DOI:
10.1039/D3FO04035F
(Paper)
Food Funct., 2024,
15, 196-207
Effects of unfolding treatment assisted glycation on the IgE/IgG binding capacity and antioxidant activity of ovomucoid†
Received
21st September 2023
, Accepted 16th November 2023
First published on 4th December 2023
Abstract
Ovomucoid is the immune-dominant allergen in the egg white of hens. Due to its structure based on nine disulfide bonds as well as its resistance to heat and enzymatic hydrolysis, the allergenicity of this food protein is difficult to decrease by technological processes. We sought to reduce its allergenicity through the Maillard reaction. The unfolding of ovomucoid with L-cysteine-mediated reduction was used to increase accessibility to conformational and linear epitopes by modifying the secondary and tertiary structures of the allergen. Glycation with different saccharides revealed the beneficial effect of maltose glycation on the IgG-binding capacity reduction. By determining the better glycation conditions of unfolded ovomucoid, we produced ovomucoid with reduced IgE binding capacity due to the glycation sites (K17, K112, K129, and K164) on epitopes. Moreover, after simulated infant and adult gastrointestinal digestion, the unfolded plus glycated ovomucoid showed higher ABTS˙+ scavenging activity, O2˙− scavenging activity, ˙OH scavenging activity, Fe2+ chelating activity, and a FRAP value; in particular, for ˙OH scavenging activity, there was a sharp increase of more than 100%.
1. Introduction
Technological processing of foods induces physicochemical modifications in the protein structure that can affect their allergenic potential. Structural changes induced by food processing may generate an alteration of the conformational and linear IgE epitopes of food proteins that may reduce or enhance their allergenicity.1,2 Among the different technological approaches, thermal treatments, including autoclaving, frying, boiling, dry or wet roasting, blanching, and baking, have been associated with a reduction in food protein allergenic capacity.3,4
The Maillard reaction (MR) is a non-enzymatic glycation accelerated by heating based on the interaction between the free amino groups of proteins and the carbonyl moiety of a reducing sugar present in the food matrix. During food processing, the naturally occurring MR not only modifies the technological properties of foods including solubility, gelling capacity, stability, water retention, texture, and flavor,5–7 but also alters the allergenicity of food antigens. Apart from inducing structural changes, the MR can reduce their allergic capacity by masking linear IgE epitopes due to the conjugation of reactive sequences to the sugar chain as it has been largely studied for milk, egg and fish allergens.8–10
Hen's egg is a ubiquitous food in the human diet and an important cause of anaphylactic reactions that are associated with an increasing prevalence of egg allergy during childhood, which has reached up to 2%.11 Ovalbumin, lysozyme, and ovomucoid are the most allergenic proteins contained in hen's egg white.12 The MR has been reported to exert a significant impact on decreasing the allergenicity of ovalbumin and lysozyme. For example, Mao et al.13 glycated ovalbumin with galactose and found that its IgE binding capacity reduced to 30.6%. In fact, recent studies have revealed that the incubation of ovalbumin in the presence of reducing sugars not only modifies conformational IgE epitopes but also blocks linear IgE epitopes, reducing its IgE binding capacity.9 Furthermore, glycated ovalbumin led to a better balance and a less allergenic immune response in vivo.14 Similarly, glycation of lysozyme effectively suppressed the production of specific IgG and IgE in a mouse model of egg allergy.15
However, glycation of hen's egg white ovomucoid has been shown to increase the allergenicity of this protein by enhancing its IgE reactivity.16 On the other hand, genetically glycosylated ovomucoid revealed reduced allergenicity and was associated with the suppression of allergic responses in vivo.17 This evidence suggests that the efficacy of glycation could be limited by the physicochemical properties of this allergen including heat stability, a high ratio of glycosylation (20–25%), and its singular conformation stabilized by nine pairs of disulfide bonds that are involved in the preservation of the IgE-binding capacity of this allergen.18,19 In this work, we sought to determine whether a prior disruption of the ovomucoid disulfide bonds using L-cysteine may enhance the glycation of this allergen and modify its allergenicity. Moreover, the antioxidant properties of the ovomucoid samples before and after simulated infant and adult gastrointestinal digestion were also evaluated.
2. Materials and methods
2.1 Preparation, stabilization, and glycation of unfolded ovomucoid
A purified solution of ovomucoid (4 mg mL−1) was obtained as previously described by our group.12 Briefly, after the pretreatment by pH adjustment and centrifugation, egg white was loaded onto the CM-sepharose FF column, and ovomucoid was obtained in the first fraction by eluting with acetate buffer (pH 4.3). The ovomucoid sample was then unfolded by adding equal volumes of L-cysteine (Aladdin, Shanghai, China) to reach a final concentration of 1 mg mL−1 and 5 mg mL−1 (labeled as LCY1 and LCY5, respectively). To avoid refolding during conformational analyses, the treated ovomucoid was stabilized by alkylation as described by Hale et al.20 The alkylation cocktail and unfolded ovomucoid were mixed at a ratio of 90
:
100 in phosphate-buffered saline (PBS). After incubation at 37 °C for 60 min, the samples were treated with a vacuum concentrator (Thermo Fisher Scientific, Waltham, USA) and subjected to ultra-filtration with a 10 kDa cutoff ultrafiltration centrifuge tube (Merck-Millipore, Darmstadt, Germany). Protein concentration was determined using a bicinchoninic acid (BCA) commercial kit following the manufacturer's recommendations (Thermo Fisher Scientific, Waltham, USA).
Glycation was performed by using a solution of the unfolded ovomucoid at 1 mg mL−1 (LCY1) without alkylation combined with glucose, galactose, and maltose at different monosaccharide/lysine ratios (1
:
1, 3
:
1, 5
:
1, 10
:
1, and 20
:
1). The samples were incubated at different temperatures (60, 70, 80, 90, and 100 °C) and time points (4, 8, 12, 16, and 24 h). After heat treatment, the samples were immediately cooled and dialysed in order to remove excess sugar and L-cysteine. The dialysis was performed using the conventional dialysis bag method (with a 10 kDa molecular weight cutoff) at 4 °C. The whole dialysis period lasted for 24 h. A solution of heated and glycated ovomucoid underwent the same thermal process in the absence of L-cysteine to be used as a control. Each treatment was performed in triplicate.
2.2 Conformational analyses
Modifications in the secondary structure of unfolded and alkylated ovomucoid were determined by far-ultraviolet (far-UV) circular dichroism and protein surface hydrophobicity was determined using fluorescence spectra. A far-UV circular dichrograph (JASCO J810 spectropolarimeter, Jasco, Pfungstadt, Germany) was used to detect the secondary structure of the treated ovomucoid at RT. The scanning range was set at 190–240 nm, the optical diameter was 1.0 mm, the scanning speed was 60 nm min−1, the wideband slit width was 1 nm, and the protein sample concentration was 0.1 mg mL−1. The results represent the average of three consecutive scans.
