DOI:
10.1039/D3MD00418J
(Research Article)
RSC Med. Chem., 2024,
15, 283-292
Discovery of new dibenzodiazepine derivatives as antibacterials against intracellular bacteria†
Received
18th August 2023
, Accepted 15th November 2023
First published on 11th December 2023
Abstract
The emergence and spread of multidrug-resistant bacteria underscore the critical need for novel antibacterial interventions. In our screening of 12 synthesized thienobenzodiazepines, pyridobenzodiazepines, and dibenzodiazepines, we successfully identified a small molecule compound SW33. Notably, SW33 demonstrated potent inhibitory activity against intracellular multidrug-resistant and fluoroquinolone-resistant strains of S. typhimurium in both macrophages and epithelial cells. Furthermore, SW33 was also effective against intramacrophagic Salmonella typhi, Yersinia enterocolitica, and Listeria monocytogenes. These significant findings suggest that SW33 possesses broad-spectrum activity against intracellular bacteria.
Introduction
Salmonella enterica is a Gram-negative facultative intracellular pathogen.1 Based on the differences in the somatic lipopolysaccharide antigen (O antigen), flagellar antigen (H antigen), and virulence antigen (Vi antigen), this species can be divided into more than 2600 serotypes.2 Moreover, S. enterica can be further classified into typhoidal and nontyphoidal groups based on differences in pathogenicity.3 In the United States and Europe, S. typhimurium is the second most prevalent nontyphoidal S. enterica serovar.4,5 Although S. typhimurium typically causes food poisoning in heathy individuals, it can also cause invasive infections, with consequences such as bacteraemia and sepsis, in immunocompromised patients and is responsible for 600
000 global infections annually with a mortality rate of up to 14.5%.6 With the increasing incidence of infections caused by multidrug-resistant (MDR) S. typhimurium strains, including those resistant to the second-line antibiotics ciprofloxacin and ofloxacin,7,8 the WHO has deemed antibiotic-resistant S. enterica as a significant priority for the development of new antibiotics.9
Tricyclic cores, such as dibenzoxazepine, pyridobenzodiazepine, and pyrrolobenzodiazepines, have been reported to exhibit antibacterial potential.10–12 Our previous study demonstrated that the antipsychotic drug loxapine (dibenzoxazepine-based tricyclic) can inhibit S. typhimurium infection in host cells, without affecting bacterial growth in medium.13 This finding suggests that loxapine might exert less selective pressure on bacteria and is less likely to induce antibiotic resistance than conventional antibiotics, making it an ideal candidate for further development. Therefore, we optimized the structure of loxapine and identified a novel compound, SW14, with potent inhibitory activity against intracellular S. typhimurium.11,14 However, the minimum nontoxic concentration of SW14 was only 5 μM, restricting its application in the prevention and treatment of Salmonella infections. Hence, we redesigned SW14 by substituting the dibenzoxazepine core with thienobenzodiazepine, pyridobenzodiazepine, or dibenzodiazepine cores. Through assessment of the antibacterial activity and cytotoxicity of the newly synthesized compounds, we discovered SW33, which exhibited antibacterial activity comparable to that of SW14 but showed no detectable toxicity in mammalian cells at concentrations up to 60 μM. Moreover, we evaluated the effects of SW33 against S. typhimurium in different types of phagocytic cells and epithelial cells, and investigated its effectiveness against other intracellular pathogens.
Results
Design, synthesis, and bioactivities of SW14 derivatives
In assessing the antibacterial activity of other antipsychotic drugs, we found that olanzapine, which has the same piperazine side chain as loxapine, exhibited antibacterial activity comparable to that of loxapine but had significantly lower cytotoxicity (Table 1). This finding raised a possibility that the replacement of the oxygen atom in the dibenzoxazepine core with a nitrogen atom may reduce its cytotoxicity. To examine this hypothesis, we designed three synthetic schemes (Schemes 1–3) for synthesizing three SW14 derivatives containing a diazepine ring, including SW41 (thienobenzodiazepine), SW30 (pyridobenzodiazepine), and SW44 (dibenzodiazepine) (Fig. 1A). We then analyzed SW14 and its derivatives against intracellular red fluorescence protein (RFP)-expressing Salmonella in infected murine RAW264.7 cells using image-based high-content analysis (HCA). Compared to SW14, SW41/30/44 exhibited slightly weaker antibacterial activities against intracellular Salmonella, but displayed dramatically lower cytotoxic effects on RAW264.7 cells (Table 1). These results proved our hypothesis that the diazepine ring is less toxic than the oxazepine ring in the tricyclic ring system (Fig. 1B, left).
