 blocks were considered and hence eighty-one combinations were drawn out of the factorial design for optimization. After carrying out all replications, lactic acid production was studied as the response.
2.6. Purification of lactic acid
After incubation for 48 h the cell biomass was precipitated by centrifugation at 12
000 rpm for 20 min and the lactic acid from the cell free supernatant was precipitated using 50 percent (w/v) ammonium sulphate and n-butanol thrice the volume of supernatant for 2 h at 30 °C in a orbital shaker (Orbitek, Scigenics Biotech, Chennai, India). The mixture was transferred to a separatory funnel and allowed to phase separate the aqueous and organic phase into distinct layers. The supernatant obtained was concentrated using rotary vaporizer at 50 °C.
2.7. Thin layer chromatography (TLC) and high performance liquid chromatography (HPLC) analysis of lactic acid
TLC of lactic acid was carried out using silica gel 60 plates (Merck, Germany) according to Lee et al.11 The solvent system for separation was a mixture of acetone
:
water
:
chloroform
:
ethanol
:
ammonium hydroxide (60
:
2
:
6
:
10
:
2, v/v/v/v/v). Lactic acid was detected by spraying the TLC sheets using an indicator solution of 0.25 g of methyl red and 0.25 g of bromophenol blue dissolved in 100 mL of 70 percent methanol and on subsequent drying spots appeared. Liquid chromatography was carried out using 24 series HPLC system (M/s Waters India Pvt. Ltd) and 515 pump equipped with UV-visible variable length detector (2489) set at 210 nm. Phosphate buffer (10 mM, pH 3.0) and acetonitrile at a ratio of 95
:
5 (v/v) was used as mobile phase with C 18 column (250 × 4.6 mm × 5 μm) Kyatech as stationary phase and the temperature of column was 42.0 °C. The mobile phase was set for both pumps (A and B) at 0.5 mL min−1 to get the final flow rate of 1.0 mL min−1. Once pump stabilization and baseline was achieved, the prepared stock solution and calibration standards were injected. Linear data obtained for the standard lactic acid was used to determine the lactic acid concentration of the unknown samples by comparisons using peak area and retention time of standard lactic acid. Chromatograms were generated using Empower® software.
2.8. Data analysis
The results were analyzed using STATISTICA release 7 (Stat Soft Inc., Tulsa, OK). An Analysis of Variance (ANOVA) test based on least significant difference (LSD) at p < 0.05 was used to determine whether experimental results are significant. Coefficient of regression (R2) indicated the quality of fit and interactive effects between the factors such as sugarcane bagasse, inoculum level, temperature of incubation and initial pH of the production medium that affects the production of lactic acid were investigated and results were graphically presented. Using profiles for predicted values and desirability, approximate-optimal values of the quadratic functions were predicted.
3. Results and discussions
3.1. Effect of carbon source, inoculum concentration, temperature and pH of the production medium for lactic acid production
Carbon sources viz. sucrose, fructose, maltose and lactose as well as cost effective regionally available carbon substrates such as sugarcane cane bagasse, mango peel, banana peel, grapes peel and orange peel and their effect on lactic acid production was investigated. Among the simple sugars tested such as fructose, glucose, lactose, maltose and sucrose for three isolates (Limosilactobacillus fermentum DFRM8, Lactobacillus plantarum DFR4 and Pediococcus acidilactici DFR5), lactose containing medium produced the maximum amount (13.5 g L−1) of lactic acid while fructose produced the least (4.5 g L−1) while glucose and sucrose as carbon substrate produced 12.0 and 10.8 g L−1 of lactic acid, respectively (Fig. 1). Isolate obtained in this present study L. fermentum DFRM8 produced lactic acid (4.5 to 13.5 g L−1) using simple sugars except fructose which was converted less into