The surface hydrophobicity of the treated ovomucoid was evaluated by measuring the fluorescence intensity of the protein in the presence of the fluorescent probe 8-phenylamino-1-naphthalenesulfonic acid (ANS). The relative fluorescence was determined with an F-2500 spectrometer (Hitachi, Tokyo, Japan). The excitation wavelength of the instrument was set at 375 nm and the emission wavelength ranged from 400 to 650 nm at a scanning speed of 1200 nm min−1 with a slit width of 5 nm and a response time of 0.5 s.
2.3 Simulated infant and adult gastrointestinal digestion
For the ovomucoid samples, simulated infant gastrointestinal digestion and simulated adult gastrointestinal digestion were performed according to Huang et al.,21 with minor modifications. The simulated infant gastric digestion was performed at pH 3.0, and the final pepsin activity was 3125 U mL−1. The simulated adult gastric digestion was performed at pH 2.0, and the final pepsin activity was 25
000 U mL−1. The simulated infant intestinal digestion and simulated adult intestinal digestion were performed at pH 6.5, and the digestion duration was set at 0, 15, 30 and 60 min.
After digestion, the samples were loaded on SDS-PAGE. The gel was made using the PAGE gel fast preparation kit (12.5%, Shanghai Epizyme Biomedical Technology). Electrophoretic separation was performed at 80 V for 30 min and then at 120 V until the end.
2.4 Antioxidant activities of ovomucoid before and after digestion
2.4.1 ABTS˙+ scavenging activity.
The experiment was performed according to Lu et al.,22 with minor modifications. The ovomucoid samples (50 μL, diluted to 0.2, 0.4, and 0.8 mg mL−1) were added with ABTS radical solution (150 μL, adjusted to an OD value of around 0.7 ± 0.02 at 734 nm). The absorbance of the samples was measured at 734 nm using a microplate reader (Thermo Fisher Scientific, USA). The ABTS˙+ scavenging activity was calculated as follows:
where AS is the absorbance of the sample group and A0 is the absorbance of the control group (distilled water instead of the sample).
2.4.2 O2˙− scavenging activity.
The experiment was performed according to Ge et al.,23 with minor modifications. Sample solutions (75 μL, diluted to 0.4, 0.6, and 0.8 mg mL−1) were added to a 96-well plate, and then 75 μL of Tris-HCl buffer solution (50 mmol L−1, pH 8.3) was added. After storage at 25 °C for 10 minutes, pyrogallic acid (50 μL, 1.5 mmol L−1) was added and reacted for 5 minutes. The absorbance value was measured at 320 nm using a microplate reader, and cysteine was used as a positive control. The O2˙− scavenging activity was calculated as follows:
A(%) = (A0 − A1)/(A0 − A2) |
where A1 is the absorbance of the sample group, A2 is the absorbance of the positive control group, and A0 is the absorbance of the control group (distilled water instead of the sample).
2.4.3 ˙OH scavenging activity.
The experiment was performed according to Ge et al.,23 with minor modifications. Sample solutions (50 μL, diluted to 0.2, 0.4, and 0.8 mg mL−1) were added to a 96-well plate, and then FeSO4 solution (50 μL, 6 mmol L−1) and H2O2 solution (50 μL, 6 mmol L−1) were sequentially added. After incubating at 37 °C for 10 min, ethanol salicylic acid solution (50 μL, 6 mmol L−1) was added and incubated at 37 °C for 30 min. The absorbance value was measured at 563 nm using a microplate reader. The ˙OH scavenging activity was calculated as follows:
where AS is the absorbance of the sample group and A0 is the absorbance of the control group (distilled water instead of the sample).
2.4.4 Fe2+ chelating activity.
The experiment was performed according to Lin et al.,24 with some modifications. Sample solutions (25 μL, diluted to 0.2, 0.4, and 0.8 mg mL−1), FeCl2 solution (15 μL, 0.5 mmol L−1), and ultrapure water (110 μL) were mixed and incubated at room temperature for 2 min. Then, Ferrozine reaction liquid (110 μL) was added into the mixture and reacted for 10 min at room temperature. The absorbance value was measured at 562 nm using a microplate reader. Fe2+ chelating activity was calculated as follows:
where AS is the absorbance of the sample group and A0 is the absorbance of the control group (distilled water instead of the sample).
2.4.5 Ferric reducing antioxidant power (FRAP).
The experiment was performed according to Ge et al.,23 with minor modifications. Sample solutions (5 μL, diluted to 0.4, 0.8, and 1.6 mg mL−1) and standards were added to a 96-well plate, and the FRAP working solution (250 μL) was added to each well and incubated at 37 °C for 15 min. The absorbance value was measured at 563 nm using a microplate reader. FRAP values were calculated using the standard curve.
2.5 Ovomucoid-specific polyclonal IgG and human IgE binding capacity
Polyclonal IgG antibodies were produced as previously described by our group,25 with minor modifications. 8-week-old New Zealand rabbits (permission number “SCXK(Yu) 2017-0010”) were subcutaneously injected with 1 mg of ovomucoid (purity >95%) in 1 mL of saline emulsified with an equal amount of Freund's complete adjuvant (Sigma-Aldrich, St Louis, USA) and boosters were carried out at a 2-week interval. Antisera were collected by centrifugation and stored at −80 °C (for the titre change during the immunization process, see Fig. S1†). An indirect ELISA was used to determine the binding ability of the treated samples to the ovomucoid-specific IgG antibodies. In brief, plates were coated with processed ovomucoid (50 μg mL−1), blocked, and rabbit polyclonal sera diluted at 1
:
100
000 were used as the primary antibody. Detection was performed with a goat anti-rabbit IgG antibody (HRP-labeled) diluted at 1
:
5000. Inhibition ELISA was used to determine the IC50 of ovomucoid glycated under optimized conditions, see Ma et al.25
Sera from ten egg-allergic subjects were provided by the Second Affiliated Hospital of Zunyi Medical University (Zunyi, China). The allergic individuals included five males and five females with egg white-specific IgE levels of 0.75, 0.83, 2.5, 0.87, 1.3, 1.3, 51.8, 1.3, 9.7, and 6.6 kU L−1. Sera were equally mixed to create an active pool (for the IgE binding capacity of unfolded ovomucoid, the former eight serum samples were used, and the other tests used all the ten serum mixtures). As a negative control, a pool of five non-allergic donors was prepared. Pooled sera were aliquoted and stored at −80 °C. The ovomucoid-specific IgE binding capacity of the treated samples was determined by an indirect ELISA as reported by Ma et al.,25 with minor modifications. Briefly, the plates were coated with processed ovomucoid (50 μg mL−1), blocked, and pooled sera diluted at 1
:
10 were used as the primary antibody. Detection was performed with a biotin-conjugated goat anti-human IgE antibody (Merck-Sigma-Aldrich, Darmstadt, Germany) diluted at 1
:
2000, and (HRP)-streptavidin (Neo Bioscience, China) was added and incubated at 37 °C for 1 h.