Table 1 Structures, anti-intracellular S. typhimurium activities, and cytotoxicities of thienobenzodiazepine, pyridobenzodiazepine, or dibenzodiazepine derivatives. Cpd., compound; CIP, ciprofloxacin; EC50, the half-maximal effective concentration; CC50, the half-maximal cytotoxic concentration
|
Cpd. |
R group |
EC50 (μM) |
CC50 (μM) |
Selectivity ratio (CC50/EC50) |
S. typhimurium ATCC14028 |
RAW264.7 |
The assessment of loxapine and SW14 has been previously reported.11 The EC50 and CC50 data are presented as the means ± SDs (n = 3 replicates per group).
|
|
Loxapinea |
|
5.77 ± 1.84 |
110.07 ± 2.77 |
19.08 |
SW14a |
|
0.30 ± 0.09 |
22.50 ± 1.22 |
75.00 |
|
|
Olanzapine |
|
5.40 ± 1.77 |
198.30 ± 1.57 |
36.72 |
SW41 |
|
4.17 ± 1.00 |
102.66 ± 1.93 |
24.62 |
SW38 |
|
2.36 ± 1.52 |
38.85 ± 1.74 |
16.46 |
SW39 |
|
1.48 ± 0.21 |
126.24 ± 4.23 |
85.30 |
SW42 |
|
1.14 ± 0.24 |
76.78 ± 1.81 |
67.35 |
|
|
SW30 |
|
8.82 ± 0.06 |
123.06 ± 5.22 |
13.95 |
SW21 |
|
5.67 ± 0.97 |
61.90 ± 2.16 |
10.92 |
SW27 |
|
6.09 ± 0.67 |
159.23 ± 18.72 |
26.15 |
SW29 |
|
4.24 ± 0.64 |
100.91 ± 1.46 |
23.80 |
|
|
SW44 |
|
>30 |
128.14 ± 9.51 |
— |
SW35 |
|
3.70 ± 0.20 |
110.19 ± 3.25 |
29.78 |
SW45 |
|
15.15 ± 2.58 |
298.75 ± 8.34 |
19.72 |
SW33 |
|
0.40 ± 0.03 |
108.74 ± 1.90 |
271.85 |
|
|
CIP |
|
0.06 ± 0.02 |
— |
— |
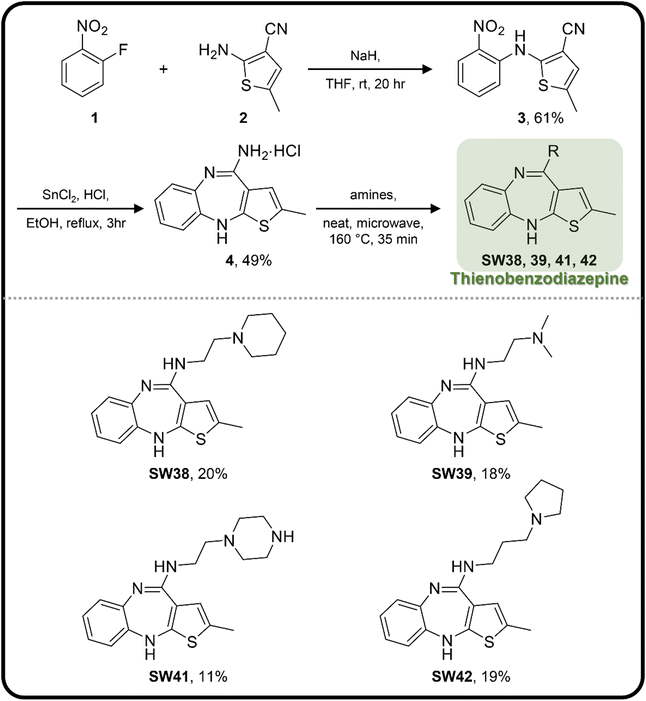 |
| Scheme 1 The synthetic scheme of the thienobenzodiazepine derivatives (SW38, 39, 41, and 42). The yield of compounds in each step is represented by percent values. | |
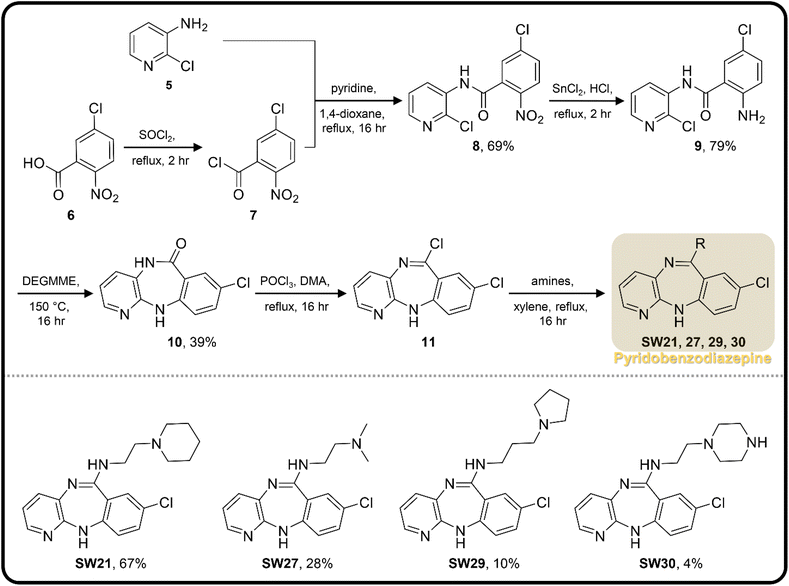 |
| Scheme 2 The synthetic scheme of the pyridobenzodiazepine derivatives (SW21, 27, 29, and 30). The yield of compounds in each step is represented by percent values. | |
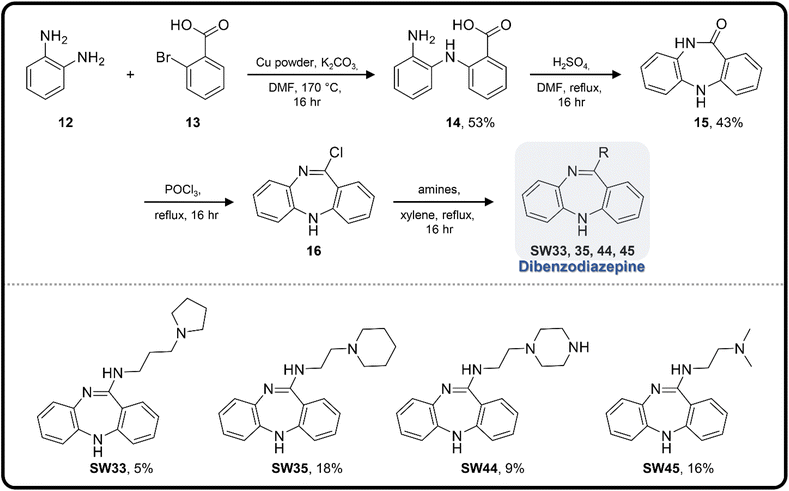 |
| Scheme 3 The synthetic scheme of the dibenzodiazepine derivatives (SW33, 35, 44, and 45). The yield of compounds in each step is represented by percent values. | |
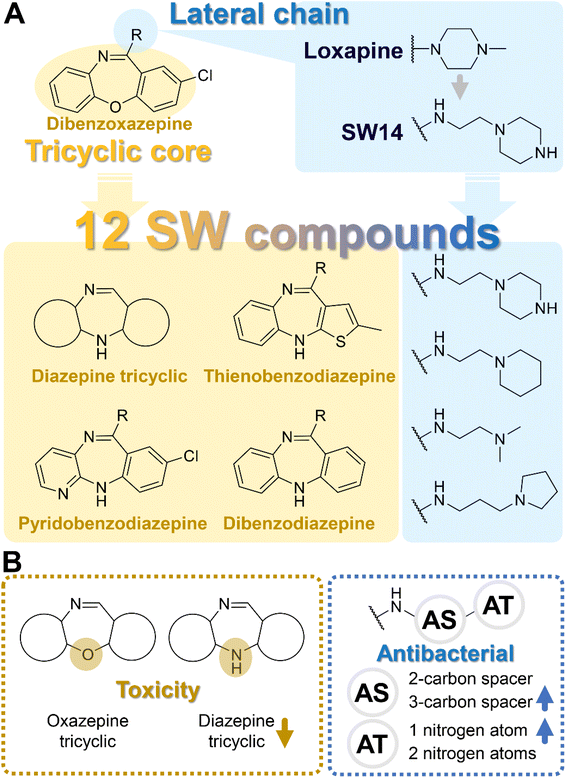 |
