DOI:
10.1039/D4NH00187G
(Communication)
Nanoscale Horiz., 2024,
9, 1301-1310
Fabrication of innovative multifunctional dye using MXene nanosheets†
Received
30th April 2024
, Accepted 7th May 2024
First published on 29th May 2024
Abstract
The increasing demand for natural and safer alternatives to traditional hair dyes has led to the investigation of nanomaterials as potential candidates for hair coloring applications. MXene nanosheets have emerged as a promising alternative in this context due to their unique optical and electronic properties. In this study, we aimed to evaluate the potential of Ti3C2Tx (Tx = –O, –OH, –F, etc.) MXene nanosheets as a hair dye. MXene nanosheet-based dyes have been demonstrated to exhibit not only coloring capabilities but also additional properties such as antistatic properties, heat dissipation, and electromagnetic wave shielding. Additionally, surface modification of MXene using collagen reduces the surface roughness of hair and upregulates keratinocyte markers KRT5 and KRT14, demonstrating the potential for tuning its physicochemical and biological properties. This conceptual advancement highlights the potential of MXene nanosheets to go beyond simple cosmetic improvements and provide improved comfort and safety by preventing the presence of hazardous ingredients and solvents while providing versatility.
New concepts
This study introduces a new concept to the field of hair coloring by exploring the unprecedented potential of MXene nanosheets as a groundbreaking alternative to traditional dyes. As the demand for natural and safe hair dyes increases, research on nanomaterials is actively underway, and MXene, a two-dimensional material with unique optical, electrical, and mechanical properties, is emerging as a promising material. Our new concept is to leverage the unique advantages of MXene nanosheets for hair coloring applications. Unlike conventional dyes that contain potentially harmful chemicals, MXene-based nanosheet dyes are safe, impart natural color to hair, and can impart several properties, including anti-static properties, heat dissipation properties, and electromagnetic wave shielding properties. Additionally, surface modification of MXene with collagen showed a reduction in hair roughness and upregulation of proteins (KRT5 and KRT14). These results demonstrate that physicochemical and biological properties can be tuned through surface modification. This conceptual advancement not only changes the cosmetic performance of hair dyes, but also improves comfort and safety by eliminating harmful ingredients and solvents and giving multifunction. This discovery highlights the potential of MXene nanosheets to revolutionize dyeing.
|
1. Introduction
Hair coloring is a common beauty practice that alters hair color to enhance appearance. The demand for dark and black hair dyes has surged as the international population ages. Hair color depends on the presence and amount of melanin pigment, which can cause grey or white hair over time.1–4 Hair dyes permanently alter color through chemical reactions under alkaline conditions. This process typically involves using a base such as ammonia to open the hair cuticles, enabling colorants such as para-phenylenediamine (PPD) and para-aminophenol (PAP) to permeate the hair.5 An oxidant, such as hydrogen peroxide, is also essential for bleaching natural melanin pigments and forming the final dye compounds.6 This process can span several hours and is limited to diffusion. However, many ingredients used in this process are toxic, with some being carcinogenic.7–9 These substances can lead to severe consequences or allergic reactions if absorbed through the skin or inhaled. In particular, an alkaline pH can increase the negative charge on hair, resulting in increased friction between fiber surfaces. This phenomenon can lead to increased hair frizz and damage to the cuticle layer.10 Traditional hair dyes frequently contain harsh chemicals that can harm the hair and scalp, prompting a growing demand for safer, more natural alternatives. Previously, hair dyes employed black ultrafine particles such as lead(II) sulfide (PbS) nanoparticles.
Moreover, in ancient times, lead salts were used to darken hair.11 Carbon nanotubes have also been used in hair dyes. However, this approach has limitations.12 In comparison, natural hair dyes, mainly obtained from plants, can safely dye hair without chemical additives. However, it is not cost-effective due to its dependence on complex extraction/purification procedures, long cultivation cycles, and limited harvest periods, as well as poor hair dyeing ability and resource limitations.13 The ideal dye solution employs a material capable of forming a uniform, thin hair coating that can endure brushing and washing. Recently, inorganic nanomaterials have emerged as promising alternatives for hair dyeing owing to their distinctive optical and electronic properties. Among these nanomaterials, MXenes are noteworthy for their unique properties.