2.6 Identification of glycated sites
Glycated ovomucoid with and without unfolding pre-treatment was digested with trypsin (50 ng mL−1, in 100 mM NH3HCO3) at 37 °C for 16 h prior to HPLC-MS/MS acquisition. HPLC-MS/MS analyses were performed on an UltiMate 3000 RSLC nano system with an RP-C18 Acclaim PepMap RSLC column (75 m × 15 cm) connected online to Orbitrap Fusion equipment (all from Thermo Fisher Scientific, Waltham, USA). The mobile phase consisted of solvent A (0.1% TFA in Milli-Q water) and solvent B (0.1% TFA in HPLC grade acetonitrile). The gradient used was as follows: 0–6 min, 4% solvent B; 6–50 min, 4%–25% solvent B; 50–58 min, 25%–40% solvent B; 58–61 min, 40%–85% solvent B; and 61–66 min, 85% solvent B. Mass spectra were recorded over the range 350–1800 m/z and Proteome Discoverer software (version 1.4, Thermo Fisher Scientific) was used to process the MS(n) spectra and perform peptide sequencing.
2.7 Statistical analysis
All the experiments were carried out in triplicate and the results are presented as mean value ± standard deviation (SD). Statistical analyses were performed using SPSS 18.0 (IBM, USA). One-way ANOVA (post-hoc comparisons used the LSD test) was used for all analyses except the glycation condition experiment. In the glycation condition experiment, the t-test was applied to compare the point with the lowest IgG binding capacity with every other point. Data were considered statistically significant for P values less than 0.05.
3. Results and discussion
3.1 Unfolding of ovomucoid reduces its IgG binding capacity but maintains its IgE reactivity
The effect of unfolding with L-cysteine and stabilization by alkylation on the secondary structure of ovomucoid was characterized by circular dichroism. Fig. 1A shows that the α-helix structure (positive peak at 190 nm) was reduced after the unfolding induced by L-cysteine, and the treatment with the lowest concentration (1 mg mL−1, LCY1) induced a more remarkable change in this structure. The β-sheet structure (negative peak at 215 nm) was enhanced after unfolding at both concentrations assayed (LCY1 and LCY5), and LCY1 also induced a more remarkable change. The surface hydrophobicity changes generated after the L-cysteine reduction treatments revealed that LCY5 had a higher hydrophobicity when compared with the untreated sample, while LCY1 showed a lower hydrophobicity than the untreated protein, and LCY1 induced a more remarkable change than LCY5 (Fig. 1B). These results indicated that the secondary structure and hydrophobicity of ovomucoid were modified after the treatment with L-cysteine at both concentrations assayed, suggesting an effective unfolding of this protein, especially for LCY1. L-cysteine has been previously used as a strategy for modifying the secondary structure of food proteins with similar results including the cleavage of the disulfide bridge26,27 and the reshuffling of these bonds.28
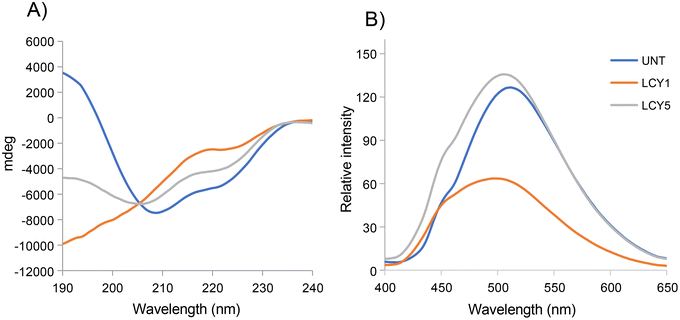 |
| Fig. 1 Secondary structure (A) and hydrophobicity (B) of ovomucoid untreated (UNT) and unfolded with L-cysteine (LCY) at 1 mg mL−1 (LCY1) or 5 mg mL−1 (LCY5). The secondary structure was determined by far-ultraviolet (far-UV) circular dichroism and the protein surface hydrophobicity was determined using fluorescence spectra. The results represent the average of three consecutive scans. | |
In order to determine whether the modification of the secondary structure of ovomucoid could reduce its allergenicity, we studied the IgG and IgE binding capacities of unfolded ovomucoid. Treatment with L-cysteine at a concentration of 1 mg mL−1 (LCY1) significantly reduced the IgG binding capacity of the allergen compared not only with the untreated protein but also with LCY5. Surprisingly, unfolding with L-cysteine at a concentration of 5 mg mL−1 (LCY5) significantly increased the IgG binding capacity compared with the untreated ovomucoid (Fig. 2A). Our results are consistent with those reported in a recent study that used a pulsed electric field treatment for the disruption of the secondary structure of ovomucoid.29 In this work, the authors showed that a certain extensive unfolding treatment of ovomucoid (40 kV cm−1) resulted in the reconstitution of the IgG binding capacity to similar levels to those found in non-treated ovomucoid. In addition, we found that the unfolding of ovomucoid with L-cysteine treatments significantly increased the IgE binding capacity compared with the untreated protein (Fig. 2B). In agreement with our results, the unfolding induced by the glutathione reduction of ovomucoid exposed sequential epitopes, thus increasing its IgE reactivity.30 Modification of the ovomucoid structure by other treatments such as heating has also been related to an increased IgE binding capacity.16
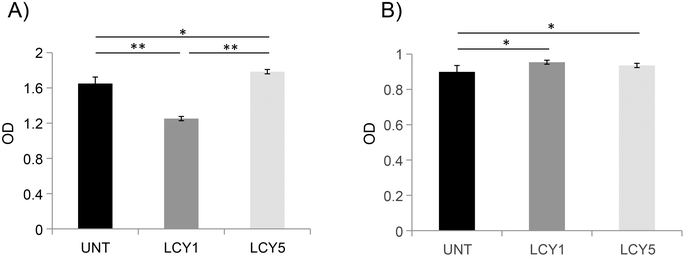 |
| Fig. 2 IgG- (A) and IgE- (B) binding capacity of ovomucoid untreated (UNT) and unfolded with L-cysteine (LCY) at 1 mg mL−1 (LCY1) or 5 mg mL−1 (LCY5). n = 3 for each sample. For the IgG binding capacity test, rabbit anti-ovomucoid sera prepared by our group were used as the primary antibody, and a goat anti-rabbit IgG antibody was used as the secondary antibody. For the IgE binding capacity test, sera from 8 egg allergic subjects (with egg white-specific IgE levels of 0.75, 0.83, 2.5, 0.87, 1.3, 1.3, 51.8, and 1.3 kU L−1) were mixed to prepare a serum pool as the primary antibody, and a biotin-conjugated goat anti-human IgE antibody was used as the secondary antibody. | |
In conclusion, we have shown that treatment with L-cysteine at a concentration of 1 mg mL−1 (LCY1) disrupts the secondary structure of this allergen, reducing its capacity to bind ovomucoid-specific IgG. These results also suggest that the unfolding induced may contribute to exhibiting linear IgE epitopes that might be masked by their conjugation with reducing sugars following a glycation treatment.