LA. Pediococcus acidilactici DFR5 produced LA in the range of 7.2 to 9.0 g L−1 and converted lactose more efficiently into LA in comparison to other simple sugars. Lactobacillus plantarum DFR4 produced LA in the range of 1.8 to 8.1 g L−1 and converted glucose into LA more efficiently while preferred fructose the least. Variation in the capability of utilisation of simple sugars may be attributed to the variation in isolates based on the food source of isolation as well as their genetic makeup. Xavier et al.12 reported during optimisation of carbon sources for microbial levan production, among simple sugars such as galactose, lactose, maltose, sucrose and xylose maximum production was noticed in the presence of sucrose while lactose, galactose and starch failed to support levan production. γ-Poly glutamic acid (γ-PGA) production during submerged fermentation was reported maximum in rice bran carbon substrate, to a limited extent when sugarcane juice, wheat bran and glucose as carbon substrates while cane molasses did not support production of γ-PGA.13
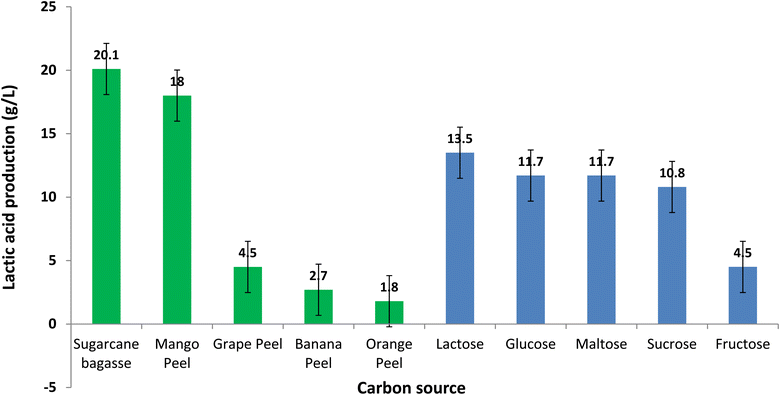 |
| Fig. 1 Effect of various carbon sources on lactic acid production (g L−1) using Limosilactobacillus fermentum DFRM8. | |
Among the cost effective carbon sources tested acid hydrolysates obtained using sugarcane bagasse produced the maximum amount (20.1 g L−1) of lactic acid on dry weight basis among the substrates tested while orange peel acid hydrolysate produced the least (1.8 g L−1) while mango peel, grape skin and banana peel acid hydrolysate as carbon substrate produced 18.0, 4.5 and 2.7 g L−1 of lactic acid, respectively (Fig. 2). LA production was maximum in the residues of sugarcane and mango peel, which may be attributed to the increased availability of residual sugars in comparison to peels obtained from banana, orange and grapes. The lactic acid production was found to be influenced by the other components such as poly phenols, dietary fiber as well as sugars present in the acid hydrolysates of the cost effective substrates used for submerged fermentation of the isolates DFRM8 and other two cultures obtained from our earlier studies. However, isolate DFRM8 was found to volarize the residues in the order of sugarcane bagasse > mango peel > grape peel > orange peel > banana peel. This may be attributed to the preference of the isolate DFRM8 for a particular substrate in comparison to others. The isolate selected in the present study based on highest titratable acidity was capable of maximum lactic acid production when compared to isolates obtained in our earlier study. Furthermore, the isolate DFRM8 was selected for optimization of lactic acid production using cost effective preferred substrate sugarcane bagasse by statistical methods.
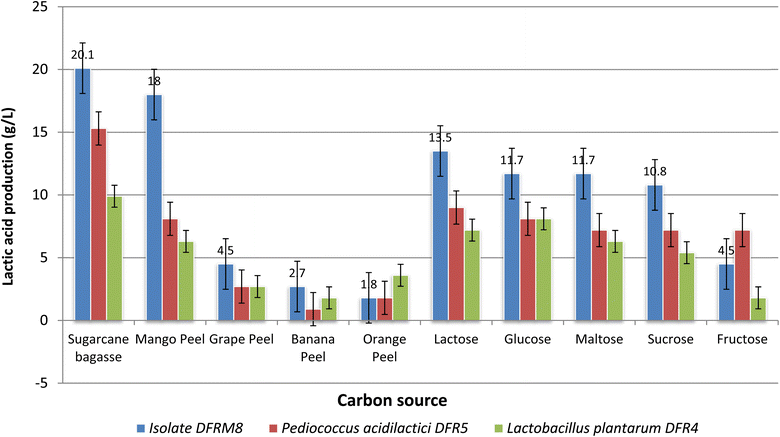 |
| Fig. 2 Effect of various carbon sources during submerged fermentation using different isolates for lactic acid production (g L−1). | |
Sabater et al.14 also reported variations in the outcome of fermentation based on the fruits and vegetable by-products. Abedi and Hashemi15 reported that lactic acid bacterial cultures preferred lactose over other simple sugars for lactic acid production which is similar to the present study. Umesh et al.16 studied effect of fruit peel waste as a promising carbon source for LA production using Lactobacillus plantarum. In their study, they used various fruit wastes such as mango, orange, banana and pineapple. Among the selected fruit waste hydrolysates highest LA (10.8 g L−1) production was observed in medium containing mango peel hydrolysate while banana and pineapple peels produced the lowest amount of LA (4.7 g L−1). Jawad et al.17 used mango peel as fermentation substrate and optimized the LA production using factorial experiments. The study concluded that the highest LA concentration was observed as 17.48 g L−1 in the optimized medium with various factors such as pH, temperature and fermentation time.