MXenes are two-dimensional (2D) planar structures comprising transition metal carbides, nitrides, and carbonitrides with excellent electrical, optical, and mechanical properties. Owing to their unique physicochemical characteristics, MXene nanosheets have been investigated for various applications, including energy storage, sensing, and catalysis.14–16 Typically, MXene is expressed by the formula Mn+1XnTx where M represents an early transition metal (e.g. Ti, Nb, Zr, Ta, Hf, V, Sc, Cr, and Mo) and X represents carbon or nitrogen. Tx represents the end groups (O, OH, F, and Cl) bound to the outer M layer. This MXene has a hexagonal structure and exhibits unique optical properties such as excellent electrical conductivity, adjustable band gap, and high optical transparency.17,18 In particular, Ti3C2Tx MXene absorbs light strongly in the visible light region and exhibits dark colors. In addition, the Ti3C2Tx MXene surface is hydrophilic, so it can adhere to various surfaces and form a smooth coating, and its two-dimensional geometric structure and high surface area provide superior film forming ability and durability compared to carbon-based particles.19 Due to these properties, MXene-based nanosheet dyes can be formulated in water without harmful ingredients or solvents owing to the presence of hydrophilic functional groups on their surfaces. When applied to hair through spraying or brushing, they can express colors ranging from gray to pitch black, depending on the MXene concentration. These MXene-based nanosheet dyes can produce highly durable coatings that imbue hair with novel optical, electrical, thermal, and biological properties, thereby enhancing comfort, cosmetic performance, and safety.
Additionally, MXene can improve its physicochemical and biological properties through surface modification with various natural and synthetic polymers. We demonstrate that improved physicochemical and biological properties of MXene-based nanosheet dyes can be achieved through surface modification of Ti3C2Tx MXene using collagen.
This study focuses on the potential application of MXene nanosheets as hair dyes. We aimed to provide a comprehensive overview of the current research on MXene nanosheets as hair dyes and address their characterization and applications. Furthermore, we discuss the challenges and future directions for the development of MXene nanosheets as safe and effective alternatives to conventional hair dyes.
2. Results
2.1 Characterization of MXene, MXene-based aqueous black hair dye, and MXene-coated hair
Transition metal carbide MXene, Ti3C2Tx (Tx = –O, –OH, –F, etc.), is highly desirable in bioengineering owing to its outstanding electrical and thermal conductivity and biocompatibility.14,20,21 Scanning electron microscope (SEM) images reveal the two-dimensional planar and layered structures of MXene flakes (Fig. 1(a) and Fig. S1a, ESI†). Furthermore, the distinctive peaks of MXene and energy-dispersive spectrometer (EDS) analysis show the distribution of carbon (C), titanium (Ti), chlorine (Cl), fluorine (F), and oxygen (O) elements (Fig. 1(b)). The XRD pattern of Ti3C2Tx MXene exclusively comprises characteristic (002) peaks and rules out the presence of residual MAX phase or other impurities in the as-synthesized MXene (Fig. 1(c)). The Raman spectrum was measured under 785 nm laser excitation (Fig. S1b, ESI†). Raman modes observed at ∼118 cm−1 (Eg(Ti, C, O)) and ∼196 cm−1 (A1g(T, C, O)) can be attributed to the in-plane and out-of-plane vibrations of the entire MXene atomic structure (Ti, C, and surface terminations), respectively. Furthermore, Raman modes at ∼289 cm−1 (Eg(Tx = OH)) and ∼368 cm−1 (Eg(Tx = O)) are exclusively associated with the surface functional groups of MXene. Meanwhile, Raman modes at ∼606 cm−1 (Eg(C)) and ∼718 cm−1 (A1g(C)) were assigned to the in-plane and out-of-plane vibrations of carbon atoms within the MXene lattice, respectively.