3.2 Glycation of unfolded ovomucoid with maltose reduces its allergenicity
We first determined the best sugar candidate to generate an effective MR that efficiently reduces the antigenicity of LCY1-unfolded ovomucoid. The monosaccharides glucose and galactose, as well as the disaccharide maltose, were used for the glycation reactions (60 °C for 1 h, a saccharide
:
lysine ratio of 3
:
1), and the immune reactivity of the glycated samples was evaluated based on their IgG binding capacity. Compared to glucose- and galactose-mediated glycation, treatment with maltose significantly reduces the IgG binding capacity of ovomucoid unfolded with 1 mg mL−1 of L-cysteine (Fig. 3A).
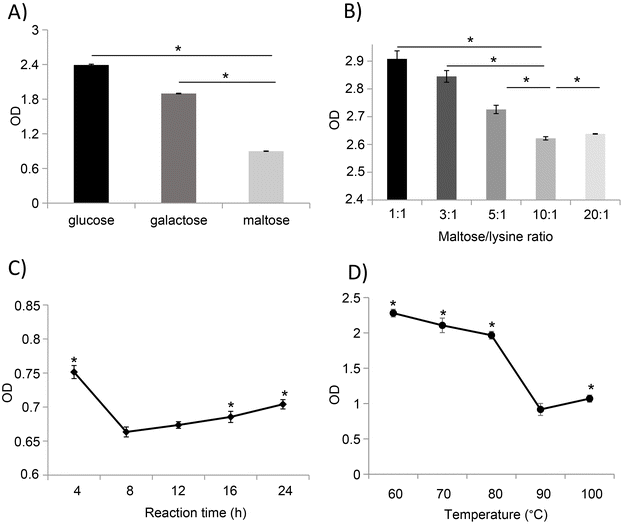 |
| Fig. 3 Optimization of the treatment conditions for the reduction of the IgG binding capacity of ovomucoid unfolded with L-cysteine (1 mg mL−1). n = 3 for each sample. (A) Glycation with glucose, galactose, and maltose. *P < 0.05 vs. the maltose group. (B) Glycation with different ratios of maltose : lysine residues. *P < 0.05 vs. the 10 : 1 group. (C) Glycation at different times of reaction. *P < 0.05 vs. the 8 h group. (D) Glycation at different temperatures. Except for the conditions studied, the other conditions were set at maltose : lysine residues at 3 : 1, and reacted at 60 °C for 60 min. *P < 0.05 vs. the 90 °C group. | |
Based on the results observed in LCY1-unfolded ovomucoid by maltose glycation, we determined the optimal conditions including the protein concentration, time, and temperature for an enhanced reduction of ovomucoid allergenicity. Our results revealed that the lowest ovomucoid-specific IgG binding capacity was achieved at a ratio of 10
:
1 (maltose
:
lysine residue) (Fig. 3B) and a reaction time of 8 h (Fig. 3C). In addition, we found that the MR with maltose performed at 90 °C strongly reduced the antigenicity of ovomucoid compared with other temperatures (Fig. 3D). Together, our results revealed that unfolded ovomucoid with 1 mg mL−1 of L-cysteine subjected to a glycation treatment with maltose at a ratio of 10
:
1 (maltose
:
lysine residue) at 90 °C for 8 h drastically reduced the IgG binding capacity compared with the untreated allergen.
Under these optimized conditions, the IgG and IgE reactivity of ovomucoid glycated after L-cysteine unfolding was significantly reduced compared with the untreated, heated and glycated control (Fig. 4). In agreement with our results, recent studies have shown that the substrate concentration, reaction time, and temperature are independent variables that remarkably affect the MR of food proteins.31,32 Despite glycation having been shown to increase the allergenicity of ovomucoid by enhancing IgE reactivity,16 we have determined an optimized protocol for its efficient reduction. Under the optimized conditions, the IgE reactivity of the processed ovomucoid was lower than that using other processing methods reported, which reduced it to about 60% and 70% of the original value, respectively.18,33
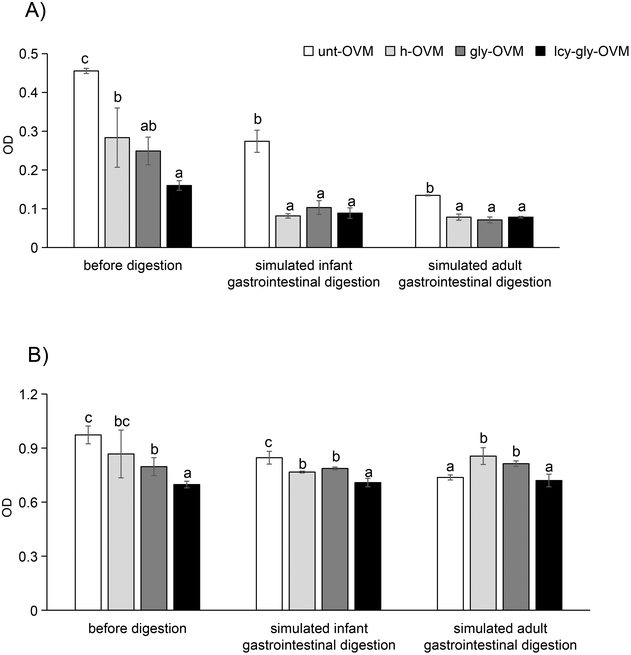 |
| Fig. 4 IgG-binding capacity (A) and IgE-binding capacity (B) of ovomucoid untreated (unt-OVM), heated for 8 h at 90 °C (h-OVM), glycated for 8 h at 90 °C (gly-OVM), and L-cysteine-unfolded and glycated for 8 h at 90 °C (LCY-gly-OVM). n = 3 for each sample. Different letters in the figure mean P < 0.05. For the IgG binding capacity test, rabbit anti-ovomucoid sera prepared by our group were used as the primary antibody, and the goat anti-rabbit IgG antibody was used as the secondary antibody. For the IgE binding capacity test, sera from 8 egg allergic subjects (with egg white-specific IgE levels of 0.75, 0.83, 2.5, 0.87, 1.3, 1.3, 51.8, 1.3, 9.7 and 6.6 kU L−1) were mixed to prepare a serum pool as the primary antibody, and the biotin-conjugated goat anti-human IgE antibody was used as the secondary antibody. | |
Considering the fact that L-cysteine unfolded the ovomucoid molecule but the induced structural change did not reduce its IgE binding capacity, we speculated that its IgE reactivity reduction after glycation under the optimized conditions was due to sugar conjugation on its linear epitopes. The specific glycation sites of the ovomucoid after the optimized treatment were determined by HPLC-MS/MS. The results revealed that previously LCY1-unfolded ovomucoid had four sites of glycation (K17, K112, K129, and K164), and they were all on lysine (K) residues (Table 1). These glycation sites are at least on one of the reported ovomucoid IgE epitopes, thus contributing to its IgE binding capacity reduction (see the reported epitope chart for ovomucoid in Fig. 5). By comparison, the ovomucoid glycated under the same conditions without unfolding has only two glycation sites, K129 and K164 (data not shown), which explains why glycated ovomucoid also reduces IgE binding capacity, while the reduction rate was not that much compared with the unfolded and glycated sample.