| Fig. 1 The building blocks and structure activity relationship (SAR) for SW compounds against Salmonella. (A) The lead compound, SW14 (structurally originated from loxapine), could be deconstructed into two components: the tricyclic core (circled in yellow) and lateral chain (circled in blue). The building blocks used for the tricyclic core and lateral chain are highlighted in yellow and blue rectangles, respectively. (B, left) In the SAR analysis of tricyclic cores, diazepine tricyclics demonstrated lower toxicity compared to oxazepane tricyclics. (B, right) In the SAR analysis of the lateral chain, the alkyl–aliphatic ring could be dissected into the alkyl spacer (AS) and aliphatic terminal (AT). For the AS, the 3-carbon spacer showed higher antibacterial potential than the 2-carbon spacer. For the AT, a single nitrogen atom within the terminal group exhibited greater potency in antibacterial activity compared to two nitrogen atoms. | |
In addition to the tricyclic core, the lateral chain (Fig. 1A, R group) also offers potential sites for optimization. Tricyclic dibenzoxazepine-based compounds have been identified with dual pharmacological activities, implying their potential application as antipsychotic and/or antibacterial agents.14,15 Since we aimed to develop antibacterial agents without antipsychotic activity, it is advisable to diminish the affinity to dopamine and serotonin receptors. This can be achieved by, for example, integrating an extended alkyl group combined with an aliphatic ring into the lateral chain.16 Such aliphatic rings containing one or two nitrogen atoms demonstrated the highest efficacy in combating Salmonella compared to aromatic rings and bulky aliphatic substituents lacking nitrogen atoms.14
To enhance the antibacterial efficacy while preserving the absence of antipsychotic effects in SW41, 30, and 44, we further designed two types of lateral chains and synthesized SW38, 21, 35, 39, 27, and 45. These lateral chains belong to the alkyl-aliphatic ring, each containing only one nitrogen atom within the aliphatic ring, such as piperidine and dimethylamino terminals (Table 1, Schemes 1–3, and Fig. 1A). The compound activity assessment revealed that such modifications made a slight improvement in antibacterial activity than its corresponding parent compounds (SW41, 30, and 44, with a piperazine terminal) (Table 1 and Fig. 1B).
