DOI:
10.1039/D3IM00078H
(Minireview)
Ind. Chem. Mater., 2024,
2, 276-283
Palladium-catalyzed carbonylation of activated alkyl halides via radical intermediates
Received
16th July 2023
, Accepted 15th August 2023
First published on 16th August 2023
Abstract
Palladium-catalyzed carbonylation is an efficient approach to prepare carbonyl-containing compounds with high atomic economy in synthetic organic chemistry. However, in comparison with aryl halides, carbonylation of alkyl halides is relatively challenging due to the decreased stability of the palladium intermediates. Carbonylation of activated alkyl halides is even more difficult, as nucleophilic substitution reactions with nucleophiles occur more easily with them. In this article, we summarize and discuss recent achievements in palladium-catalyzed carbonylative reactions of activated alkyl halides. The transformations proceed through radical intermediates which are generated in various manners. Under a relatively high pressure of carbon monoxide, the corresponding aliphatic carboxylic acid derivates were effectively prepared with various nucleophiles as the reaction partners. Besides alcohols, amines and organoboron reagents, four-component reactions in combination with alkenes or alkynes were also developed. Case-by-case reaction mechanisms are discussed as well and a personal outlook has also been provided.
Keywords: Carbonyl group; Palladium catalysis; Carbonylation; Activated alkyl halides; Radical intermediates.
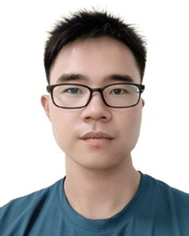 Zhi-Peng Bao | Zhi-Peng Bao was born in Anhui, China in 1996. He received his M.S. degree in 2021 from Zhejiang Sci-Tech University. He is currently studying towards his PhD degree under the joint training of the Leibniz Institute for Catalysis (Germany) and Dalian Institute of Chemical Physics (DICP) under the supervision of Prof. Xiao-Feng Wu. His current research interests focus on palladium-catalyzed carbonylation of activated alkyl halides. |
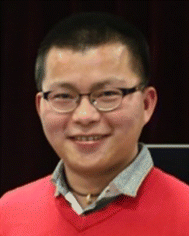 Xiao-Feng Wu | Xiao-Feng Wu was born in China. He was educated at Zhejiang Sci-Tech University (bachelor, 2003–2007, China), Rennes 1 University (Master, 2007–2009, France) and the Leibniz-Institute for Catalysis-Rostock University (PhD, 2009–2012, Germany). Then, he started his independent research at LIKAT and ZSTU where he was promoted to professor in 2013 and defended his Habilitation from Rennes 1 University (2017, France). In 2020, he joined Dalian Institute of Chemical Physics (DICP). Xiao-Feng has authored >550 publications in international journals; meanwhile, he is also the editor or author of over 10 books. |
1 Introduction
Carbon monoxide (CO) is an inexpensive and abundant one-carbon source in chemical synthesis, and employing it to undertake the carbonylative process is a direct and efficient way to construct valuable carbonyl-containing compounds.1,2 Remarkable progress has been made in transition-metal-catalyzed carbonylation reactions after decades of development. This kind of transformation has now become one of the most powerful methods for the synthesis of carbonylated compounds and has also been successfully used as a key step in the total synthesis of many natural products.3,4 More importantly, many carbonylative procedures have been industrialized. For instance, the catalytic double carbonylative reaction of benzyl halides and the carbonylation of epoxides have been performed at large scale, and the Monsanto or Cativa processes make the majority of the world's acetic acid production through methanol carbonylation.2,5,6
Palladium-catalyzed carbonylative reactions have experienced rapid development during past decades. In 1974, Heck and co-workers reported the first palladium-catalyzed carbonylation reaction of aryl halides and benzyl halogenated compounds.7 Then, Ryu and co-workers reported their study on the free-radical-mediated carbonylation of unactivated alkyl halides in 1990.8 The same group also reported a Pd/light-induced multicomponent carbonylative coupling reaction of activated halides with alkenes in 2011.9 In 2020, Arndtsen and co-workers developed a seminal strategy that exploits simple visible-light excitation of palladium to drive both oxidative addition and reductive elimination with low barriers. Both aryl halides and unactivated halides can be effectively converted into the corresponding carbonylated compounds (Scheme 1).10
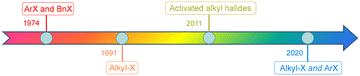 |
| Scheme 1 Landmarks of palladium-catalyzed carbonylative transformations of various halides. | |
In synthetic chemistry, the efficient assembly of complex compounds from simple starting materials is a challenging and long-standing goal. In this article, we summarize and discuss recent studies on palladium-catalyzed carbonylative transformations of activated alkyl halides which are able to construct a wide variety of carbonylated compounds and their derivatives with valuable functional groups, including fluorinated groups, cyano groups, and ester groups. The contents are mainly categorized according to two aspects: i) palladium-catalyzed direct carbonylation of activated alkyl halides with nucleophiles (Scheme 2); ii) palladium-catalyzed carbonylation of activated alkyl halides and nucleophiles with alkenes or alkynes (Scheme 3).