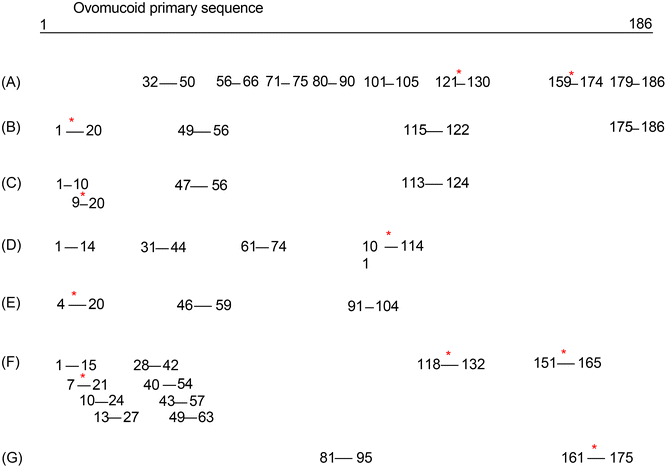 |
| Fig. 5 Reported epitopes for ovomucoid. * stands for the specific glycation sites of the ovomucoid after the optimized treatment located on the epitopes. [(A) Ref. 34; (B) ref. 35; (C) ref. 36; (D). ref. 37; (E) ref. 38; (F) ref. 39; (G) ref. 40]. | |
Table 1 Glycation sites of L-cysteine-unfolded ovomucoid glycated with maltose (ratio 10
:
1, maltose
:
lysine residues) for 8 h at 90 °C by using HPLC-MS/MS
No. |
St. |
End |
Sequence |
Glycated site |
1 |
8 |
24 |
FPNATDKEGKDVLVCNK |
K17 |
2 |
90 |
121 |
AFNPVCGTDGVTYDNECLLCAHKVEQGASVDK |
K112 |
3 |
129 |
159 |
KELAAVSVDCSEYPKPDCTAEDRPLCGSDNK |
K129 |
4 |
160 |
185 |
TYGNKCNFCNAVVESNGTLTLSHFGK |
K164 |
Subsequently, we investigated whether the IgG and IgE reactivity of ovomucoid is still reduced after simulated infant and adult gastrointestinal digestion. It is clear from Fig. 6A and C that all ovomucoid samples can resist pepsin digestion even for 60 min. After simulated infant intestinal digestion for 60 min and simulated adult intestinal digestion for 15 min, the treated ovomucoid band (regardless of whether heated, glycated, or glycated after L-cysteine unfolding) had almost disappeared (Fig. 6B and D), although the untreated ovomucoid still resisted digestion. Benedé et al.41 found that ovomucoid is digestion enzyme resistant; even after heating it at 65 °C for 30 min and 90 °C for 3 min, it still resists gastrointestinal digestion. In the current work, the treated ovomucoid can be digested, quite probably because we heated it at 90 °C for a much longer duration, which makes ovomucoid less stable. After simulated infant and adult gastrointestinal digestion, the IgG binding capacity of ovomucoid glycated after L-cysteine-assisted unfolding is similar to that of the heated and glycated control, while its IgE reactivity is still reduced.
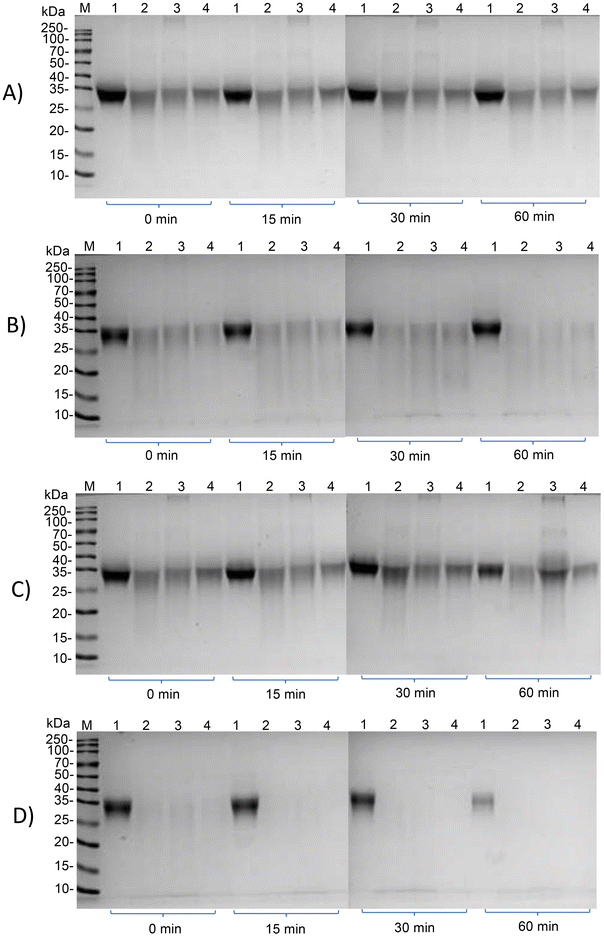 |
| Fig. 6 SDS-PAGE results of the ovomucoid samples before and after simulated infant and adult gastrointestinal digestion. M stands for the marker, lanes 1–4 stand for untreated ovomucoid, ovomucoid heated for 8 h at 90 °C, ovomucoid glycated for 8 h at 90 °C and L-cysteine-unfolded ovomucoid glycated for 8 h at 90 °C, respectively. (A) Ovomucoid samples after simulated infant gastric digestion for 0, 15, 30 and 60 min. (B) Ovomucoid samples after simulated infant gastric digestion for 60 min, followed by simulated infant intestinal digestion for 0, 15, 30 and 60 min. (C) Ovomucoid samples after simulated adult gastric digestion for 0, 15, 30 and 60 min. (D) Ovomucoid samples after simulated adult gastric digestion for 60 min, followed by simulated adult intestinal digestion for 0, 15, 30 and 60 min. | |
3.3 Glycation of unfolded ovomucoid has increased antioxidant activity after digestion
The oxidative process in the food industry is challenging as it affects proteins, lipids, and pigments and thus shortens the shelf life of food products. Research has proven that the addition of antioxidants, either naturally contained in foods or generated during food processing, can delay these deleterious processes.42,43 Therefore, after the potential allergenicity detection, we verified whether the optimized conditions of unfolding and maltose-mediated glycation in the reduction of ovomucoid IgE binding capacity could affect the antioxidant properties of ovomucoid. Considering that L-cysteine is an antioxidant, we ensured that dialysis removed excess L-cysteine from the samples before the antioxidant activity detection. A 10 kDa ultrafiltration tube was used and after general centrifugation, liquid outside the tube was collected. Its ABTS˙+ scavenging activity was detected, which was not statistically different from the blank control (data not shown). Thus the interference of L-cysteine was eliminated.