The longer alkyl group on the lateral chain also enhanced the antibacterial activity against Salmonella,14 we then included different lengths of alkyl groups in the lateral chain design (Fig. 1A). SW42, 29, and 33 also possessed only one nitrogen atom at the aliphatic ring (pyrrolidine terminal) but with an elongated alkyl group (from a two-carbon to a three-carbon spacer). These three compounds demonstrated the lowest EC50 values of intracellular Salmonella and the highest selectivity ratio in the group of each core (Table 1). These results supported that one nitrogen atom terminal coupled with a longer (three-carbon) spacer is an ideal lateral chain to link to the thienobenzodiazepine, pyridobenzodiazepine, and dibenzodiazepine cores (Fig. 1B).
Among the tested compounds, SW33 exhibited antibacterial activity comparable to that of SW14 and had a significant reduction in cellular toxicity (Table 1). To validate the HCA results, we measured the inhibitory activity of SW33 on intracellular S. typhimurium using a colony-forming unit (CFU) assay to determine the number of viable intracellular bacteria. The results were consistent with those of the HCA experiment, showing that the number of intracellular S. typhimurium decreased with increasing concentrations of SW33, with a half-maximal effective concentration (EC50) similar to that observed in the HCA (Fig. 2A). This consistent result indicated that the inhibitory effect of SW33 on intracellular bacteria observed in the HCA was not due to interference with fluorescence signals of RFP. Moreover, we analyzed the toxicity of SW33 in RAW264.7 cells using a thiazolyl blue tetrazolium bromide (MTT) assay and found that SW33 exhibited no significant cytotoxicity even at concentrations as high as 60 μM (Fig. 2B).
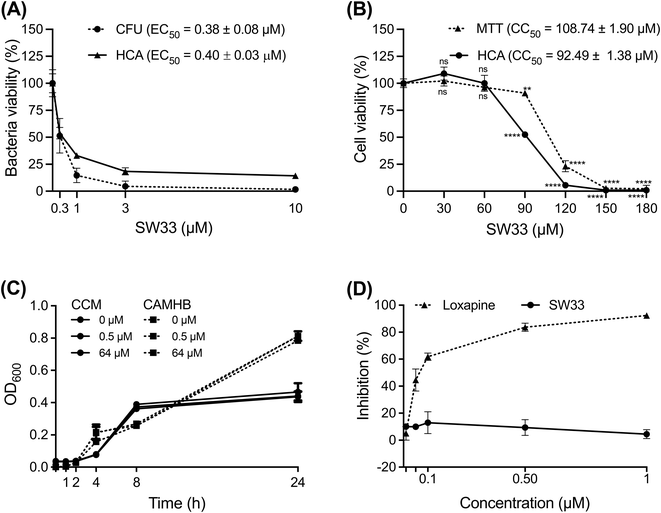 |
| Fig. 2 SW33 does not suppress Salmonella growth in broth and does not bind to recombinant D2Rs. (A) RAW264.7 cells were infected with S. typhimurium and then treated with various concentrations of SW33 in the presence of 20 mg L−1 gentamicin for 24 h. The viability of intracellular bacteria was assayed using HCA or a CFU assay. The data are expressed as percentages relative to the mock (DMSO)-treated control and are presented as the means ± SDs (n = 3 replicates per group). (B) The viability of RAW264.7 cells treated with increasing concentrations of SW33 was assessed using HCA or an MTT cell viability assay. The data are expressed as percentages relative to the mock (DMSO)-treated control and are presented as the means ± SDs (n = 3 replicates per group). ns, not significant; **, P < 0.01; ****, P < 0.0001 for the comparison of the cytotoxicity of SW33 at each concentration with that of the mock control. (C) The growth of S. typhimurium in CAMH broth (CAMHB) or cell culture medium (CCM) containing 0, 0.5, or 64 μM SW33 was monitored by measuring the OD600 of each bacterial culture at designated time points over a 24 h period. The data are presented as the means ± SDs (n = 3 replicates per group). (D) The binding of isotope-labelled spiperone to recombinant dopamine D2 receptors in the presence of different concentrations of loxapine or SW33 was assessed. The data are expressed as percentages of inhibition relative to the mock (DMSO)-treated control and are presented as the means ± SDs (n = 3 replicates per group). | |
Overall, these findings suggest that SW33 exhibits potent antibacterial activity against intracellular Salmonella while demonstrating minimal cytotoxicity and is thus a promising candidate for further investigation.