3.2. Optimization of selective medium components using factorial experiments
Statistical optimisation method based on 34 factorial experiments (Statsoft software, Version, 7) was carried out to optimise suitable parameters to maximize the production of lactic acid (g L−1). Four factors (independent variables) such as carbon source concentration (%), inoculum level (%), temperature (°C) and pH at three levels, 3 blocks and 3 replicates were designed resulting in 81 combinations. One-factor-at a time experiments carried out indicated that production medium inoculated with bacterial culture, need to be incubated at 37 °C for 48 h for LA production. However, variables such as carbon source (sugarcane bagasse), inoculum level, temperature and pH of production media for LA by L. fermentum were optimized by factorial experiments. The fermentation conditions obtained through preliminary experiments were not modified during the optimization process. Responses (lactic acid production g L−1) for 81 experiments using factorial method, and the actual and predicted LA production are shown (Table 1 and ESI Table 2†). Production of LA ranged from 2.4 to 22.2 g L−1 on dry weight basis and was maximum for the 26th run under the experimental conditions: carbon source (%) = 5.0; inoculum level (%) = 5.0; temperature (°C) = 30.0; and pH = 6.0. The lowest production was reported in 1st run with the conditions of carbon source (%) = 2.0; inoculum level (%) = 1.0; temperature (°C) = 20.0 and pH = 4.0. The optimized carbon source and inoculum concentration and production conditions such as incubation temperature and initial pH of the medium for obtaining desirable response of maximized LA production was designed based on the above findings. Kotzamanidis et al.18 studied the effect of the three variables including sucrose concentration, yeast extract and CaCO3, individually at five levels, and their interactive effects on lactic acid concentration. Significant interactions were reported between sucrose and yeast extract, as well as sucrose and CaCO3 levels. Saavedra et al. (2021) carried out LA optimization using cane molasses (carbon source) as a factor in which highest LA production was observed in medium containing cane molasses concentration of 8.0 percent, and concluded the linear effect of cane molasses on LA production. Cane molasses at 8.0 (%) was used as carbon source for LA production and a linear effect was observed for increase in carbon source.19 Cost effective carbon sources such as rice bran and sugarcane juice for production of exopolysaccharides such as poly glutamic acid13 and levan12 using Response Surface Methodology (RSM). According to Ha et al.20 temperature optima for growth of lactic acid bacteria varies in the range of 20 °C to 45 °C and obviously it varies from species to species. Ilmen et al.21 reported lactic acid production of maximum 33.72 g L−1 at 37 °C by L. casei. From these observations, it is clear that a temperature range of 37 °C to 40 °C was considered as optimal for lactose conversion by bacterial cells during production of LA. Sarkar and Paul22 optimized LA concentration using temperature as a factor with levels including 20 °C, 25 °C, 30 °C, 37 °C, 45 °C and 50 °C. According to their findings the highest LA concentration (g L−1) was found at 37 °C and lowest at 20 °C with 43.6 and 10.12, respectively. Wang et al.23 indicated a pH range of 6.0–7.0 for LA production using L. casei, which is similar to our present study. However, Ha et al.20 suggested pH 5.5 as the optimum for LA production using L. helveticus. Similarly, Hofvendahl and Hahn-Hagerdal24 reported that almost 95 percent lactose conversion up to 33.48 g L−1 lactic acid production (w/v) with an initial medium pH of 6.5.
