DOI:
10.1039/D3FO04761J
(Review Article)
Food Funct., 2024,
15, 2381-2405
Hypoglycemic effects and associated mechanisms of resveratrol and related stilbenes in diet†
Received
31st October 2023
, Accepted 19th January 2024
First published on 20th February 2024
Abstract
Hyperglycemia has become a global health problem due to changes in diet and lifestyle. Most importantly, persistent hyperglycemia can eventually develop into type II diabetes. While the usage of current drugs is limited by their side effects, stilbenes derived from fruits and herbal/dietary plants are considered as important phytochemicals with potential hypoglycemic properties. Herein, the most common stilbenoids in consumed foods, i.e. resveratrol, pterostilbene, piceatannol, oxyresveratrol, and 2,3,5,4′-tetrahydroxystilbene-2-O-β-glucopyranoside (THSG), are reviewed in this paper. These stilbenes are found to regulate glucose homeostasis via (a) modulation of feeding behaviour and nutrition absorption; (b) restoration of insulin signalling by enhancing insulin production/insulin sensitivity; (c) improvement of gut permeability, gut microbial profile and resulting metabolomes; and (d) amelioration of circadian rhythm disruption. In this review, we have summarized the underlying mechanisms for the hypoglycemic effects of the five most common dietary stilbenoids listed above, providing a comprehensive framework for future study and applications.
1. Introduction
Hyperglycemia, elevated blood sugar level especially during fasting status, is an essential risk factor for various diseases including diabetes mellitus (DM), metabolic syndrome, coronary heart disease and even some cancers.1,2 Depending on the abnormality, hyperglycemia can be divided into two categories: impaired fasting glucose (prediabetes) which ranges from 100 to 125 mg dL−1 and type II diabetes which exceeds 125 mg dL−1.3 According to the CDC National Diabetes Statistic Report 2020, 34.5% of all US adults had prediabetes based on their fasting glucose or hemoglobin A1C level. Moreover, once hyperglycemia persists and develops into type II diabetes mellitus (T2DM), there is no cure and patients have to live with it life-long. Hyperglycemia often occurs when the insulin-responding organs and tissues, mainly the liver, muscles, and adipose tissues, become insulin-resistant or when the body is under various stresses either from physical or mental health issues. In the case of T2DM, insulin resistance (IR) featuring an impaired biological response of organs and tissues to insulin commonly occurs, and the stress in producing excess insulin to cover the inefficiency caused by IR would compromise the body's original function and ultimately lead to a reduction in insulin level.4 In the past few decades, efforts have been made to reveal the root causes of such symptoms and the involvement of, among others, unhealthy diet or overload nutrition-induced obesity and physical inactivity was widely recognized.5 These risk factors can initiate and accelerate the progression of various pathological issues, such as IR, loss of function and apoptosis of pancreatic β-cells, and alterations in the gut environment and gut microbiota, and ultimately impact hyperglycemia and related disease. Aiming to reduce hyperglycemia, particularly in patients with T2DM, different targets are interfered with by several types of drug in current therapy: (1) promotion of insulin secretion by insulin secretagogues such as sulphonylureas, meglitinides, dipeptidylpeptidase 4 inhibitors, glucagon-like peptide 1 (GLP-1) agonists etc.; (2) reduction of hepatic glucose output, which can be achieved by metformin without affecting the level of insulin; (3) improvement of insulin sensitivity, for which thiazolidinediones and related drugs serve as peroxisome proliferator-activated receptor-γ (PPAR-γ) agonists; and (4) suppression of glucose uptake in the intestine by α-glucosidase inhibitors; and a decrease in the reabsorption of glucose in the kidneys and the excretion of extra glucose through the urine by sodium-glucose cotransporter 2 (SGLT-2) inhibitors.6,7 However, although these therapeutics can help ameliorate certain symptoms of diabetes, their significant side effects, such as weight gain, heart failure and potential intolerance, remain unexplored.7 Most importantly, it has been suggested that the majority of cases in T2DM are preventable in the stage of solely hyperglycemia, which is also closely related to other metabolic diseases. Based on the above considerations, new therapy and treatment with fewer side effects and with an emphasis on the prevention of persistent hyperglycemia are needed.
The intake of fruits, nuts and their products has been associated with some health benefits and is considered as part of a healthy eating diet. Grapes and related products are shown to promote health status. For instance, the consumption of grape powder, grape skin extract and grape seed oil successfully prevented increased fasting blood glucose and dyslipidemia in mice, and red grape pomace extract, which comes from winemaking by-products, was able to suppress postprandial hyperglycemia (PPHG).8 These grape products are also capable of decreasing oxidative stress and inflammation and promoting cardiac health.9 Blueberry, a “superfruit” that is commonly consumed in Western countries and has gained increasing popularity in Asia in recent years, is well-linked to several health-promoting effects. Dietary supplementation of whole blueberry, blueberry juice, and blueberry leaf extract has been proved to improve IR as well as postprandial glucose management.10 Mulberry (Morus alba L.), another edible berry mainly consumed in Asia, is also known for its health-promoting functions. Research on dietary supplementation with mulberry fruit extracts has shown their protective effects against hyperglycemia, IR, neurotoxins, atherosclerosis and tumors via antioxidant and immunomodulatory properties.11 Moreover, consistent with the fact that mulberry branches are used as a traditional medicine for DM patients in Asia, the bark powder12 was proved to significantly improve hyperglycemia and regulate insulin secretion. The functional properties of these fruits have been attributed to abundant phenolic compounds within them, i.e., anthocyanins, phenolic acids and flavonoids. Nevertheless, the antioxidant capacity of many products depends not only on the total amount of phenolic compounds but also on the exact polyphenol profile. Therefore, it is also important for us to study other polyphenols such as stilbene compounds.
Stilbenes, commonly secreted secondary metabolites as protective agents against stress, such as environmental threats, occur naturally in plants. They are a group of phenolic compounds known for diverse biological activities, including antioxidant, anti-inflammatory, anti-obesity, anti-diabetic and other properties.13 Recent studies have revealed the hypoglycemic and anti-diabetic effects of several stilbene compounds commonly found in grapes, berries and other foods, these effects being attributed to their influence on food intake and nutrient absorption, glucose homeostasis, apoptosis and function of pancreatic β-cells, gut permeability, composition of gut microbiota as well as circadian rhythm. Since most abovementioned mechanisms are similar to those of currently used medicines, these stilbenes are considered as potential agents to control hyperglycemia, or as preventive dietary supplements with less safety concern.
In this review, common dietary stilbenoids, that is, resveratrol, pterostilbene, 2,3,5,4′-tetrahydroxystilbene-2-O-β-glucopyranoside (THSG), piceatannol, and oxyresveratrol, are discussed in-depth in terms of structure, dietary source and pharmacokinetics. Recent study results regarding their preventive and therapeutic effects on hyperglycemia and diabetes are summarized, and the potential mechanisms of their actions are discussed. Although compared with resveratrol and pterostilbene, there is significantly less research concerning THSG, piceatannol, and oxyresveratrol, they are included here due to their structural similarity to resveratrol, better bioavailability and excellent activities in preventing other diseases.
2. Overview of resveratrol, pterostilbene, THSG, piceatannol and oxyresveratrol
Structures of stilbenes can be characterized by C6–C2–C6, a basic 14-carbon skeleton of 1,2-diphenylethylene, in other words, two aromatic rings linked by an ethylene moiety. Owing to various modifications such as hydroxylation, glycosylation, methylation and polymerization that take place at different positions of the basic stilbene structure, many stilbenes have been identified.13 The chemical structures of selected stilbenoids are shown in ESI Fig. S1.†
Resveratrol (trans-3,5,4′-trihydroxystilbene), one of the most extensively studied monomeric stilbene compounds in the past few decades, is present in a variety of plants such as berries and grapes and reaches the highest concentration in the seeds of Paeonia suffruticosa.14 According to current reports, resveratrol possesses quite a few biological functions, ranging from antioxidant, anti-cancer, and anti-aging to cardioprotective and neuroprotective effects.15 Through studying the analogues of resveratrol, it was found that structural differences, particularly the number and position of hydroxyl groups on the ring, were closely related to its free-radical scavenging capacity, which was believed to play an essential role in its overall bioactivities.16
Pterostilbene (trans-3,5-dimethoxy-4′-hydroxystilbene), a natural dimethylated analog of resveratrol predominately found in blueberries, exhibits similar or better biological functions.17,18 The replacement of the two hydroxyl groups on the A-benzene ring of resveratrol with two methoxy groups is believed to increase lipophilicity and reduce overall reactions with phase II metabolic enzymes like glucuronidase in the human body.19,20 Therefore, in comparison with resveratrol, pterostilbene may have a higher membrane permeability and stronger metabolic stability, thus achieving a better bioavailability.17,19,21 Compared with resveratrol, pterostilbene was also proved to exert additional protective effects against aging, demonstrated by the improvement of cognitive behavioural deficits, memory loss, and dopamine release.22
THSG is a stilbene glycoside and one of the major bioactive phytochemicals found in the medicinal plant named Polygonum multiflorum (PM), which is popularly grown and consumed in Southeast Asia since it is traditionally known for an anti-aging effect and the ability to darken hair. Different from resveratrol, THSG possesses an extra hydroxyl group at the 2-position connected with a 2-O-glycoside. Once the sugar moiety is dissociated in vivo, an additional hydroxyl group will present at the ortho-position, giving it a much higher antioxidant activity and stronger protective effect against oxidative stress induced by reactive oxygen species (ROS).23
Piceatannol (trans-3,5,3′,4′-tetrahydroxystilbene) is a hydroxylated metabolite of resveratrol and it can be naturally found in white tea, passion fruit, peanuts, berries, and the skin of grapes. Although piceatannol was at first less noticed due to its relatively low content in wine, it was later found that a considerable amount of piceatannol was present in the seeds of passion fruit (Passiflora edulis) with an amount of 4.8 mg g−1 freeze-dried seed.24 Meanwhile, piceatannol exhibits similar or even better biological properties compared with resveratrol. For instance, piceatannol is more metabolically stable and possesses a better antioxidant capacity due to the additional hydroxyl group at the 3′-position which facilitates the formation of a semiquinone structure.25 As a result, piceatannol has gradually drawn the attention of scientists in the last decade.
Oxyresveratrol (trans-2,4,3′,5′-tetrahydroxystilbene), another hydroxylated derivative of resveratrol, is a natural stilbenoid that has similar biological activities to resveratrol.26 However, due to an extra hydroxyl group at the 2-position, oxyresveratrol exhibited a stronger antioxidant and anticancer effect than resveratrol.27 The distribution of oxyresveratrol occurs in certain plants sporadically and concentrates in two genera from the Moraceae (or mulberry family), namely Artocarpus (jackfruit) and Moru (mulberry). Commonly, oxyresveratrol is extracted from the bark and root of Morus plants, i.e. Morus alba.26
The contents of these five stilbenes reported in common foods and edible plants are listed in Table 1. Of note, these compounds are not only present in the fruit of the plant but also in other parts which are commonly treated as waste, including the stems and leaves. Therefore, the extraction of stilbenes from the waste after fruit-picking or even juicing allows a more complete utilization of the entire plant. Methods such as UV-C radiation and cotreatment with other compounds can be used to stimulate the accumulation of stilbenes and increase their levels in the fruits and stems. For instance, in common grapes, the resveratrol content increased from 0.78 μg g−1 to 1.63 μg g−1 after UV-C radiation28,29 while in peanut callus cotreated with chitin, the resveratrol content can rise to 10.43 μg g−1.30 Detailed information of other experiments is summarized in Table 1.
Table 1 Content of stilbenes found in common food and edible plant
Food |
Scientific name |
Stilbene compounds |
Content in different parts of plant |
Ref. |
Common grapes |
Vitis vinifera
|
Resveratrol |
Fruit: 36.6 μg g−1 |
14
|
Resveratrol |
Fruit: 3.18 μg g−1 before UV-C radiation |
28
|
8.13 μg g−1 after UV-C radiation |
Stem: 50 μg g−1 |
Resveratrol |
Pinot noir cane after storage: up to around 2800 μg g−1 dried weight |
174
|
Piceatannol |
Fruit: 0.78 μg g−1 before UV-C radiation |
28
|
1.63 μg g−1 after UV-C radiation |
Stem: 5 μg g−1 |
Piceatannol |
Pinot noir cane after storage: up to around 800 μg g−1 dried weight |
174
|
Pterostilbene |
Fresh weight: 0.2–4.7 μg g−1 |
29
|
Skin of grapes after UV radiation: 0.8–20 μg g−1 fresh weight |
Red wine |
|
Resveratrol |
0.27 mg per 100 ml |
175
|
Piceatannol |
0.58 mg per 100 ml |
Phenol-explorer |
Mulberry |
Morus sp. |
Resveratrol |
Fruit: 7.95 μg g−1 |
14
|
Resveratrol |
3.3 μg g−1 |
176
|
Piceatannol |
Fruit: 2–10.19 μg g−1 |
177
|
Oxyresveratrol |
Bark to stem: 150–680 μg g−1 |
178
|
Oxyresveratrol |
Fruit: up to 29.5 μg g−1 |
176
|
Fruit marc: up to 37.3 μg g−1 |
Leave: up to 179.9 μg g−1 depending on cultivar |
Peanut |
Arachis hypogaea
|
Resveratrol |
0.4 μg g−1 |
175
|
Resveratrol |
Dry-blanched peanut skin: 3.6 μg g−1 |
179
|
Resveratrol |
Peanut callus after UV-C radiation: up to 11.97 μg g−1 |
180
|
Resveratrol |
Peanut callus cotreated with chitin: 10.43 μg g−1 |
30
|
Piceatannol |
Peanut callus after UV-C radiation: up to 5.31 μg g−1 |
180
|
Piceatannol |
Peanut callus cotreated with chitin: 2.55 μg g−1 |
30
|
Pterostilbene |
N/A |
181
|
Blueberries |
Vaccinium sect. Cyanococcus |
Resveratrol |
Fruit: 6.7 μg g−1 |
175
|
Pterostilbene |
Dried fruit: up to 0.151 μg g−1 |
182
|
Piceatannol |
Dried fruit: up to 0.422 μg g−1 |
182
|
Deerberry |
Vaccinium stamineum L. |
Pterostilbene |
Dried fruit: 0.52 μg g−1 |
182
|
Piceatannol |
Dried fruit: up to 0.195 μg g−1 |
182
|
Lingonberries |
|
Resveratrol |
30 μg g−1 |
175
|
Heshouwu |
Polygonum multiflorum thunb.
|
THSG |
Root tuber (edible part): 5% |
183
|
Sugarcane |
Saccharum spp. |
Resveratrol |
Billet stalks after 7 days incubation: 73 μg g−1; juice: 1.2 mg L−1 |
184
|
Piceatannol |
Billet stalks after 7 days incubation: 1659 μg g−1; juice: 8.5 mg L−1 |
184
|
Jackfruit |
Artocarpus heterophyllus Lam. |
Oxyresveratrol |
N/A |
185
|
Passion fruit |
Passiflora edulis
|
Piceatannol |
Raw passion fruit seed: 2.2 mg g−1 |
24
|
Resveratrol |
Raw passion fruit seed: 0.1 mg g−1 |
24
|
3. Hypoglycemic effect of resveratrol and related stilbenes and potential mechanisms
Several studies have demonstrated the efficacy of these five stilbenes, i.e. resveratrol, pterostilbene, oxyresveratrol, piceatannol, and THSG, to relieve hyperglycemia and improve insulin sensitivity in rodent models of IR and T2DM. Animal studies that used resveratrol, pterostilbene, THSG, piceatannol, and oxyresveratrol as dietary intervention managed to improve some symptoms including lowered fasting blood glucose level, improvement in glucose tolerance and insulin sensitivity, alleviation of loss in β-cell functions, reduced weight gain, improved lipid profile and others. They will be discussed in the following sections. Commonly used dosages, durations, and outcomes for related research are listed in Tables 2 and 3. For a better comparison of dosages between animal studies and clinical trials, the conversion of human dosages to animal equivalent doses can be calculated using the FDA drafted guideline, in which the dosages for mouse and rat are approximately calculated as multiplying the human dosage (mg kg−1) by 12.3 and 6.2 respectively.31 Commonly, body weight is assumed as 60 kg per person. Therefore, since resveratrol treatment in clinical trials (discussed in section 4) ranged from 100–1000 mg day−1 (1.67–16.7 mg kg−1), its corresponding dosages for mice and rat are 20.5–205.4 mg kg−1 and 10.3–103.5 mg kg−1 respectively. The dosage used in most of the studies fell into this range. For studies applying stilbenes in water (0.005% to 0.02%) and in feed (2 or 4 g kg−1), the corresponding dosage is calculated as 12 to 48 mg kg−1 and 480 to 960 mg kg−1 (water and food intake for mice assumed as 6 mL and 6 g respectively).32 Since mice sometimes tend to gnaw the food without eating it, actual food intake could be much less than the 6 g that was recorded, and thus 2 g kg−1 might still be reasonable. However, the dosages used in some experiments were extremely high, reaching 400 mg kg−1 or even 800 mg kg−1via oral gavage for several months, and hence these experiments were marked specifically with symbols (***) in Tables 2 and 3. The regulatory effect of these stilbenes on hyperglycemia may be ascribed to the aspects listed below and they are also briefly depicted in Fig. 1.