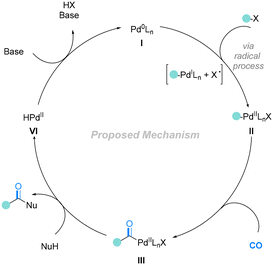 |
| Scheme 2 Palladium-catalyzed direct carbonylation of activated alkyl halides with nucleophiles. | |
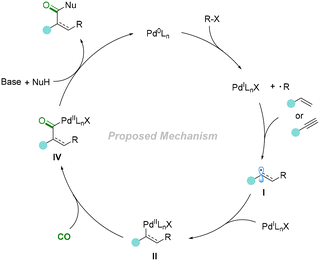 |
| Scheme 3 Palladium-catalyzed carbonylation of activated alkyl halides and nucleophiles with alkenes or alkynes. | |
2 Palladium-catalyzed direct carbonylation of activated alkyl halides with nucleophiles
Organic fluorides play a vital role in medical chemistry, and materials science due to their special physical and chemical properties. The introduction of a fluorine atom into organic molecules could change their biological activity and physical properties.11–14 On the other hand, employing commercially available fluorine-containing activated halides to introduce fluorine atoms into molecules is an efficient method to construct fluorine-containing compounds. In 2016, Zhang's group15 and Skrydstrup's group16 reported elegant transformations of Pd-catalyzed carbonylative coupling reactions of difluoroalkyl bromides. The main challenge is that the reaction intermediates (Rf–M) are less prone to undergoing carbonyl insertion because the σ-bonds between fluoroalkyl groups and transitional metals are stronger than their normal hydrocarbon counterparts. This transformation not only provides us with a new way of viewing palladium-catalyzed fluoroalkylative carbonylation but also inspires us to probe this strategy to build up some valuable bioactive molecules (Scheme 4).
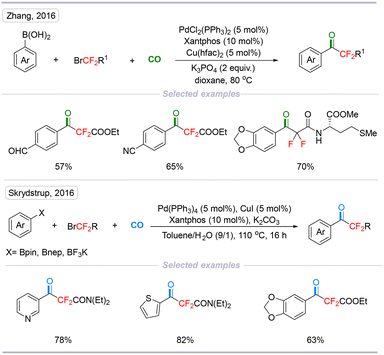 |
| Scheme 4 Palladium-catalyzed direct carbonylation of difluoroalkyl bromides and aryl boron reagents. | |
Later, in 2018, Skrydstrup's group provided a direct route to construct α,α-difluoro-β-alkyl-β-ketoamides via Pd-catalyzed carbonylative coupling between bromodifluoroacetamides and alkyl boron reagents.17 This transformation has good chemoselectivity and provided a broad selection of α,α-difluoro-β-alkyl-β-ketoamides which are suitable for further synthetic manipulation (Scheme 5).
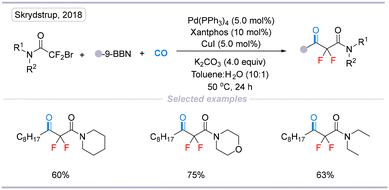 |
| Scheme 5 Palladium-catalyzed direct carbonylation of difluoroalkyl bromides and alkyl boron reagents. | |
In 2021, Zhang's group reported a palladium-catalyzed carbonylative cross-coupling of difluoroalkyl halides with alkyl-9-BBN under 1 bar of CO.18 The reaction overcomes the hydrodehalogenation and β-hydride elimination of unactivated difluoroalkyl halides, representing the first example of catalytic carbonylation of unactivated difluoroalkyl halides (Scheme 6).