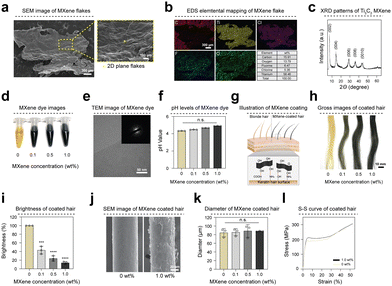 |
| Fig. 1 Characterization of MXene and MXene-based hair dyeing and physical properties. (a) SEM image showing the surface of the MXene flake. (b) EDS elemental mapping (C, Ti, Cl, F, O) image of MXene flakes. (c) XRD pattern of the MXene flake. (d) Gross images of MXene-based hair dyes containing chitosan and L-ascorbic acid. (e) The TEM image of the MXene hair dye shows the smooth and clean surface of the MXene nanosheet, and the SAED pattern of the MXene dye shows the hexagonal symmetry of the nanosheet. (f) pH of the MXene dye measured with a pH meter. (g) Simplified schematic diagram of hair coating using MXene-based hair dyes. (h) Gross images of hair coated with MXene dye at various concentrations (0–1.0 wt%). (i) Brightness of hair coated with MXene dye. (j) SEM images of hair (0 wt%) (left) and hair coated with MXene dye (1.0 wt%) (right). (k) Diameter of hair coated with MXene dye at various concentrations. (l) Stress–strain curves of uncoated hair (0 wt%) and hair coated with MXene dye (1.0 wt%). All data represent mean ± SD (n = 3). ***p < 0.001, ****p < 0.0001 The symbol * indicates comparisons with 0 wt%. Scale bars = 300 μm (a, b), 100 μm (magnified images, a), 50 nm (e), 10 mm (h), 20 μm (j). | |
The MXene-based dye used in this study comprised various concentrations, incorporating additives such as L-ascorbic acid (vitamin C) and chitosan in a 1
:
1 ratio. L-Ascorbic acid nourishes hair and can be dissolved in solution. The chitosan exhibits excellent biocompatibility and enhances hair surface adhesion, ensuring a uniform coating. MXene dye was obtained in a consistently dispersed state through sonication for 30 minutes (Fig. 1(d)). Transmission electron microscopy (TEM) images reveal the smooth and transparent surfaces of the MXene nanosheets. The corresponding selected area electron diffraction (SAED) patterns confirm the hexagonal symmetry of these nanosheets (Fig. 1(e) and Fig. S1c, ESI†). The MXene nanosheets to exhibit weak acidic characteristics (pH 4.0–5.0), causing the hair cuticle layer to shrink and resulting in visibly tighter and firmer (Fig. 1(f)). Fig. 1(g) shows schematic diagrams of blonde and MXene-coated hair. The MXene surface functional groups (F, O, OH–) contained in the MXene dye facilitated the coating of the keratin hair surface with an amine (NH2) and a carboxyl group (COOH–). Consequently, hair coated with MXene dye exhibited a black shade depending on the MXene concentration (Fig. 1(h)). To compare the brightness of hair coated with dye, we measured the RGB values of the hair. RGB average values of 166.89 and 23.11 were obtained for hair coated at 0 wt% and 1.0 wt% concentrations, respectively. The brightness of coated hair was quantified as a relative value using RGB values. The brightness of MXene-coated hair group decreased by 86.19% compared with that of the 0 wt% group at this concentration (Fig. 1(i)). SEM and microscopic images of hair samples show that the MXene dye was uniformly coated on the hair surface without aggregation (Fig. 1(j) and Fig. S1d, e, ESI†). Furthermore, EDS mapping analysis of the MXene-coated hair (1.0 wt%) indicates an even distribution of MXene (Fig. S1f, ESI†). The thickness of the hair coating layer after applying MXene dye coating was approximately 2.9 μm (Fig. 1(k)) compared to the control group. Moreover, no significant difference (yield strength: 191.22 MPa for the control and 212.77 MPa for the coated group) in tensile strength was observed between 0 wt% and the MXene-coated hair group (Fig. 1(l) and Fig. S1g, ESI†).
2.2 The multifunctional performance of MXene-coated hair
Hair is highly susceptible to static electricity owing to its large friction area, particularly on dry days, which leads to discomfort and a flyaway effect on hair.22,23 MXene-based coatings, with exceptional electrical conductivity, effectively and immediately dissipate static electricity in hair. We induced static electricity in hair samples to assess their antistatic properties by rubbing them with a rubber balloon ten times (Fig. 2(a)). The surface potential of each hair sample was measured using a static meter. Hair not coated with MXene dye exhibited an average surface potential of 6.6 kV against the induced static electricity from friction. In contrast, hair coated with MXene dye exhibited surface potentials of approximately 3.96, 1.13, and 0.7 kV, respectively. The surface potential decreased as the MXene concentration increased (Fig. 2(b)). Furthermore, it exhibited antistatic properties even on hair coated with low concentrations of the MXene dye (Fig. S2a and b, ESI†). The high electrical conductivity of MXenes imparts antistatic properties to hair.