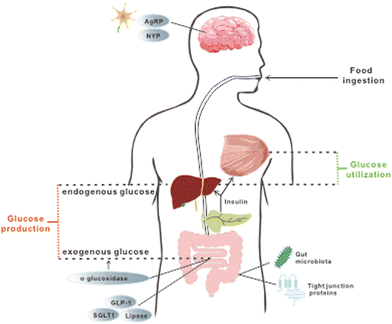 |
| Fig. 1 Organs, tissues and downstream targets that are involved in the maintenance of glucose homeostasis during treatment with selected stilbenoids. The stilbenes are found to exert regulatory effects on glucose homeostasis via a few aspects, including (a) management of exogenous glucose intake by modulating feeding behavior and nutrition absorption (targets are marked in grey circles); (b) improvement of glucose utilization and endogenous glucose production through restoration of insulin production and further signalling (details are elaborated in Fig. 2); and (c) attenuation of systemic oxidative stress and inflammation via enhancing gut permeability and positively altering gut microbial profile and related metabolomes. | |
Table 2 Effect of resveratrol on glycemic control in different diabetic animal models
Animal models |
Dose/dosing method/period |
Hypoglycemic and anti-diabetic function |
Involvment of mechanisms |
Ref. |
High fat diet-induced obese wild type C57BL/6J mice with insulin resistance and GLP 1r−/− mice |
60 mg kg−1 day−1; mixed in diet; 5 weeks |
Glucose tolerance↑ |
Increasing GLP-1 level and promoting its further action |
35
|
Insulin level↑ |
High fat diet-fed C57BL/6J mice with insulin resistance |
30 mg kg−1; oral gavage; one time |
Postprandial blood glucose after sucrose-loading or starch-loading↓ |
Inhibition of α-glucosidase |
37
|
High fat diet-induced obese Wistar rats |
20 mg kg−1; dissolved in saline; daily basis; 8 weeks |
Plasma triglycerides↓ |
Inhibition of pancreatic lipase |
39
|
Fecal triglycerides level↑ |
Insulin sensitivity↑ |
HOMA-IR↓ |
Blood glucose↓ |
High fat diet-fed C57BL6J mice with insulin-resistance |
100 mg kg−1; daily basis; intragastric administration; 6 weeks |
Blood glucose and serum insulin level↓ |
Modulation of lncRNAs in the insulin signalling pathway (G6PC, FOXO1 and suppressor of cytokine signalling 3 (SOCS3)↓; phosphorylation of Akt and FOXO1↑) |
51
|
The quantitative insulin sensitivity check index (QUIACKI)↑ |
High fat diet-fed C57BL/6J mice with insulin-resistance |
100 mg kg−1; daily basis; intragastric administration; 6 weeks |
Significantly enhanced PI3K/Akt insulin signaling pathway |
Mmu-miR-363-3p expression↑ |
52
|
High fat diet-fed C57BL/6J mice with insulin-resistance |
100 mg kg−1; oral administration; 8 weeks |
QUIACKI↑; insulin sensitivity↑ |
Expression of AMPK kinase: ataxia-telangiectasia mutated (ATM) ↑ |
54
|
Blood glucose and serum insulin level↓ |
Phosphorylation of AMPK↑ |
Lipid accumulation↓ |
COXIV↑ |
Insulin-resistant KKAγmice |
2 or 4 g kg−1 diet; 12 weeks |
Blood glucose and serum insulin level↓ |
Upregulation of Sirt1 protein in liver and muscle and consequent AMPK activation |
49
|
Insulin and glucose tolerance↑ |
Methylglyoxal-induced insulin-resistant Balb/C mice |
10 mg kg−1; daily basis; oral gavage; 12 weeks |
Serum glucose level↓ |
Phosphorylation of Nrf2 in pancreas and Tyr-phosphorylated IRS-1 level in liver↑ |
50
|
Significantly lower ITT |
Insulin level↓ |
C57BL/KsJ-db/db mice |
0.005%, 0.02%; diet; 6 weeks |
Hepatic glycogen and blood glucose↓ |
Activation of AMPK and downstream targets |
99
|
Serum free fatty acid and triglycerides↓ |
Hepatic gluconeogentic enzymatic activity↓ |
Plasma insulin level↑ |
Upregulation of GLUT4 protein and protection of pancreas |
Monosodium glutamate-induced T2DM CD1 mice |
30 mg kg−1; dissolved in water; 60 days |
Plasma insulin, glucose and triglyceride level↓ |
Slc2a4 mRNA (GLUT 4 encoding gene), GLUT4 protein and nuclear Sirt1 protein expression↑; Slc2a2 mRNA (GLUT 2 encoding gene) and GLUT2 protein expression level in liver↓ |
105
|
Pck1 mRNA expression level↓ |
Sprague-Dawley rats with chronic intermittent hypoxia induced-insulin resistance |
3, 30, 60 mg kg−1; daily basis; oral gavage; 28 days |
Improved IR; HOMA-IR↓ |
Insulin receptor and GLUT2 expression in liver↓ |
104
|
Phosphorylation of PI3K and AKT↑ |
Activation of Sirt1 |
STZ-induced diabetic rats supplemented with insulin |
10 mg kg−1; daily basis; intraperitoneal injection; 30 days |
Improved glycemic control |
Slc2a2 mRNA and GLUT2 protein expression level in liver↓; Pck1and G6pc mRNA expression↓ |
103
|
Goto-Kakizaki rats |
20 mg kg−1; daily basis; oral gavage; 10 weeks |
Improved glucose tolerance, blood glucose↓, insulin level↑; better preservation of islets |
Protein expression level of Akt and its phosphorylation↓ |
61
|
ACC level and its phosphorylation in muscle↓ |
Male C57BL/6J mice |
400 mg kg−1; daily basis; oral administration (dissolved in saline); 16 weeks*** |
Function of insulin secretion both in vitro and in vivo↑ |
Elevating Sirt1 expression and inhibiting UCP2 expression in islets; pancreatic cell apoptosis and oxidative stress↓ |
65
|
Improved insulin resistance |
Blood glucose and FFA↓ |
Improved islet morphology including less islet hypertrophy |
STZ-nicotinamide-induced Wistar rats |
5 mg per kg body weight; daily basis; oral administration; 30 days |
Blood glucose↓ |
Antioxidative function↑ |
74
|
Glycosylated hemoglobin↓ |
Oxidative stress↓ (elevating level of SOD, GPx, GST, GSH, vitamin C and E) |
Plasma insulin↑ |
Level of inflammatory cytokines↓ |
Protection of pancreatic β-cells |
STZ-induced diabetic Wistar rats |
1, 5, 10 mg per kg body weight; daily basis; oral treatment; 30 days |
Fasting blood glucose↓ |
RAGE expression↓ |
77
|
Total antioxidant capacity↑ |
Antioxidant content (in liver)↑ |
Total oxidant status↓ |
|
STZ-induced diabetic Wistar rats |
1, 5, 10 mg per kg body weight; daily basis; oral treatment; 30 days |
Fasting blood glucose↓ |
RAGE expression↓ |
76
|
Serum insulin level↑ |
Antioxidant content (in kidney)↑ |
Total antioxidant capacity↑ |
|
C57BL/6 with CML-induced pancreatic dysfunction |
10 mg kg−1; intraperitoneal injection; 12 weeks |
Improved OGTT; blood glucose↓ |
PDX-1, PPARγ, Nrf2 and GCL (rate-limiting enzyme in GSH production) expression↑ |
78
|
Insulin sensitivity↑ |
C/EBPβ expression in pancreas↓ |
Insulin level↑ |
GSH level↑ |
Inhibited pancreatic damage and elevated numbers of islets |
Phosphorylation of Akt and Nrf2 in muscle and liver↑ |
STZ-nicotinamide-induced rats |
10 mg kg−1; oral aqueous suspension; 30 days |
Improved insulin tolerance |
Normalizing the mRNA and protein expression level of Nrf2, Keap1 and other related genes |
75
|
Proinflammatory cytokines level in kidney↓ |
Serum level of AGE↓ |
Level of antioxidant in kidney↑ |
|
STZ-induced T1DM mice |
50 mg kg−1; daily basis; intraperitoneal injection; 12 days |
Fasting blood glucose level↓ |
Inhibited expression of CXCL16/NF-κB |
84
|
Serum insulin level↑ |
Normalized CD45 and CXCL16 (T cell regulators) expression in islets |
Food intake↓ |
Inhibited macrophage infiltration |
Serum level of GSH↑ |
Level of MDA↓ |
High fat diet-fed C57BL/6J mice with insulin-resistance |
200 mg kg−1 or 400 mg kg−1; daily basis; oral gavage; 18 weeks*** |
Blood glucose↓ |
F4/80 (murine macrophage marker)↓ |
90
|
HOMA-IR↓; insulin sensitivity↑ |
Serum cytokine↓ |
|
Reduced macrophage infiltration and inflammation via CCR2 (regulates monocyte mobilization) modulation in adipose tissue |
High fat diet-induced obese C57/BL6J mice |
4 g kg−1 diet; 16 weeks |
Improved insulin and glucose tolerance |
Downregulation of MAPK pathway |
86
|
Fasting blood glucose↓ |
Expression of TLR2 and TLR4↓ |
HFD-induced inflammation in skeletal muscle↓ |
Enhanced percentage of T regulatory cells |
Switched from M1 to M2 macrophage |
High fat diet-induced insulin resistant C57BL/6J mice |
100 mg kg−1; daily basis; oral gavage; 12 weeks |
Ameliorate insulin resistance, dyslipidaemia, hyperglycemia, inflammation and endotoxemia |
Expression of tight junction proteins↑ |
125
|
Modifying the composition of intestinal microbiome |
Table 3 Effect of pterostilbene, piceatannol and oxyresveratrol on glycemic control in diabetic animal models
Animal models |
Dose/dosing method/period |
Anti-diabetic function |
Involvement of mechanisms |
Ref. |
Pterostilbene |
Fructose-induced diabetic Sprague-Dawley rats |
20, 40 mg kg−1; daily basis |
Fasting blood glucose↓ |
Reduction of hepatic oxidative stress via increasing level of hepatic SOD and GSH while decreasing TBARS (lipid peroxidation marker) |
57
|
Oral gavage; 8 weeks |
Improved OGTT |
Fasting serum insulin level↓ |
HOMA-IR↓ |
Insulin sensitivity index↑ |
Glycated haemoglobin↓ |
Mice with AGE-induced insulin resistance |
200 mg kg−1 pterostilbene added in high-AGE diet |
Fasting blood glucose↓ |
AGE accumulation in liver and serum↓ |
58
|
Lower AGE accumulation |
Improved hepatic insulin signaling |
STZ-induced diabetic Swiss Albino mice |
5, 10 mg kg−1; intraperitoneal injection; 5 weeks |
Improved blood glucose level |
Nrf2 and its downstream targets expression in pancreas↑ |
62
|
Insulin level↑ |
Restoring glycogen level in liver |
Preserve a better structure for insulin-positive cells |
Enzymatic activity of hexokinase, G6PD↑ |
Enzymatic activity of G6P and F1, 6BP↓ |
STZ-induced diabetic Sprague-Dawley rats |
20, 40, 80 mg kg−1; daily basis; oral gavage; 8 weeks |
Improved fasting blood glucose and glucose tolerance |
Inflammatory cytokines such as TNF-α and IL-6↓ |
64
|
Increased insulin level and improved insulin resistance |
Reducing oxidative stress by upregulating SOD level and decreasing MDA level |
Improved histopathology of pancreas |
Protein expression level of PI3K, p-Akt and GLUT4, IRS-1 and PPAR-γ↑ |
Obesogenic diet-fed Wistar rats |
15, 30 mg kg−1; mixed with diet; 6 weeks |
Basal glucose and insulin↓ |
Enzymatic activity of GK↑ |
106
|
HOMA-IR↓ |
Phosphorylated Akt/total Akt ratio↑; expression of GLUT4 in muscle↑ |
Improved glucose tolerance |
STZ-induced diabetic Swiss Albino mice |
5, 10 mg kg−1; intraperitoneal injection; 5 weeks |
Fasting blood glucose↓ |
Serum pro-inflammatory cytokines↓ |
63
|
Insulin level↑ |
Activating Nrf2 and its downstream genes |
Pancreatic islet apoptosis↓ |
iNOS expression, nitrate and nitrite level in pancreas↓ |
Improved pancreatic islet structure |
Piceatannol |
HFD-induced obese mice |
0.1% or 0.25% piceatannol in feed; 18 weeks |
Blood glucose↓ |
Modulation of gut microbiota |
186
|
Reduced lipid accumulation and adipocyte size via increasing pAMPK and decreasing PPARy expression |
High fat diet-induced diabetic C57BL/6J mice |
3, 10, 30 mg kg−1; oral gavage; daily basis; 5 weeks |
Fasting blood glucose↓ |
NA |
187
|
T2DM db/db mice |
50 mg kg−1 in suspension; intubated; 3 weeks |
Fasting blood glucose↓ |
NA |
110
|
Improved glucose tolerance |
High fat diet-fed diabetic C57BL/6J mice |
10 mg kg−1; daily basis; oral gavage; 4 weeks |
Improved glucose control (OGTT test) |
Hepatic gene expression of insulin receptor, AMPK, Sirt1, Sirt3, Sirt6, PGC1-α and FOXO1↑ |
111
|
TNF-α↓ |
Oxyresveratrol |
STZ-induced diabetic ICR mice |
600 mg kg−1; once daily; oral administration; 22 days*** |
Fasting blood glucose↓ |
GLUT2 transcription↑ |
188
|
Glycogen level↑ |
High-fat diet-induced |
800 mg kg−1; once daily; oral gavage; 14 weeks*** |
Pancreatic insulin↓ |
Insulin secretion↑ |
67
|
C57BLKS/J db/db mice |
Plasma insulin↑ |
Pancreatic ROS level↓ |
Improved pancreatic inflammation |
High-fat diet-induced |
0.25% and 0.5% |
Fasting blood glucose↓ |
Expression of glucose-6-phosphatase and GLUT4 ↑ |
112
|
C57bl/6 diabetic mice |
Plasma insulin↑ |
Expression of IRS1, AMPKα2, PGC-1α, Sirt1↑ |
3.1 Influence on food intake and nutrition absorptions
Long-term exposure to elevated levels of free fatty acid (FFA) and glucose has been considered as the cause of IR, which is an important biomarker in patients with persistent hyperglycemia prior to the onset of T2DM.5 Consequently, controlling elevated levels of both plasma FFA and glucose can prevent or alleviate hyperglycemia. Since both substances are used as energy fuels in the body, this could be achieved by promoting energy expenditure and reducing energy intake. Regulation of feeding behavior and satiety by neuropeptides and hormones, as well as modulation of nutrient absorption in the gut, are effective ways to alter this energy balance and they are reported to be part of the mechanisms through which resveratrol and pterostilbene exert their hypoglycemic functions.
The regulatory effect of resveratrol on food consumption has been examined. Neuropeptide Y (NPY) and agouti-related peptide (AgRP) were postulated to exert powerful stimulation on feeding behaviour. However, in mice injected with resveratrol, a decline in accumulative food intake over 48 h was noticed and resveratrol was shown to downregulate both NPY and AgRP33 in neuron cells. In line with this study, another research study found that intervention with resveratrol caused a concomitant decrease in energy intake and an increase in metabolic rate, leading to a reduced body mass in non-human primates.34 Nevertheless, future works focusing on the detailed changes of biomarkers of satiety are necessary to investigate the effect of resveratrol on feeding behaviours in mice with hyperglycemia. In addition, GLP-1, an incretin hormone mainly produced by the intestinal endocrine cells, seems to be involved in the regulatory effect of resveratrol. It can reduce food intake by delaying the process of emptying the stomach, reducing bowel movement and glycogen secretion. Upregulation of GLP-1 in mice with hyperglycemia was reported after supplementation with resveratrol for 8 weeks.35,36 Together, these results indicate that resveratrol is effective in modulating food consumption.
Once the food is ingested, the next step to reduce energy intake is to modulate nutrition absorption in vivo, which can be accomplished by regulating enzymatic activity and nutrient transporters. α-Glucosidase, a membrane-bound enzyme in the GI tract, is responsible for the hydrolysis of polysaccharides to glucose and other monosaccharides. When a large amount of glucose generated from the hydrolysis is taken up by the gut and enters the circulation, PPHG occurs. Therefore, suppression of α-glucosidase can alleviate PPHG and subsequently lessen the stress for insulin production, thus protecting the normal functions of the pancreas. In vitro experiments have illustrated the ability of resveratrol to significantly repress the enzyme activity of both yeast and mammalian α-glucosidase.37,38 Further in vivo research in obese mice demonstrated that the administration of resveratrol prior to sucrose or starch loading can indeed lower postprandial blood glucose.37 Besides managing PPHG, another key point is to reduce the elevated level of FFA, of which dysregulation can ultimately contribute to IR and further persistent hyperglycemia. The inhibitory effect on pancreatic lipase, an enzyme that cleaves triglycerides to fatty acids, along with the drastically higher level of triglycerides in faeces, was observed after resveratrol treatment, implying delayed and reduced FA absorption by the intestinal enterocytes.38,39 Besides, reduced intestinal glucose uptake from isolated porcine jejunum and ileum was reported in vitro after pre-incubation with resveratrol, and the inhibition of SGLT-1, a transporter that is predominately in charge of dietary glucose uptake in the small intestine, was postulated as the underlying mechanism.40–42 However, further in vivo experiments are needed to establish such a direct relationship. These studies illustrate the ability of resveratrol to interfere with digestion and nutrient absorption in general, thus ameliorating hyperglycemia.
Similar to resveratrol, oxyresveratrol exhibits some of the functions mentioned above, such as inhibition of α-glucosidase. However, a recent study stated that its inhibitory effect was greatly compromised when applied to membrane-bound α-glucosidase in differentiated Caco-2 cells.43 Piceatannol, on the other hand, presented convincing information on its ability to attenuate PPHG in vivo.37 As for pterostilbene and THSG, data are rather limited yet pterostilbene has been shown to be effective in inhibiting α-glucosidase with relatively limited efficacy when compared with resveratrol.37
3.2 Regulation of glucose homeostasis
The maintenance of glucose homeostasis plays a crucial role in the prevention and treatment of hyperglycemia. In healthy individuals, different levels of insulin are released into the circulation to maintain glucose homeostasis under different situations. In post-absorptive states, a low level of insulin is released in pulses to ensure the necessary protein synthesis and maintain a normal level of hepatic glucose production. However, in the postprandial period, the insulin level is rapidly upregulated in response to glucose absorption in the gut, which will subsequently enhance glucose uptake in tissues and organs and reduce hepatic glucose output by inhibiting gluconeogenesis and glycogenolysis.44,45 By contrast, in patients with hyperglycemia, disruption of glucose homeostasis occurs because of the abnormality in insulin function or secretion. Hyperinsulinemia would commonly be observed in the first stage as it is proposed as a compensatory mechanism for progressing IR, in which responses to insulin in the targeted tissues and organs are significantly compromised.5 On the other hand, decreased levels of insulin may also occur when pancreatic β-cells, which are responsible for insulin production, are exhausted under excessive stress. Both situations not only alter normal hepatic enzymatic activities which in turn lead to excessive gluconeogenesis and glycogenolysis during the fasting state but also impair glucose transportation and uptake by the target tissues, producing insufficient clearance of blood glucose after meals.46 Therefore, the improvement not only in IR but also in insulin secretion via protecting pancreatic structure and function, along with the subsequent enhancement in glucose deposition to the responding tissues and organs and in the recovery of related hepatic enzymatic activities, might be considered as potential mechanisms in the prevention and treatment of hyperglycemia and related diseases. Resveratrol and other stilbenes mentioned above were reported to exert hypoglycemic functions through the proposed mechanisms summarized in Fig. 2.