Table 1 Optimization of lactic acid production using 34 factorial layoutsa
Carbon source (%) |
Inoculum concentration (%) |
Temperature (°C) |
pH |
Lactic acid (g L−1) |
Observed value |
Predicted value |
Residual |
Values are derived from three replications and expressed as mean ± S.D.
|
2.00 |
1.00 |
20.00 |
4.00 |
2.4 ± 0.52 |
2.4 ± 0.52 |
3.3 ± 0.00 |
−0.60 |
2.00 |
1.00 |
30.00 |
8.00 |
5.7 ± 0.52 |
5.7 ± 0.52 |
5.57 ± 0.00 |
−0.17 |
2.00 |
1.00 |
40.00 |
6.00 |
4.2 ± 0.52 |
4.2 ± 0.52 |
5.3 ± 0.00 |
−1.70 |
2.00 |
3.00 |
20.00 |
8.00 |
5.1 ± 0.52 |
5.1 ± 0.52 |
5.6 ± 0.00 |
−0.20 |
2.00 |
3.00 |
30.00 |
6.00 |
7.5 ± 0.52 |
7.5 ± 0.52 |
7.3 ± 0.00 |
−0.10 |
2.00 |
3.00 |
40.00 |
4.00 |
7.2 ± 0.00 |
7.2 ± 0.00 |
6.27 ± 0.00 |
0.93 |
2.00 |
5.00 |
20.00 |
6.00 |
9.3 ± 1.04 |
9.3 ± 1.04 |
8.27 ± 0.00 |
1.63 |
2.00 |
5.00 |
30.00 |
4.00 |
8.7 ± 0.52 |
8.7 ± 0.52 |
9.2 ± 0.00 |
−0.20 |
2.00 |
5.00 |
40.00 |
8.00 |
10.2 ± 1.04 |
10.2 ± 1.04 |
9.5 ± 0.00 |
1.30 |
3.00 |
1.00 |
20.00 |
8.00 |
10.5 ± 1.37 |
10.5 ± 1.37 |
9.8 ± 0.00 |
−0.80 |
3.00 |
1.00 |
30.00 |
6.00 |
12.3 ± 0.52 |
12.3 ± 0.52 |
11.5 ± 0.00 |
1.10 |
3.00 |
1.00 |
40.00 |
4.00 |
9.9 ± 0.90 |
9.9 ± 0.90 |
10.47 ± 0.00 |
−0.57 |
3.00 |
3.00 |
20.00 |
6.00 |
11.4 ± 1.04 |
11.4 ± 1.04 |
11.53 ± 0.00 |
−0.73 |
3.00 |
3.00 |
30.00 |
4.00 |
12.0 ± 1.04 |
12.0 ± 1.04 |
12.47 ± 0.00 |
0.13 |
3.00 |
3.00 |
40.00 |
8.00 |
12.0 ± 0.52 |
12.0 ± 0.52 |
12.77 ± 0.00 |
−0.17 |
3.00 |
5.00 |
20.00 |
4.00 |
14.1 ± 0.52 |
14.1 ± 0.52 |
13.43 ± 0.00 |
0.07 |
3.00 |
5.00 |
30.00 |
8.00 |
14.7 ± 0.52 |
14.7 ± 0.52 |
15.7 ± 0.00 |
−1.30 |
3.00 |
5.00 |
40.00 |
6.00 |
16.2 ± 0.90 |
16.2 ± 0.90 |
15.43 ± 0.00 |
0.77 |
5.00 |
1.00 |
20.00 |
6.00 |
15.9 ± 0.52 |
15.9 ± 0.52 |
16.47 ± 0.00 |
−0.27 |
5.00 |
1.00 |
30.00 |
4.00 |
17.7 ± 0.52 |
17.7 ± 0.52 |
17.4 ± 0.00 |
0.60 |
5.00 |
1.00 |
40.00 |
8.00 |
18.9 ± 0.00 |
18.9 ± 0.00 |
17.7 ± 0.00 |
1.20 |
5.00 |
3.00 |
20.00 |
4.00 |
18.3 ± 0.52 |
18.3 ± 0.52 |
17.43 ± 0.00 |
0.57 |
5.00 |
3.00 |
30.00 |
8.00 |
20.4 ± 1.04 |
20.4 ± 1.04 |
19.7 ± 0.00 |
0.10 |
5.00 |
3.00 |
40.00 |
6.00 |
18.6 ± 0.52 |
18.6 ± 0.52 |
19.43 ± 0.00 |
−0.53 |
5.00 |
5.00 |
20.00 |
8.00 |
19.5 ± 0.52 |
19.5 ± 0.52 |
20.67 ± 0.00 |
−1.77 |
5.00 |
5.00 |
30.00 |
6.00 |
22.2 ± 1.04 |
22.2 ± 1.04 |
22.37 ± 0.00 |
−0.77 |
5.00 |
5.00 |
40.00 |
4.00 |
21 ± 0.52 |
21.0 ± 0.52 |
21.33 ± 0.00 |
−0.63 |
3.3. Fitting and verification of the model used for lactic acid production