SW33 does not directly inhibit bacterial growth in medium, nor does it bind to dopamine D2 receptors (D2Rs)
To investigate the mechanism of action of SW33, we first tested its effect on the growth of S. typhimurium in cation-adjusted Mueller–Hinton broth (CAMHB), a nutrient-rich medium commonly used to assess bacterial antibiotic susceptibility. However, we observed no significant difference in bacterial growth, even at SW33 concentrations of up to 64 μM (Fig. 2C). We then further assessed the growth of S. typhimurium in the cell culture medium [Dulbecco's modified Eagle's medium (DMEM) containing 10% fetal bovine serum] of RAW264.7 cells and found no significant changes in bacterial growth with increasing concentrations of SW33 (Fig. 2C). These results suggest that the antibacterial activity of SW33 against intracellular bacteria is not mediated by direct inhibition of bacterial growth. Given that SW33 was derived from SW14, known for its capacity to suppress intracellular Salmonella via reducing bacterial resistance to host ROS stress,11 it was reasonable to speculate that SW33 might function similarly to SW14. Furthermore, structurally similar pyrrolobenzodiazepine tricyclic compounds selectively interacted with guanine bases within the minor groove of DNA,17,18 indicating that DNA binding might also be one of the possible mechanisms triggered by SW33.
Since both loxapine and olanzapine can bind D2Rs and exhibit clear activities against intracellular Salmonella, we aimed to investigate whether SW33 affects intracellular bacterial infection through interactions with D2Rs. To answer this question, we conducted a competitive inhibition assay using the antagonist spiperone and recombinant D2R to assess the binding activity of loxapine and SW33. As shown in Fig. 2D, SW33 showed no binding activity towards the recombinant D2R, unlike loxapine. This finding indicates that the antibacterial activity of SW33 against intracellular bacteria is not mediated by its interaction with the D2R.
Collectively, these results suggest that the mechanism of action by which SW33 inhibits intracellular bacterial infection is not mediated through direct bacterial growth inhibition or D2R interactions. Further studies are needed to elucidate the specific target and mode of action of SW33 in combating intracellular bacteria.
SW33 inhibits Salmonella infection of both phagocytic and nonphagocytic cells
During infection, Salmonella can invade and proliferate within intestinal epithelial cells and then infect and hijack macrophages to cause systemic infection.19 Thus, we investigated whether SW33 can inhibit S. typhimurium infection of other phagocytic cells and epithelial cells, and assessed its toxicity in these cells. Initially, we observed that the inhibitory effect of SW33 on intracellular Salmonella in another murine macrophage cell line, J774.1 (EC50 = 0.42 μM; Fig. 3A), was comparable to that in RAW264.7 cells (EC50 = 0.38 μM; Fig. 2A). Subsequently, we evaluated the susceptibility of intracellular Salmonella to SW33 in human THP-1 monocytes both with and without phorbol 12-myristate 13-acetate (PMA) treatment, which stimulates THP-1 cell differentiation into macrophage-like cells. Our results showed that Salmonella in macrophage-like THP-1 cells (EC50 = 0.52 μM; Fig. 3B) exhibited greater vulnerability to SW33 than Salmonella in monocytic THP-1 cells (EC50 = 1.02 μM; Fig. 3C).