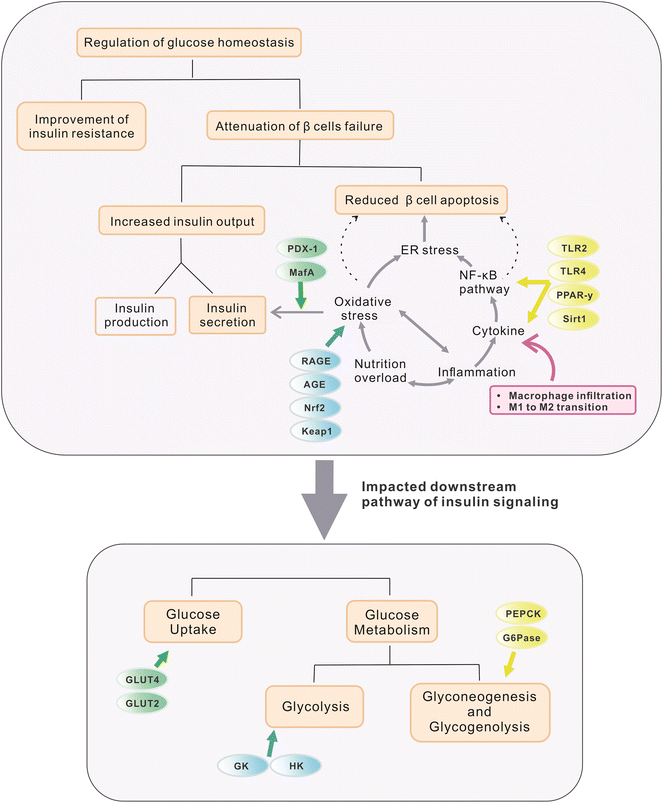 |
| Fig. 2 Proposed mechanisms and targeted genes through which selected stilbenes exert hypoglycemic and anti-diabetic functions. The light orange rectangles mark major aspects affected in glucose deposition and metabolism. The gray circle illustrates the main pathway involved in β cell apoptosis (mainly oxidative stress and inflammation). Ellipses in different colors represent targeted genes modulated by selected stilbenes. The pink rectangle shows the effects of stilbenes on macrophages. Regulation of glucose homeostasis can be achieved mainly through improving IR and increasing insulin output (reduce β-cell apoptosis and enhance β-cell function in insulin productivity and secretion). Meanwhile, downstream targets of insulin signalling were also upregulated by stilbenoids, thus ameliorating hyperglycemia. | |
3.2.1 Improvement of insulin resistance.
Insulin resistance, also known as impaired insulin actions, is characterized by a reduced response of the liver, muscles and other organs and tissues to insulin. Several factors are proposed as contributors in the development of IR, including direct harmful effects posed by excess lipids and glucose on the metabolism in organs and tissues, activation of the endoplasmic reticulum (ER) stress pathway and enhanced inflammatory reactions. A detailed review of the molecular and metabolic mechanisms of insulin resistance is done by Muoio and Newgard.47 In brief, the excess glucose and lipids in the liver will reduce the lipid oxidation and promote the production of lipid with a signalling property that further results in the inactivation of key insulin signalling molecules, whereas, in muscle, upregulation of fatty acid oxidation caused by excess lipids is not accompanied by the concurrent upregulation of downstream metabolic processes such as the tricarboxylic acid cycle, thus compromising insulin signalling due to the incomplete lipid metabolism in the mitochondria. Besides the changes in lipid metabolism, reduced glucose uptake and metabolism are also commonly observed, characterizing the impaired insulin actions during IR. In particular, the inhibition of insulin receptor substrate-1 (IRS-1) plays a key part in this process. IRS-1 is an important mediator that transmits the signal from the insulin receptors to intracellular pathways for glucose uptake. Under normal conditions, tyrosine phosphorylation of IRS-1 by the phosphoinositide 3-kinase (PI3K)/protein kinase B (Akt) cascade is required for insulin actions, which further influence glucose uptake through glucose transporter 4 (GLUT4) and glucose metabolism (glycogen synthase kinase 3 etc.) (see section 3.2.3. for a detailed explanation). However, the phosphorylation of IRS-1 on serine residues is involved in the negative feedback loop that terminates insulin signalling, and such phosphorylation could be induced by common agents such as free fatty acids, cytokines, and cellular stress.48 Nutrition overload and related metabolic changes are linked to the ER stress-induced activation of c-Jun-N-terminal kinase (JNK), which can phosphorylate insulin receptor substrate-1 (IRS-1) and further interfere with insulin signalling.47
The ability of resveratrol to ameliorate IR was reported in several studies. In cells and rodents with IR, overproduction of insulin was observed yet a decreased insulin level, together with an improvement in glucose tolerance, was reported after resveratrol treatment.49–51 These results indicated an effective attenuation of IR, which could be attributed to the regulation in long noncoding RNA (lncRNA)51 and micro RNA52 that potentially regulates the interaction of the encoded transcript and protein in the insulin signalling pathway, restoration of autophagic flux,53 improvement in fatty acid oxidation efficiency and activity of key enzymes in the respiratory electron transport chain: cytochrome oxidase subunit IV(COXIV),54 oxidative stress and subsequent apoptosis in insulin-responding cells and tissues and others.55,56
A similar function of pterostilbene was also reported. Recent in vitro and in vivo studies indicated that pterostilbene administration can effectively decrease the homeostasis model assessment (HOMA)-IR57 and rescue symptoms of IR via enhancing (IRS-1)/(PI3K)/Akt insulin signalling58 or reversing the activation of c-Jun N-terminal kinase (JNK) which in turn alters the IRS-1/Akt/glycogen synthase kinase-3β insulin signalling pathway.59
3.2.2 Improvement of insulin production and secretion.
Apart from the amelioration in IR, the promoting effect of resveratrol on the production and secretion of insulin is also a key part in the regulation of glucose homeostasis. Depending on the time point when the rodents were sacrificed, a decline in insulin level was also observed in diabetic animal models,60 but resveratrol was reported to elevate insulin production.61 The pancreas is one of the most important organs in maintaining a normal glucose level. There are several types of cell in the pancreatic islets such as α, β, and δ-cells, among which β-cells are responsible for the production and secretion of insulin. As mentioned above, IR associated with chronic exposure to elevated levels of FFA and glucose and a further requirement for the overproduction of insulin can ultimately result in progressive β-cell failure, which includes mainly two aspects, a decrease in β-cell mass (cell number decrease) and the impairment of β-cell function (reduced output of insulin). The main form of cell death in β-cell failure is apoptosis, induced either by cytokines, nutrient overload, or the combination of both and they may involve the activation of ER stress and increased oxidative stress.4 Therefore, protecting β-cells from excessive apoptosis and functional damage caused by free radicals like ROS and increased pro-inflammatory cytokines, should be beneficial for the prevention of hyperglycemia and related diseases.
A few studies have illustrated a positive role of selected stilbenes in the stimulation of insulin production, potentially through protecting β-cells from apoptosis and reducing pancreatic functional damage. Histologic analysis showed an improved insulin secretion (more positive staining for insulin) and better preservation of pancreatic β-cells (better morphology with less distorted β-cells) after treatment with resveratrol and pterostilbene.50,61–64 This was further confirmed by a decreased expression level of B cell leukemia/lymphoma 2 (Bcl2)/Bcl2-associated X and reduced activation of caspase 3, the main executor of apoptosis.63,65,66 As for oxyresveratrol and piceatannol, although there are no available data concerning their influence on the pancreas, they were reported to lower fasting blood glucose. Oxyresveratrol can increase plasma insulin level in vivo and stimulate insulin secretion in a pancreatic cell line.67 Study results attributed the above benefits to the anti-oxidative and anti-inflammatory properties of these stilbenes and the underlying mechanisms will be discussed in the following sections.
3.2.2.1 Improvement of oxidative stress-related pancreatic damage and insulin secretion.
Excessive oxidative stress is a common feature associated with long-term hyperglycemia. Several mechanisms have been proposed, which mainly refer to the direct promotion of ROS generation or compromised cellular antioxidant defense mechanisms.46 Regulation of cellular redox homeostasis primarily happens at the transcriptional level, and the nuclear factor erythroid 2-related factor-2 (Nrf2)/kelch-like ECH-associated protein 1 (Keap1)/antioxidant response elements (ARE) pathway is the primary mediator of this response. However, dysfunction of this pathway is commonly seen in patients with hyperglycemia and diabetes, thus weakening their ability to clear ROS and contributing to an imbalanced redox state.68 In addition, such an imbalance can deteriorate by the accumulation of advanced glycation end products (AGEs) mediated through their cell surface receptor widely expressed in the tissues, that is, RAGE. AGE-ligand interaction can lead to activation of the nicotinamide adenine dinucleotide phosphate (NADPH) oxidase system,46 which along with the mitochondria serves as the major source of ROS production in the biological system.69 Even worse, owing to the naturally low expression level of free radical-scavenging enzymes, pancreatic β-cells are particularly sensitive to oxidative stress-mediated damage when compared with other cell types. Consequently, the imbalanced redox state brings about impaired pancreatic β-cell functions and further interrupts insulin secretion potentially through reducing the expression level of related transcription factors, mainly pancreatic and duodenal homeobox-1 (PDX1) and V-Maf avian musculoaponeurotic fibrosarcoma oncogene homolog A (MafA).68 PDX1 is an essential insulin transcription factor in both the development of the pancreas and normal insulin secretion. Increased oxidative stress can reduce the transcriptional activity of PDX-1 by inhibiting its translocation from the cytoplasm to the nucleus and this process is associated with the activation of JNK, through forkhead box O1 (FOXO1).70 Similarly, MafA, another important transcriptional factor for cell differentiation in the pancreas and insulin secretion71 was found to be downregulated in glucotoxic pancreatic cells. It was further reported that the use of antioxidants can preserve its protein expression and its binding to the insulin promoter, hence underscoring the role of oxidative stress in pancreatic dysfunction.72 In addition, overexpression of uncoupling protein 2 (UCP2), which is known to be upregulated in pancreatic islet patients with T2DM, could result in the reduction of insulin secretion through decreased mitochondrial function and increased ER stress through an oxidative stress-mediated pathway.73 Therefore, the ability to suppress ROS production and to remove ROS from the current overall oxidative environment is crucial for the survival of β-cells and the restoration of their functions.
Resveratrol has been reported to improve redox balance and attenuate the apoptosis and dysfunction of pancreatic cells. In isolated human islets, resveratrol significantly reduced hypoxia and oxidative stress-induced pancreatic cell apoptosis.66 In parallel, increased ROS, reduced non-enzymatic antioxidants such as glutathione (GSH) and vitamin C in plasma, along with diminished activities of superoxide dismutase (SOD), catalase (CAT), glutathione peroxidase (GPx), and glutathione-S-transferase (GST) in the pancreas, were observed in diabetic rats. However, resveratrol treatment reversed the aforementioned diminished enzyme activities and significantly reduced lipid peroxide, hydroperoxides, and glycated proteins nearly to normalcy,74 which may be attributed to their regulatory effects on the following aspects. Firstly, in diabetic rats, resveratrol significantly reduced serum AGE level75 and RAGE expression in organs,76,77 thus suppressing ROS production. However, up to now, there is no report regarding the effect of resveratrol on pancreatic RAGE expression. Therefore, its direct effect on the pancreas needs further confirmation. Secondly, studies have suggested that in hyperglycemic or diabetic mice, resveratrol administration can not only promote Nrf2 phosphorylation in the pancreas50 and the liver and muscle tissues,78 but also normalize renal expression of Nrf2/Keap1 and their downstream targets.75 Furthermore, recovery of oxidative stress-influenced insulin secretion was noticed after resveratrol treatment, evidenced by (1) the elevated PDX-1 expression via activation of silencing information regulator 2 related enzyme 1 (Sirt1)79 in glucose-stimulated cells and via activation of PPAR-γ and Nrf2 in mice with pancreatic dysfunction;78 and (2) the reinstated reduction in transcriptional activity of MafA via activation of Sirt1 in pancreatic β cells with palmitate-induced dysfunction.80
Similarly, the administration of pterostilbene has been shown to reduce oxidative stress via inhibiting the accumulation of AGEs in both the liver and serum.58 It also activated anti-oxidative genes (heme oxygenase-1, SOD, CAT, and GPx) and increased pancreatic Nrf2 expression, thus preserving pancreatic structure and insulin secretion. Moreover, pterostilbene can promote Nrf2 binding to ARE, further inducing the expression of a set of cytoprotective genes.62
3.2.2.2 Improvement of cytokine-induced β-cell inflammation and related damage.
Besides the stress brought about by ROS, the elevated level of inflammatory cytokines has been considered as one of the major causes for the impaired functions and apoptosis of pancreatic β-cells. Islet inflammation, characterized by increased immune cell infiltration, cell death, and others, are observed under diabetes, and this inflammation is associated with hyperglycemia.4 Studies have proposed a role of macrophage-secreted cytokines in this process as cytokine treatments including interleukin 1β (IL-1β), tumor necrosis factor α (TNF-α), and interferon gamma (IFN-γ) can result in a marked decrease in islet dysregulation possibly through nuclear factor kappa B (NF-κB)-induced excessive nitric oxide (NO) production.81–83 Concordantly, a significant reversal occurred with the addition of a competitive NO synthase inhibitor, proving the essential role of NO in cytokine-induced β-cell apoptosis,83 as elevated NO may result in a disrupted mitochondrial membrane potential that causes bioenergetic stress, increased BAX/Bcl-2 ratio (indicator for increased apoptosis) and activation of executioner protease for cell apoptosis, caspase-3.63 Therefore, the ability to reduce the levels of macrophage-secreted cytokines and suppress their following pathways should be helpful in reducing inflammation-induced β-cell damage, including apoptosis and loss of functions.
Administration of resveratrol has been reported to lower the levels of cytokines and ameliorate cytokine-induced inflammation in vitro and in vivo. Resveratrol has been proved to suppress macrophage infiltration to pancreatic islets in diabetic rats.84 Moreover, research proposed that the shift of overall macrophage polarity towards the pro-inflammatory M1-like phenotype contributes to islet inflammation and further β-cell dysfunction,85 yet resveratrol effectively prevented the accumulation of macrophages in skeletal muscle tissue while promoting the M2 polarization of macrophages rather than M1,86 thus reducing cytokine production and related inflammation. However, no data regarding the M1/M2 macrophages in the pancreas after supplementation of resveratrol are currently available and future experiments are needed. Resveratrol also reduced the expression of Toll-like receptor 2 (TLR2) and/or TLR4 in skeletal muscles and liver, which are essential mediators of inflammation in both muscles and islets.86–88 In line with this, resveratrol reinstated the elevated levels of cytokines in different tissues of diabetic mice, including TNF-α, IL-1β, and interleukin 6 (IL-6).84,89,90 Through all these improvements in the whole body, overall systemic inflammation may be attenuated, hence reducing the exposure of pancreatic β-cells to cytokines. Moreover, resveratrol can directly protect β-cells by inhibiting transcriptional NF-κB activity and suppress downstream nitric oxide synthase (iNOS) expression and NO production, potentially via the activation of PPAR-γ and Sirt1.91,92
Studies have reported similar inhibitory effects of pterostilbene on cytokine production and pancreatic apoptosis. Administration of pterostilbene was found to reduce serum TNF-α levels and increase the M1/M2 macrophage ratio in adipose tissue.93 It also significantly enhanced the protein expression of PPAR-γ, PI3K, and p-Akt64 and inhibited JNK activation,59 which may suppress NF-κB expression and ameliorate inflammation. In addition, pterostilbene treatment was shown to reduce NO production in the pancreas of diabetic mice via inhibition of iNOS while attenuating islet cell apoptosis, demonstrated by a reduced BAX/Bcl-2 ratio and caspase 3 level.63 Administration of oxyresveratrol also improved pancreatic inflammation and insulin secretion.67
Although currently there is no comparative study for anti-inflammatory effects between resveratrol and its analogues in islet cells, related data are available from an experiment that applies microglial neurological cells, in which excessive NO was also proposed to mediate macrophage-dependent inflammation. In this study, pterostilbene demonstrated the strongest ability to reduce the level of LPS-induced proinflammatory cytokine secretion, followed by THSG, oxyresveratrol and resveratrol. Pterostilbene also exhibited the best suppression effect on NO production, followed by resveratrol, oxyresveratrol and THSG.94
3.2.3 Influence on the insulin downstream pathway.
The improvement by stilbenes in IR, insulin production and secretion has been addressed previously, but the mechanisms through which these changes affect glucose homeostasis remain to be elaborated in detail. In fact, they may be bridged by the modulation of glucose uptake and metabolism in the responding organs and tissues. GLUT4, an essential transporter in the glucose uptake of muscles and adipose tissue, contributes to the control of whole-body glycemia critically. Commonly, GLUT4 is distributed in the plasma membrane and several intracellular compartments including endosomes and vesicles. Upon insulin stimulation, GLUT4 could be released from these intracellular compartments via exocytosis and get further transported to the plasma membrane, thus enhancing the shuttling of glucose into the muscles and adipose tissue.95 The activation of the PI3K/Akt cascade through the insulin signalling pathway, which is previously mentioned in section 3.2.1, is known to stimulate such GLUT4 translocation to the plasma membrane. Besides PI3K, Akt can also be activated by AMP-activated protein kinase (AMPK) under stresses and its activation can lead to the phosphorylation, in other words, the deactivation of FOXO1, a major regulator that promotes the expression of gluconeogenic genes and glucose production.96 In addition, PPAR-γ coactivator (PGC-1α), another essential transcriptional factor for gluconeogenic actions, also requires FOXO1 to exert robust functions.97 Therefore, the regulation of Akt by insulin and other bioactive compounds can greatly affect the utilization and metabolism of glucose in the liver. Key genes involved in these processes are discussed in section 3.2.3.2.
