DOI:
10.1039/D3IM00091E
(Perspective)
Ind. Chem. Mater., 2024,
2, 270-275
Designing zeolites for the removal of aqueous PFAS: a perspective
Received
19th August 2023
, Accepted 16th October 2023
First published on 20th October 2023
Abstract
Zeolites possess unique sieving properties that offer a high selectivity for removing pollutants, such as per- and polyfluoroalkyl substances (PFAS). However, there are limited studies examining the efficacy of zeolites as PFAS sorbents. Previous literature explores the effects of certain frameworks and the silica alumina ratio (SAR), and only one study has shown the effect of silanol defects on the hydrophobicity of the adsorbent. Since most zeolites are synthesized in hydroxide media, this leads to formation of silanol defects, which increase hydrophilicity with a greater effect than the inclusion of non-Si T atoms. It is critical that specific characterizations be performed to demonstrate the specific effects of different properties of the zeolites. In particular, synthesis, modification, and/or repair in fluoride media can be used to increase the hydrophobicity of zeolites by reducing silanol defects, and increasing Lewis acidity.
Keywords: Zeolites; Aqueous adsorption; PFAS; Hydrophobic interaction; Silica–alumina ratio.
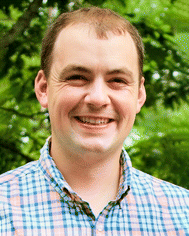 Charles Ponge | Charles Ponge earned his B.S. and M.S. degrees in chemical engineering (minor in engineering entrepreneurship) from Villanova University in 2016 and 2021, respectively. He ran the Thermodynamics Laboratory at the Chemours Company (2017–2021) measuring vapor–liquid equilibria and thermophysical properties of fluorocarbon mixtures. He is currently pursuing his PhD at the University of Kansas as a member of the Shiflett research group. His research is focused on the adsorption of per- and polyfluoroalkyl substances (PFAS) using zeolites. |
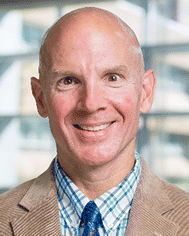 Mark B. Shiflett | Mark B. Shiflett is a Distinguished Foundation Professor in the Department of Chemical and Petroleum Engineering and Director of the Institute for Sustainable Engineering at the University of Kansas (KU). Prof. Shiflett joined KU in August 2016 after retiring from the DuPont Company with 28 years of service. Prof. Shiflett received his PhD and M.S. degrees in chemical engineering (ChE) from the University of Delaware and B.S. degree in ChE from N.C. State University. Prof. Shiflett is an inventor on 45 U.S. patents and has published 125 articles. To learn more about Prof. Shiflett's research, visit his group's website: https://shiflettresearch.com. |
1 Introduction
Research into the sorption of per- and polyfluoroalkyl substances (PFAS) has increased significantly over the last decade as the health risks and extent of exposure have grown more evident with improved and more sensitive analytical techniques.1 Furthermore, the continued production and release of PFAS remain a concern with both environmental and human safety advocates.2 Due to the unique properties of PFAS, the elucidation of the sorption mechanisms is often a research focus, with the hydrophobic and electrostatic interactions being the mechanisms most often cited as responsible for PFAS sorption.3 The fluoroalkyl backbone is primarily responsible for the hydrophobic interaction, while the functional group dictates electrostatic interaction. The most studied systems for PFAS sorption include soils, clays, and metal oxides4—which provide insight into sorption mechanisms for long- and short-carbon chain PFAS. Namely, long-chain PFAS are more hydrophobic and typically have stronger sorption, compared to short-chain PFAS. Fig. 1 demonstrates an idealized silica surface adsorbing PFAS molecules under two conditions. While these studies are invaluable for clarifying environmental accumulation of PFAS, as the total sorption and capacity are not very high and the affinity for sorbing PFAS—especially short chain—is fairly low, these natural materials are not suitable for long term remediation of PFAS. Furthermore, the range of performance within these systems is inconsistent due to high variability.