An Analysis of Variance (ANOVA) test was used to determine whether experimental results are significant and to determine whether to reject null hypothesis or to accept alternate hypothesis. It was also observed that main effects (linear and quadratic) such as carbon source concentration (%), inoculum level (%), temperature (°C) and pH were statistically significant (p < 0.005) in comparison to interactive effects among the factors tested which are graphically represented using normal probability plot. This plot illustrates the magnitude, direction and the importance of the effects and interactive effects of the independent variables on the dependant variable i.e. lactic acid production. Effects which are farther from the value zero (0) of the x-axis show statistically significant effect on the dependant variable or response i.e. lactic acid production. Therefore, main effects such as carbon source and inoculum level showed a statistically significant linear effect on lactic acid production (ESI Fig. 2†).
Effects estimate of all the four factors tested, indicated a highly significant (p < 0.005) positive relationship and ranked in the order; carbon source concentration (%) > inoculum level (%) > temperature (°C) > pH (ESI Tables 3 and 4†). Data obtained from factorial design was assessed statistically for univariate ANOVA (main effects only) using least significant difference (LSD). ANOVA values of LA production for factorial design is shown (Table 2). Significance level of each regression coefficient for LA production was obtained based on the p-value of F-test by regression analysis (ANOVA) (Table 2). A lower p-value (p < 0.05) for a given coefficient indicates a more significant effect of that coefficient on the LA production. The high coefficient of determination, R2 = 97.06 percentage, indicated that the model is a good fit for the experimental data and suggested a close relationship between the experimental data and the predicted values. An R2 value greater than 75 percent indicates a high degree of aptness and quality of the model at a significance level of 0.0005%. In the present study, the R2 value suggests that the quadratic model can explain more than 95 percent of the variability in the response, which indicates that the model is highly suitable for explaining the experimental data. The expected versus residual values of LA production clustered around the diagonal line (ESI Fig. 3†), indicating a close relationship between the experimental and predicted values and a satisfactory model for the optimization of LA production. Overall, the results suggest that the model is highly effective in predicting LA production, and the coefficients with lower p-values are the most significant for optimizing LA production.
Table 2 Analysis of variance (ANOVA) and regression analysis for factorial design of lactic acid production (g L−1)a
Term |
Sum of squares (SS) |
Degrees of freedom (DF) |
Mean square (MS) |
F-Value |
p-Value |
*Values are significant at p < 0.05.