3.2.3.1 Modulation of glucose uptake in insulin-responding organs and tissues.
The modulatory effect of resveratrol on glucose uptake has been illustrated in several studies. In mice with IR, resveratrol treatment successfully restored the insulin-mediated glucose uptake98 and such an effect may be attributed to the upregulation in the expression of GLUT4.99 In cells or animals with IR, a reduction in GLUT4 expression was observed yet resveratrol greatly reversed the reduction in the muscles and adipocytes.98–101 Concordantly, these effects were ascribed to the activation of the Sirt1-pAMPK-pAkt and Sirt1-pIRS-pAkt pathways, which further promote the GLUT4 translocation to the plasma membrane, hence improving insulin sensitivity and promoting glucose uptake in the responding tissues.49,100 In addition, an elevated level of GLUT2, another major glucose transporter that serves as an important regulator of hepatic glucose output, was reported in the liver of diabetic mice102,103 or IR mice,104 yet such a change was restored by resveratrol, which potentially led to a direct reduction in hepatic glucose efflux and an improvement in glycemic control.103,105
Similar modulatory effects of the other four selected stilbenes on glucose uptake were also recorded. In insulin-resistant and diabetic rats fed with pterostilbene, the expression of GLUT4 was upregulated due to the elevated phosphorylation of Akt.106 Research demonstrated that the cytokine cardiotrophin-1 can regulate glucose homeostasis via the activation of Akt107 and increased PPAR-γ was potentially involved.64 In regard to piceatannol, an enhancement in glucose uptake was also reported, possibly through increased phosphorylation of IRS-1 in the insulin signalling pathway,108 upregulation of Akt and FOXO1 phosphorylation,109 promotion of GLUT4 translocation via AMPK activation110 and increased hepatic insulin receptors.111 For oxyresveratrol, increased GLUT 4112 was observed along with elevated glycogen content in supplemented mice, suggesting the stimulation of hepatic glucose uptake and glycogen storage. Meanwhile, PM extract rich in THSG was shown to enhance insulin signalling via the insulin receptor-α/IRS-1/GLUT4 pathway.113
3.2.3.2 Modulation of hepatic glucose metabolism.
Besides glucose deposition after food digestion and absorption, glucose metabolism, consisting of glycolysis, gluconeogenesis and glycogenesis and glycogenolysis, is also important in maintaining glucose homeostasis. Elevated hepatic glucose production, mainly resulting from abnormal glyconeogenesis and glycogenolysis, is a major contributor to long-term hyperglycemia, and several rate-limiting hepatic enzymes including glucose-6-phosphatase (G6Pase) and phosphoenolpyruvate carboxykinase (PEPCK) are involved.52,105 Meanwhile, glucokinase (GK), a key enzyme in both glycogen synthesis and glycolysis, and pyruvate kinase (PK), a rate-limiting enzyme in glycolysis, are both inhibited in diabetic rats. Since hepatic glycogen is one of the main storage forms of glucose in the body, suppressed GK activity will result in reduced glucose utilization. In parallel, decreased PK activity can lead to reduced glucose consumption and metabolism; hence the imbalance between total glucose production (from both dietary uptake and endogenous production) and glucose utilization exacerbates hyperglycemia and compromises glucose homeostasis.99 A decrease in the activity of these enzymes and the mRNA expression of gluconeogenic coding genes for PEPCK and G6Pase, pepck1 and g6pc, was noticed after resveratrol treatment52,99,105 whereas a boost in the activity of glycolytic enzymes including GK and PK, which facilitated the utilization and clearance of glucose in the blood, was reported.99 Therefore, by suppressing hepatic glucose production and promoting glucose consumption, resveratrol effectively reduced the net hepatic glucose output and assisted the regulation of glucose homeostasis.
Such alterations in enzymatic activities were also seen after the administration of other selected stilbenes. Pterostilbene reduced the production of glucose via inhibiting the activity of G6Pase and PEPCK in diabetic rats62,114 and such effects may be partially attributed to the activation of AMPK and subsequent downregulation of FOXO1 and PGC-1α based on the result of cell study.59,115 For both trans and cis-THSG, significant suppression in the transcription of PEPCK was observed in HepG2 cells, implying their potential use as hypoglycemic agents.116 Meanwhile, an improvement in glucose utilization was reported after the stilbene supplement. While both pterostilbene and oxyresveratrol increased the activity of glucokinase,106,117 pterostilbene also markedly promoted the activity of three other glycolytic enzymes, namely hexokinase, glucose-6-phosphate dehydrogenase and fructose-1,6-biphosphates, in diabetic mice,62 further increasing glycolysis and the usage of glucose for energy production. For piceatannol and oxyresveratrol, upregulation of PGC-1α was also recorded.111,112
3.3 Improvement of gut permeability and modulation of gut microbiota
As previously described, relatively low bioavailability is a common feature of resveratrol and some derivatives, majorly due to poor aqueous solubility and extensive metabolism. Hence, increased attention to stilbenes has been paid to the influence on the gut and the interaction with gut microbiota, as a great part of the stilbenoids is metabolized in the gut.118 Studies have proposed that resveratrol, pterostilbene, and THSG can alleviate hyperglycemia through protecting the intestinal barrier and modulating gut microbiota, which are detailed below.
3.3.1 Improvement of gut permeability and related intestinal inflammation.
Loss of gut barrier integrity can facilitate the progression of IR and cause damage in the islets by not only allowing the progressive leakage of antigens, pathogens, and microbial metabolites from the lumen to the blood that further leads to endotoxemia119,120 and potentially systemic inflammation121–123 but also triggering the activation of islet-reactive T cells.124 Therefore, the ability to improve gut permeability and reduce intestinal inflammation should be important targets of hypoglycemic treatment.
Resveratrol and pterostilbene have been shown to enhance barrier functions that were once disrupted by hyperglycemia via increasing tight junction proteins (TJPs) and promoting the proliferation of intestinal epithelial cells. TJPs, mainly consisting of occludins, claudins, and zonula occludens (ZO), seal the adjacent intestinal epithelial cells (IECs) with junction adhesion molecules to form a strict defence line. Upon the dysregulation of TJPs, luminal microbes may enter the circulation through passing between the IECs.121 Reduction of TJP expression was observed in IR mice, yet resveratrol administration partially reversed such changes.125,126 In line with this, resveratrol can restore the impaired production of TJPs in IECs by improving the activity of SOD, CAT, and GPx via the P13K/Akt-mediated Nrf2 signalling pathway and inhibiting the activation of protein kinase C (PKC) and p38 mitogen-activated protein kinase (MAPK) phosphorylation possibly by the upregulation of heme oxygenase-1(HO-1).127,128 Moreover, a similar modulatory effect on TJPs was observed in mice with severe intestinal barrier defects, which potentially involved inhibiting the phosphorylation of multiple inflammatory signalling molecules, including NF-κB, stress activated-phosphokinase (SAPK)/JNK and extracellular signal-regulated kinase (ERK) 1/2.126 These results proved that resveratrol helped to maintain the integrity of the gut barrier under various challenges. Likewise, injection of pterostilbene was reported to alleviate the intestinal damage and inflammation resulting from lipopolysaccharides (LPS) challenge in broiler chickens and this might be attributed to its resistance to oxidative stress.129 Reduced phosphorylation of p38 MAPK and ERK1/2 was also proposed as the mechanism for attenuating the effect of pterostilbene on the gut barrier.130 Further experiments are still needed to confirm the involvement of these pathways in the regulation of TJPs by resveratrol and pterostilbene using IR or diabetic animal models.
Regulatory effects of oxyresveratrol on gut permeability were also reported in recent studies, manifesting the enhanced production of TJPs and mucin in Caco-2 cells and goblet cells after exposure to oxyresveratrol or conditioned culture with oxyresveratrol.131–133 Such effects might be associated with the activation of the PKC and MAPK pathways132 and the upregulation of trefoil factor 3.133
3.3.2 Modulation of gut microbiota.
The gut microbiota plays a key role in the modulation of the host immune system and the development of organs and host physiology. A large body of evidence demonstrated that the dysbiosis of gut microbiota is associated with the development of hyperglycemia and diabetes, yet most results remain observational without determining a causal relationship between these two.120,134 A previous study has shown that in contrast to normal mice on an HFD, germ-free mice were protected from IR and gained less weight when fed the same diet, yet this effect was abolished and they developed IR and glucose intolerance after receiving a fecal transplant from conventionally raised mice, indicating that the gut microbiota was closely related to the development of persistent hyperglycemia and related diseases.135 Recent studies regarding the dietary intervention of selected stilbenes indicated their ability to partially abort microbial changes induced by hyperglycemia and to manipulate the structure of the microbial profile, functional pathways, and metabolome in a way that was different from normal mice.
3.3.2.1 Stilbenes modulated microbiota composition, metabolome, and functional pathway.
Modulation in the structural profile of the gut microbiota was noticed after supplement of resveratrol in several studies. Compared with obese/diabetic mice, a reduction in the Firmicutes to Bacteroidetes ratio was observed in normal or hyperglycemic mice that received resveratrol treatment,136 yet the alteration in specific microbiota varied from one study to another. Detailed changes in microbial structure in each study are listed in Table 4. Generally, the promotion of certain beneficial bacteria by resveratrol has been observed, including Parabacteroides and Alistipes, which have been found to be negatively correlated with inflammation, IR or obesity, some other short-chain fatty acid (SCFA)-producing bacteria such as Ruminococcaceae, Blautia, Alloprevotella, and Odoribacter and other microbes.137,138 Generated by microbes in the fermentation of non-digestible carbohydrates, SCFAs are considered as signalling molecules in the crosstalk between the host and the gut microbiota, exerting significant metabolic functions and a crucial impact on intestinal health. They are essential substrates in maintaining the mucosal integrity of the gut and in improving insulin sensitivity by modulating metabolism in the adipose, liver, and muscle tissues.139 Meanwhile, the suppression of the growth of some other bacteria was observed after resveratrol treatment, such as of Lachnospiraceae, Enterococcus and Proteobacteria,136,140,141 indicating an improved disease state as such microbes are commonly related to obesity and other metabolic disorders.142–144 In particular, a bloom of Proteobacteria is often viewed as characteristic of diseases like intestinal inflammation and it was positively correlated with the levels of two pro-inflammatory substances, phosphatidylcholines and sphingomyelin, in obese mice with hyperglycemia.136,140
Table 4 Changes in microbial structure and related key functions caused by resveratrol, pterostilbene and THSG treatment
Animal experiment |
Dose/method/period |
Changes of key microbes |
Examples of key functions |
Reported effects |
Ref. |
Resveratrol |
db/db and db/dm mice |
10 mg kg−1 daily for 12 weeks via oral gavage |
Firmicutes/Bacteroidetes↓ |
NA |
Intestinal barrier function↑ |
137
|
Bacteroides, Alistipes, Alloprevotella, Odoribacter, Parabacteroides, Rikenella↑ |
Intestinal permeability and inflammation↓ |
Bacterial richness, α-diversity↑ |
Male db/db mice (FMT) |
200 μL microbe suspension via oral gavage for 7 days |
Firmicutes, Tenericutes, Deferribacteres↓ |
NA |
Improved renal functions↑ |
137
|
Turicibacter, Alistipes, Odoribacter, Rikenella↑ |
Intestinal characteristics↑ |
Enterococci↓ |
Inflammatory response↓ |
(IL-6, IL-1β, IFN-γ and TNF-α)↓ |
High-fat diet-fed mice |
300 mg kg−1 daily for 16 weeks via intragastric injection*** |
Firmicutes/Bacteroidetes↓ |
NA |
Changed microbial structure |
138
|
Blautia, Parabacteroides, Lachnoclostridium, Lachnospiraceae_NK4A136_group, Ruminiclostridium_9↑ |
High-fat diet-fed mice (FMT) |
200 μL microbe suspension via oral gavage for 16 weeks |
Bacteroidetes↑ |
NA |
Insulin sensitivity↑ weight gain↓ |
138
|
Proteobacteria↓ |
Intestinal barrier integrity and function↑ |
Desulfovibrio, Ruminiclostridium, Anaerotruncus, Oscillibacter↓ |
Improvement of intestinal morphology |
Allobaculum↑ |
Reduced oxidative stress and inflammation↓ |
High-fat diet-fed mice |
300 mg kg−1 daily via oral gavage for 16 weeks*** |
Bacteroidetes↑ |
CAZy subsystem relating to degradation of dietary plant polysaccharide↑ |
Body weight↓, insulin resistance↑ |
141
|
Blautia, Lachnoclostridium↑ |
Gene-encoding products associated with carbohydrate, amino acid and energy metabolism↑ |
mRNA expression of lipogenic gene and thermogenic genes↑ |
Desulfovibrio↓ |
Reversed HFD-induced enrichment in nutrient processing, including phosphotransferase system (PTS), ATP-binding cassette (ABC) transport, methane metabolism, LPS biosynthesis and carbohydrate metabolism |
Intestinal ROS level↓ |
Lachnospiraceae_NK4A136_group↓ |
Amino acid cofactor and vitamin metabolism↑ |
Pathway involved in small metabolite generation↑ |
High-fat diet-induced mice (FMT) |
200 μL microbe suspension daily via oral gavage for 16 weeks |
Allobaculum, Bacteroides and Alistipes↑ |
Pathway involved in small metabolite generation↑ |
Fasting glucose; insulin concentration↓ |
141
|
Desulfovibrio↓ |
Promotion in gut metabolite production in amino acid biosynthesis and metabolism such as glycolic acid, tartaric acid, aminomalonic acid and 4-HPP |
Glucose and insulin tolerance↑ |
mRNA expression level of lipogenic, fatty acid oxidation and thermogenic genes↑ |
Intestinal barrier integrity and function↑ |
High fat/high sugar diet-fed mice |
0.4% Resveratrol in diet for 8 weeks |
Turicibacteraceae, Moryella, Lachnospiraceae, Akkermansia↓ |
Top discriminative microbial pathways include carbohydrate, amino acid and energy metabolism, along with replication and repair pathways |
Improved glucose homeostasis |
140
|
Bacteroides, Parabacteroides↑ |
Altered acylcarnitine and phosphatidylcholine metabolism |
Firmicutes/Bacteroidetes↓ |
High fat/high sugar diet-fed (FMT) |
200 μL microbe suspension via oral gavage at day 1, 3, 5 |
Allobaculum, Bacteroides and Alistipes↑ |
Glycan biosynthesis, genetic information processing, and replication and repair pathways↑ |
Improve glucose homeostasis without reduction in body weight; alteration in metabolic profile: butyrylcarnitine (C4)↓; (independent of direct supplementation of SCFAs) |
140
|
Desulfovibrio↓ |
High-fat diet-fed mice (FMT) |
50, 75 and 100 mg kg−1via drinking water, 16 weeks |
Firmicutes/Bacteroidetes↓ |
NA |
Reduced blood glucose level |
136
|
Enterococcus faecalis↓ |
Reduced serum triglyceride level, cholesterol and LDL protein |
Desulfovibrionaceae↓ (compared with HFD group) |
Improved antioxidative state and inflammation Increased gut microbiota diversity |
Pterostilbene |
Zucker (fa/fa) rats |
15 mg kg−1 daily via oral gavage for 6 weeks |
Firmicutes, Gammaproteobacteria↓ |
NA |
Improved glucose tolerance and HOMA-IR index; reduction in serum insulinAttenuated adiposity |
145
|
Verrucomicrobia, Rikenellaceae↑ |
Odoribacter, Akkermansia↑ |
THSG |
HF-HSD induced IR rats |
12 mg kg−1 daily via oral gavage for 12 weeks |
Firmicutes/Bacteroidetes↓ |
Amino acid metabolism↑ |
Relieved insulin resistance |
146
|
Parabacteroides↓ |
Energy metabolism↑ |
Fasting blood glucose↓ |
Bacteroides spp.↑ |
Glucose tolerance↑ |
Bifidobacterium spp.↑ |
Clostridium spp.↓ |
Desulfovibrio spp.↓ |
Oscillibacter spp.↓ |
A consequentially profound impact brought by the shift in microbial structure is the marked separation of the gut metabolome. Apart from SCFAs, phosphatidylcholines and sphingomyelin mentioned before, some other metabolites also showed a strong correlation with microbial composition. In a recent study, non-targeted metabolomic analysis in faeces has identified a significant increase in the abundance of metabolites involved in amino acid biosynthesis and metabolism after resveratrol treatment, among which 4-hydroxyphenylacetic acid (4-HPA) and 3-hydroxyphenylpropionic acid (3-HPP) appeared to be the gut metabolites of resveratrol that improve lipid metabolism. Consistently, metabolites involved in fatty acid and methane metabolism, which were once elevated in obese mice, were restored to a normal level, including palmitic acid, stearic acid, and cholesterol.141 In another study, acylcarnitine was proposed to correlate with Verrucomicrobiota and Actinobacteria.140
An alteration in dominant functional pathways was also recorded in a few studies. Sung et al. (2017)140 predicted a shift in dominant pathways from bacterial chemotaxis, flagellum assembly and motility in obese mice to carbohydrates, amino acids, and energy metabolism as well as replication and repair pathways in resveratrol-fed obese mice. However, a significant enrichment of carbohydrate metabolism, along with other new pathways in obese mice and a further reversal by resveratrol treatment, was reported by Wang and collegues.141
Likewise, in pterostilbene-fed obese mice, an alteration in microbial profile was observed from phylum to genus or further species level, yet the description of a pterostilbene-induced change in gut microbiota is rather limited due to the lack of research. A similar reduction in Firmicutes to Bacteroidetes ratio and a significant increase in Verrucomicrobia at the phylum level and Rikenellaceae at the family level were observed in obese rats fed with pterostilbene. In particular, certain SCFA-producing bacteria were enriched by pterostilbene treatment, including Odoribacter splanchnicus, which has a strong inverse correlation with body weight and Akkermansia muciniphila, which was negatively correlated with adiposity.145 Depletion of Gammaproteobacteria was also reported after pterostilbene treatment.145
Changes in gut microbiota after THSG treatment were also observed, although only one report was published using IR rats. Like the other two stilbene compounds mentioned above, dietary intervention with THSG resulted in a reduction in the Firmicutes to Bacteroidetes ratio. It also significantly elevated the abundance of some beneficial bacteria such as Bacteroides spp. and Bifidobacterium spp. while reducing the levels of Clostridium spp., Desulfovibrio spp., and Oscillibacter spp. In addition, KEGG metabolic pathway analysis reported that under IR conditions, the pathway related to amino acid metabolism was downregulated. This was reversed by THSG, concomitantly with the upregulation of genes in the energy metabolism pathway.146
3.3.2.2 Hypoglycemic effect of stilbenes was partially mediated through gut microbiota.
Although alterations in bacterial profile were observed after treatment with stilbenoid compounds, it remains to be clarified whether the hypoglycemic effects exhibited by these compounds depends on the selectively altered microbiota. While the exact mechanisms linking microbes to hyperglycemia and related diseases need further exploration, these changes in microbial composition are believed to cause corresponding functional changes and convey the benefits of dietary supplementation via multiple mechanisms, which can be proved by conducting fecal microbial transplantation (FMT).120
Transplantation of gut microbiome from resveratrol-fed donor mice to diet-induced obese or diabetic mice was able to reproduce multiple modulatory effects once seen in mice directly given resveratrol treatments, including improved glucose homeostasis, insulin sensitivity, intestinal permeability, as well as systemic inflammation and oxidative stress.137,138,140,141 Microbiota collected from resveratrol-fed donor mice was suggested to exert benefits on recipient mice through two aspects. One was to protect the gut barrier in the recipients via the reduction in proinflammatory cytokines, ROS and malondialdehyde (MDA), enhancement in SOD and total antioxidant capacity, as well as upregulation of TJPs and major mucin genes and related transcription factors resistin-like molecule β and regenerating islet-derived protein 3 gamma (Reg3γ).138 The increased expression of mucins, along with antimicrobial peptide Reg3γ, which can influence the structure of the microbial community and promote spatial segregation in the outer mucus layer,147 are believed to reinforce the whole mucus barrier covering the luminal surface of the epithelial cells and prevent the colonization of the epithelium by gut microbiota and potential pathogens. Another aspect was that the FMT process altered the microbial structure in recipient mice to a certain extent, which is consistent with resveratrol-fed donor mice. For instance, an increased Bacteroidetes to Firmicutes ratio and some SCFA-producing bacteria were also observed in mice that received FMT.137,140 In general, these results indicated that changes in the microbiota at least partially mediated the overall benefits brought about by the administration of resveratrol. However, to determine whether the direct impact of resveratrol on the intestinal environment outweighs the effect mediated by microbes, future experiments applying germ-free mice or antibiotic treatment will be needed.