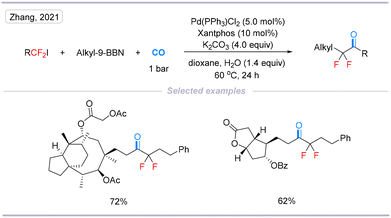 |
| Scheme 6 Palladium-catalyzed direct carbonylation of unactivated difluoroalkyl halides with alkylboranes. | |
In 2023, our group developed a novel palladium-catalyzed carbonylative procedure for bromoacetonitrile using amines, phenols, and alcohols as the nucleophiles via a radical intermediate. A variety of valuable 2-cyano-N-acetamide and 2-cyanoacetate products were obtained in excellent yields under mild reaction conditions. Additionally, a scale-up reaction was performed smoothly with low catalyst loading. Moreover, this transformation can be carried out under atmospheric pressure and provide alternative routes to 7 drug precursors. This transformation overcame the challenge that activated haloalkanes are prone to undergoing nucleophilic substitution reactions with strong nucleophiles and tend to be reduced by dehalogenation (Scheme 7).19
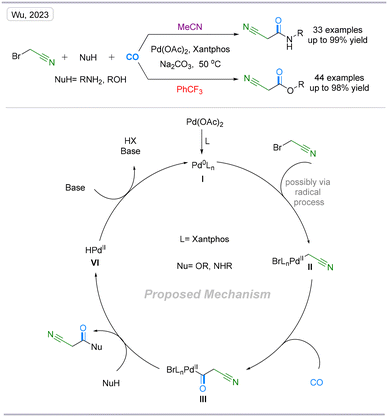 |
| Scheme 7 Palladium-catalyzed direct carbonylation of bromoacetonitrile to synthesize 2-cyano-N-acetamide or 2-cyanoacetate compounds. | |
3 Palladium-catalyzed carbonylation of activated alkyl halides and nucleophiles with alkenes or alkynes
Multicomponent reactions (MCRs), in which three or more reactants are combined in a single reaction vessel ending up with one main product, is an efficient way to synthesize highly functionalized molecules from readily available synthons. Carbon monoxide (CO) is a cheap industrial raw material, and employing it in multicomponent reactions to build up some valuable scaffolds is an efficient and attractive method.20–23
In 2011, Ryu's group developed a pioneering and interesting carbonylative four-component transformation comprising α-substituted iodoalkanes, alkenes, and alcohols. The reaction proceeded smoothly to give functionalized esters in good yields under photoirradiation conditions using xenon light, and a three-component coupling reaction accompanied by intramolecular esterification proceeded to give lactones in good yields (Scheme 8).9
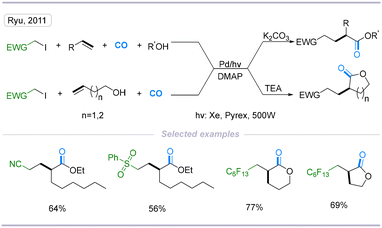 |
| Scheme 8 Palladium-catalyzed four-component fluoroalkylative carbonylation. | |
In 2016, Liang's group reported a Pd-catalyzed four-component radical carbonylation and difluoroalkylation reaction utilizing ethyl difluoroiodoacetate as a CF2 radical precursor under a low pressure of CO in a single step using alcohols, phenols, and amines as nucleophiles.24 In 2017, the same group developed a Pd-catalyzed four-component perfluoroalkylative carbonylation by using arylboronic acids as carbon nucleophiles under 1 bar of CO at room temperature (Scheme 9).25
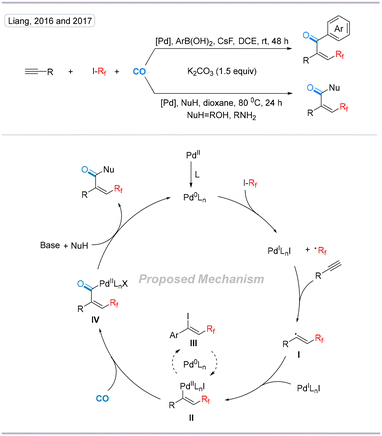 |
| Scheme 9 Palladium-catalyzed four-component fluoroalkylative carbonylation of aryl alkynes. | |
In 2017, Skrydstrup's group reported a convenient method to access perfluoroalkyl-substituted enones using a four-component palladium-catalyzed carbonylative coupling of aryl boronic acids together with terminal alkynes and perfluoroalkyl iodides in the presence of CO. A wide range of highly functionalized enones can be prepared in a single operation in good yields. With 2-aminophenyl alkynes as the substrates, intramolecular aminocarbonylation proceeded smoothly to provide the desired indolin-2-one framework. Finally, the authors expanded the two-chamber technology to synthesize the target compounds with 13C-isotope labeling (Scheme 10).26