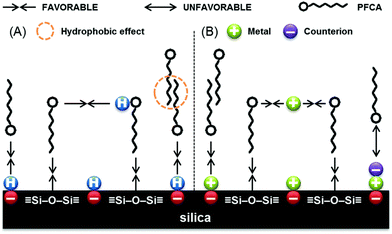 |
| Fig. 1 Possibly enhanced perfluorocarboxylic acid (PFCA) aggregation through solute–silica and solute–solute H-bonding at low pH (A), and anion shielding by background electrolytes (B) reprinted from ref. 5. Copyright 2017 RSC. | |
While activated carbon remains a popular option for adsorbing PFAS and other pollutants,5 it often lacks the specificity for these compounds, and is not easily regenerable and when landfilled can compound the problem. Ion exchange resins are also a popular option,6 but suffer from decreased efficiency in aqueous systems with competing anions, and regenerability is difficult. Adjacent to zeolites, mesoporous silicas have shown affinity to adsorb PFAS analytes, but the high silanol content typically needs to be reduced to increase hydrophobicity.10 Additionally, mesopores don't provide the same sieving effects as micropores, so silicas are more sensitive to competition. Table 1 shows some selected capacity values for typical PFOA adsorbents. Amine groups added by silanes show strong targeted adsorption of PFAS compounds, but extraction typically requires acidic solvents that reduce and destroy the surface, making regeneration difficult.8,9,11 Although, recently Min et al. showed that grafting silane groups onto mesoporous silica that combine electrostatic interaction provided by amine groups, and hydrophobic interaction provided by fluoroalkyl groups can adsorb high amounts of short-chain PFAS, while being indefinitely regenerated.12 Zeolites, on the other hand, may be a more optimal solution to targeting PFAS analytes, and have a high thermal resistance that is optimal for regeneration. Zeolites are crystalline aluminosilicates, and are typically utilized in gas-phase applications, but can also operate successfully in aqueous systems. Compared to activated carbon and mesoporous silicas, zeolites have the benefit of more targeted removal of specific compounds through sieving effects. The external surface of zeolites being rich in silanol groups and can be functionalized by silane grafting while the internal surface is left for adsorption.
Table 1 Zeolite and other selected adsorbents PFOA adsorption capacity
Adsorbent |
Adsorption capacity (mg g−1) |
Ref. |
Activated carbon |
2.69–426.49 |
3
|
Zeolite |
16.8–371.4 |
7
|
Mesoporous silica |
15.1–868 |
8 and 9 |
Anion-exchange resin |
331.25–1436.82 |
3
|
2 PFAS adsorption using zeolites
2.1 Properties of zeolites leading hydrophobicity
Previous literature13,14 attributes increased PFAS–zeolite sorption to the hydrophobicity of high silica–alumina ratios (SAR). Although this ratio and the hydrophobicity are correlated, there are other zeolite characteristics to be considered when trying to understand the sorption mechanism. Specifically, the presence of silanol groups can have a much more detrimental effect on the hydrophobicity than a decrease in the SAR.15 While SAR is often seen as one of the most important factors in controlling catalytic activity and hydrophobicity of the zeolite, the synthesis method must also be considered. Notably, the medium in which the zeolite is produced has a large effect on the crystallinity and silanol defect content, which oftentimes far outweighs the SAR in determining the properties of the zeolite. Zeolites produced in fluoride (F−) media have far fewer silanol defects, compared to those made in hydroxide (OH−) media.16 This, in turn, leads to higher hydrophobicity in the fluoride media zeolite, even if it was created with the same SAR as the hydroxide-made zeolite. Aside from the safety concerns from synthesizing in fluoride media such as hydrogen fluoride (HF), synthesizing in (F−) media typically leads to larger crystallite size,17 which can decrease diffusion by lowering external surface area. Crystallite size can be decreased by milling, which can lead to defects and silanol formation that can be repaired with post-synthesis treatment.18 Clark et al. proposed a modification to this procedure to make it more applicable to low silica zeolites.19
2.2 Super hydrophobic zeolites' enhanced sorption of PFOA