3.4 Improvement of the circadian clock
To adapt to the cyclic environment, notably the natural light–dark cycle and fluctuation of the temperature, organisms have evolved a circadian rhythm to align biological processes including metabolism, energy expenditure and food intake to external changes. The synchronization of the endogenous timing system in both the central circadian and peripheral circadian rhythm is fundamental for proper and robust homeostasis within the body and the misalignment of such a system may negatively impact the overall health condition. The impact of circadian rhythm on wellness was previously reviewed by others.148 In brief, the crosstalk between the circadian clock and metabolism is achieved through a transcriptional-translational feedback loop consisting of several clock genes, such as circadian locomotor output cycles kaput protein (CLOCK), brain and muscle arnt-like protein-1 (BMAL1), period proteins (PERs) and cryptochromes (CRY). The heterodimer of BMAL1 and CLOCK activates the transcription of many downstream genes including Per and Cry, the accumulation of which would result in new dimer formation and in turn give negative feedback. Together with other loops, rhythmic oscillations in gene expression are formed.
Currently, limited research and data are available to reveal the underlying molecular mechanisms associating circadian rhythm and hyperglycemia, yet many observational studies supported this theory. For instance, consuming a greater share of total calories early in the day improved insulin resistance compared with a longer feeding period.149 It was also demonstrated that from the meta-analysis of observational studies, people with shift work had a 9% higher risk of diabetes when compared with people without shift work.150,151 Furthermore, the risk of diabetes for people with rotating shifts is even higher than in ones with fixed shifts.152 Since circadian rhythms were observed for many components involved in the action of insulin,148 it is not surprising that circadian misalignment or disruption by interfering behavioural cycles or external cues can result in the decrease of insulin sensitivity and further IR.149 However, treatment with resveratrol was shown to elevate insulin level or attenuate IR by improving disturbed circadian rhythms in mice exposed to various interferences,153 in human skeletal muscle,154 in FFA-treated HepG2 cells155 and in HFD treated-mice.156–158 These processes may involve the activation of Sirt1, which is also an important downstream circadian regulator in insulin signaling, secondly, the recovery of bidirectional communication between inner-mitochondrial genes and rhythmic gene expression154 and lastly, the modulation of glucose and lipid metabolism via regulating BMAL1-dependent phosphorylation of acetyl-CoA carboxylase, AMPK, AKT and IRS-1.155
Similar regulatory effects on circadian rhythm were also observed after treatments with pterostilbene and oxyresveratrol. Pterostilbene has been shown to decrease fasting blood glucose and ameliorate circadian misalignment in sleep-restricted mice via restoring the rhythm of AMPK phosphorylation activity, Sirt1 and PGC-1α deacetylation activity, which in turn interact with clock genes.159 Moreover, pterostilbene managed to prevent increased food intake and weight gain as well as produce a recomposition of gut microbial profiles in mice with circadian rhythm dysregulation.160 Likewise, in PER::LUC mice with a high fat diet, a significant delay in the phase change of PER2 had been observed and the administration of oxyresveratrol successfully advanced the rhythm, thus potentially preventing circadian disturbance.161 Hence, since circadian rhythms are closely interrelated with overall metabolism, the effects of stilbenes against circadian dysregulation highlight their potential as precautionary therapeutics, especially under various disruptions stemming from changes in modern lifestyle.
4. Human studies of resveratrol and related stilbenes in managing diabetes and its complications
According to the review published by Zhu et al. in 2017,162 a systemic review and meta-analysis of data from 9 previous clinical trials showed that resveratrol significantly improved the fasting plasma glucose and insulin level, as well as HOMA-IR index while showing no significant effect on low or high-density cholesterol. The author also pointed out that resveratrol treatment >100 mg day−1 revealed a significant difference in fasting plasma glucose. To further investigate the effect of resveratrol, clinical trials of resveratrol on diabetes and some diabetic complications published mainly in the past 5 years were reviewed here. In total, 7 clinical trials are found. The majority of them are randomized, double-blind and placebo-controlled trials. Among these trials, except one study that reported no measurable effects of resveratrol in T2DM patients163 and another study that only reported changes in oxidative stress markers,164 all other studies recorded positive results upon resveratrol administration, showing that resveratrol alone, or together with another anti-diabetic drug, can improve insulin resistance, increase antioxidant capacity, and reduce the levels of insulin, proinflammatory cytokines and blood glucose.165–169 Variation in the modulation of biomarkers may be observed in different trials and to better compare the method, duration, dosage and outcome among trials, related information is listed in Table 5. In general, the number of participants ranges from 13–112 people, with the dosage varying from 100 mg day−1 to 1000 mg day−1 for a duration of 1–6 months. In most studies, a dosage of 500 mg day−1 was applied, and no major adverse reactions were reported. In particular, resveratrol administration significantly contributed to the reduction in urinary albumin excretion when patients with diabetic nephropathy were cotreated with the drug losartan, thus proving the potential usage of resveratrol as an effective adjunct for certain medicines.137
Table 5 Clinicals trials of effects of resveratrol on T2DM and related complications
Number of participants |
Dose/method period |
Anti-diabetic function |
Involvement of mechanisms |
Ref. |
Resveratrol |
N = 110 |
200 mg; daily basis; oral administration; 6 months |
Fasting blood glucose↓ |
Downregulation of micro RNA 34a, 375, 21 and 196 |
165
|
Double-blind, randomized, placebo-controlled |
Insulin level↓ |
Upregulation of micro RNA 126 and 132 |
Insulin resistance↓ |
Inflammatory cytokines↓ |
N = 48 |
800 mg; daily basis: oral administration; 2 months |
No significant changes in plasma glucose, insulin and HOMA-IR compared with control |
Increased plasma total antioxidant capacity |
164
|
Double-blind, randomized, placebo-controlled |
Increased Nrf2 and SOD expression |
N = 13 |
500 mg twice daily; oral administration; 2 months |
Significantly decreased in fasting blood glucose at 2 months but not 1 month |
Increased plasma total antioxidant capacity |
166
|
Exploratory clinical trials (type: N/A) |
No significant differences in insulin level and HOMA-IR |
Decreased MDA |
N = 60 |
500 mg, daily basis; oral administration; 3 months |
Reduced urinary albumin excretion in diabetic nephropathy |
N/A |
167
|
Double-blind, randomized, placebo-controlled |
Cotreatment with losartan |
N = 192 |
40, 500, daily basis; oral administration; 6 months |
No significant differences in all measured markers |
N/A |
163
|
Double-blind, randomized, placebo-controlled |
N = 472 |
500 mg, daily basis; oral administration 6 months |
Improved glucose metabolism and insulin tolerance |
Inflammatory cytokines↓ |
168
|
Single-blind, randomized, controlled |
N = 56 |
500 mg, daily basis, oral administration 1 month |
Fasting blood glucose↓ |
Increased total antioxidant capacity |
169
|
Double-blind, randomized, placebo-controlled |
Insulin sensitivity↓ |
Activation of PPAR-γ and Sirt1 |
Insulin resistance↓ |
In a few most recent clinical studies, some molecular alterations after resveratrol treatment were also explored. For instance, regulations in several micro RNAs, including micro RNA-34a which is a tumor suppressor, micro RNA-375 which regulates β cell development and function, and micro RNA-21 which regulates apoptosis and inflammation, among others, were observed.165 Also, the upregulation of Nrf2 was noticed.164
For other stilbenes, limited clinical trials were found, especially on hyperglycemia and diabetes. In a clinical study, patients with hypercholesterolemia were given 125 mg pterostilbene twice a day for 52 days and no adverse drug side effects on hepatic, renal and blood glucose markers were reported, proving that pterostilbene is safe for use at a dosage of 250 mg day−1.170 Another clinical trial has revealed the effect of pterostilbene in reducing blood pressure also at 250 mg day−1.171 For oxyresveratrol, no clinical study was found using the pure compound but two human studies with the administration of leaf extract of mulberry (in which oxyresveratrol is commonly present) reported significantly decreased postprandial plasma glucose levels and lower insulin levels compared with the control group within 120 min.172,173 In one of the studies, the intake of 3.3 g mulberry leaf extract per day successfully reduced the postprandial glucose from 209 mg dL−1 to 148 mg/mg dL−1 in the patients’ group and from 125 mg dL−1 to 97 mg dL−1 in healthy people.172
5. Conclusion
Over the past several decades, dietary intervention with natural bioactive compounds from plants has gained broad interest and was intensely investigated as an alternative approach to prevent and treat hyperglycemia, obesity, diabetes, and other metabolic complications. One type of representative compound is stilbenes, which include the most studied resveratrol and others that are less noticed, such as pterostilbene, THSG, piceatannol, and oxyresveratrol. A line of cell and animal studies has illustrated the potential hypoglycemic effect of these five stilbenes and their ability to improve IR. Both resveratrol and pterostilbene have been shown to exert bioactivity via multiple mechanisms, including reducing food intake, inhibiting nutrition absorption, regulation of glucose homeostasis by improving IR, insulin production, and downstream signalling targets, enhancing gut permeability and modulating gut microbiota, and ameliorating disruptions in circadian rhythm. To further confirm the effect of stilbenes on reducing food intake and inhibiting nutrition absorption, an in vivo study applying a diabetic mouse model should be conducted with detailed changes of biomarkers of satiety being recorded. Also, attention should be paid to future studies on the molecular mechanisms explaining the correlation between hyperglycemia and disrupted circadian rhythm, and consequently the future studies on the influence of stilbenes on these molecular mechanisms. In addition, research regarding the changes in microbiota structure brought about by stilbene administration and their impacts on the amelioration of symptoms has emerged in the last decade. However, the underlying mechanisms through which gut microbiota exhibit their benefits, and the importance of their existence remains obscure. Meanwhile, due to the influence caused by the environment, the resulting changes in specific microbial species in each report can vary dramatically and limit their value as reference standards. Therefore, future experiments using germ-free mice will be necessary and investigation should be extended to the level of single species or strains. Moreover, as the development of delivery systems can greatly improve the bioavailability of resveratrol and its derived stilbenes, more studies can be performed to construct an ideal system and investigate their potential application in the improvement and prevention of hyperglycemia-related diseases.
Of note, even though some research has also been conducted for THSG, piceatannol, and oxyresveratrol to explore and confirm these related hypoglycemic mechanisms, data are quite limited due to a dearth of reports. Therefore, further detailed investigations on these three stilbenes are warranted. Moreover, comparative studies applying multiple stilbenes would give more straightforward results when trying to select the stilbene with better hypoglycemic effects. A comprehensive measurement of related parameters, including all the aspects mentioned in this review, for both the liver, intestine, muscles and pancreas, will further contribute to a solid comparative study. Another important aspect is that dosage and duration should be carefully considered in the experimental design. While the applied dosage and duration of most animal studies were below the highest dosage and time applied in the human clinical trials (no obvious side effects are observed), some studies use much higher dosages for a long time. Therefore, the potential toxicity of such high dosages of stilbene should be verified for better results. Also, currently, there are no available clinical trials except for resveratrol and pterostilbene, with the latter only establishing a safety use limit up to 250 mg day−1. Therefore, more animal studies or clinical trials should be conducted to explore and establish the highest dosage and proper duration for future studies.
Author contributions
Jie Peng: conceptualization, writing – original draft, review & editing; Chenyang Lu: conceptualization, writing – review & editing; Yue Luo: investigation, writing – review & editing. Xiurong Su: supervision, funding aqusition. Chi-tang Ho: supervision, writing – review & editing. Shiming Li: conceptualization, supervision, writing – review & editing.
Conflicts of interest
The authors declare no conflicts of interest.
Acknowledgements
This work was supported by the National Key R&D Program of China (2018YFD0901102), Fund of State Key Laboratory for Managing Biotic and Chemical Threats to the Quality and Safety of Agro-products, Ningbo University (ZS20190105), and K.C. Wong Magna Fund of Ningbo University of China.
References
- P. Ramteke, A. Deb, V. Shepal and M. K. Bhat, Hyperglycemia Associated Metabolic and Molecular Alterations in Cancer Risk, Progression, Treatment, and Mortality, Cancers, 2019, 11, 1402 CrossRef CAS PubMed.
- R. Alfahel, T. Sawicki, M. Jabłońska and K. E. Przybyłowicz, Anti-Hyperglycemic Effects of Bioactive Compounds in the Context of the Prevention of Diet-Related Diseases, Foods, 2023, 12, 3698 CrossRef CAS PubMed.
- M. B. Davidson, Historical review of the diagnosis of prediabetes/intermediate hyperglycemia: Case for the international criteria, Diabetes Res. Clin. Pract., 2022, 185, 109219 CrossRef PubMed.
- M. Cnop, N. Welsh, J. C. Jonas, A. Jörns, S. Lenzen and D. L. Eizirik, Mechanisms of pancreatic beta-cell death in type 1 and type 2 diabetes: many differences, few similarities, Diabetes, 2005, 54(Suppl 2), S97–107 CrossRef CAS PubMed.
- J. P. Felber and A. Golay, Pathways from obesity to diabetes, Int. J. Obes. Relat. Metab. Disord., 2002, 26(Suppl 2), S39–S45 CrossRef CAS PubMed.
- E. C. Chao and R. R. Henry, SGLT2 inhibition–a novel strategy for diabetes treatment, Nat. Rev. Drug Discovery, 2010, 9, 551–559 CrossRef CAS PubMed.
- D. E. Moller, New drug targets for type 2 diabetes and the metabolic syndrome, Nature, 2001, 414, 821–827 CrossRef CAS PubMed.
- V. Moodi, S. Abedi, M. Esmaeilpour, O. Asbaghi, F. Izadi, M. Shirinbakhshmasoleh, M. Behrouzian, A. Shahriari, E. Ghaedi and M. Miraghajani, The effect of grapes/grape products on glycemic response: A systematic review and meta-analysis of randomized controlled trials, Phytother. Res., 2021, 35, 5053–5067 CrossRef CAS PubMed.
- L. M. Vislocky and M. L. Fernandez, Biomedical effects of grape products, Nutr. Rev., 2010, 68, 656–670 CrossRef PubMed.
- S. Nunes, P. Vieira, P. Gomes, S. D. Viana and F. Reis, Blueberry as an attractive functional fruit to prevent (pre)diabetes progression, Antioxidants, 2021, 10, 1162 CrossRef CAS PubMed.
- Q. Yuan and L. Zhao, The Mulberry (Morus alba L.) Fruit-A review of characteristic components and health benefits, J. Agric. Food Chem., 2017, 65, 10383–10394 CrossRef CAS PubMed.
- X. L. Yin, H. Y. Liu and Y. Q. Zhang, Mulberry branch bark powder significantly improves hyperglycemia and regulates insulin secretion in type II diabetic mice, Food Nutr. Res., 2017, 61, 1368847 CrossRef PubMed.
- P. Pecyna, J. Wargula, M. Murias and M. Kucinska, More than resveratrol: new insights into stilbene-based compounds, Biomolecules, 2020, 10, 1111 CrossRef CAS PubMed.
- B. Tian and J. Liu, Resveratrol: a review of plant sources, synthesis, stability, modification and food application, J. Sci. Food Agric., 2020, 100, 1392–1404 CrossRef CAS PubMed.
- X. Meng, J. Zhou, C. N. Zhao, R. Y. Gan and H. B. Li, Health Benefits and Molecular Mechanisms of Resveratrol: A Narrative Review, Foods, 2020, 9, 340 CrossRef CAS PubMed.
- M. Murias, W. Jäger, N. Handler, T. Erker, Z. Horvath, T. Szekeres, H. Nohl and L. Gille, Antioxidant, prooxidant and cytotoxic activity of hydroxylated resveratrol analogues: structure-activity relationship, Biochem. Pharmacol., 2005, 69, 903–912 CrossRef CAS PubMed.