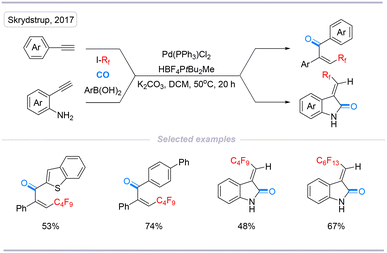 |
| Scheme 10 Palladium-catalyzed perfluoroalkylative carbonylation of alkyne to synthesize perfluoroalkyl-substituted enones and indolin-2-ones. | |
In 2018, Liang's group reported a palladium-catalyzed perfluoroalkylative/difluoroalkylative carbonylation of alkyne with alkyne as the nucleophile. This interesting transformation proceeded under atmospheric pressure at room temperature (Scheme 11).27
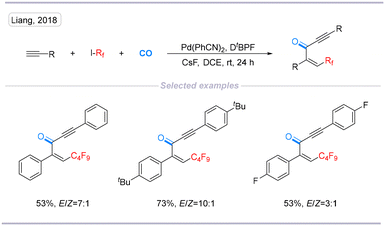 |
| Scheme 11 Palladium-catalyzed perfluoroalkylative and difluoroalkylative carbonylation of alkyne using alkyne as a nucleophile. | |
In 2021, our group reported a palladium-catalyzed four-component perfluoroalkylative carbonylation of unactivated alkenes with perfluoroalkyl halides, amines, or phenols (Scheme 12). With dioxane as a solvent, we got β-perfluoroalkyl esters while β-perfluoroalkyl amides were obtained with benzotrifluoride as the solvent. This transformation proceeded under mild conditions and is suitable for the modification of pharmaceutical and drug molecules. Furthermore, a wide range of substrates, including phenols, anilines, alkylamines, sulfonamides, and hydrazines, are suitable reaction partners for this catalyst system, resulting in various β-perfluoroalkyl amides with good functional-group tolerance and excellent chemoselectivity.28,29 In 2022, our group reported a novel palladium-catalyzed procedure for the difluoroalkylative carbonylation of aryl olefins (Scheme 13).30
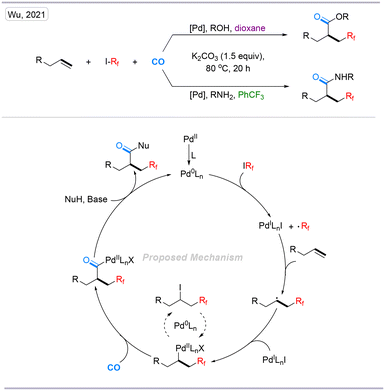 |
| Scheme 12 Palladium-catalyzed perfluoroalkylative carbonylation of unactivated alkenes to synthesize β-perfluoroalkyl esters and amides. | |
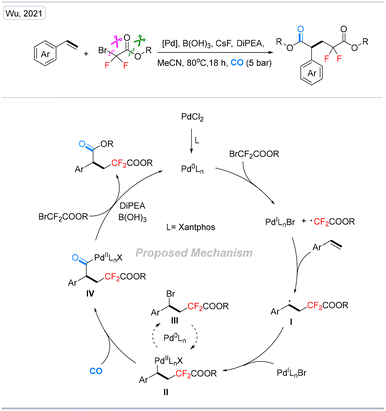 |
| Scheme 13 Palladium-catalyzed difluoroalkylative carbonylation of aryl alkenes toward corresponding difluoropentanedioates. | |
The catalytic cycle was initiated from the active catalyst Pd0Ln species, which was produced from the PdCl2 pre-catalyst. Then, the Pd0Ln complex induced an SET (single-electron transfer) process of bromodifluoroacetate to give the corresponding difluoroacetate radical and a PdILnX species, followed by the addition of the difluoroacetate radical to aryl alkenes to give a secondary benzylic radical I. Then, the PdILnX species was reincorporated with carbon radical I to obtain the vital intermediate II. It should be mentioned that complex II could be converted into III through reductive elimination. However, the reaction is reversible, and compound III could react with the reactive Pd0Ln species and reconverted into II. After the insertion of CO, complex II will be transformed into intermediate IV. Finally, complex IV reacts with another molecule of bromodifluoroacetate and gives the final product after reductive elimination. Meanwhile, in the presence of DiPEA, Pd0Ln will be regenerated for the next catalytic cycle.