|
Analysis of variance
|
Blocks |
3.620 |
2 |
1.810 |
3.765 |
0.029719* |
Carbon source (%) |
2100.56 |
2 |
1050.28 |
1068.599 |
0.000000* |
Inoculum (%) |
249.68 |
2 |
124.84 |
127.017 |
0.000000* |
Temperature (°C) |
40.22 |
2 |
20.11 |
20.461 |
0.000000* |
pH |
8.06 |
2 |
4.03 |
4.1 |
0.020705* |
Error |
68.8 |
70 |
0.983 |
|
|
Total SS |
2470.94 |
80 |
|
|
|
Regression analysis
|
Model |
2398.52 |
8 |
580.93 |
298.08 |
0.000000 |
Residual |
72.42 |
72 |
1.0058 |
|
|
Total |
2470.940 |
80 |
|
|
|
3.4. Effect of media components and culture conditions on lactic acid production
Contour and surface plot for desirability based on quadratic fit for lactic acid production (g L−1) is shown (ESI Fig. 4 and 5†). Behaviour of LA production (above) and desirability function (below) with changes in experimental conditions and carbon source concentration (sugarcane bagasse) is shown (ESI Fig. 6†). The horizontal dotted line on the response graph shows the highest LA production achieved by this model (22.508). The horizontal line on the desirability graph indicates the maximum achieved desirability (0.958). The vertical lines correspond to the approximate optimal value of the factors i.e. the parameters at which the maximum value for LA production and desirability is achieved: 5.0 (%) cane bagasse, 5.0 (%) inoculum level, 35.0 °C and 7.0 pH; at this specified levels LA production was 22.5 (g L−1). The desirability function between 0 and 1 indicates the degree of performance for the predicted response with the given factors corresponding value and the mean value of the remaining factors.
3.5. Characterization of lactic acid
3.5.1. Identification using TLC and HPLC analysis.
Lactic acid obtained was analysed using TLC in order to screen for LA production. Presence of LA was visualized as a red spot using an indicator dye (combination of methyl red and bromophenol blue). LA produced by Limosilactobacillus fermentum DFRM8 (retention factor 0.52) was compared with lactic acid standard (retention factor 0.52) (ESI Fig. 7†). Chen et al.25 carried out thin layer chromatography for genus identification of Bifidobacterium in comparison with other lactic acid bacterial strains and got retention factor for lactic acid in a range of 0.35–0.54.
Culture free supernatant of three cultures such as Lactobacillus plantarum DFR4, Pediococcus acidilactici DFR5 along with Limosilactobacillus fermentum DFRM8 were partially purified by precipitation method using 50 percent (w/v) ammonium sulphate and n-butanol to obtain LA. They were also screened for the presence of LA using HPLC. HPLC chromatogram was obtained for the standard lactic acid and partially purified LA obtained using the isolate Limosilactobacillus fermentum DFRM8. Isolate DFRM8 showed the presence of LA at a retention time of 3.953 minutes with the highest concentration in comparison with other cultures tested (ESI Fig. 8 a and b†). Zamanova et al.26 carried out simultaneous HPLC-UV determination of ethyl acetate, lactide, lactic acid and glycolic acid, glycolide monomers for production of biodegradable polymers. They carried out HPLC using reversed phase C-18 column and the samples were analyzed. From their study, the peak corresponding to retention time 4.99 minutes was identified as LA. They also conducted analysis of glycolic acid which had a retention time of 4.69 minutes. Bai et al.27 in their study determined organic acids in fermentation medium of Rhizopus oryzae using Wakosil II 5C18RS column using 0.01 mol L−1 phosphoric acid (pH 2.5) as the mobile phase with a flow rate of 1.0 mL min−1 and UV detection at 210 nm. The retention time of LA was found to be 3.820 minute.
4. Conclusions and future prospects
Lactic acid producing L. fermentum DFRM8 isolated in the current investigation could be used for microbial lactic acid production. The optimized fermentation medium using sugarcane bagasse can be considered as a suitable cost effective regional substrate for up scaling lactic acid production. Scalability and optimisation of the LA production on larger scale using fermenter systems would be useful as L. fermentum has GRAS status and applications such as food preservatives, immunity booster, as well as biomedical use as an alternative for antibiotics. The generated lactic acid could be used as a precursor for synthesis of Poly Lactic Acid (PLA), a biodegradable polymer comprising of lactic acid monomers for the development of green composites, based on agro residues such as (banana fiber, corn cob powder, paddy husk, coffee berry waste), for food applications is being explored in our laboratory. Creation of microbial consortia of LAB and investigation of their adaptability to various agro-industrial residues will contribute to expansion of versatility and efficiency of the proposed approach for LA production.