A zeolite adsorbent that stands out as unique is an all silica beta zeolite synthesized in fluoride media.21 Van den Bergh compared three different beta zeolites, two synthesized in hydroxide media and one synthesized in fluoride media, providing evidence that increased SAR does not necessarily result in higher perfluorooctanoic acid (PFOA) and perfluorooctane sulfonic acid (PFOS) sorption. The beta-22 and beta-300 that were tested showed nearly identical sorption behavior and the synthesis method seemed to have a much larger effect on the hydrophobicity. The author provides evidence that in the case of the all silica beta synthesized in fluoride media, PFOA molecules enter the zeolite pores as protonated dimers, which is unexpected as the pKa of PFOA is −0.2.26 The possibility of fluorinated carboxylic acid dimers forming in aqueous solutions is low, but there is some evidence for this occurring with non-fluorinated compounds.27–29 In a separate publication, Bergh tried sorbing PFOA with silicalite-1 (MFI) made in hydroxide media and showed little to no sorption. It was proposed that the sorption was sterically hindered due to the critical diameter of PFOA (6.5 Å) being too large to fit into the pore of MFI (5.3 Å × 5.6 Å). It is noted in Table 2 that there is some discrepancy in reported PFOA dimensions between various authors. Additionally, the reported size of zeolite pore openings are often not clarified by authors whether they are assuming ionic or covalent oxygen, as shown in Table 3.30 For aqueous systems there is the added complication that estimations of molecule and pore dimensions, typically made in the absence of water, are not necessarily accurate. A path forward to fully explore silicalite-1's potential would be to synthesize it in fluoride media, try smaller crystallite sizes (nano), or introduce mesoporosity through fluoride etching31 to reduce diffusion limitations.
Table 2 Reported sizes of some PFAS molecules
Molecule |
Length (Å) |
Width (Å) |
Height (Å) |
Lennard Jones radius (Å) |
Ref. |
Perfluorooctanoic acid (PFOA) |
11.1 |
3.6 |
3.5 |
— |
20
|
Perfluorooctanoic acid (PFOA) |
13.0 |
6.5 |
6.5 |
— |
21
|
Perfluorooctanoic acid (PFOA) |
11.0 |
3.6 |
3.6 |
— |
22
|
Perfluorooctanoic acid (PFOA) |
— |
— |
— |
4.73 |
23
|
Perfluorooctane sulfonic acid (PFOS) |
13.6 |
2.3 |
3.6 |
— |
24
|
Potassium perfluorooctane sulfonate (K-PFOS) |
10.88 |
— |
— |
— |
25
|
Table 3 Idealized pore opening dimensions for selected zeolite frameworks30
Framework code |
Number of T atoms in largest pore opening |
Largest pore opening assuming ionic oxygen (Å) |
Largest pore opening assuming covalent oxygen (Å) |
CHA |
8 |
3.8 |
5.0 |
LTA |
8 |
4.1 |
5.3 |
MFI |
10 |
5.3 × 5.6 |
6.3 × 6.8 |
FAU |
12 |
7.4 |
8.6 |
BEA |
12 |
7.6 × 6.4 |
8.8 × 7.6 |
MOR |
12 |
6.5 × 7.0 |
7.7 × 8.2 |
2.3 Mesoporosity and silanization
The introduction of mesoporosity, while giving potential benefits to sorption, also introduces silanol on the external surface of the zeolite. Since silanol defects have a large effect on the hydrophobicity of the zeolite, modeling efforts should reflect actual crystalline structure for the zeolites of interest.32 Similarly, to mesoporous silica, silanol defects can also be targeted as sites to graft silanes onto zeolites. Elimination of silanol will increase hydrophobicity of the zeolite, while also providing functionality from amine, fluorine, or other chemical groups. Silanes are relatively simple to graft to zeolites, but require a dry environment as the hydrolysable groups can react with free water. Different hydrolysable groups also affect the ease of reaction with the zeolite surface, with chloro groups being more reactive than methyl groups, but are more susceptible to self-polymerization by free water. A number of authors have shown that grafting silanes to zeolites is possible, and can utilize the external surface of the zeolite for adsorption.33–35 Additionally, silanization can control the adsorption kinetics into the internal surface of the zeolite, so specific effort is needed to select silanes