In the same year, we developed a palladium-catalyzed four-component difluoroalkylative carbonylation of aryl olefins. Notably, ethylene gas, as an original C2 synthon, can also be transformed into the corresponding products with moderate yields (Scheme 14).31
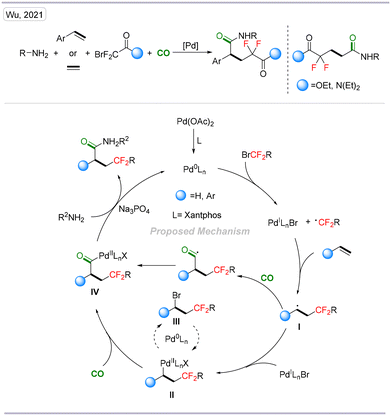 |
| Scheme 14 Palladium-catalyzed four-component difluoroalkylative carbonylation of aryl alkenes and ethylene gas. | |
In 2022, we developed a difluoroalkylative carbonylative cyclization of unactivated alkenes and ethylene gas enabled by palladium catalysis (Scheme 15). This transformation proceeded in mild conditions with good functional-group and heterocyclic compatibility, which is suitable for late-stage functionalization. Moreover, this procedure can also be applied to the synthesis of gemigliptin, which is a medicine approved for the treatment of type 2 diabetes mellitus.32
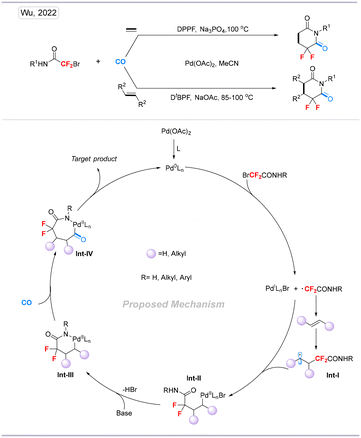 |
| Scheme 15 Palladium-catalyzed difluoroalkylative carbonylation of alkenes to carbonyl difluoro-containing heterocycles. | |
In 2022, our group reported an efficient palladium-catalyzed multicomponent perfluoroalkylative carbonylation to synthesize various amides, and 2-allyl trifluoromethanesulfonates, perfluoroalkyl halides and amines were applied in this tandem process to get the corresponding β-perfluoroalkyl amides with good functional-group tolerance and high chemoselectivity (Scheme 16). The various final products were controlled by the different bases applied.33
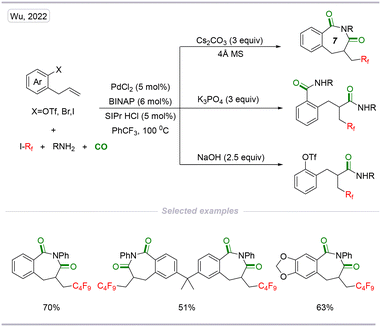 |
| Scheme 16 Palladium-catalyzed perfluoroalkylative carbonylation of 2-allylaryl trifluoromethanesulfonates. | |
In the same year, a palladium-catalyzed cascade radical cyclization and carbonylation of 1,7-enynes with perfluoroalkyl iodides and alcohols was developed by our group (Scheme 17). This method provides a facile and efficient route for the construction of 3,4-dihydroquinolin-2(1H)-one scaffolds by applying benzene-1,3,5-triyl triformate (TFBen) as the CO source. This method enables the incorporation of both perfluoroalkyl and carbonyl units into the 3,4-dihydroquinolin-2(1H)-one scaffolds, providing different 3,4-dihydroquinolin-2(1H)-one derivatives in moderate to high yields with excellent E/Z selectivity.34
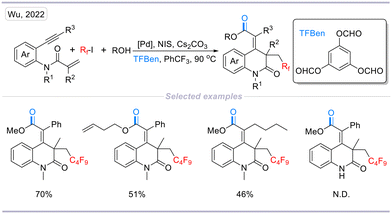 |
| Scheme 17 Palladium-catalyzed cascade carbonylative synthesis of perfluoroalkyl and carbonyl-containing 3,4-dihydroquinolin-2(1H)-ones. | |
In 2023, a one-pot two-step radical-mediated carbonylative cyclization of 1,7-enynes with perfluoroalkyl iodides catalyzed by palladium was developed, and a variety of polycyclic 3,4-dihydroquinolin-2(1H)-one derivatives containing perfluoroalkyl and carbonyl units were obtained in good yields (Scheme 18).35