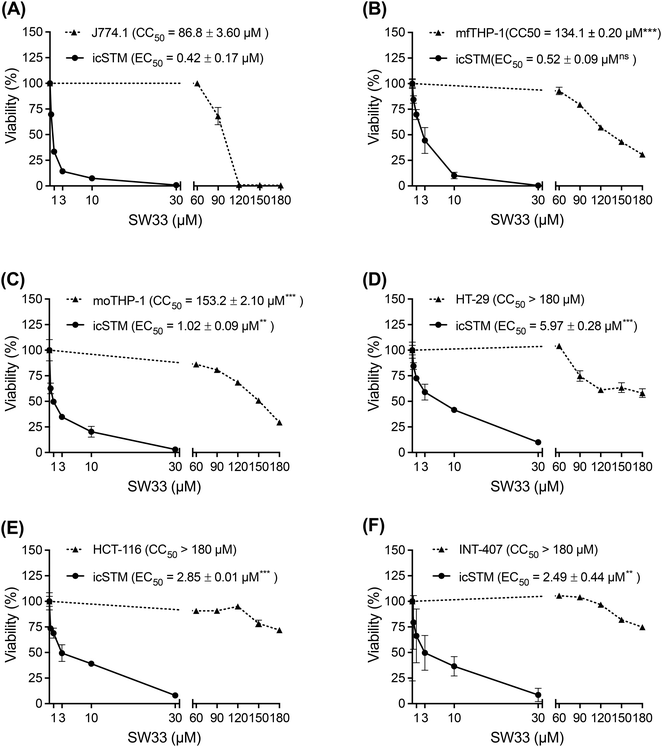 |
| Fig. 3 SW33 exhibits a stronger inhibitory effect on S. typhimurium infection in phagocytic cells than in epithelial cells. (A–F) Salmonella-infected J774.1 cells (A), macrophage-like THP-1 cells (B), monocytic THP-1 cells (C), HT-29 cells (D), HCT116 cells (E), and INT-407 cells (F) were treated with different concentrations of SW33 in combination with 20 mg L−1 gentamicin for 24 h. The survival of intracellular S. typhimurium (icSTM) was assessed using a CFU assay. Additionally, the viability of each cell line after exposure to SW33 combined with 20 mg L−1 gentamicin for 24 h was measured using an MTT cell viability assay. The data are expressed as percentages relative to the mock (DMSO)-treated control and are presented as the means ± SDs (n = 3 replicates per group). ns, not significant; **, P < 0.01; ***, P < 0.001; ****, P < 0.0001 for the comparison of the anti-intracellular Salmonella activity and cytotoxicity of SW33 in individual cell lines with those in RAW264.7 cells. | |
Next, we extended our investigation to Salmonella in human HT-29 and HCT-116 colon epithelial cells, as well as human INT-407 intestinal epithelial cells. Our data showed that while the EC50 values of SW33 against Salmonella in HT-29, HCT-116, and INT-407 cells were higher than those in macrophage-like THP-1 cells (5.97 μM, 2.85 μM, and 2.49 μM, compared to 0.52 μM; Fig. 3D–F and B, respectively), the cytotoxic effects of SW33 on these epithelial cells were attenuated. The half-maximal cytotoxic concentration (CC50) of SW33 in all epithelial cells was >180 μM but was 134.1 μM in macrophage-like THP-1 cells (Fig. 3D–F and B). Taken together, our data suggest that SW33 demonstrates greater activity against Salmonella in phagocytic cells.
SW33 is effective against MDR S. typhimurium
The increasing incidence of infections caused by antibiotic-resistant S. typhimurium strains constitutes a grave global public health concern.20 More than 40% of antibiotic-resistant S. typhimurium clinical isolates exhibit ampicillin, chloramphenicol, streptomycin, sulfamethoxazole, and tetracycline (ACSSuT) resistance phenotypes. Additionally, the emergence of ciprofloxacin-resistant clinical isolates has also been reported.21 To determine whether SW33 can overcome the resistance mechanisms of Salmonella, we acquired an ACSSuT-resistant strain (NL10) and a ciprofloxacin-resistant strain (CGC18) from the Taiwan Centers for Disease Control and assessed their susceptibility to conventional antibiotics. As shown in Table S1,† both strains exhibited resistance to multiple classes of antibiotics. Subsequently, we evaluated the inhibitory activity of SW33 against these two MDR Salmonella strains in RAW264.7 cells using a CFU assay. SW33 effectively inhibited both MDR-S. typhimurium strains in macrophages. However, its EC50 values (2.28 μM and 1.27 μM against NL10 and CGC18, respectively; Fig. 4A) against these strains were higher than that against the reference strain ATCC14028 (EC50 = 0.38 μM; Fig. 2A). These findings suggest that a specific mechanism of resistance of S. typhimurium to common antibiotics may reduce the antibacterial activity of SW33.