We found that the treatment did not increase the antioxidant activity of ovomucoid. In contrast, after simulated infant and adult gastrointestinal digestion, the ABTS˙+ scavenging activity, O2˙− scavenging activity, ˙OH scavenging activity, Fe2+ chelating activity, and the FRAP value all increased (P < 0.05), compared with the untreated, heated, and glycated control (Fig. 7). In particular, for the ˙OH scavenging activity, the increase was more than 100%. The ABTS˙+ scavenging activity, O2˙− scavenging activity, ˙OH scavenging activity, Fe2+ chelating activity, and the FRAP value are the indexes frequently used to describe the antioxidant activity changes of the food constituents. These methods share the same principle: after generating a redox-active compound or a synthetic colored radical, the samples are added to scavenge the radical or to reduce the redox-active compound, and the corresponding color changes are monitored by a spectrophotometer.44 However, although these methods employ the same principle, multiple methods are recommended to better reflect the antioxidant reactivity of food constituents. Moreover, these methods have their own advantages or focus. For example, the ABTS˙+ scavenging assay is rapid and can be used over a wide pH range. The ˙OH scavenging activity attracts attention because the ˙OH radicals are the most active among reactive oxygen species, which have the strongest toxicity to the body. The results in this work indicate that all five indexes that describe the antioxidant activity of ovomucoid digests were significantly improved after unfolding and glycation, which fully explains the increase in its antioxidant activity.
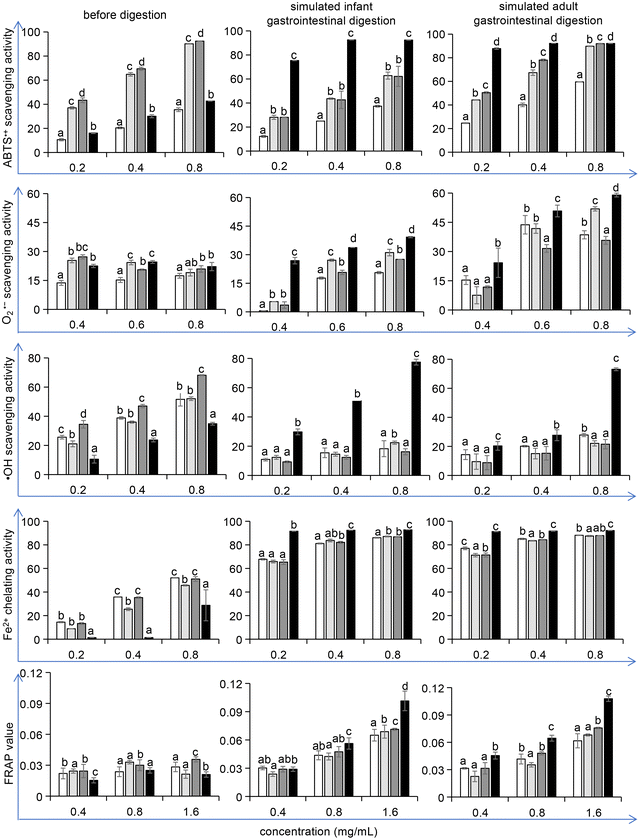 |
| Fig. 7 Antioxidant ability of the ovomucoid samples before and after simulated infant and adult gastrointestinal digestion (simulated gastric digestion was performed for 60 min and then simulated intestinal digestion was performed for 60 min). untreated ovomucoid; ovomucoid heated for 8 h at 90 °C; ovomucoid glycated for 8 h at 90 °C; L-cysteine-unfolded ovomucoid glycated for 8 h at 90 °C. (A) ABTS˙+ scavenging activity, tested by the addition of ABTS radical solution and detected at 734 nm. (B) O2˙− scavenging activity, tested by the addition of pyrogallic acid and detected at 320 nm. (C) ˙OH scavenging activity, tested by the addition of FeSO4 solution, H2O2 solution, and ethanol salicylic acid solution, and detected at 563 nm. (D) Fe2+ chelating activity, tested by the addition of FeCl2 solution and Ferrozine reaction liquid, and detected at 562 nm. (E) FRAP value, tested by the addition of FRAP working solution, and detected at 563 nm. Different letters in the figure mean P < 0.05. | |
In recent years, hydrolysates of major egg proteins like ovalbumin, ovotransferrin, and lysozyme have been reported to have antioxidant properties.45–47 However, for ovomucoid, research focused on its allergenicity, and seldom any attention has been paid to its antioxidant activity. This work demonstrates for the first time that after unfolding, glycation and simulated digestion, the ovomucoid peptides can possess increased antioxidant activity.
4. Conclusion
In conclusion, we have determined an optimized protocol to glycate the hen's egg white allergen ovomucoid that efficiently reduces IgE binding capacity even after simulated digestion. It consists of a pre-unfolding treatment with L-cysteine followed by an MR with maltose at 90 °C for 8 h. Moreover, the digested peptide possesses increased antioxidant activity. Although in vivo experiments that verify our results are needed to draw a sound conclusion, this work provides new insight into the potential strategies to generate hypoallergenic egg products with higher antioxidant activity.
Author contributions
Conceptualization, X. J. M.; investigation, X. X. and R. L; writing – original draft, D. L. and X. X.; visualization, Y. L. and J. D. L.; writing – review and editing, X. J. M. and Y. Y. All authors have read and agreed to the published version of the manuscript.
Conflicts of interest
The authors declare that they have no known competing financial interests or personal relationships that could have appeared to influence the work reported in this paper.
Acknowledgements
The work was supported by the National Natural Science Foundation of China (Grant number 31660443), the Young Talents Program of Zunyi Medical College (Grant number 18zy-009), the Guizhou Province Science and Technology Projects (Grant number 2019-1348), and the Guizhou Health and Family Planning Project (Grant number D-488).
References
- D. Bogahawaththa, R. Ashraf, J. Chandrapala, O. Donkor and T. Vasiljevic, In vitro immunogenicity of various native and thermally processed bovine milk proteins and their mixtures, J. Dairy Sci., 2018, 101, 8726–8736 CrossRef CAS.