that won't enter the pores so grafting is restricted to the external surface of the zeolites. The amount of silanes should be tuned as to not cause pore blockage. Ideal silane groups to increase PFAS adsorption should carry cationic charge as well as being hydrophobic. The addition of polar amine groups coupled with fluoroalkyl chains enabled rapid sorption of perfluorobutanoic acid (PFBA) onto a modified mesoporous silica.12Fig. 2 shows this adsorption scheme extended to an external zeolite surface. The addition of fluorine on an alkyl chain has the dual effect of making the surface more hydrophobic, as well as providing a potential for fluorophilic interaction.36 This type of adsorption could be extended to zeolite structures, with the internal siloxane surface providing additional hydrophobic surface for adsorption of fluoroalkyl chains, while cations and or T-atoms provide electrostatic charge for the head groups of PFAS molecules. Adjacent to silane grafting, surfactant modified zeolites have already shown the ability to adsorb negatively charged organic anionic pollutants.37
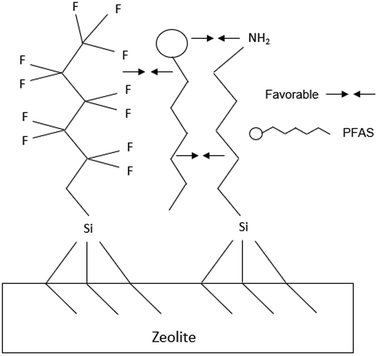 |
| Fig. 2 Possible adsorption scheme of anion form of PFAS at zeolite surface with grafted fluoroalkyl and amino alkyl silane. | |
2.4 Ion exchange of zeolites
Ion exchange of zeolites is a common method of changing the properties of the zeolite. Mancinelli showed that a change in cation improved sorption of PFAS.14 H+ forms of zeolites have the least chance for steric hindrance and pore blockage, but make the zeolite more acidic and hydrophilic so having a non-acidic, positively charged cation might increase sorption by hydrophobic and/or electrostatic effects, but could lead to decreased sorption and/or kinetics due to a decrease in diffusion. Punyapalakul et al.8 showed sorption of PFOA and PFOS on HY and NaY, and in contrast to the more recent publication14 the addition of a cation decreased total sorption and kinetics. This could be due to larger diameter of Na+ compared to Ag+ and/or the location of the cation in the zeolite Y structure. The placement of cations is specific to cation-framework structures pairs and conditions of exchange.38Fig. 3 shows an idealized PFOA adsorption in a straight 12 ring pore, where cations bound to aluminum in the framework balances not only the negative framework, but also to the negatively charged headgroups on PFOA. The illustration also assumes that the framework has no silanol defects, so the siloxane bridges provide hydrophobic interaction which attracts the hydrophobic fluoroalkyl chain.
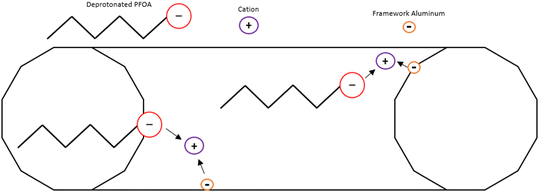 |
| Fig. 3 Possible adsorption scheme of deprotonated PFOA in a 1D, 12-ring pore, ion exchanged zeolite. | |
2.5 T-atom substitution
While zeolites are typically aluminosilicates, there are often substitutions of the silicon and aluminum, known as T atoms, for other atoms.39 Titanium and tin are options in trying to increase PFAS sorption. Titanium in particular displays less hydrophilicity than aluminum per atom40 and has a +4 charge when tetrahedrally coordinated41 making the framework neutral. A neutral framework should reduce the electrostatic repulsion from anions compared to an anionic framework. Additionally, the low electronegativity of Ti increases Lewis acid strength, making it more likely to have attractive interactions with negatively charged head groups of PFAS analytes. Iron containing beta zeolites have already been shown to be able to destroy some PFAS molecules.42,43 Given the photo-catalytic activity of Ti (ref. 44) and its resilience in harsh conditions (H2O2)45 makes Ti-based zeolites, such as beta, promising in sorption and catalytic decomposition of PFAS analytes. There is also PFAS sorption potential for zeolite-like materials such as aluminophosphates (AlPOs), but the instability in many aqueous systems may make them unsuitable for use in water sorption.41 Other metal silicates with zeolite frameworks such as CIT-6 (zinco silicate) should be considered, but lack of commercial availability makes these harder to obtain for testing.