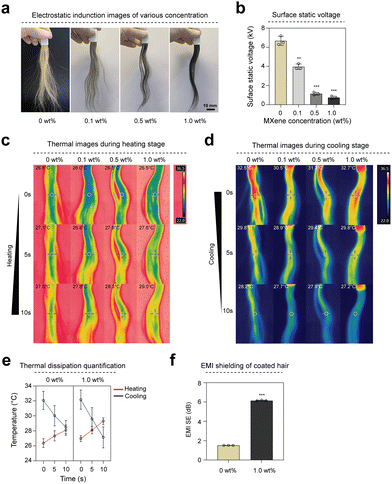 |
| Fig. 2 Multifunctional properties of MXene-coated hair. (a) Electrostatically induced gross images of hair coated with MXene dye at various concentrations. (b) Quantitative graph of the surface potential of hair coated with MXene dye at various concentrations induced by electrostatic force. (c), (d) Thermal dissipation characteristic gross images of hair coated with MXene dye at various concentrations captured by an IR camera. (e) Quantitative graph of thermal dissipation properties of hair coated with MXene dye at various concentrations. (f) EMI shielding performance of uncoated hair (0 wt%) samples and hair coated with MXene dye (1.0 wt%). All data represent mean ± SD (n = 3). **p < 0.01, ***p < 0.001, The symbol * indicates comparisons with 0 wt%. Scale bars = 10 mm (a). | |
Hair plays a pivotal role in the complex biological system regulating body temperature.24 Improving the thermal conductivity of hair through MXene coating enhances the rapid heat dissipation from the head, thereby increasing comfort. We conducted heating and cooling tests at various rates to compare the thermal dissipation properties of hair coated with 1.0 wt% and 0 wt% groups. Initially, we brought hair samples into contact with a hot plate set to the human body temperature of 36.5 °C. Images were captured using an infrared (IR) camera during heating (Fig. 2(c)). Hair coated with MXene dye exhibited a temperature increase that was 2 °C faster than the control group within 5 s of heating. Next, we preheated hair samples to 36.5 °C and removed them from the hot plate to measure the cooling rate (Fig. 2(d)). The IR camera captured images revealing that hair coated with MXene dye dissipated heat 2 °C faster than the control group within 5 s (Fig. 2(e)). This temperature change is sufficiently significant to be perceived by the skin, and the rapid heat dissipation prevents local heat accumulation on the skin, thereby increasing comfort and decreasing hair damage. Moreover, in the MXene dye-coated hair (1.0 wt%), the electromagnetic interference (EMI) shielding was 2.5 times higher than that of uncoated hair (0 wt%) (0 wt%: 1.82 dB and 1.0 wt%: 5.1 dB) because of the excellent electrical conductivity of MXene (Fig. 2(f) and Table S1, ESI†).
2.3 The durability of MXene-coated hair dye
The abundant –O, –F, and –OH groups on the MXene surface interacted with the –NH2 and –OH groups of chitosan via hydrogen bonding.25,26 MXene hair dye incorporates chitosan, which possesses high surface adhesion and adheres to the hair surface during the coating process, making it resistant to washing. Additionally, the amino group within the chitosan molecule becomes a cation under acidic conditions, whereas the hair surface becomes an anion under the same conditions. This results in ion interactions that lead to the adsorption of chitosan onto the hair surfaces. Cross-sectional SEM images and EDS mapping analysis of hair show that dyeing with MXene nanosheets did not damage the cuticle layer, did not penetrate the hair, and homogeneously coated the hair surface (Fig. 3(a) and Fig. S3a, ESI†). The MXene dye-coated hair samples were cleaned using shampoo to evaluate the sustainability of the MXene dye coating. The coated hair retained its color even after 30 washes (Fig. 3(b)). The brightness of hair was quantified by measuring the average RGB values before and after washing (Fig. 3(c)). Additionally, accelerated life test (85/85 test) results demonstrate that the color of coated hair was consistently maintained for 5 days (Fig. S3b, ESI†). These results indicate that the MXene dye effectively covered the hair surface and retained its color for an extended period.