List of abbreviations
AHA | Alpha-hydroxy acid |
AR | Analytical reagent |
ANOVA | Analysis of variance |
GRAS | Generally recognised as safe |
HPLC | High performance liquid chromatography |
LA | Lactic acid |
LAB | Lactic acid bacteria |
LSD | Least significant difference |
MRS | de Man–Rogosa and Sharpe |
NCBI-BLAST | National Center for Biotechnology Information Basic Local Alignment Search Tool |
γ-PGA | γ-Poly glutamic acid |
PLA | Poly lactic acid |
TLC | Thin layer chromatography |
RSM | Response surface methodology |
Conflicts of interest
On behalf of all authors, the corresponding author states that there is no conflict of interest.
Acknowledgements
The authors thank The Director, DFRL Mysore for providing his valuable suggestions during this work.
References
- D. Chauliac, P. C. Pullammanappallil, L. O. Ingram and K. T. Shanmugam, A combined thermochemical and microbial process for recycling polylactic acid polymer to optically pure l-lactic acid for reuse, J. Polym. Environ., 2020, 28, 1503–1512 CrossRef CAS.
- R. E. Izzaty, B. Astuti and N. Cholimah, Microbial production of lactic acid: the latest development, Angew. Chem., Int. Ed., 1967, 6(11), 951–952 CrossRef.
- I. A. Adesokan, B. B. Odetoyinbo and B. M. Okanlawon, Optimization of Lactic Acid Production by Lactic Acid Bacteria Isolated from Some Traditional Fermented Food in Nigeria, Pak. J. Nutr., 2009, 8(5), 611–615 CAS.
- V. Juturu and J. C. Wu, Microbial production of lactic acid: the latest development, Crit. Rev. Biotechnol., 2016, 36(6), 967–977 CrossRef CAS PubMed.
- N. E. A. Tayyem, K. T. Elhello and A. A. Elmanama, Isolation, identification and growth conditions of calcite producing bacteria from urea-rich soil, Afr. J. Microbiol. Res., 2021, 15(1), 37–46 CrossRef.
-
S. M. Bhatt, Economical lactic acid production and optimization strategies, Fungal Biorefineries, 2018, pp. 85–105 Search PubMed.
- V. H. Shiphrah, S. Sahu, A. R. Thakur and S. R. Chaudhuri, Screening of Bacteria for Lactic Acid Production from Whey Water, Am. J. Biochem. Biotechnol., 2013, 9(2), 118–123 CrossRef CAS.
- U. F. Umar Farooq, F. M. Anjum, T. Z. Tahir Zahoor, S. U. R. Sajjad-ur-Rahman, M. A. Randhawa, A. A. Anwaar Ahmed and K. A. Kashif Akram, Optimization of lactic acid production from cheap raw material: sugarcane molasses, Pak. J. Bot., 2012, 44(1), 333–338 Search PubMed.
- M. Alexandri, R. Schneider, K. Mehlmann and J. Venus, Recent advances in d-lactic acid production from renewable resources: case studies on agro-industrial waste streams, Food Technol. Biotechnol., 2019, 57(3), 293–304 CrossRef CAS PubMed.
- T. Ghaffar, M. Irshad, Z. Anwar, T. Aqil, Z. Zulifqar, A. Tariq and S. Mehmood, Recent trends in lactic acid biotechnology: a brief review on production to purification, J. Radiat. Res. Appl. Sci., 2014, 7(2), 222–229 CAS.
- K. Y. Lee, J. S. So and T. R. Heo, Thin layer chromatographic determination of organic acids for rapid identification of bifidobacteria at genus level, J. Microbiol. Methods, 2001, 45(1), 1–6 CrossRef CAS PubMed.
- J. R. Xavier and K. V. Ramana, Optimization of levan production by cold-active Bacillus licheniformis ANT 179 and fructooligosaccharide synthesis by its levansucrase, Appl. Biochem. Biotechnol., 2017, 181, 986–1006 CrossRef CAS PubMed.
- J. R. Xavier, M. M. Madhan Kumarr, G. Natarajan, K. V. Ramana and A. D. Semwal, Optimized production of poly (γ-glutamic acid)(γ-PGA) using Bacillus licheniformis and its application as cryoprotectant for probiotics, Biotechnol. Appl. Biochem., 2020, 67(6), 892–902 CrossRef CAS PubMed.