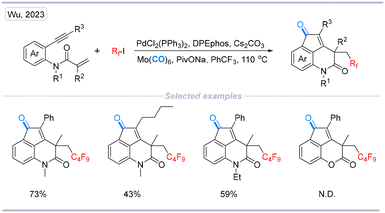 |
| Scheme 18 Palladium-mediated cascade carbonylative synthesis of perfluoroalkyl and carbonyl-containing polycyclic 3,4-dihydroquinolin-2(1H)-one derivatives. | |
4 Summary and outlook
In conclusion, this review has surveyed recent advances in the area of palladium-catalyzed carbonylative coupling conversion of activated alkyl halides via radical intermediates. These transformations can be performed under a low pressure of CO, unlike traditional free radical carbonylation reactions. More importantly, these methods provide alternative routes to construct some bioactive drug molecules due to the introduction of some interesting functional groups. Although palladium-catalyzed carbonylative coupling reactions of activated alkyl halides have developed very well, several interesting directions still need to be probed: i) employing cheaper metal catalysts to achieve these transformations; ii) improving these interesting methods to synthesize some useful drug molecules or bioactive compounds with shorter steps; iii) developing a novel catalyst system to achieve the enantioselective carbonylation reaction of activated alkyl halides. Finally, we hope that this review will inspire future developments in this area.
Conflicts of interest
There are no conflicts to declare.
Acknowledgements
We thank the financial support from DICP and K. C. Wong Education Foundation (GJTD-2020-08).
References
-
(a) J.-B. Peng, H.-Q. Geng and X.-F. Wu, The chemistry of CO: Carbonylation, Chem, 2019, 5, 526–552 CrossRef CAS;
(b) Z. Yin, J.-X. Xu and X.-F. Wu, No making without breaking: nitrogen-centered carbonylation reactions, ACS Catal., 2020, 10, 6510–6531 CrossRef CAS;
(c)
X.-F. Wu, B. Han, K. Ding and Z. Liu, The Chemical Transformations of C1 Compounds, Wiley-VCH, Weinheim, 2022 CrossRef.
- J.-B. Peng, F. P. Wu and X. F. Wu, First-row transition-metal-catalyzed carbonylative transformations of carbon electrophiles, Chem. Rev., 2019, 119, 2090–2127 CrossRef CAS PubMed.
- Y. Bai, D. C. Davis and M. Dai, Natural product synthesis via palladium-catalyzed carbonylation, J. Org. Chem., 2017, 82, 2319–2328 CrossRef CAS PubMed.
- Y. Wang and Z. X. Yu, Rhodium-catalyzed [5+2+1] cycloaddition of ene–vinylcyclopropanes and CO: Reaction design, development, application in natural product synthesis, and inspiration for developing new reactions for synthesis of eight-membered carbocycles, Acc. Chem. Res., 2015, 48, 2288–2296 CrossRef CAS.
- T. W. Dekleva and D. Forster, The rhodium-catalyzed carbonylation of linear primary alcohols, J. Am. Chem. Soc., 1985, 107, 3565–3567 CrossRef CAS.