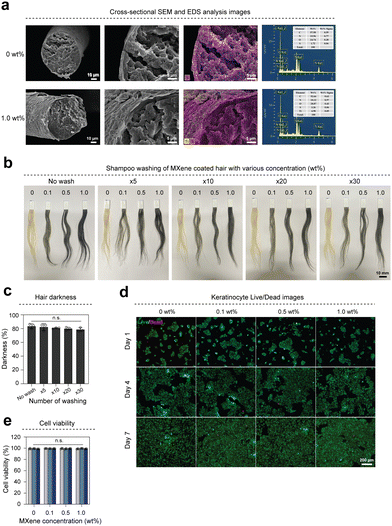 |
| Fig. 3 Evaluation of coating properties and durability of MXene dye and cytotoxicity analysis. (a) Cross-sectional SEM and EDS analysis images of uncoated hair (0 wt%) samples and hair coated with MXene dye (1.0 wt%). (b) Gross images of hair coated with MXene dye (1.0 wt%) before and after shampoo washing. (c) Hair brightness of hair coated with MXene dye (1.0 wt%) after shampoo washing. (d) Live/dead images of HaCaT cells cultured with hair coated with various concentrations of MXene dye (0 wt%, 0.1 wt%, 0.5 wt%, and 1.0 wt%) on 1, 4, and day 7, respectively, were obtained at Transwell®. Green (calcein–AM) indicates live cells, and red (Ethidium homodimer-1) indicates dead cells. (e) Quantification of cell viability obtained from live/dead analysis. Scale bars = 10 μm (a, left), 5 μm (a, middle and right), 10 mm (b), 200 μm (d). All data represent mean ± SD (n = 3). | |
Owing to its biological characteristics, such as its ability to promote cell proliferation and antimicrobial effects, MXenes have garnered considerable interest in biology.27,28 However, several studies have reported the potential cytotoxicity of MXene.29 To confirm the biocompatibility of hair coated with MXene dye, we evaluated the cell viability of HaCaT (human, adult, low calcium, high temperature) cells using a live/dead kit (Fig. 3(d)). The survival rate of HaCaT cells remained above 98% until D7 (Fig. 3(e)).
2.4 Comparison of MXene dye and commercial products
Traditional hair dyes incorporate chemical ingredients to permeate the hair and alter its pigmentation, which may lead to various health problems, including allergic reactions, skin irritation, and an increased risk of certain types of cancer.30,31 To determine whether MXene-based dyes without chemical additives can replace dyes containing various chemical components for hair dyeing, blonde hair samples were dyed with MXene dye (1.0 wt%), commercial dye product (C1), and colored shampoo (C2) (Fig. 4(a)). Hair coated with MXene dye (1.0 wt%) and commercial dye (C1) exhibited brightness values of 17.3% and 10.6%, respectively. The brightness difference between the colored shampoo (C2) and the MXene dye (1.0 wt%) was 49.6% (Fig. 4(b)). To compare the multifunctional characteristics of MXene dyes with those of various commercial dye products, their antistatic properties were confirmed by inducing static electricity on hair samples coated with different dyes (Fig. 4(c)). The surface potential of the electrostatically induced hair was measured. The surface potential of the hair covered with the MXene dye was 0.73 kV, whereas that of the commercial products C1 and C2 was 4.8 and 5.9 kV, respectively. Hair coated with the MXene dye (1.0 wt%) exhibited the lowest surface potential (Fig. 4(d)). To compare the thermal properties of hair coated with MXene dye, the heat dispersion characteristics of hair coated with commercial dye and colored shampoo were examined (Fig. 4(e)). The difference in temperature change between the MXene dye (1.0 wt%) and commercial dye (C1) was 2 °C, indicating a higher rate of temperature change in hair coated with MXene dye (Fig. 4(f)). In addition, hair with MXene dye had approximately six times higher EMI shielding performance (coated: 5.1 dB, C1: 0.8 dB) than the commercial dye product C1 (Fig. 4(g)).
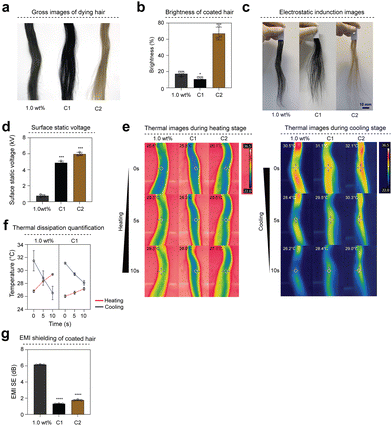 |
| Fig. 4 Comparison of MXene dyes and commercialized hair dyes. (a) Gross image of hair coated with various dyes Hair coated with MXene dye (left), commercial hair dye 1 (middle), and commercial hair dye 2 (right). (b) Brightness of hair coated with various dyes. (c) Electrostatically induced gross image of hair coated with various dyes. (d) Surface potential of hair coated with various dyes induced by electrostatic forces. (e) Gross image of heat dissipation properties of hair coated with various dyes. (f) Quantification graph of heat dissipation properties of hair coated with various dyes. (g) EMI shielding performance of hair coated with various dyes. All data represent mean ± SD (n = 3). *p < 0.05, ***p < 0.001, ****p < 0.0001 The symbol * indicates comparisons with 1.0 wt%. Scale bars = 10 mm (c). | |