- C. Sabater, L. Ruiz, S. Delgado, P. Ruas-Madiedo and A. Margolles, Valorization of Vegetable Food Waste and By-Products Through Fermentation Processes, Front. Microbiol., 2020, 11, 581997, DOI:10.3389/fmicb.2020.581997.
- E. Abedi and S. M. B. Hashemi, Lactic acid production - producing microorganisms and substrates sources-state of art, Heliyon, 2020, 6(10), e04974, DOI:10.1016/j.heliyon.2020.e04974.
- M. Umesh and D. K. Preethi, Fermentative Utilization of Fruit Peel Waste for Lactic Acid Production by Lactobacillus plantarum, Indian J. Appl. Res., 2014, 4(9), 449–451 Search PubMed.
- A. H. Jawad, A. F. M. Alkarkhi, O. C. Jason, A. Mat Easa and N. A. Nik Norulaini, Production of the lactic acid from mango peel waste – factorial experiment, J. King Saud Univ., Sci., 2013, 25, 39–45 CrossRef.
- C. H. Kotzamanidis, T. Roukas and G. Skaracis, Optimization of lactic acid production from beet molasses by Lactobacillus delbrueckii NCIMB 8130, World J. Microbiol. Biotechnol., 2002, 18, 441–448 CrossRef CAS.
- A. K. Srivastava, A. D. Tripathi, A. Jha, A. Poonia and N. Sharma, Production, optimization and characterization of lactic acid by Lactobacillus delbrueckii NCIM 2025 from utilizing agro-industrial byproduct (cane molasses), J. Food Sci. Technol., 2015, 52(6), 3571–3578, DOI:10.1007/s13197-014-1423-6.
- M. Ha, S. Kim, Y. Lee, M. Kim and S. Kim, Kinetics analysis of growth and lactic acid production in pH-controlled batch cultures of Lactobacillus casei KH-1 using yeast extract/corn steep liquor/glucose medium, J. Biosci. Bioeng., 2003, 96, 134–140 CrossRef CAS PubMed.
- M. Ilmen, K. Koivuranta, L. Ruohonen, P. Suominen and M. Penttila, Efficient production of (L) lactic acid from xylose by Pichia stipitis, Appl. Environ. Microbiol., 2007, 73, 117–123 CrossRef CAS PubMed.
- D. Sarkar and G. Paul, A study on optimization of lactic acid production from whey by Lactobacillus sp isolated from curd sample, Res. J. Life Sci., Bioinf., Pharm. Chem. Sci., 2019, 5(2), 816 CAS.
- J. Wang, J. Huang, H. Laffend, S. Jiang, J. Zhang, Y. Ning and S. Liu, Optimization of immobilized Lactobacillus pentosus cell fermentation for lactic acid production, Bioresour. Bioprocess., 2020, 7, 1–14 CrossRef.
- K. Hofvendahl and B. Hahn-Hagerdal, L-Lactic acid production from whole wheat flour hydrolyzate using strains of Lactobacilli and Lactococci, Enzyme Microb. Technol., 1997, 27, 301–307 CrossRef.
- J. Chen, J. Wang and H. Zheng, Characterization of Bifidobacterium apousia sp. nov., Bifidobacterium choladohabitans sp. nov., and Bifidobacterium polysaccharolyticum sp. nov., three novel species of the genus Bifidobacterium from honey bee gut, Syst. Appl. Microbiol., 2021, 44(5), 126247 CrossRef CAS PubMed.
- M. K. Zamanova, V. Glotova, T. Izhenbina, D. S. Krutas and V. Novikov, Simultaneous HPLC-UV determination of lactic acid, glycolic acid, glycolide, lactide and ethyl acetate in monomers for producing biodegradable polymers, Procedia Chem., 2014, 10, 244–251 CrossRef CAS.
- D. M. Bai, R. Ban, X. M. Zhao and Z. D. Hu, Determination of lactic acid in fermentation broth of Rhizopus oryzae by reversed-phase high performance liquid chromatography (RP-HPLC), Chin. J. Chromatogr., 2018, 6, 527–528 Search PubMed.
|
This journal is © The Royal Society of Chemistry 2024 |
Click here to see how this site uses Cookies. View our privacy policy here.