- A. Haynes, P. M. Maitlis, G. E. Morris, G. J. Sunley, H. Adams, P. W. Badger, C. M. Bowers, D. B. Cook, P. I. P. Elliott, T. Ghaffar, H. Green, T. R. Griffin, M. Payne, J. M. Pearson, M. J. Taylor, P. W. Vickers and R. J. Watt, Promotion of Iridium-catalyzed methanol carbonylation: Mechanistic studies of the cativa process, J. Am. Chem. Soc., 2004, 126, 2847–2861 CrossRef CAS PubMed.
- A. Schoenberg, I. Bartoletti and R. F. Heck, Palladium-catalyzed carboalkoxylation of aryl, benzyl, and vinylic halides, J. Org. Chem., 1974, 39, 3318–3326 CrossRef CAS.
- I. Ryu, K. Kusano, A. Ogawa, N. Kambe and N. Sonoda, Free radical carbonylation. Efficient trapping of carbon monoxide by carbon radicals, J. Am. Chem. Soc., 1990, 112, 1295–1297 CrossRef CAS.
- A. Fusano, S. Sumino, T. Fukuyama and I. Ryu, Vicinal C-functionalization of alkenes. Pd/light-induced multicomponent coupling reactions leading to functionalized esters and lactones, Org. Lett., 2011, 13, 2114–2117 CrossRef CAS PubMed.
-
(a) G. M. Torres, Y. Liu and B. A. Arndtsen, A dual light-driven palladium catalyst: Breaking the barriers in carbonylation reactions, Science, 2020, 368, 318–323 CrossRef CAS PubMed;
(b) G.-Z. Wang, R. Shang, W.-M. Cheng and Y. Fu, Irradiation-Induced Heck Reaction of Unactivated Alkyl Halides at Room Temperature, J. Am. Chem. Soc., 2017, 139, 18307–18312 CrossRef CAS PubMed.
- Y. Zhou, J. Wang, Z. Gu, S. Wang, W. Zhu, J. L. Aceña, V. A. Soloshonok, K. Izawa and H. Liu, Next generation of fluorine-containing pharmaceuticals, compounds currently in phase II–III clinical trials of major pharmaceutical companies: New structural trends and therapeutic areas, Chem. Rev., 2016, 116, 422–518 CrossRef CAS.
- M. Salwiczek, E. K. Nyakatura, U. I. M. Gerling, S. Ye and B. Koksch, Fluorinated amino acids: Compatibility with native protein structures and effects on protein–protein interactions, Chem. Soc. Rev., 2012, 41, 2135–2171 RSC.
- W. Zhang, Fluorous linker-facilitated chemical synthesis, Chem. Rev., 2009, 109, 749–795 CrossRef CAS PubMed.
- W. Zhou, W.-J. Pan, J. Chen, M. Zhang, J.-H. Lin, W. Cao and J.-C. Xiao, Transition-metal difluorocarbene complexes, Chem. Commun., 2021, 57, 9316–9329 RSC.
- H. Y. Zhao, Z. Feng, Z. Luo and X. Zhang, Carbonylation of difluoroalkyl bromides catalyzed by palladium, Angew. Chem., Int. Ed., 2016, 55, 10401–10405 CrossRef CAS.
- T. L. Andersen, M. W. Frederiksen, K. Domino and T. Skrydstrup, Direct access to α, α-difluoroacylated arenes by palladium-catalyzed carbonylation of (hetero)aryl boronic acid derivatives, Angew. Chem., Int. Ed., 2016, 55, 10396–10400 CrossRef CAS PubMed.
- H. Yin, J. J. Kumke, K. Domino and T. Skrydstrup, Palladium catalyzed carbonylative coupling of alkyl boron reagents with bromodifluoroacetamides, ACS Catal., 2018, 8, 3853–3858 CrossRef CAS.
- H. Y. Zhao, M. Zhou and X. Zhang, Palladium-catalyzed carbonylative cross-coupling of difluoroalkyl halides with alkylboranes under 1 atm of CO, Org. Lett., 2021, 23, 9106–9111 CrossRef CAS PubMed.
- Z.-P. Bao and X.-F. Wu, Palladium-catalyzed direct carbonylation of bromoacetonitrile to synthesize 2-cyano-N-acetamide and 2-cyanoacetate compounds, Angew. Chem., 2023, 62, e202301671 CrossRef CAS PubMed.