2.5 Collagen-modified MXene dye (MXene@Col) characteristics
Type 1 Collagen is the most abundant protein in the human body and exhibits excellent biocompatibility and bioactivity.32 Collagen can also improve the radiance of nails, hair, and skin. In particular, collagen structurally resembles keratin, a hair component that strengthens hair texture. To modify the MXene surface with collagen, the positively charged collagen was ionically bonded to the negatively charged MXene surface in a slightly acidic environment.33Fig. 5(a) shows a schematic diagram of the MXene surface and collagen interactions. Successful modification of the MXene surface with collagen was demonstrated by X-ray photoelectron spectroscopy (XPS) measurements (Fig. 5(b)). The survey scan spectra of the MXene and MXene@Col dyes exhibited Ti 2p, C 1s, O 1s, F 1s, and Cl 2p peaks, which are typically observed for MXene (Fig. S4a and b, ESI†). However, the MXene@Col dye exclusively exhibited an N 1s peak originating from collagen. Furthermore, the chemical bonds of collagen resulted in significant changes in the high-resolution C 1s spectra of the MXene@Col dye compared to those of the MXene dye (1.0 wt%). The C 1s spectrum of the MXene dye can be deconvoluted into five peaks corresponding to C–Ti, C–O–Ti, C–C, C–O, and O–C–O bonds. In addition to the enhanced C–O peak, the C 1s spectrum of the MXene@Col dye contains new peaks corresponding to the C–N and N–C
O bonds of collagen (Fig. 5(c)). Moreover, to confirm the surface modification of collagen, the zeta potential of each group (0 wt%, 1.0 wt%, MXene@Col) was measured (Fig. 5(d) and Table S2, ESI†). MXenes exhibit a negative charge because of their abundant –OH groups. Consequently, the hair surface coated with MXene dye exhibited a negative zeta potential of −21.97 mV. Meanwhile, collagen has a positive charge; therefore, MXene@Col comprises MXene surface-modified with collagen. The zeta potential of hair coated with MXene@Col dye was −7.81 mV, higher than that of hair coated with MXene dye. As previously described, an evenly dispersed MXene@Col dye was produced using MXene modified with collagen (Fig. S5a, ESI†). The TEM image of the fabricated MXene@Col dye reveals the clean surface of the MXene@Col nanosheet. The SAED pattern shows the hexagonal symmetry of the nanosheet (Fig. S5b, ESI†). Hair samples were coated with the dye prepared using collagen surface-modified MXene (Fig. 5(e)). The brightness of hair coated with MXene@Col dye was nearly identical to that of hair coated with MXene dye (1.0 wt%), with a difference of approximately 4.8% in darkness (Fig. 5(f)). Additionally, the color properties of 0 wt% and 1.0 wt% or MXene@Col groups were examined based on changes in the Commission internationale de l’éclairage (CIE) L*a*b* values. The 0 wt% group was blonde, with L*, a*, and b* values of 76.45, 3.02, and 25.36, respectively. However, after MXene dye (1.0 wt%) and MXene@Col dye coating, the hair color changed to black (Fig. S5c and Table S3, ESI†). The SEM images and EDS mapping analysis show that the hair surface was smoothly coated with the MXene@Col dye (Fig. 5(g) and Fig. S5d, ESI†). To determine whether collagen affects hair roughness, we measured the surface roughness of hair coated with the MXene@Col dye using a 3D Optical Profiler. Hair coated with MXene@Col dye had multifunctional properties (Fig. S5e and f, ESI†) and lower surface roughness than hair coated with MXene dye without surface modification (Fig. 5(h) and (i)).