2.6 Characterization considerations
When using custom synthesized materials or materials with no available relevant property data, researchers can measure hydrophobicity by relative humidity water sorption experiments. Attributing hydrophobicity to specific characteristics of the zeolite is more complicated.35 Si-29 NMR can quantify silanol content, but can be prohibitively expensive. FTIR is a low cost alternative to measure SAR and to check relative amounts of silanol content.46 Standard XRF gives higher accuracy of SAR but will need lithium tetraborate fusion when SAR gets very high.47 NMR, FTIR, XRD, XPS, and XRF can provide information about the changes when performing zeolite modifications.
3 Conclusions
• While SAR has been the most explored aspect of PFAS–zeolite sorption, it is vital that researchers broaden their scope to include other unique properties of zeolites.
• Further research regarding synthesis media, SAR, T-atom identity, and repair and/or replacement of silanol with fluoride is recommended.
• Quantification of silanol, measurement of water sorption, and composition measurement to verify successful grafting of silanes.
• Effects of sorption beyond SAR and framework-type need to be taken into account. Specifically, quantification of silanol and/or hydrophobicity of zeolites seem to be much more important than previously thought.
• Experimental evidence showing the hydrogen bond enabled dimer adsorption mechanism for PFOA described by van den Bergh should be validated and/or replicated for PFOA and other PFAS molecules.
• Tuning hydrophobicity through SAR could be a precise way to target specific analytes as short chain PFAS might benefit from some electrostatic interaction, but would also benefit from a hydrophobic environment for their fluoroalkyl tails. Post synthesis modifications such as ion exchange and ammonium fluoride treatments should be explored and synergized to increase adsorption.
Conflicts of interest
The authors declare that they have no known competing financial interest or personal relationships that could have appeared to influence the work reported in this paper.
Acknowledgements
This work has been supported by a grant Novel 3D Printed Zeolite Passive Samplers Enabling Fast and Reliable Measurements of PFAS in Complex Environmental Matrices (EL-32) from the U. S. Army Engineer Research and Development Center.
References
- D. Li, L. Zhang, Y. Zhang, S. Guan, X. Gong and X. Wang, Maternal exposure to perfluorooctanoic acid (PFOA) causes liver toxicity through PPAR-α pathway and lowered histone acetylation in female offspring mice, Environ. Sci. Pollut. Res., 2019, 26, 18866–18875 CrossRef CAS
.
- M. G. Evich, M. J. B. Davis, J. P. McCord, B. Acrey, J. A. Awkerman, D. R. U. Knappe, A. B. Lindstrom, T. F. Speth, C. Tebes-Stevens, M. J. Strynar, Z. Wang, E. J. Weber, W. M. Henderson and J. W. Washington, Per- and polyfluoroalkyl substances in the environment, Science, 2022, 375, eabg9065 CrossRef CAS PubMed
.
- E. Gagliano, M. Sgroi, P. P. Falciglia, F. G. A. Vagliasindi and P. Roccaro, Removal of poly- and perfluoroalkyl substances (PFAS) from water by adsorption: Role of PFAS chain length, effect of organic matter and challenges in adsorbent regeneration, Water Res., 2020, 171, 115381 CrossRef CAS
.