- Y. Liu, Y. You, J. Lu, X. Chen and Z. Yang, Recent advances in synthesis, bioactivity, and pharmacokinetics of pterostilbene, an important analog of resveratrol, Molecules, 2020, 25, 5166 CrossRef CAS PubMed.
- W. S. Lin, J. V. Leland, C. T. Ho and M. H. Pan, Occurrence, Bioavailability, anti-inflammatory, and anticancer effects of pterostilbene, J. Agric. Food Chem., 2020, 68, 12788–12799 CrossRef CAS PubMed.
- R. W. Dellinger, A. M. Garcia and F. L. Meyskens Jr., Differences in the glucuronidation of resveratrol and pterostilbene: altered enzyme specificity and potential gender differences, Drug Metab. Pharmacokinet., 2014, 29, 112–119 CrossRef CAS PubMed.
- P. Wang and S. Sang, Metabolism and pharmacokinetics of resveratrol and pterostilbene, BioFactors, 2018, 44, 16–25 CrossRef CAS PubMed.
- I. I. M. Kapetanovic, M. Muzzio, Z. Huang, T. N. Thompson and D. L. McCormick, Pharmacokinetics, oral bioavailability, and metabolic profile of resveratrol and its dimethylether analog, pterostilbene, in rats, Cancer Chemother. Pharmacol., 2011, 68, 593–601 CrossRef CAS PubMed.
- J. A. Joseph, D. R. Fisher, V. Cheng, A. M. Rimando and B. Shukitt-Hale, Cellular and behavioral effects of stilbene resveratrol analogues: implications for reducing the deleterious effects of aging, J. Agric. Food Chem., 2008, 56, 10544–10551 CrossRef CAS PubMed.
- L. S. Lv, X. H. Gu, J. Tang and C. T. Ho, Antioxidant activity of stilbene glycoside from Polygonum multiflorum Thunb in vivo, Food Chem., 2007, 104, 1678–1681 CrossRef CAS.
- Y. Matsui, K. Sugiyama, M. Kamei, T. Takahashi, T. Suzuki, Y. Katagata and T. Ito, Extract of passion fruit (Passiflora edulis) seed containing high amounts of piceatannol inhibits melanogenesis and promotes collagen synthesis, J. Agric. Food Chem., 2010, 58, 11112–11118 CrossRef CAS PubMed.
- M. Dvorakova and P. Landa, Anti-inflammatory activity of natural stilbenoids: A review, Pharmacol. Res., 2017, 124, 126–145 CrossRef CAS PubMed.
- K. Likhitwitayawuid, Oxyresveratrol: Sources, Productions, Biological Activities, Pharmacokinetics, and Delivery Systems, Molecules, 2021, 26, 4212 CrossRef CAS PubMed.
- Y. Yang, G. Zhang, C. Li, S. Wang, M. Zhu, J. Wang, H. Yue, X. Ma, Y. Zhen and X. Shu, Metabolic profile and structure-activity relationship of resveratrol and its analogs in human bladder cancer cells, Cancer Manage. Res., 2019, 11, 4631–4642 CrossRef CAS PubMed.
- E. Cantos, J. C. Espín, M. J. Fernández, J. Oliva and F. A. Tomás-Barberán, Postharvest UV-C-irradiated grapes as a potential source for producing stilbene-enriched red wines, J. Agric. Food Chem., 2003, 51, 1208–1214 CrossRef CAS PubMed.
- M. Adrian, P. Jeandet, A. C. Douillet-Breuil, L. Tesson and R. Bessis, Stilbene content of mature Vitis vinifera berries in response to UV-C elicitation, J. Agric. Food Chem., 2000, 48, 6103–6105 CrossRef CAS PubMed.
- M. H. Yang, C. H. Kuo, W. C. Hsieh and K. L. Ku, Investigation of microbial elicitation of trans-resveratrol and trans-piceatannol in peanut callus led to the application of chitin as a potential elicitor, J. Agric. Food Chem., 2010, 58, 9537–9541 CrossRef CAS PubMed.
-
U.S Foof and Drug Administration, Guidance for industry: Estiminating the maximum safe starting dose in initial clinical trials for therapeutics in adult healthy volunteers, 2005. https://www.fda.gov/regulatory-information/search-fda-guidance-documents/estimating-maximum-safe-starting-dose-initial-clinical-trials-therapeutics-adult-healthy-volunteers Search PubMed.
- A. A. Bachmanov, D. R. Reed, G. K. Beauchamp and M. G. Tordoff, Food intake, water intake, and drinking spout side preference of 28 mouse strains, Behav. Genet., 2002, 32, 435–443 CrossRef PubMed.
- S. J. Kim, Y. H. Lee, M. D. Han, W. Mar, W. K. Kim and K. W. Nam, Resveratrol, purified from the stem of Vitis coignetiae Pulliat, inhibits food intake in C57BL/6J Mice, Arch. Pharmacal Res., 2010, 33, 775–780 CrossRef CAS PubMed.
- A. Dal-Pan, S. Blanc and F. Aujard, Resveratrol suppresses body mass gain in a seasonal non-human primate model of obesity, BMC Physiol., 2010, 10, 11 CrossRef PubMed.
- T. M. Dao, A. Waget, P. Klopp, M. Serino, C. Vachoux, L. Pechere, D. J. Drucker, S. Champion, S. Barthélemy, Y. Barra, R. Burcelin and E. Sérée, Resveratrol increases glucose induced GLP-1 secretion in mice: a mechanism which contributes to the glycemic control, PLoS One, 2011, 6, e20700 CrossRef CAS PubMed.
- S. S. Thazhath, T. Wu, M. J. Bound, H. L. Checklin, S. Standfield, K. L. Jones, M. Horowitz and C. K. Rayner, Administration of resveratrol for 5 wk has no effect on glucagon-like peptide 1 secretion, gastric emptying, or glycemic control in type 2 diabetes: a randomized controlled trial, Am. J. Clin. Nutr., 2016, 103, 66–70 CrossRef CAS PubMed.
- A. J. Zhang, A. M. Rimando, C. S. Mizuno and S. T. Mathews, α-Glucosidase inhibitory effect of resveratrol and piceatannol, J. Nutr. Biochem., 2017, 47, 86–93 CrossRef CAS PubMed.
- C. Carpéné, F. Les, G. Cásedas, C. Peiro, J. Fontaine, A. Chaplin, J. Mercader and V. López, Resveratrol anti-obesity effects: rapid inhibition of adipocyte glucose utilization, Antioxidants, 2019, 8, 74 CrossRef PubMed.
- E. F. Khaleel, G. A. Abdelaleem and D. G. Mostafa, Resveratrol improves high-fat diet induced fatty liver and insulin resistance by concomitantly inhibiting proteolytic cleavage of sterol regulatory element-binding proteins, free fatty acid oxidation, and intestinal triglyceride absorption, Can. J. Physiol. Pharmacol., 2018, 96, 1 CrossRef PubMed.
- M. Guschlbauer, S. Klinger, M. Burmester, J. Horn, S. E. Kulling and G. Breves, trans-Resveratrol and ε-viniferin decrease glucose absorption in porcine jejunum and ileum in vitro, Comp. Biochem. Physiol., Part A: Mol. Integr. Physiol., 2013, 165, 313–318 CrossRef CAS PubMed.
- S. Klinger and G. Breves, Resveratrol inhibits porcine intestinal glucose and alanine transport: Potential roles of Na+/K+-ATPase activity, protein kinase A, AMP-activated protein kinase and the association of selected nutrient transport proteins with detergent resistant membranes, Nutrients, 2018, 10, 302 CrossRef PubMed.
- C. Schulze, G. Kottra and H. Daniel, Inhibition of the sodium-coupled glucose transporter SGLT1 by plant extracts and metabolites derived from apple and grape vine, Genes Nutr., 2012, 6, S61 Search PubMed.
- M. Wongon and N. Limpeanchob, Inhibitory effect of Artocarpus lakoocha Roxb and oxyresveratrol on α-glucosidase and sugar digestion in Caco-2 cells, Heliyon, 2020, 6, e03458 CrossRef CAS PubMed.
- R. A. Haeusler, T. E. McGraw and D. Accili, Biochemical and cellular properties of insulin receptor signalling, Nat. Rev. Mol. Cell Biol., 2018, 19, 31–44 CrossRef CAS PubMed.
- M. C. Petersen, D. F. Vatner and G. I. Shulman, Regulation of hepatic glucose metabolism in health and disease, Nat. Rev. Endocrinol., 2017, 13, 572–587 CrossRef CAS PubMed.
- J. L. Rains and S. K. Jain, Oxidative stress, insulin signaling, and diabetes, Free Radicals Biol. Med., 2011, 50, 567–575 CrossRef CAS PubMed.
- D. M. Muoio and C. B. Newgard, Molecular and metabolic mechanisms of insulin resistance and β-cell failure in type 2 diabetes, Nat. Rev. Mol. Cell Biol., 2008, 9, 193–205 CrossRef CAS PubMed.
- P. Gual, Y. Le Marchand-Brustel and J. F. Tanti, Positive and negative regulation of insulin signaling through IRS-1 phosphorylation, Biochimie, 2005, 87, 99–109 CrossRef CAS PubMed.
- S. Chen, J. Li, Z. Zhang, W. Li, Y. Sun, Q. Zhang, X. Feng and W. Zhu, Effects of resveratrol on the amelioration of insulin resistance in KKAy mice, Can. J. Physiol. Pharmacol., 2012, 90, 237–242 CrossRef CAS PubMed.
- A. S. Cheng, Y. H. Cheng, C. Y. Lee, C. Y. Chung and W. C. Chang, Resveratrol protects against methylglyoxal-induced hyperglycemia and pancreatic damage in vivo, Nutrients, 2015, 7, 2850–2865 CrossRef CAS PubMed.
- L. Shu, G. Hou, H. Zhao, W. Huang, G. Song and H. Ma, Resveratrol improves high-fat diet-induced insulin resistance in mice by downregulating the lncRNA NONMMUT008655.2, Am. J. Transl. Res., 2020, 12, 1–18 CAS.
- L. Shu, H. Zhao, W. Huang, G. Hou, G. Song and H. Ma, Resveratrol upregulates mmu-miR-363-3p via the PI3K-Akt pathway to improve insulin resistance induced by a high-fat diet in mice, Diabetes Metab. Syndr. Obes., 2020, 13, 391–403 CrossRef CAS PubMed.
- Y. C. Chang, H. W. Liu, Y. T. Chen, Y. A. Chen, Y. J. Chen and S. J. Chang, Resveratrol protects muscle cells against palmitate-induced cellular senescence and insulin resistance through ameliorating autophagic flux, J. Food Drug Anal., 2018, 26, 1066–1074 CrossRef CAS PubMed.
- Y. J. Zhang, H. Zhao, L. Dong, Y. F. Zhen, H. Y. Xing, H. J. Ma and G. Y. Song, Resveratrol ameliorates high-fat diet-induced insulin resistance and fatty acid oxidation via ATM-AMPK axis in skeletal muscle, Eur. Rev. Med. Pharmacol. Sci., 2019, 23, 9117–9125 Search PubMed.
- F. Faghihzadeh, A. Hekmatdoost and P. Adibi, Resveratrol and liver: A systematic review, J. Res. Med. Sci., 2015, 20, 797–810 CrossRef CAS PubMed.
- R. Guo, W. Li, B. Liu, S. Li, B. Zhang and Y. Xu, Resveratrol protects vascular smooth muscle cells against high glucose-induced oxidative stress and cell proliferation in vitro, Med. Sci. Monit. Basic Res., 2014, 20, 82–92 CrossRef PubMed.
- R. Kosuru and S. Singh, Pterostilbene ameliorates insulin sensitivity, glycemic control and oxidative stress in fructose-fed diabetic rats, Life Sci., 2017, 182, 112–121 CrossRef CAS PubMed.
- W. Yu, L. Fan, M. Wang, B. Cao and X. Hu, Pterostilbene improves insulin resistance caused by advanced glycation end products (AGEs) in hepatocytes and mice, Mol. Nutr. Food Res., 2021, 65, e2100321 CrossRef PubMed.
- S. A. Malik, J. D. Acharya, N. K. Mehendale, S. S. Kamat and S. S. Ghaskadbi, Pterostilbene reverses palmitic acid mediated insulin resistance in HepG2 cells by reducing oxidative stress and triglyceride accumulation, Free Radical Res., 2019, 53, 815–827 CrossRef CAS PubMed.
- G. Sadi, M. B. Pektaş, H. B. Koca, M. Tosun and T. Koca, Resveratrol improves hepatic insulin signaling and reduces the inflammatory response in streptozotocin-induced diabetes, Gene, 2015, 570, 213–220 CrossRef CAS PubMed.
- K. Szkudelska, M. Deniziak, I. Hertig, T. Wojciechowicz, M. Tyczewska, M. Jaroszewska and T. Szkudelski, Effects of resveratrol in Goto-Kakizaki Rat, a model of type 2 diabetes, Nutrients, 2019, 11, 2488 CrossRef CAS PubMed.
- B. Elango, S. Dornadula, R. Paulmurugan and K. M. Ramkumar, Pterostilbene ameliorates streptozotocin-induced diabetes through enhancing antioxidant signaling pathways mediated by Nrf2, Chem. Res. Toxicol., 2016, 29, 47–57 Search PubMed.
- D. Sireesh, M. R. Ganesh, U. Dhamodharan, M. Sakthivadivel, S. Sivasubramanian, P. Gunasekaran and K. M. Ramkumar, Role of pterostilbene in attenuating immune mediated devastation of pancreatic beta cells via Nrf2 signaling cascade, J. Nutr. Biochem., 2017, 44, 11–21 CrossRef CAS PubMed.
- H. Sun, X. Liu, S. R. Long, W. Teng, H. Ge, Y. Wang, S. Yu, Y. Xue, Y. Zhang, X. Li and W. Li, Antidiabetic effects of pterostilbene through PI3K/Akt signal pathway in high fat diet and STZ-induced diabetic rats, Eur. J. Pharmacol., 2019, 859, 172526 CrossRef CAS PubMed.
- J. Zhang, L. Chen, J. Zheng, T. Zeng, H. Li, H. Xiao, X. Deng and X. Hu, The protective effect of resveratrol on islet insulin secretion and morphology in mice on a high-fat diet, Diabetes Res. Clin. Pract., 2012, 97, 474–482 CrossRef CAS PubMed.
- S. Keshtkar, M. Kaviani, Z. Jabbarpour, I. H. Al-Abdullah, M. H. Aghdaei, S. Nikeghbalian, A. Shamsaeefar, B. Geramizadeh, N. Azarpira and M. H. Ghahremani, Significant reduction of apoptosis induced via hypoxia and oxidative stress in isolated human islet by resveratrol, Nutr., Metab. Cardiovasc. Dis., 2020, 30, 1216–1226 CrossRef CAS PubMed.
- S. Y. Park, B. Jin, J. H. Shin, S. Adisakwattana and O. Kwon, Standardized Mori ramulus, extract improves insulin secretion and insulin sensitivity in C57BLKS/J db/db mice
and INS-1 cells, Biomed. Pharmacother., 2017, 92, 308–315 CrossRef CAS PubMed.
- S. Baumel-Alterzon, L. S. Katz, G. Brill, A. Garcia-Ocaña and D. K. Scott, Nrf2: The Master and Captain of Beta Cell Fate, Trends Endocrinol. Metab., 2021, 7–19 CrossRef CAS PubMed.
- M. Valko, D. Leibfritz, J. Moncol, M. T. Cronin, M. Mazur and J. Telser, Free radicals and antioxidants in normal physiological functions and human disease, Int. J. Biochem. Cell Biol., 2007, 39, 44–84 CrossRef CAS PubMed.
- D. Kawamori, H. Kaneto, Y. Nakatani, T. A. Matsuoka, M. Matsuhisa, M. Hori and Y. Yamasaki, The forkhead transcription factor Foxo1 bridges the JNK pathway and the transcription factor PDX-1 through its intracellular translocation, J. Biol. Chem., 2006, 281, 1091–1098 CrossRef CAS PubMed.
- Y. Zhu, Q. Liu, Z. Zhou and Y. Ikeda, PDX1, Neurogenin-3, and MAFA: critical transcription regulators for beta cell development and regeneration, Stem Cell Res. Ther., 2017, 8, 240 CrossRef PubMed.
- J. S. Harmon, R. Stein and R. P. Robertson, Oxidative stress-mediated, post-translational loss of MafA protein as a contributing mechanism to loss of insulin gene expression in glucotoxic beta cells, J. Biol. Chem., 2016, 280, 11107–11113 CrossRef PubMed.
- R. Inoue, T. Tsuno, Y. Togashi, T. Okuyama, A. Sato, K. Nishiyama, M. Kyohara, J. Li, S. Fukushima, T. Kin, D. Miyashita, Y. Shiba, Y. Atobe, H. Kiyonari, K. Bando, A. M. J. Shapiro, K. Funakoshi, R. N. Kulkarni, Y. Terauchi and J. Shirakawa, Uncoupling protein 2 and aldolase B impact insulin release by modulating mitochondrial function and Ca(2+) release from the ER, iScience, 2022, 25, 104603 CrossRef CAS PubMed.
- P. Palsamy and S. Subramanian, Ameliorative potential of resveratrol on proinflammatory cytokines, hyperglycemia mediated oxidative stress, and pancreatic beta-cell dysfunction in streptozotocin-nicotinamide-induced diabetic rats, J. Cell Physiol., 2010, 224, 423–432 CrossRef CAS PubMed.
- P. Palsamy and S. Subramanian, Resveratrol protects diabetic kidney by attenuating hyperglycemia-mediated oxidative stress and renal inflammatory cytokines via Nrf2-Keap1 signaling, Biochim. Biophys. Acta, 2011, 1812, 719–731 CrossRef CAS PubMed.
- H. Moridi, J. Karimi, N. Sheikh, M. T. Goodarzi, M. Saidijam, R. Yadegarazari, M. Khazaei, I. Khodadadi, H. Tavilani, H. Piri, S. Asadi, S. Zarei and A. Rezaei, Resveratrol-dependent down-regulation of receptor for advanced glycation end-products and oxidative stress in kidney of rats with diabetes, Int. J. Endocrinol. Metab., 2015, 13, e23542 Search PubMed.