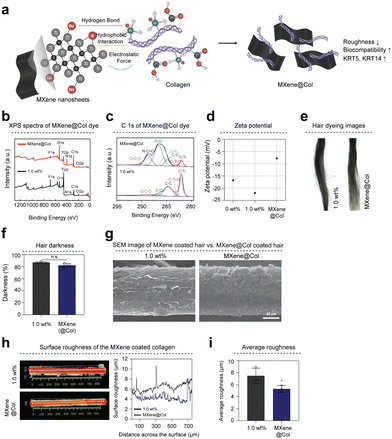 |
| Fig. 5 Characterization of collagen surface-modified MXene and MXene@Col dye. (a) Schematic of MXene surface modification using collagen type 1. (b) XPS spectra of MXene dye and MXene@Col dye. (c) C 1s of MXene dye and MXene@Col dye. (d) Zeta-potential of uncoated (0 wt%), MXene dye coated (1.0 wt%), MXene@Col-coated hair. (e) Surface-modified MXene-based dye hair coating gross image. (f) Brightness of hair coated with surface-modified MXene dye. (g) SEM image showing the surface of hair coated with surface-modified MXene dye. (h) Three-dimensional surface roughness measurement image and Surface roughness quantification graph of hair coated with the MXene@Col dye. (i) Average roughness quantification graph of hair coated with MXene@Col dye. All data represent mean ± SD (n = 3). *p < 0.05 The symbol * indicates comparisons with 1.0 wt%. Scale bars = 40 μm (g). | |
2.6 Biological properties of MXene@Col dye
To confirm the biocompatibility of MXene@Col dye, live/dead assay on keratinocytes was performed (Fig. 6(a)). Cell viability showed a high survival rate of over 99.12% up to 7 days (Fig. 6(b)). Additionally, we confirmed the expression of KRT5 and KRT14 by immunofluorescence staining analysis (Fig. 6(c)). As a result, overexpression of KRT5 and KRT14 was observed in the group coated with MXene dye and MXene@Col dye (Fig. 6(d)). We further examined KRT5 and KRT 14 gene expression levels in cultured HaCaT in three groups (0 wt%, 1.0 wt%, MXene@Col). The expression of KRT5 was upregulated by 3.02-fold and 5.21-fold in the 1.0 wt% and MXene@Col groups, respectively, compared to 0 wt%. The expression of KRT14 was also upregulated the most in the MXene@Col group, 5.81-fold compared to 0 wt% (Fig. 6(e)). To confirm whether the MXene@Col dye coating can block ultraviolet (UV), we evenly applied each group (0 wt%, 1.0 wt%, MXene@Col) to BALB/c-nu mice and irradiated UV-A with a wavelength of 395 nm to penetrate the skin. The extent of skin damage was confirmed (Fig. 6(f)). UV-induced skin damage was assessed through histological analysis (Fig. 6(g)). In hematoxylin-eosin staining, except for the 0 wt% group, almost no damage to the stratum corneum, which exists in the outermost layer of the epidermis layer, was observed. Still, in the UV-treated 0 wt% group, the stratum corneum thickness was significantly thinner (9.28 μm) (Fig. 6(h)). Additionally, TUNEL (Terminal deoxynucleotidyl transferase biotin-dUTP nick and labelling) immunofluorescence staining results were used to confirm cell damage caused by UV. The cell apoptosis rate by UV treatment showed the highest rate of apoptosis (7.85%) in the 0 wt% group (Fig. 6(i)).
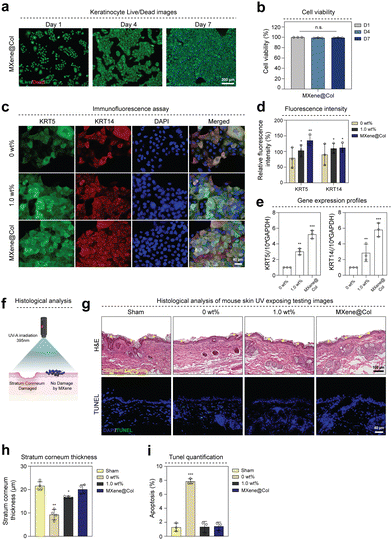 |
| Fig. 6 Evaluation of biological properties of MXene@Col dye. (a) Live/dead image of HaCaT cells co-cultured with MXene@Col dye-coated hair. (b) Quantification of cell viability obtained from live/dead assay. (c) Immunofluorescence staining image of keratinocytes cultured together in uncoated and coated hair (green: KRT5, red: KRT14, blue: DAPI), (d) Quantification of the relative intensity of KRT5 and KRT14. (e) Gene expression profile of hair coated with MXene@Col dye. (f) Schematic diagram of UV protection experiments of MXene@Col dye. (g) Hematoxylin and eosin (H&E) staining and TUNEL staining images of UV-treated tissues from different groups. (h) Quantification of stratum corneum thickness in each group. (i) Quantification of apoptosis cells in each group. All data represent mean ± SD (n ≥ 3). *p < 0.05, **p < 0.01, ***p < 0.001 The symbol * indicates comparison with 0 wt% (b), (d) and (e) and sham (h) and (i), respectively. Scale bars = 200 μm (a), 40 μm (c), 100 μm (g, top), 40 μm (g, bottom). | |
3. Discussion
As the worldwide aging population increases and the demand for hair dyes to improve appearance increases, research into safe and effective hair dyes is expanding.34–38 This study explored the potential of MXene nanosheet-based hair dyes for safe and multifunctional hair dyeing. In this study, the MXene hair dye produced contains chitosan and ascorbic acid, natural polymers manufactured in a sprayable liquid formulation, enabling simple and fast dyeing. There was little difference in the stress–strain curves before and after dye-coated hair, supporting that dye-coated hair does not cause hair damage. Interestingly, due to the unique properties of MXene with high surface area and excellent electrical and thermal properties, MXene-based hair dyes could impart antistatic properties, excellent heat dissipation function, and electromagnetic wave shielding performance to dyed hair.39,40
Additionally, the functional groups on the MXene surface interact with the hair's keratin surface, allowing it to be evenly coated. In particular, the unique color of Ti3C2Tx MXene makes it possible to dye natural dark hair colors. By adjusting the concentration of MXene, we could fine-tune the colors to darker tones or gradient colors. In contrast, compared to existing commercial products, hair dyeing with MXene dyes showed no dark color differences and exhibited multifunctional capabilities. MXene nanosheet-based dyes can be prepared without potentially toxic chemical additives, which represents a promising way to overcome the limitations of conventional dyes and achieve hair comfort due to their multi-functionality. The safety of the MXene nanosheet-based dye was confirmed through biocompatibility evaluation. Cell viability evaluation for HaCaT showed good biocompatibility, suggesting favorable safety for clinical application.