- A. V. Alves, M. Tsianou and P. Alexandridis, Fluorinated surfactant adsorption on mineral surfaces: Implications for PFAS fate and transport in the environment, Surfaces, 2020, 3, 516–566 CrossRef CAS
.
- P. S. Pauletto and T. J. Bandosz, Activated carbon versus metal-organic frameworks: A review of their PFAS adsorption performance, J. Hazard. Mater., 2022, 425, 127810 CrossRef CAS
.
- F. Dixit, R. Dutta, B. Barbeau, P. Berube and M. Mohseni, PFAS removal by ion exchange resins: A review, Chemosphere, 2021, 272, 129777 CrossRef CAS
.
- H. Li, A. L. Junker, J. Wen, L. Ahrens, M. Sillanpää, J. Tian, F. Cui, L. Vergeynst and Z. Wei, A recent overview of per- and polyfluoroalkyl substances (PFAS) removal by functional framework materials, Chem. Eng. J., 2023, 452, 139202 CrossRef CAS
.
- P. Punyapalakul, K. Suksomboon, P. Prarat and S. Khaodhiar, Effects of surface functional groups and porous structures on adsorption and recovery of perfluorinated compounds by inorganic porous silicas, Sep. Sci. Technol., 2013, 48, 775–788 CrossRef CAS
.
- A. Kuvayskaya, B. Lotsi, R. Mohseni and A. Vasiliev, Mesoporous adsorbents for perfluorinated compounds, Microporous Mesoporous Mater., 2020, 305, 110374 CrossRef CAS
.
-
R. K. Iler, Chemistry of silica – solubility, polymerization, colloid and surface properties and biochemistry, John Wiley & Sons, 1979 Search PubMed
.
- M. Nassi, E. Sarti, L. Pasti, A. Martucci, N. Marchetti, A. Cavazzini, F. Di Renzo and A. Galarneau, Removal of perfluorooctanoic acid from water by adsorption on high surface area mesoporous materials, J. Porous Mater., 2014, 14, 423–432 Search PubMed
.
- X. Min and Y. Wang, Enhanced adsorption of short-chain perfluorobutanoic acid by functionalized periodic mesoporous organosilica: Performance and mechanisms, J. Hazard. Mater., 2023, 449, 131047 CrossRef CAS PubMed
.
- V. Ochoa-Herrera and R. Sierra-Alvarez, Removal of perfluorinated surfactants by sorption onto granular activated carbon, zeolite and sludge, Chemosphere, 2008, 72, 1588–1593 CrossRef CAS
.
- M. Mancinelli, C. Stevanin, M. Ardit, T. Chenet, L. Pasti and A. Martucci, PFAS as emerging pollutants in the environment: A challenge with FAU type and silver-FAU exchanged zeolites for their removal from water, J. Environ. Chem. Eng., 2022, 10, 108026 CrossRef CAS
.
- M. J. Cordon, J. W. Harris, J. C. Vega-Vila, J. S. Bates, S. Kaur, M. Gupta, M. E. Witzke, E. C. Wegener, J. T. Miller, D. W. Flaherty, D. D. Hibbitts and R. Gounder, Dominant role of entropy in stabilizing sugar isomerization transition states within hydrophobic zeolite pores, J. Am. Chem. Soc., 2018, 140, 14244–14266 CrossRef CAS
.
- M. A. Camblor, A. Corma and S. Valencia, Synthesis in fluoride media and characterisation of aluminosilicate
zeolite beta, J. Mater. Chem., 1998, 8, 2137–2145 RSC
.
- D. P. Serrano, R. Van Grieken, P. Sánchez, R. Sanz and L. Rodríguez, Crystallization mechanism of all-silica zeolite beta in fluoride medium, Microporous Mesoporous Mater., 2001, 46, 35–46 CrossRef CAS
.
- K. Iyoki, K. Kikumasa, T. Onishi, Y. Yonezawa, A. Chokkalingam, Y. Yanaba, T. Matsumoto, R. Osuga, S. P. Elangovan, J. N. Kondo, A. Endo, T. Okubo and T. Wakihara, Extremely stable zeolites developed via designed liquid-mediated treatment, J. Am. Chem. Soc., 2020, 142, 3931–3938 CrossRef CAS
.