- M. Khazaei, J. Karimi, N. Sheikh, M. T. Goodarzi, M. Saidijam, I. Khodadadi and H. Moridi, Effects of resveratrol on receptor for advanced glycation end products (RAGE) expression and oxidative stress in the liver of rats with type 2 diabetes, Phytother. Res., 2016, 30, 66–71 CrossRef CAS PubMed.
- B. H. Lee, C. C. Lee, Y. H. Cheng, W. C. Chang, W. H. Hsu and S. C. Wu, Graptopetalum paraguayense and resveratrol ameliorates carboxymethyllysine (CML)-induced pancreas dysfunction and hyperglycemia, Food Chem. Toxicol., 2013, 62, 492–498 CrossRef CAS PubMed.
- L. Vetterli, T. Brun, L. Giovannoni, D. Bosco and P. Maechler, Resveratrol potentiates glucose-stimulated insulin secretion in INS-1E beta-cells and human islets through a SIRT1-dependent mechanism, J. Biol. Chem., 2011, 286, 6049–6060 CrossRef CAS PubMed.
- L. Wu, L. Zhou, Y. Lu, J. Zhang, F. Jian, Y. Liu, F. Li, W. Li, X. Wang and G. Li, Activation of SIRT1 protects pancreatic β-cells against palmitate-induced dysfunction, Biochim. Biophys. Acta, 2012, 1822, 1815–1825 CrossRef CAS PubMed.
- Y. Imai, A. D. Dobrian, M. A. Morris and J. L. Nadler, Islet inflammation: a unifying target for diabetes treatment?, Trends Endocrinol. Metab., 2013, 24, 351–360 CrossRef CAS PubMed.
- M. Cetkovic-Cvrlje and D. L. Eizirik, TNF-alpha and IFN-gamma potentiate the deleterious effects of IL-1 beta on mouse pancreatic islets mainly via generation of nitric oxide, Cytokine, 1994, 6, 399–406 CrossRef CAS PubMed.
- S. Oyadomari, K. Takeda, M. Takiguchi, T. Gotoh, M. Matsumoto, I. Wada, S. Akira, E. Araki and M. Mori, Nitric oxide-induced apoptosis in pancreatic beta cells is mediated by the endoplasmic reticulum stress pathway, Proc. Natl. Acad. Sci. U. S. A., 2001, 98, 10845–10850 CrossRef CAS PubMed.
- M. A. Darwish, A. M. Abo-Youssef, B. A. S. Messiha, A. A. Abo-Saif and M. S. Abdel-Bakky, Resveratrol inhibits macrophage infiltration of pancreatic islets in streptozotocin-induced type 1 diabetic mice via attenuation of the CXCL16/NF-κB p65 signaling pathway, Life Sci., 2021, 272, 119250 CrossRef CAS PubMed.
- H. Cucak, L. G. Grunnet and A. Rosendahl, Accumulation of M1-like macrophages in type 2 diabetic islets is followed by a systemic shift in macrophage polarization, J. Leukocyte Biol., 2014, 95, 149–160 CrossRef PubMed.
- M. Shabani, A. Sadeghi, H. Hosseini, M. Teimouri, R. B. Khorzoughi, P. Pasalar and R. Meshkani, Resveratrol alleviates obesity-induced skeletal muscle inflammation via decreasing M1 macrophage polarization and increasing the regulatory T cell population, Sci. Rep., 2020, 10, 3791 CrossRef CAS PubMed.
- J. A. Ehses, D. T. Meier, S. Wueest, J. Rytka, S. Boller, P. Y. Wielinga, A. Schraenen, K. Lemaire, S. Debray, L. Van Lommel, J. A. Pospisilik, O. Tschopp, S. M. Schultze, U. Malipiero, H. Esterbauer, H. Ellingsgaard, S. Rütti, F. C. Schuit, T. A. Lutz, M. Böni-Schnetzler, D. Konrad and M. Y. Donath, Toll-like receptor 2-deficient mice are protected from insulin resistance and beta cell dysfunction induced by a high-fat diet, Diabetologia, 2010, 53, 1795–1806 CrossRef CAS PubMed.
- G. Wang, Z. Hu, Q. Fu, X. Song, Q. Cui, R. Jia, Y. Zou, C. He, L. Li and Z. Yin, Resveratrol mitigates lipopolysaccharide-mediated acute inflammation in rats by inhibiting the TLR4/NF-κBp65/MAPKs signaling cascade, Sci. Rep., 2017, 7, 45006 CrossRef CAS PubMed.
- K. Szkudelska, M. Okulicz, I. Hertig and T. Szkudelski, Resveratrol ameliorates inflammatory and oxidative stress in type 2 diabetic Goto-Kakizaki rats, Biomed. Pharmacother., 2020, 125, 110026 CrossRef CAS PubMed.
- S. Ding, J. Jiang, Z. Wang, G. Zhang, J. Yin, X. Wang, S. Wang and Z. Yu, Resveratrol reduces the inflammatory response in adipose tissue and improves adipose insulin signaling in high-fat diet-fed mice, PeerJ, 2018, 6, e5173 CrossRef PubMed.
- F. Chen, X. Zhou, Y. Lin, C. Jing, T. Yang, Y. Ji, Y. Sun and X. Han, Resveratrol prevents interleukin-1β-induced dysfunction of pancreatic β-cells, J. Biomed. Res., 2010, 24, 381–388 CrossRef CAS PubMed.
- J. H. Lee, M. Y. Song, E. K. Song, E. K. Kim, W. S. Moon, M. K. Han, J. W. Park, K. B. Kwon and B. H. Park, Overexpression of SIRT1 protects pancreatic beta-cells against cytokine toxicity by suppressing the nuclear factor-kappaB signaling pathway, Diabetes, 2009, 58, 344–351 CrossRef CAS PubMed.
- Y. C. Koh, S. J. Lin, K. Nagabhushanam, C. T. Ho and M. H. Pan, The anti-Obesity and anti-inflammatory capabilities of pterostilbene and its colonic metabolite pinostilbene protect against tight junction disruption from western diet feeding, Mol. Nutr. Food Res., 2022, 66, e2200146 CrossRef PubMed.
- L. Wang, H. Zhao, L. Wang, Y. Tao, G. Du, W. Guan, J. Liu, C. Brennan, C. T. Ho and S. Li, Effects of selected resveratrol analogues on activation and polarization of lipopolysaccharide-stimulated BV-2 microglial cells, J. Agric. Food Chem., 2020, 68, 3750–3757 CrossRef CAS PubMed.
- D. Leto and A. R. Saltiel, Regulation of glucose transport by insulin: traffic control of GLUT4, Nat. Rev. Mol. Cell Biol., 2012, 13, 383–396 CrossRef CAS PubMed.
- F. Han, C. F. Li, Z. Cai, X. Zhang, G. Jin, W. N. Zhang, C. Xu, C. Y. Wang, J. Morrow, S. Zhang, D. Xu, G. Wang and H. K. Lin, The critical role of AMPK in driving Akt activation under stress, tumorigenesis and drug resistance, Nat. Commun., 2018, 9, 4728 CrossRef PubMed.
- P. Puigserver, J. Rhee, J. Donovan, C. J. Walkey, J. C. Yoon, F. Oriente, Y. Kitamura, J. Altomonte, H. Dong, D. Accili and B. M. Spiegelman, Insulin-regulated hepatic gluconeogenesis through FOXO1-PGC-1alpha interaction, Nature, 2003, 423, 550–555 CrossRef CAS PubMed.
- D. J. Den Hartogh, F. Vlavcheski, A. Giacca and E. Tsiani, Attenuation of free fatty acid (FFA)-induced skeletal muscle cell insulin resistance by resveratrol is linked to activation of AMPK and inhibition of mTOR and p70 S6K, Int. J. Mol. Sci., 2020, 21, 4900 CrossRef CAS PubMed.
- G. M. Do, U. J. Jung, H. J. Park, E. Y. Kwon, S. M. Jeon, R. A. McGregor and M. S. Choi, Resveratrol ameliorates diabetes-related metabolic changes via activation of AMP-activated protein kinase and its downstream targets in db/db mice, Mol. Nutr. Food Res., 2012, 56, 1282–1291 CrossRef CAS PubMed.
- S. Chen, Z. Zhao, L. Ke, Z. Li, W. Li, Z. Zhang, Y. Zhou, X. Feng and W. Zhu, Resveratrol improves glucose uptake in insulin-resistant adipocytes via Sirt1, J. Nutr. Biochem., 2018, 55, 209–218 CrossRef CAS PubMed.
- F. Vlavcheski, D. J. Den Hartogh, A. Giacca and E. Tsiani, Amelioration of high-insulin-induced skeletal muscle cell insulin resistance by resveratrol Is linked to activation of AMPK and restoration of GLUT4 translocation, Nutrients, 2020, 12, 914 CrossRef CAS PubMed.
- B. Thorens, GLUT2, glucose sensing and glucose homeostasis, Diabetologia, 2015, 58, 221–232 CrossRef CAS PubMed.
- C. Y. Yonamine, E. Pinheiro-Machado, M. L. Michalani, H. S. Freitas, M. M. Okamoto, M. L. Corrêa-Giannella and U. F. Machado, Resveratrol improves glycemic control in insulin-treated diabetic rats: participation of the hepatic territory, Nutr. Metab., 2016, 13, 44 CrossRef PubMed.
- Q. Wang, X. Sun, X. Li, X. Dong, P. Li and L. Zhao, Resveratrol attenuates intermittent hypoxia-induced insulin resistance in rats: involvement of Sirtuin 1 and the phosphatidylinositol-4,5-bisphosphate 3-kinase/AKT pathway, Mol. Med. Rep., 2015, 11, 151–158 CrossRef CAS PubMed.
- C. Y. Yonamine, E. Pinheiro-Machado, M. L. Michalani, A. B. Alves-Wagner, J. V. Esteves, H. S. Freitas and U. F. Machado, Resveratrol improves glycemic control in Type 2 diabetic obese mice by regulating glucose transporter expression in skeletal muscle and liver, Molecules, 2017, 22, 1180 CrossRef PubMed.
- S. Gómez-Zorita, A. Fernández-Quintela, L. Aguirre, M. T. Macarulla, A. M. Rimando and M. P. Portillo, Pterostilbene improves glycaemic control in rats fed an obesogenic diet: involvement of skeletal muscle and liver, Food Funct., 2015, 6, 1968–1976 RSC.
- M. J. Moreno-Aliaga, N. Pérez-Echarri, B. Marcos-Gómez, E. Larequi, F. J. Gil-Bea, B. Viollet, I. Gimenez, J. A. Martínez, J. Prieto and M. Bustos, Cardiotrophin-1 is a key regulator of glucose and lipid metabolism, Cell Metab., 2011, 14, 242–253 CrossRef CAS PubMed.
- S. O. Jeong, Y. Son, J. H. Lee, Y. K. Cheong, S. H. Park, H. T. Chung and H. O. Pae, Resveratrol analog piceatannol restores the palmitic acid-induced impairment of insulin signaling and production of endothelial nitric oxide via activation of anti-inflammatory and antioxidative heme oxygenase-1 in human endothelial cells, Mol. Med. Rep., 2015, 12, 937–944 CrossRef CAS PubMed.
- Y. Li, P. Yang, Q. Chang, J. Wang, J. Liu, Y. Lv, T. T. Y. Wang, B. Gao, Y. Zhang and L. L. Yu, Inhibitory effect of piceatannol on TNF-α-mediated inflammation and insulin resistance in 3T3-L1 adipocytes, J. Agric. Food Chem., 2017, 65, 4634–4641 CrossRef CAS PubMed.
- M. Minakawa, Y. Miura and K. Yagasaki, Piceatannol, a resveratrol derivative, promotes glucose uptake through glucose transporter 4 translocation to plasma membrane in L6 myocytes and suppresses blood glucose levels in type 2 diabetic model db/db mice, Biochem. Biophys. Res. Commun., 2012, 422, 469–475 CrossRef CAS PubMed.
- H. J. Lee, M. G. Kang, H. Y. Cha, Y. M. Kim, Y. Lim and S. J. Yang, Effects of piceatannol and resveratrol on sirtuins and hepatic inflammation in high-fat diet-fed mice, J. Med. Food, 2019, 22, 833–840 CrossRef CAS PubMed.
- H. Y. Tan, I. M. Tse, E. T. Li and M. Wang, Oxyresveratrol supplementation to C57bl/6 mice fed with a high-fat diet ameliorates obesity-associated symptoms, Nutrients, 2017, 9, 147 CrossRef PubMed.
- W. P. Tang, J. F. Zhan, S. M. Li, Y. Liu and C. T. Ho, Hypoglycemic effects of naturally processed Polygonum multiflorum extract in KK CgAy/J mice and its mechanism of action, Food Sci. Hum. Wellness, 2022, 11, 1177–1182 CrossRef CAS.
- L. Pari and M. A. Satheesh, Effect of pterostilbene on hepatic key enzymes of glucose metabolism in streptozotocin- and nicotinamide-induced diabetic rats, Life Sci., 2006, 79, 641–645 CrossRef CAS PubMed.
- G. Ren, A. M. Rimando and S. T. Mathews, AMPK activation by pterostilbene contributes to suppression of hepatic gluconeogenic gene expression and glucose production in H4IIE cells, Biochem. Biophys. Res. Commun., 2018, 498, 640–645 CrossRef CAS PubMed.
- W. Tang, S. Li, Y. Liu, J. C. Wu, M. H. Pan, M. T. Huang and C. T. Ho, Anti-diabetic activities of cis- and trans-2,3,5,4′-tetrahydroxystilbene 2-O-β-glucopyranoside from Polygonum multiflorum, Mol. Nutr. Food Res., 2017, 61, 1600871 CrossRef PubMed.
- Y. C. Zheng, H. He, X. Wei, S. Ge and Y. H. Lu, Comparison of regulation mechanisms of five mulberry ingredients on insulin secretion under oxidative stress, J. Agric. Food Chem., 2016, 64, 8763–8772 CrossRef CAS PubMed.
- T. Walle, Bioavailability of resveratrol, Ann. N. Y. Acad. Sci., 2011, 1215, 9–15 CrossRef CAS PubMed.
- O. Vaarala, M. A. Atkinson and J. Neu, The “perfect storm” for type 1 diabetes: the complex interplay between intestinal microbiota, gut permeability, and mucosal immunity, Diabetes, 2008, 57, 2555–2562 CrossRef CAS PubMed.
- M. Gurung, Z. Li, H. You, R. Rodrigues, D. B. Jump, A. Morgun and N. Shulzhenko, Role of gut microbiota in type 2 diabetes pathophysiology, EBioMedicine, 2020, 51, 102590 CrossRef PubMed.
- M. J. Saad, A. Santos and P. O. Prada, Linking Gut Microbiota and Inflammation to Obesity and Insulin Resistance, Physiology, 2016, 31, 283–293 CrossRef CAS PubMed.
- L. Zhou, X. D. Song, H. Xu, G. Q. Liang, F. Wang, L. R. Zhang, F. Huang, J. Cai and G. R. Jiang, Exogenous 3-deoxyglucosone-induced carbonyl and oxidative stress causes β-cells dysfunction by impairing gut permeability in rats, Biochemistry, 2018, 83, 1358–1368 CAS.
- K. Vehik and D. Dabelea, The changing epidemiology of type 1 diabetes:
why is it going through the roof?, Diabetes/Metab. Res. Rev., 2011, 27, 3–13 CrossRef PubMed.
- C. Sorini, I. Cosorich, M. Lo Conte, L. De Giorgi, F. Facciotti, R. Lucianò, M. Rocchi, R. Ferrarese, F. Sanvito, F. Canducci and M. Falcone, Loss of gut barrier integrity triggers activation of islet-reactive T cells and autoimmune diabetes, Proc. Natl. Acad. Sci. U. S. A., 2019, 116, 15140–15149 CrossRef CAS PubMed.
- K. Chen, H. Zhao, L. Shu, H. Xing, C. Wang, C. Lu and G. Song, Effect of resveratrol on intestinal tight junction proteins and the gut microbiome in high-fat diet-fed insulin resistant mice, Int. J. Food Sci. Nutr., 2020, 71, 965–978 CrossRef CAS PubMed.
- Y. Mayangsari and T. Suzuki, Resveratrol ameliorates intestinal barrier defects and inflammation in colitic mice and intestinal cells, J. Agric. Food Chem., 2018, 66, 12666–12674 CrossRef CAS PubMed.
- N. Wang, Q. Han, G. Wang, W. P. Ma, J. Wang, W. X. Wu, Y. Guo, L. Liu, X. Y. Jiang, X. L. Xie and H. Q. Jiang, Resveratrol protects oxidative stress-induced intestinal epithelial barrier dysfunction by upregulating heme oxygenase-1 expression, Dig. Dis Sci., 2016, 61, 2522–2534 CrossRef CAS PubMed.
- Y. Zhuang, H. Wu, X. Wang, J. He, S. He and Y. Yin, Resveratrol attenuates oxidative stress-induced intestinal barrier injury through PI3K/Akt-mediated Nrf2 signaling pathway, Oxid. Med. Cell. Longevity, 2019, 2019, 7591840 Search PubMed.
- H. Zhang, Y. Chen, Y. Chen, Y. Li, P. Jia, S. Ji, Y. Zhou and T. Wang, Dietary pterostilbene supplementation attenuates intestinal damage and immunological stress of broiler chickens challenged with lipopolysaccharide, J. Anim. Sci., 2020, 98, skz373 CrossRef PubMed.
- G. Serreli, M. P. Melis, S. Zodio, M. R. Naitza, E. Casula, P. Peñalver, R. Lucas, R. Loi, J. C. Morales and M. Deiana, Altered paracellular permeability in intestinal cell monolayer challenged with lipopolysaccharide: Modulatory effects of pterostilbene metabolites, Food Chem. Toxicol., 2020, 145, 111729 CrossRef CAS PubMed.
- D. Hwang, H. Jo, S. H. Ma and Y. H. Lim, Oxyresveratrol stimulates mucin production in an NAD(+)-dependent manner in human intestinal goblet cells, Food Chem. Toxicol., 2018, 118, 880–888 CrossRef CAS PubMed.