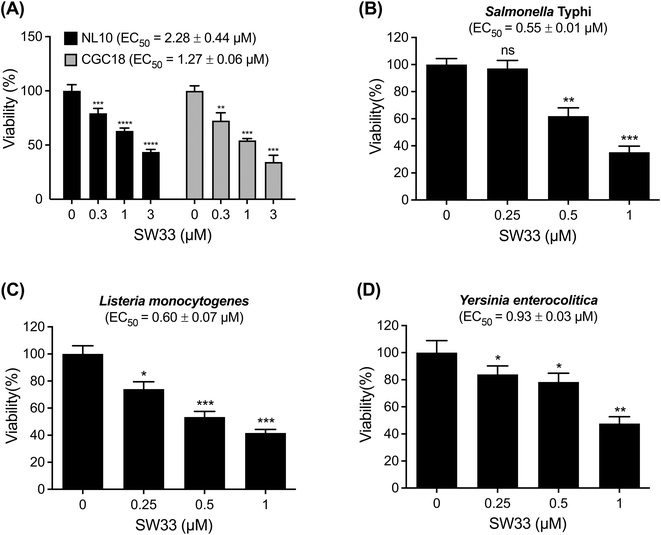 |
| Fig. 4 SW33 inhibits infection with MDR S. typhimurium and other pathogenic bacteria in macrophages. (A) RAW264.7 cells infected with the MDR isolate CGC18 or the ciprofloxacin-resistant isolate NL10 of S. typhimurium were treated with various concentrations of SW33 in combination with 20 mg L−1 gentamicin for 24 h. Intracellular bacterial survival was determined using a CFU assay, and the results are expressed as percentages relative to the mock (DMSO)-treated control. The data are presented as the means ± SDs (n = 3 replicates per group). **, P < 0.01; ***, P < 0.001; ****, P < 0.0001. (B–D) RAW264.7 cells infected with S. typhi (B), Y. enterocolitica (C), or L. monocytogenes (D) were treated with SW33 at concentrations ranging from 0 to 1 μM for 24 h. Subsequently, the number of viable intracellular bacteria was determined using a CFU assay. The data are presented as the means ± SDs (n = 3 replicates per group). ns, not significant; *, P < 0.05; **, P < 0.01; ***, P < 0.001. | |
SW33 demonstrates broad-spectrum effectiveness against other pathogenic bacteria in macrophages
In addition to S. typhimurium, numerous other pathogenic bacteria can infect macrophages and replicate within them.22 To investigate the efficacy of SW33 in controlling the infections caused by other pathogenic bacteria in macrophages, we treated RAW264.7 cells infected with S. typhi Ty2 (Fig. 4B), Listeria monocytogenes (Fig. 4C), or Yersinia enterocolitica (Fig. 4D) with various concentrations of SW33 for 24 h. Subsequently, we determined the number of intracellular bacteria using a CFU assay and found that SW33 exhibited inhibitory effects against all tested bacteria in RAW264.7 cells, with EC50 values of 0.55, 0.60, and 0.93 μM against S. typhi Ty2, L. monocytogenes, and Y. enterocolitica, respectively. Therefore, SW33 can be considered as a broad-spectrum antibacterial agent that can effectively combat different pathogenic bacteria in macrophages.
Conclusions
Due to inappropriate antibiotic use, the spread and prevalence of antibiotic-resistant S. typhimurium is increasing, constituting a significant global healthcare concern. However, the development of new antibiotic classes is slow, and the number of new antibiotics is almost stagnant. Consequently, there is an urgent need for novel therapeutic strategies to control Salmonella infections. In this study, we focused on optimizing the structure of SW14, a loxapine derivative, to reduce its cytotoxicity while maintaining its efficacy against intracellular S. typhimurium. Our lead compound SW33 demonstrates potent activity not only against intracellular MDR S. typhimurium but also against other intracellular pathogenic bacteria. Interestingly, SW33 does not directly inhibit bacterial growth in culture medium, suggesting that its antibacterial mechanism might target host cell factors or bacterial virulence factors, thereby reducing the likelihood of bacterial resistance development. Therefore, SW33 has the potential for development as a promising new type of antibacterial agent.
Author contributions
LHC and MYL contributed equally to this work. JCS, CWS, FWY, HWL, and MYL designed and synthesized all the compounds. LHC and HCL performed the biological studies. JCS and HCC conceptualized the work, designed the experiments, and supervised the whole project. The manuscript was written with the contributions of all the authors. All the authors have approved the final version of the manuscript.
Conflicts of interest
There are no conflicts to declare.
Acknowledgements
This work was supported by the National Science and Technology Council, Taiwan (grant numbers: NSTC 111-2628-B-002-015, NSTC 111-2628-B-A49-010, and NSTC 112-2320-B-A49-023) and National Taiwan University (grant numbers: 112L7719 and 112L7819).
References
- L. A. Knodler and J. R. Elfenbein, Salmonella enterica, Trends Microbiol., 2020, 28(1), 83 CrossRef CAS PubMed
.
- R. A. Cheng, C. R. Eade and M. Wiedmann, Embracing Diversity: Differences in Virulence Mechanisms, Disease Severity, and Host Adaptations Contribute to the Success of Nontyphoidal Salmonella as a Foodborne Pathogen, Front. Microbiol., 2019, 10, 1368 CrossRef PubMed
.
- N. A. Feasey,
et al., Invasive non-typhoidal salmonella disease: an emerging and neglected tropical disease in Africa, Lancet, 2012, 379(9835), 2489–2499 CrossRef PubMed
.