-
F. Clark, R. Garcia and A. Turrina, Comparison of different defect-healing treatments for Zeolite Beta, presented in part at in 20th International Zeolite Conference, Valencia, July, 2022 Search PubMed
.
- P.-H. Chang, W.-T. Jiang and Z. Li, Removal of perfluorooctanoic acid from water using calcined hydrotalcite – A mechanistic study, J. Hazard. Mater., 2019, 368, 487–495 CrossRef CAS
.
- M. Van den Bergh, A. Krajnc, S. Voorspoels, S. R. Tavares, S. Mullens, I. Beurroies, G. Maurin, G. Mali and D. E. De Vos, Highly selective removal of perfluorinated contaminants by adsorption on all-silica zeolite beta, Angew. Chem., Int. Ed., 2020, 59, 14086–14090 CrossRef CAS
.
- X. Liu, C. Zhu, J. Yin, J. Li, Z. Zhang, J. Li, F. Shui, Z. You, Z. Shi, B. Li, X.-H. Bu, A. Nafady and S. Ma, Installation of synergistic binding sites onto porous organic polymers for efficient removal of perfluorooctanoic acid, Nat. Commun., 2022, 13, 2132 CrossRef CAS
.
- M. Zhang, F. Sicard, T. S. Erkal, G. M. Bowers and A. O. Yazaydin, The role of surface thermodynamics and kinetics in the removal of PFOA from aqueous solutions, Surf. Interfaces, 2023, 41, 103271 CrossRef CAS
.
- P.-H. Chang, Z. Li and W.-T. Jiang, Calcination of hydrotalcite to enhance the removal of perfluorooctane sulfonate from water, Appl. Clay Sci., 2020, 190, 105563 CrossRef CAS
.
- C. Zhao, J. Zhang, G. He, T. Wang, D. Hou and Z. Luan, Perfluorooctane sulfonate removal by nanofiltration membrane the role of calcium ions, Chem. Eng. J., 2013, 233, 224–232 CrossRef CAS
.
- E. Steinle-Darling and M. Reinhard, Nanofiltration for trace organic contaminant removal: Structure, solution, and membrane fouling effects on the rejection of perfluorochemicals, Environ. Sci. Technol., 2008, 42, 5292–5297 CrossRef CAS PubMed
.
- J. M. Briggs, T. B. Nguyen and W. L. Jorgensen, Monte Carlo simulations of liquid acetic acid and methyl acetate with the OPLS potential functions, J. Phys. Chem., 1991, 95, 3315–3322 CrossRef CAS
.
- J. Chen, C. L. Brooks and H. A. Scheraga, Revisiting the carboxylic acid dimers in aqueous solution: Interplay of hydrogen bonding, hydrophobic interactions, and entropy, J. Phys. Chem. B, 2008, 112, 242–249 CrossRef CAS
.
- D. Tzeli, G. Theodorakopoulos, I. D. Petsalakis, D. Ajami and J. Rebek, Theoretical study of hydrogen bonding in homodimers and heterodimers of amide, boronic acid, and carboxylic acid, free and in encapsulation complexes, J. Am. Chem. Soc., 2011, 133, 16977–16985 CrossRef CAS
.
- L. Abrams and D. R. Corbin, Probing intrazeolite space, J. Inclusion Phenom. Mol. Recognit. Chem., 1995, 21, 1–46 CrossRef CAS
.
- Z. Qin, J.-P. Gilson and V. Valtchev, Mesoporous zeolites by fluoride etching, Curr. Opin. Chem. Eng., 2015, 8, 1–6 CrossRef
.
- R. F. DeJaco, M. Dorneles de Mello, H. G. T. Nguyen, M. Y. Jeon, R. D. van Zee, M. Tsapatsis and J. I. Siepmann, Vapor- and liquid-phase adsorption of alcohol and water in silicalite-1 synthesized in fluoride media, AIChE
J., 2020, 66, e16868 CrossRef CAS
.