- J. Gou, R. Liang, H. Huang and X. Ma, Maillard Reaction induced changes in allergenicity of food, Foods, 2022, 11, 530 CrossRef CAS PubMed.
- M. Faisal, T. Vasiljevic and O. N. Donkor, Effects of selected processing treatments on antigenicity of banana prawn (Fenneropenaeus merguiensis) tropomyosin, Int. J. Food Sci. Technol., 2019, 54, 183–193 CrossRef CAS.
- J. M. Rolland, N. P. Varese, J. B. Abramovitch, J. Anania, R. Nugraha, S. Kamath, A. Hazard, A. L. Lopata and R. E. O'Hehir, Effect of heat processing on IgE reactivity and cross-reactivity of tropomyosin and other allergens of Asia-Pacific mollusc species: identification of novel sydney rock oyster tropomyosin Sac g 1, Mol. Nutr. Food Res., 2018, 62, e1800148 CrossRef PubMed.
- J. M. Bühler, B. L. Dekkers, M. E. Bruins and A. J. van der Goot, Modifying faba bean protein concentrate using dry heat to increase water holding capacity, Foods, 2020, 9, 1077 CrossRef.
- G. Liu, D. Yuan, Q. Wang, W. Li, J. Cai, S. Li, O. Lamikanra and X. Qin, Maillard-reaction-functionalized egg ovalbumin stabilizes oil nanoemulsions, J. Agric. Food Chem., 2018, 66, 4251–4258 CrossRef CAS PubMed.
- Y. Song, S. Yang and J. Li, Effect of Maillard reaction conditions on the solubility and molecular properties of wheat gluten-maltose conjugates, Food Sci. Nutr., 2020, 8, 5898–5906 CrossRef CAS PubMed.
- X. M. Wang, Y. H. Ye, Z. C. Tu, Y. M. Hu, H. Wang and T. Huang, Mechanism of the reduced IgG/IgE binding abilities of glycated β-lactoglobulin and its digests through high-resolution mass spectrometry, J. Agric. Food Chem., 2021, 69, 3741–3750 CrossRef CAS.
- Y. Yang, G. Liu, Z. Tu, H. Wang, Y. Hu, J. Mao and J. Zhang, Insight into the mechanism of reduced IgG/IgE binding capacity in ovalbumin as induced by glycation with monose epimers through liquid chromatography and high-resolution mass spectrometry, J. Agric. Food Chem., 2020, 68, 6065–6075 CrossRef CAS.
- M. Zhang, Z. C. Tu, J. Liu, Y. M. Hu, H. Wang, J. H. Mao and J. L. Li, The IgE/IgG binding capacity and structural changes of Alaska Pollock parvalbumin glycated with different reducing sugars, J. Food Biochem., 2021, 45, e13539 CAS.
- X. Ma, R. Liang, Q. Xing and D. Lozano-Ojalvo, Can food processing produce hypoallergenic egg?, J. Food Sci., 2020, 85, 2635–2644 CrossRef CAS.
- X. Ma, R. Liang, X. Yang, J. Gou, Y. Li and D. Lozano-Ojalvo, Simultaneous separation of the four major allergens of hen egg white, J. Chromatogr. B: Anal. Technol. Biomed., 2020, 1152, 122231 CrossRef CAS PubMed.
- J. H. Mao, K. Zhang, Y. F. He, J. Liu, Y. H. Shao and Z. C. Tu, Molecular structure, IgE binding capacity and gut microbiota of ovalbumin conjugated to fructose and galactose:A comparative study, Int. J. Biol. Macromol., 2023, 234, 123640 CrossRef CAS PubMed.
- P. Rupa and Y. Mine, Comparison of glycated ovalbumin–monosaccharides in the attenuation of ovalbumin-induced allergic response in a BALB/C mouse model, J. Agric. Food Chem., 2019, 67, 8138–8148 CrossRef CAS PubMed.
- K. Arita, E. E. Babiker, H. Azakami and A. Kato, Effect of chemical and genetic attachment of polysaccharides to proteins on the production of IgG and IgE, J. Agric. Food Chem., 2001, 49, 2030–2036 CrossRef CAS PubMed.
- R. Jiménez-Saiz, J. Belloque, E. Molina and R. López-Fandiño, Human immunoglobulin E (IgE) binding to heated and glycated ovalbumin and ovomucoid before and after in vitro digestion, J. Agric. Food Chem., 2011, 59, 10044–10051 CrossRef.
- P. Rupa, S. Nakamura and Y. Mine, Genetically glycosylated ovomucoid third domain can modulate Immunoglobulin E antibody production and cytokine response in BALB/c mice, Clin. Exp. Allergy, 2007, 37, 918–928 CrossRef CAS.
- P. W. Wen, Z. C. Tu, Y. M. Hu and H. Wang, Effects of superheated steam treatment on the allergenicity and structure of chicken egg ovomucoid, Foods, 2022, 11, 238 CrossRef CAS PubMed.
- N. Stănciuc, A. A. Creţu, I. Banu and I. Aprodu, Advances on the impact of thermal processing on structure and antigenicity of chicken ovomucoid, J. Sci. Food Agric., 2018, 98, 3119–3128 CrossRef.
- J. E. Hale, J. P. Butler, V. Gelfanova, J. S. You and M. D. Knierman, A simplified procedure for the reduction and alkylation of cysteine residues in proteins prior to proteolytic digestion and mass spectral analysis, Anal. Biochem., 2004, 333, 174–181 CrossRef CAS PubMed.
- M. Huang, H. Tan, Z. Xiong, W. Hu, Y. Wu, X. Meng, H. Chen and X. Li, Allergenicity evaluation of peptides from milk and yogurt after gastrointestinal digestion based on epitopes, Food Funct., 2022, 13, 10769–10789 RSC.
- X. Lu, L. Zhang, Q. Sun, G. Song and J. Huang, Extraction, identification and structure-activity relationship of antioxidant peptides from sesame (Sesamum indicum L.) protein hydrolysate, Food Res. Int., 2019, 116, 707–716 CrossRef CAS PubMed.
- X. Ge, L. Jing, K. Zhao, C. Su, B. Zhang, Q. Zhang, L. Han, X. Yu and W. Li, The phenolic compounds profile, quantitative analysis and antioxidant activity of four naked barley grains with different color, Food Chem., 2021, 335, 127655 CrossRef CAS.
- J. Lin, H. Hong, L. Zhang, C. Zhang and Y. Luo, Antioxidant and cryoprotective effects of hydrolysate from gill protein of bighead carp (Hypophthalmichthys nobilis) in preventing denaturation of frozen surimi, Food Chem., 2019, 298, 124868 CrossRef CAS PubMed.