-
CDC, National Enteric Disease Surveillance: Salmonella Annual Report, 2016, 2018 Search PubMed
.
- European Food Safety Authority and European Centre for Disease Prevention and Control (EFSA and ECDC), The European Union summary report on trends and sources of zoonoses, zoonotic agents and food-borne outbreaks in 2017, EFSA J., 2018, 16(12), e05500 Search PubMed
.
- Collaborators, G.B.D.N.-T.S.I.D., The global burden of non-typhoidal salmonella invasive disease: a systematic analysis for the Global Burden of Disease Study 2017, Lancet Infect. Dis., 2019, 19(12), 1312–1324 CrossRef
.
- J. A. Crump,
et al., Epidemiology, Clinical Presentation, Laboratory Diagnosis, Antimicrobial Resistance, and Antimicrobial Management of Invasive Salmonella Infections, Clin. Microbiol. Rev., 2015, 28(4), 901–937 CrossRef CAS
.
- F. C. Fang, Fluoroquinolone Resistance in Salmonella and the Utility of Perfloxacin Disk Diffusion, J. Clin. Microbiol., 2015, 53(11), 3401–3404 CrossRef CAS PubMed
.
- E. Tacconelli,
et al., Discovery, research, and development of new antibiotics: the WHO priority list of antibiotic-resistant bacteria and tuberculosis, Lancet Infect. Dis., 2018, 18(3), 318–327 CrossRef PubMed
.
- P. Andriollo,
et al., C8-Linked Pyrrolobenzodiazepine Monomers with Inverted Building Blocks Show Selective Activity against Multidrug Resistant Gram-Positive Bacteria, ACS Infect. Dis., 2018, 4(2), 158–174 CrossRef CAS PubMed
.
- C. Y. Hsu,
et al., A Novel Dibenzoxazepine Attenuates Intracellular Salmonella Typhimurium Oxidative Stress Resistance, Microbiol. Spectrum, 2021, 9(3), e0151921 CrossRef PubMed
.
- W. Seebacher,
et al., Synthesis of new pyrido-benzodiazepine salts and their antimicrobial activities, Monatsh. Chem., 2017, 148, 263–274 CrossRef CAS
.
- C. Y. Yang,
et al., Loxapine, an antipsychotic drug, suppresses intracellular multiple-antibiotic-resistant Salmonella enterica serovar Typhimurium in macrophages, J. Microbiol., Immunol. Infect., 2019, 52(4), 638–647 CrossRef CAS PubMed
.
- H. C. Lin,
et al., Discovery of antipsychotic loxapine derivatives against intracellular multidrug-resistant bacteria, RSC Med. Chem., 2022, 13(11), 1361–1366 RSC
.
- E. Hjerde, S. G. Dahl and I. Sylte, Atypical and typical antipsychotic drug interactions with the dopamine D2 receptor, Eur. J. Med. Chem., 2005, 40(2), 185–194 CrossRef CAS PubMed
.
- J. F. Liegeois,
et al., New pyridobenzodiazepine derivatives: modifications of the basic side chain differentially modulate binding to dopamine (D(4.2), D(2L)) and serotonin (5-HT(2A)) receptors, J. Med. Chem., 2002, 45(23), 5136–5149 CrossRef CAS PubMed
.
- D. Antonow and D. E. Thurston, Synthesis of DNA-interactive pyrrolo[2,1-c][1,4]benzodiazepines (PBDs), Chem. Rev., 2011, 111(4), 2815–2864 CrossRef CAS
.
- P. Picconi,
et al., Covalent DNA Binding Is Essential for Gram-Negative Antibacterial Activity of Broad Spectrum Pyrrolobenzodiazepines, Antibiotics, 2022, 11(12), 1770 CrossRef CAS PubMed
.
- J. E. Galan, Salmonella Typhimurium and inflammation: a pathogen-centric affair, Nat. Rev. Microbiol., 2021, 19(11), 716–725 CrossRef CAS PubMed
.
- P. F. McDermott, S. Zhao and H. Tate, Antimicrobial Resistance in Nontyphoidal Salmonella, Microbiol. Spectrum, 2018, 6(4) DOI:10.1128/microbiolspec.ARBA-0014-2017
.
- W. T. Tiew,
et al., Emergence and spread in Taiwan of multidrug-resistant serotypes of nontyphoidal Salmonella, Infection, 2022, 50(2), 475–482 CrossRef CAS PubMed
.
- L. Diacovich and J. P. Gorvel, Bacterial manipulation of innate immunity to promote infection, Nat. Rev. Microbiol., 2010, 8(2), 117–128 CrossRef CAS PubMed
.
|
This journal is © The Royal Society of Chemistry 2024 |
Click here to see how this site uses Cookies. View our privacy policy here.