- C. M. Volla, I. Atodiresei and M. Rueping, Catalytic C–C bond-forming multi-component cascade or domino reactions: Pushing the boundaries of complexity in asymmetric organocatalysis, Chem. Rev., 2014, 114, 2390–2431 CrossRef CAS.
- G. H. Posner, Multicomponent one-pot annulations forming 3 to 6 bonds, Chem. Rev., 1986, 86, 831–844 CrossRef CAS.
- D. M. D'Souza and T. J. J. Müller, Multi-component syntheses of heterocycles by transition-metal catalysis, Chem. Soc. Rev., 2007, 36, 1095–1108 RSC.
- C. M. Marson, Multicomponent and sequential organocatalytic reactions: Diversity with atom-economy and enantiocontrol, Chem. Soc. Rev., 2012, 41, 7712–7722 RSC.
- Q. Wang, Y. T. He, J. H. Zhao, Y. F. Qiu, L. Zheng, J. Y. Hu, Y. C. Yang, X. Y. Liu and Y. M. Liang, Palladium-catalyzed regioselective difluoroalkylation and carbonylation of alkynes, Org. Lett., 2016, 18, 2664–2667 CrossRef CAS PubMed.
- Q. Wang, L. Zheng, Y. T. He and Y. M. Liang, Regioselective synthesis of difluoroalkyl/perfluoroalkyl enones via Pd-catalyzed four-component carbonylative coupling reactions, Chem. Commun., 2017, 53, 2814–2817 RSC.
- H. Yin and T. Skrydstrup, Access to perfluoroalkyl-substituted enones and indolin-2-ones via multicomponent Pd-catalyzed carbonylative reactions, J. Org. Chem., 2017, 82, 6474–6481 CrossRef CAS.
- Q. Wang, X. Yu, J. Jin, Y. Wu and Y. Liang, Difunctionalization of alkynes: Synthesis of novel fluoropolymer materials, Chin. J. Chem., 2018, 36, 223–226 CrossRef CAS.
- Y. Zhang, H. Q. Geng and X.-F. Wu, Palladium-catalyzed perfluoroalkylative carbonylation of unactivated alkenes: Access to β-perfluoroalkyl esters, Angew. Chem., 2021, 60, 24292–24298 CrossRef CAS.
- Y. Zhang, H. Q. Geng and X. F. Wu, Palladium-catalyzed carbonylative four-component synthesis of β-perfluoroalkyl amides, Chem. – Eur. J., 2021, 27, 17682–17687 CrossRef CAS PubMed.
- Z.-P. Bao, Y. Zhang and X.-F. Wu, Palladium-catalyzed difluoroalkylative carbonylation of styrenes toward difluoropentanedioates, Chem. Sci., 2022, 13, 9387–9391 RSC.
- Z.-P. Bao, Y. Zhang and X.-F. Wu, Palladium-catalyzed four-component difluoroalkylative carbonylation of aryl olefins and ethylene, J. Catal., 2022, 413, 163–167 CrossRef CAS.
- Z.-P. Bao, Y. Zhang, L.-C. Wang and X.-F. Wu, Difluoroalkylative carbonylation of alkenes to access carbonyl difluoro-containing heterocycles: Convenient synthesis of gemigliptin, Sci. China: Chem., 2022, 66, 139–146 CrossRef.
- Y. Zhang, Z.-P. Bao, J.-X. Xu and X.-F. Wu, Palladium-catalyzed perfluoroalkylative carbonylation of 2-allylaryl trifluoromethanesulfonates: Base-controlled selective access to β-perfluoroalkyl amides, Org. Lett., 2022, 24, 6845–6850 CrossRef CAS.
- S. Wang, J. Zhang, J. S. Wang, J. Ying and X. F. Wu, Palladium-catalyzed cascade carbonylative synthesis of perfluoroalkyl and carbonyl-containing 3,4-dihydroquinolin-2(1H)-one derivatives, Org. Lett., 2022, 24, 8843–8847 CrossRef CAS.
- S. Wang, J. Zhang, J. Ying and X. F. Wu, Palladium-catalyzed carbonylative synthesis of polycyclic 3,4-dihydroquinolin-2(1H)-one scaffolds containing perfluoroalkyl and carbonyl units, Org. Lett., 2023, 25, 5314–5318 CrossRef CAS.
|
This journal is © Institute of Process Engineering of CAS 2024 |