- K. A. Northcott, J. Bacus, N. Taya, Y. Komatsu, J. M. Perera and G. W. Stevens, Synthesis and characterization of hydrophobic zeolite for the treatment of hydrocarbon contaminated ground water, J. Hazard. Mater., 2010, 183, 434–440 CrossRef CAS PubMed
.
- L. Bonaccorsi, P. Bruzzaniti, L. Calabrese and E. Proverbio, Organosilanes functionalization of alumino-silica zeolites for water adsorption applications, Microporous Mesoporous Mater., 2016, 234, 113–119 CrossRef CAS
.
- D. Sirikittikul, A. Fuongfuchat and W. Booncharoen, Chemical modification of zeolite beta surface and its effect on gas permeation of mixed matrix membrane, Polym. Adv. Technol., 2009, 20, 802–810 CrossRef CAS
.
- C. Fang, Z. Sobhani, M. Megharaj and R. Naidu, Electrochemical proof of fluorophilic interaction among fluoro-carbon chains, Electroanalysis, 2018, 30, 2349–2355 CrossRef CAS
.
- Q. Xie, J. Xie, Z. Wang, D. Wu, Z. Zhang and H. Kong, Adsorption of organic pollutants by surfactant modified zeolite as controlled by surfactant chain length, Microporous Mesoporous Mater., 2013, 179, 144–150 CrossRef CAS
.
-
W. J. Mortier, Compilation of Extra Framework Sites In Zeolites, Butterworth Scientific Limited, Surrey, 1982 Search PubMed
.
- R. Gounder and M. E. Davis, Beyond shape selective catalysis with zeolites: Hydrophobic void spaces in zeolites enable catalysis in liquid water, AIChE J., 2013, 59, 3349–3358 CrossRef CAS
.
- S. P. Mirajkar, A. Thangaraj and V. P. Shiralkar, Sorption properties of titanium silicate molecular sieves, J. Phys. Chem., 1992, 96, 3073–3079 CrossRef CAS
.
-
R. Szostak, Molecular sieves principles of synthesis and identification, Blackie Academic & Professional, London, 1998 Search PubMed
.
- L. Qian, A. Georgi, R. Gonzalez-Olmos and F.-D. Kopinke, Degradation of perfluorooctanoic acid adsorbed on Fe-zeolites with molecular oxygen as oxidant under UV-A irradiation, Appl. Catal., B, 2020, 278, 119283 CrossRef CAS
.
- L. Qian, F.-D. Kopinke, T. Scherzer, J. Griebel and A. Georgi, Enhanced degradation of perfluorooctanoic acid by heat-activated persulfate in the presence of zeolites, Chem. Eng. J., 2022, 429, 132500 CrossRef CAS
.
- S. C. E. Leung, P. Shukla, D. Chen, E. Eftekhari, H. An, F. Zare, N. Ghasemi, D. Zhang, N.-T. Nguyen and Q. Li, Emerging technologies for PFOS/PFOA degradation and removal: A review, Sci. Total Environ., 2022, 827, 153669 CrossRef CAS PubMed
.
- X. Fang, Q. Wang, A. Zheng, Y. Liu, Y. Wang, X. Deng, H. Wu, F. Deng, M. He and P. Wu, Fluorine-planted titanosilicate with enhanced catalytic activity in alkene epoxidation with hydrogen peroxide, Catal. Sci. Technol., 2012, 2, 2433–2435 RSC
.
-
E. Flanigen, Structural Analysis by Infrared Spectroscopy, in Zeolite chemistry and catalysis ACS Monograph 171, American Chemical Society, 1976, pp. 80–118 Search PubMed
.
- J. R. Sieber, Matrix-independent XRF method for certification of standard reference materials, Adv. X-Ray Anal., 2002, 45, 493–504 CAS
.
|
This journal is © Institute of Process Engineering of CAS 2024 |
Click here to see how this site uses Cookies. View our privacy policy here.