- H. Jo, D. Hwang, J. K. Kim and Y. H. Lim, Oxyresveratrol improves tight junction integrity through the PKC and MAPK signaling pathways in Caco-2 cells, Food Chem. Toxicol., 2017, 108, 203–213 CrossRef CAS PubMed.
- D. Hwang, H. Jo, S. Hwang, J. K. Kim, I. H. Kim and Y. H. Lim, Conditioned medium from LS 174T goblet cells treated with oxyresveratrol strengthens tight junctions in Caco-2 cells, Biomed. Pharmacother., 2017, 85, 280–286 CrossRef CAS PubMed.
- M. Knip and H. Siljander, The role of the intestinal microbiota in type 1 diabetes mellitus, Nat. Rev. Endocrinol., 2016, 12, 154–167 CrossRef CAS PubMed.
- F. Bäckhed, H. Ding, T. Wang, L. V. Hooper, G. Y. Koh, A. Nagy, C. F. Semenkovich and J. I. Gordon, The gut microbiota as an environmental factor that regulates fat storage, Proc. Natl. Acad. Sci. U. S. A., 2004, 101, 15718–15723 CrossRef PubMed.
- C. L. Campbell, R. Yu, F. Li, Q. Zhou, D. Chen, C. Qi, Y. Yin and J. Sun, Modulation of fat metabolism and gut microbiota by resveratrol on high-fat diet-induced obese mice, Diabetes Metab. Syndr. Obes., 2019, 12, 97–107 CrossRef CAS PubMed.
- T. T. Cai, X. L. Ye, R. R. Li, H. Chen, Y. Y. Wang, H. J. Yong, M. L. Pan, W. Lu, Y. Tang, H. Miao, A. M. Snijders, J. H. Mao, X. Y. Liu, Y. B. Lu and D. F. Ding, Resveratrol modulates the gut microbiota and inflammation to protect against diabetic nephropathy in mice, Front. Pharmacol., 2020, 11, 1249 CrossRef CAS PubMed.
- P. Wang, D. Li, W. Ke, D. Liang, X. Hu and F. Chen, Resveratrol-induced gut microbiota reduces obesity in high-fat diet-fed mice, Int. J. Obes., 2020, 44, 213–225 CrossRef PubMed.
- E. E. Canfora, J. W. Jocken and E. E. Blaak, Short-chain fatty acids in control of body weight and insulin sensitivity, Nat. Rev. Endocrinol., 2015, 11, 577–591 CrossRef CAS PubMed.
- M. M. Sung, T. T. Kim, E. Denou, C. M. Soltys, S. M. Hamza, N. J. Byrne, G. Masson, H. Park, D. S. Wishart, K. L. Madsen, J. D. Schertzer and J. R. Dyck, Improved glucose homeostasis in obese mice treated with resveratrol is associated with alterations in the gut microbiome, Diabetes, 2017, 66, 418–425 CrossRef CAS PubMed.
- P. Wang, J. Gao, W. Ke, J. Wang, D. Li, R. Liu, Y. Jia, X. Wang, X. Chen, F. Chen and X. Hu, Resveratrol reduces obesity in high-fat diet-fed mice via modulating the composition and metabolic function of the gut microbiota, Free Radicals Biol. Med., 2020, 156, 83–98 CrossRef CAS PubMed.
- M. Vacca, G. Celano, F. M. Calabrese, P. Portincasa, M. Gobbetti and M. De Angelis, The Controversial Role of Human Gut Lachnospiraceae, Microorganisms, 2020, 8, 573 CrossRef CAS PubMed.
- C. V. de Almeida, A. Taddei and A. Amedei, The controversial role of Enterococcus faecalis, in colorectal cancer, Ther. Adv. Gastroenterol., 2018, 11, 1756284818783606 Search PubMed.
- N. R. Shin, T. W. Whon and J. W. Bae, Proteobacteria: microbial signature of dysbiosis in gut microbiota, Trends Biotechnol., 2015, 33, 496–503 CrossRef CAS PubMed.
- U. Etxeberria, E. Hijona, L. Aguirre, F. I. Milagro, L. Bujanda, A. M. Rimando, J. A. Martínez and M. P. Portillo, Pterostilbene-induced changes in gut microbiota composition in relation to obesity, Mol. Nutr. Food Res., 2017, 61, 1500906 CrossRef PubMed.
- Q. Bi, W. Gu, F. Meng, X. Yang, L. Zeng, L. Liang, M. Yang, T. Zhang and J. Yu, Pharmacological and metagenomics evidence of polysaccharide from Polygonum multiflorum in the alleviation of insulin resistance, Int. J. Biol. Macromol., 2020, 164, 1070–1079 CrossRef CAS PubMed.
- S. Vaishnava, M. Yamamoto, K. M. Severson, K. A. Ruhn, X. Yu, O. Koren, R. Ley, E. K. Wakeland and L. V. Hooper, The antibacterial lectin RegIIIgamma promotes the spatial segregation of microbiota and host in the intestine, Science, 2011, 334, 255–258 CrossRef CAS PubMed.
- S. A. Bae, M. Z. Fang, V. Rustgi, H. Zarbl and I. P. Androulakis, At the Interface of Lifestyle, Behavior, and Circadian Rhythms: Metabolic Implications, Front. Nutr., 2019, 6, 132 CrossRef PubMed.
- D. J. Stenvers, F. Scheer, P. Schrauwen, S. E. La Fleur and A. Kalsbeek, Circadian clocks and insulin resistance, Nat. Rev. Endocrinol., 2019, 15, 75–89 CrossRef PubMed.
- I. C. Mason, J. Qian, G. K. Adler and F. Scheer, Impact of circadian disruption on glucose metabolism: implications for type 2 diabetes, Diabetologia, 2020, 63, 462–472 CrossRef CAS PubMed.
- Y. Gan, C. Yang, X. Tong, H. Sun, Y. Cong, X. Yin, L. Li, S. Cao, X. Dong, Y. Gong, O. Shi, J. Deng, H. Bi and Z. Lu, Shift work and diabetes mellitus: a meta-analysis of observational studies, Occup. Environ. Med., 2015, 72, 72–78 CrossRef PubMed.
- C. Vetter, H. S. Dashti, J. M. Lane, S. G. Anderson, E. S. Schernhammer, M. K. Rutter, R. Saxena and F. Scheer, Night shift work, genetic risk, and type 2 diabetes in the UK Biobank, Diabetes Care, 2018, 41, 762–769 CrossRef PubMed.
- J. Jiang, Y. Gu, S. Ding, G. Zhang and J. Ding, Resveratrol reversed ambient particulate matter exposure-perturbed oscillations of hepatic glucose metabolism by regulating SIRT1 in mice, Environ. Sci. Pollut. Res. Int., 2023, 30, 31821–31834 CrossRef CAS PubMed.
- B. M. Gabriel, A. Altıntaş, J. A. B. Smith, L. Sardon-Puig, X. Zhang, A. L. Basse, R. C. Laker, H. Gao, Z. Liu, L. Dollet, J. T. Treebak, A. Zorzano, Z. Huo, M. Rydén, J. T. Lanner, K. A. Esser, R. Barrès, N. J. Pillon, A. Krook and J. R. Zierath, Disrupted circadian oscillations in type 2 diabetes are linked to altered rhythmic mitochondrial metabolism in skeletal muscle, Sci. Adv., 2021, 7, eabi9654 CrossRef CAS PubMed.
- J. Li, L. Wei, C. Zhao, J. Li, Z. Liu, M. Zhang and Y. Wang, Resveratrol maintains lipid metabolism homeostasis via one of the mechanisms associated with the key circadian regulator Bmal1, Molecules, 2019, 24, 2916 CrossRef CAS PubMed.
- J. Liu, B. Zhou, M. Yan, R. Huang, Y. Wang, Z. He, Y. Yang, C. Dai, Y. Wang, F. Zhang and Q. Zhai, CLOCK and BMAL1 regulate muscle insulin sensitivity via SIRT1 in male mice, Endocrinology, 2016, 157, 2259–2269 CrossRef CAS PubMed.
- B. Zhou, Y. Zhang, F. Zhang, Y. Xia, J. Liu, R. Huang, Y. Wang, Y. Hu, J. Wu, C. Dai, H. Wang, Y. Tu, X. Peng, Y. Wang and Q. Zhai, CLOCK/BMAL1 regulates circadian change of mouse hepatic insulin sensitivity by SIRT1, Hepatology, 2014, 59, 2196–2206 CrossRef CAS PubMed.
- L. Sun, Y. Wang, Y. Song, X. R. Cheng, S. Xia, M. R. Rahman, Y. Shi and G. Le, Resveratrol restores the circadian rhythmic disorder of lipid metabolism induced by high-fat diet in mice, Biochem. Biophys. Res. Commun., 2015, 458, 86–91 CrossRef CAS PubMed.
- Y. Liu, H. Lang, M. Zhou, L. Huang, S. Hui, X. Wang, K. Chen and M. Mi, The preventive effects of pterostilbene on the exercise intolerance and circadian misalignment of mice subjected to sleep restriction, Mol. Nutr. Food Res., 2020, 64, e1900991 CrossRef PubMed.
- Y. C. Koh, P. S. Lee, Y. L. Kuo, K. Nagabhushanam, C. T. Ho and M. H. Pan, Dietary pterostilbene and resveratrol modulate the gut microbiota influenced by circadian rhythm dysregulation, Mol. Nutr. Food Res., 2021, 65, e2100434 CrossRef PubMed.
- T. Yamamoto, S. Iwami, S. Aoyama, H. Maruki-Uchida, S. Mori, R. Hirooka, K. Takahashi, M. Morita and S. Shibata, Effect of piceatannol on circadian Per2 expression in vitro and in vivo, J. Funct. Foods, 2019, 56, 49–56 CrossRef CAS.
- X. Zhu, C. Wu, S. Qiu, X. Yuan and L. Li, Effects of resveratrol on glucose control and insulin sensitivity
in subjects with type 2 diabetes: systematic review and meta-analysis, Nutr. Metab., 2017, 14, 60 CrossRef PubMed.
- S. Bo, V. Ponzo, G. Ciccone, A. Evangelista, F. Saba, I. Goitre, M. Procopio, G. F. Pagano, M. Cassader and R. Gambino, Six months of resveratrol supplementation has no measurable effect in type 2 diabetic patients. A randomized, double blind, placebo-controlled trial, Pharmacol. Res., 2016, 111, 896–905 CrossRef CAS PubMed.
- B. I. García-Martínez, M. Ruiz-Ramos, J. Pedraza-Chaverri, E. Santiago-Osorio and V. M. Mendoza-Núñez, Effect of resveratrol on markers of oxidative stress and Sirtuin 1 in elderly adults with type 2 diabetes, Int. J. Mol. Sci., 2023, 24, 7422 CrossRef PubMed.
- W. Mahjabeen, D. A. Khan and S. A. Mirza, Role of resveratrol supplementation in regulation of glucose hemostasis, inflammation and oxidative stress in patients with diabetes mellitus type 2: A randomized, placebo-controlled trial, Complement. Ther. Med., 2022, 66, 102819 CrossRef PubMed.
- A. Movahed, P. Raj, I. Nabipour, M. Mahmoodi, A. Ostovar, M. Kalantarhormozi and T. Netticadan, Efficacy and safety of resveratrol in type 1 diabetes patients: A two-month preliminary exploratory trial, Nutrients, 2020, 12, 161 CrossRef CAS PubMed.
- A. Sattarinezhad, J. Roozbeh, B. Shirazi Yeganeh, G. R. Omrani and M. Shams, Resveratrol reduces albuminuria in diabetic nephropathy: A randomized double-blind placebo-controlled clinical trial, Diabetes Metab., 2019, 45, 53–59 CrossRef CAS PubMed.
- N. Ma and Y. Zhang, Effects of resveratrol therapy on glucose metabolism, insulin resistance, inflammation, and renal function in the elderly patients with type 2 diabetes mellitus: A randomized controlled clinical trial protocol, Medicine, 2022, 101, e30049 CrossRef CAS PubMed.
- A. Hoseini, G. Namazi, A. Farrokhian, Ž. Reiner, E. Aghadavod, F. Bahmani and Z. Asemi, The effects of resveratrol on metabolic status in patients with type 2 diabetes mellitus and coronary heart disease, Food Funct., 2019, 10, 6042–6051 RSC.
- D. M. Riche, C. L. McEwen, K. D. Riche, J. J. Sherman, M. R. Wofford, D. Deschamp and M. Griswold, Analysis of safety from a human clinical trial with pterostilbene, J. Toxicol., 2013, 2013, 463595 Search PubMed.
- D. M. Riche, K. D. Riche, C. T. Blackshear, C. L. McEwen, J. J. Sherman, M. R. Wofford and M. E. Griswold, Pterostilbene on metabolic parameters: a randomized, double-blind, and placebo-controlled trial, J. Evidence-Based Complementary Altern. Med., 2014, 2014, 459165 Search PubMed.
- S. Nakamura, M. Hashiguchi and Y. Yamaguchi, Hypoglycemic effects of Morus alba leaf extract on postprandial glucose and insulin levels in patients with type 2 diabetes treated with sulfonylurea hypoglycemic agents, J. Diabetes Metab., 2011, 02, 1045309 Search PubMed.
- M. Mudra, N. Ercan-Fang, L. Zhong, J. Furne and M. Levitt, Influence of mulberry leaf extract on the blood glucose and breath hydrogen response to ingestion of 75 g sucrose by type 2 diabetic and control subjects, Diabetes Care, 2007, 30, 1272–1274 CrossRef PubMed.
- G. S. De. Bona, N. Bertazzon, E. Angelini and S. Vincenzi, Influence of pruning time and viral infection on stilbenoid levels in Pinot noir, grape canes, J. Sci. Food Agric., 2020, 100, 1741–1747 CrossRef CAS PubMed.
- E. Cione, C. La Torre, R. Cannataro, M. C. Caroleo, P. Plastina and L. Gallelli, Quercetin, epigallocatechin gallate, curcumin, and resveratrol: from dietary sources to human MicroRNA modulation, Molecules, 2019, 25, 63 CrossRef PubMed.
- W. Song, H. J. Wang, P. Bucheli, P. F. Zhang, D. Z. Wei and Y. H. Lu, Phytochemical profiles of different mulberry (Morus sp.) species from China, J. Agric. Food Chem., 2009, 57, 9133–9140 CrossRef CAS PubMed.
- Q. Jin, J. Yang, L. Ma, J. Cai and J. Li, Comparison of polyphenol profile and inhibitory activities against oxidation and α-glucosidase
in mulberry (Genus Morus) cultivars from China, J. Food Sci., 2015, 80, C2440–C2451 CAS.
- J. Zhou, S. X. Li, W. Wang, X. Y. Guo, X. Y. Lu, X. P. Yan, D. Huang, B. Y. Wei and L. Cao, Variations in the levels of mulberroside A, oxyresveratrol, and resveratrol in mulberries in different seasons and during growth, Sci. World J., 2013, 2013, 380692 Search PubMed.
- Y. Ma, A. Kosińska-Cagnazzo, W. L. Kerr, R. Amarowicz, R. B. Swanson and R. B. Pegg, Separation and characterization of phenolic compounds from dry-blanched peanut skins by liquid chromatography-electrospray ionization mass spectrometry, J. Chromatogr. A, 2014, 1356, 64–81 CrossRef CAS PubMed.
- K. L. Ku, P. S. Chang, Y. C. Cheng and C. Y. Lien, Production of stilbenoids from the callus of Arachis hypogaea: a novel source of the anticancer compound piceatannol, J. Agric. Food Chem., 2005, 53, 3877–3881 CrossRef CAS PubMed.
- V. S. Sobolev, S. I. Khan, N. Tabanca, D. E. Wedge, S. P. Manly, S. J. Cutler, M. R. Coy, J. J. Becnel, S. A. Neff and J. B. Gloer, Biological activity of peanut (Arachis hypogaea) phytoalexins and selected natural and synthetic stilbenoids, J. Agric. Food Chem., 2011, 59, 1673–1682 CrossRef CAS PubMed.
- A. M. Rimando, W. Kalt, J. B. Magee, J. Dewey and J. R. Ballington, Resveratrol, pterostilbene, and piceatannol in vaccinium berries, J. Agric. Food Chem., 2004, 52, 4713–4719 CrossRef CAS PubMed.
- L. Zhang, K. L. Tan, H. M. Liao and Z. H. Hu, Content changes of stilbene glucoside in Polygonum multiflorum Thun., Acta Bot. Sin., 2010, 30, 1481–1484 CAS.
- S. M. Boue, B. Y. Shih, M. E. Burow, G. Eggleston, S. Lingle, Y. B. Pan, K. Daigle and D. Bhatnagar, Postharvest accumulation of resveratrol and piceatannol in sugarcane with enhanced antioxidant activity, J. Agric. Food Chem., 2013, 61, 8412–8419 CrossRef CAS PubMed.
- J. Li, Z. Lin, X. Tang, G. Liu, Y. Chen, X. Zhai, Q. Huang and Y. Cao, Oxyresveratrol extracted from Artocarpus heterophyllus Lam. inhibits tyrosinase and age pigments in vitro and in vivo, Food Funct., 2020, 11, 6595–6607 RSC.
- Y. C. Tung, Y. H. Lin, H. J. Chen, S. C. Chou, A. C. Cheng, N. Kalyanam, C. T. Ho and M. H. Pan, Piceatannol Exerts Anti-Obesity Effects in C57BL/6 Mice through Modulating Adipogenic Proteins and Gut Microbiota, Molecules, 2016, 21, 1419 CrossRef PubMed.
- H. Uchida-Maruki, H. Inagaki, R. Ito, I. Kurita, M. Sai and T. Ito, Piceatannol lowers the blood glucose level in diabetic mice, Biol. Pharm. Bull., 2015, 38, 629–633 CrossRef CAS PubMed.
- E. Ahn, J. Lee, Y. H. Jeon, S. W. Choi and E. Kim, Anti-diabetic effects of mulberry (Morus alba L.) branches and oxyresveratrol in streptozotocin-induced diabetic mice, Food Sci. Biotechnol., 2017, 26, 1693–1702 CrossRef CAS PubMed.
|
This journal is © The Royal Society of Chemistry 2024 |
Click here to see how this site uses Cookies. View our privacy policy here.