- X. J. Ma, H. B. Chen, J. Y. Gao, C. Q. Hu and X. Li, Conformation affects the potential allergenicity of ovalbumin after heating and glycation, Food Addit. Contam., Part A: Chem., Anal., Control, Exposure Risk Assess., 2013, 30, 1684–1692 CrossRef CAS PubMed.
- B. Goldenbogen, N. Brodersen, A. Gramatica, M. Loew, J. Liebscher, A. Herrmann, H. Egger, B. Budde and A. Arbuzova, Reduction-sensitive liposomes from a multifunctional lipid conjugate and natural phospholipids: reduction and release kinetics and cellular uptake, Langmuir, 2011, 27, 10820–10829 CrossRef CAS PubMed.
- A. E. M. Beedle, M. Mora, S. Lynham, G. Stirnemann and S. Garcia-Manyes, Tailoring protein nanomechanics with chemical reactivity, Nat. Commun., 2017, 8, 15658 CrossRef CAS PubMed.
- N. Yang, S. Qian, Z. Jiang and J. Hou, Cysteine inducing formation and reshuffling of disulfide bonds in cold-extruded whey protein molecules: From structural and functional characteristics to cytotoxicity, Food Chem., 2021, 360, 130121 CrossRef CAS PubMed.
- Y. Li, S. Zhang, P. Jiang, L. Zhong, S. Lin and N. Sun, Exploration of structure-activity relationship between IgG1 and IgE binding ability and spatial conformation in ovomucoid with pulsed electric field treatment, LWT-Food Sci. Technol., 2021, 14, 110891 CrossRef.
- F. Roth-Walter, P. Starkl, T. Zuberbier, K. Hummel, K. Nöbauer, E. Razzazi-Fazeli, R. Brunner, I. Pali-Schöll, J. Kinkel, F. Felix, E. Jensen-Jarolim and T. Kinaciyan, Glutathione exposes sequential IgE-epitopes in ovomucoid relevant in persistent egg allergy, Mol. Nutr. Food Res., 2013, 57, 536–544 CrossRef CAS PubMed.
- P. Gao, W. Xia, X. Li and S. Liu, Optimization of the Maillard reaction of xylose with cysteine for modulating aroma compound formation in fermented tilapia fish
head hydrolysate using response surface methodology, Food Chem., 2020, 331, 127353 CrossRef CAS.
- T. D. Capar and H. Yalcin, Protein/polysaccharide conjugation via Maillard reactions in an aqueous media: Impact of protein type, reaction time and temperature, LWT-Food Sci. Technol., 2021, 152, 112252 CrossRef.
- J. Ma, J. Zhou, L. Chen, H. Zhang, Y. Wang and L. Fu, Effects of deglycosylation and the Maillard reaction on conformation and allergenicity of the egg ovomucoid, J. Food Sci., 2021, 86, 3014–3022 CrossRef CAS PubMed.
- Y. Mine and J. Wei Zhang, Identification and fine mapping of IgG and IgE epitopes in ovomucoid, Biochem. Biophys. Res. Commun., 2002, 292, 1070–1074 CrossRef CAS PubMed.
- S. K. Cooke and H. A. Sampson, Allergenic properties of ovomucoid in man, J. Immunol., 1997, 159, 2026–2032 CrossRef CAS.
- K. M. Järvinen, K. Beyer, L. Vila, L. Bardina, M. Mishoe and H. A. Sampson, Specificity of IgE antibodies to sequential epitopes of hen's egg ovomucoid as a marker for persistence of egg allergy, Allergy, 2007, 62, 758–765 CrossRef.
- E. Holen, B. Bolann and S. Elsayed, Novel B and T cell epitopes of chicken ovomucoid (Gal d 1) induce T cell secretion of IL-6, IL-13, and IFN-gamma, Clin. Exp. Allergy, 2001, 31, 952–964 CrossRef CAS PubMed.
- J. Martínez-Botas, I. Cerecedo, J. Zamora, C. Vlaicu, M. C. Dieguez, D. Gómez-Coronado, V. de Dios, S. Terrados and B. de la Hoz, Mapping of the IgE and IgG4 sequential epitopes of ovomucoid with a peptide microarray immunoassay, Int. Arch. Allergy Immunol., 2013, 161, 11–20 CrossRef PubMed.
- M. Suprun, R. Getts, G. Grishina, A. Tsuang, M. Suárez-Fariñas and H. A. Sampson, Ovomucoid epitope-specific repertoire of IgE, IgG4, IgG1, IgA1, and IgD antibodies in egg-allergic children, Allergy, 2020, 75, 2633–2643 CrossRef CAS.
- M. Besler, A. Petersen, H. Steinhart and A. Paschke, Identification of IgE-binding peptides derived from chemical and enzymatic cleavage of ovomucoid (Gal d 1), Internet Symp. Food Allergens, 1999, 1, 1–12 CAS.
- S. Benedé, L. Pérez-Rodríguez and E. Molina, Caco-2 cell response induced by peptides released after digestion of heat-treated egg white proteins, Foods, 2022, 11, 3566 CrossRef PubMed.
- B. Moreira, E. Pereira, T. C. Finimundy, J. Pinela, R. C. Calhelha, M. Carocho, D. Stojković, M. Sokovic, I. C. F. R. Ferreira, C. Caleja and L. Barros, Pineapple by-products as a source of bioactive compounds with potential for industrial food application, Food Funct., 2022, 13, 9959–9972 RSC.
- Y. Zhou, X. She, Z. Chen, Y. Wei, Y. Xiao and X. Zhou, Tartary buckwheat (Fagopyrum tataricum (L.) Gaertn) protein-derived antioxidant peptides: mechanisms of action and structure-activity relationship in Caco-2 cell models, Food Sci. Hum. Wellness, 2022, 11, 1580–1590 CrossRef CAS.
- İ. Gulcin, Antioxidants and antioxidant methods: an updated overview, Arch. Toxicol., 2020, 94, 651–715 CrossRef.
- E. Bueno-Gavilá, A. Abellán, F. Girón-Rodríguez, J. M. Cayuela and L. Tejada, Bioactivity of hydrolysates obtained from chicken egg ovalbumin using artichoke (Cynara scolymus L.) proteases, Foods, 2021, 10, 246 CrossRef PubMed.
- Y. Huang, S. Chen, Y. Yao, N. Wu, M. Xu, H. Du, Y. Zhao and Y. Tu, Ovotransferrin alleviated acute gastric mucosal injury in BALB/c mice caused by ethanol, Food Funct., 2023, 14, 305–318 RSC.
- S. Benedé and E. Molina, Chicken egg proteins and derived peptides with antioxidant properties, Foods, 2020, 9, 735 CrossRef PubMed.
|
This journal is © The Royal Society of Chemistry 2024 |
Click here to see how this site uses Cookies. View our privacy policy here.