Moreover, it was confirmed that MXene@Col dye, in which the surface of MXene was modified with collagen, softened the hair surface and induced overexpression of type II keratin KRT5 and type I keratin KRT14. KRT5 and KRT14 expressed in keratinocytes form an intracellular cytoskeleton and help maintain cell shape.41,42 This demonstrates the potential for overexpression of KRT5 and KRT14 due to MXene@Col dye coating to lead to an essential role in strength and health by shaping the external structure and helping hair remain solid and elastic.43 In addition, the results of the skin damage test caused by UV irradiation of MXene@Col dye showed that MXene@Col dye coating can prevent direct skin damage caused by UV. As a result, it shows the possibility of designing various physicochemical and biological properties of dyes by modifying various synthetic and natural polymers in addition to collagen on the MXene surface.33,44,45
However, despite the promising potential of MXene nanosheet-based hair dyes, several challenges remain to be addressed. One notable consideration is the scalability of the MXene synthesis and formulation process. As demand for these innovative hair dyes increases, efforts must be made to develop efficient and cost-effective methods for large-scale production without compromising the quality and safety of the final product. Additionally, the darkening process of MXene flakes requires further optimization to achieve consistent and predictable results. Research to understand factors affecting permanent coatings and color intensity will contribute to the reproducibility and customization of MXene-based hair colors. The potential impact of long-term use of MXene-based dyes on hair health and integrity also requires thorough investigation. Although the non-reactive and non-invasive nature of MXene nanosheets suggests their safety, their long-term effects on hair structure and scalp health must be comprehensively assessed through rigorous studies.
4. Conclusion
In this study, we proposed a safe dye based on MXene nanosheets that can provide natural hair color and multifunction. This MXene dye was coated on the hair surface and demonstrated antistatic properties, heat dissipation, and electromagnetic interference shielding properties. The biocompatibility of the MXene nanosheet-based dye showed high cell viability for HaCaT. In addition, when coating the hair with MXene@Col dye through MXene collagen surface modification, surface roughness was reduced, and the expression of KRT5 and KRT14 was upregulated. Overall, we show that hair dyes utilizing MXene nanosheets can provide functionality and comfort by imparting antistatic properties, heat dissipation, and electromagnetic shielding performance to hair and providing safety. These MXene-based dyes have promising potential as innovative hair dyes that can replace traditional hair dyes.
5. Experimental section
5.1 Materials
Delaminated Ti3C2Tx MXene, few-layer nanoflakes (NANOPLEXUS), (Product Code: MXNTI3C2TX-FLN) were purchased from Haydale Technologies Co., Ltd (UK). Chitosan (medium molecular weight, 448877) and L-ascrobic acid (99%, A92902) were purchased from Sigma-Aldrich. Type I bovine collagen solution (TeloCol®-10, Advanced Biomatrix, #5226) was purchased from Cellink (Sweden). Human hair samples were purchased from Hairmadang (Korea, 20513).
5.2 Preparation of MXene hair dyes
Add 25 mg of L-ascorbic acid to a 20 mL vial and 10 mL of distilled water. Place the vial containing L-ascorbic acid and distilled water on a 70 °C hotplate. Stir the warm L-ascorbic acid solution well. Add chitosan to the solution to make it a 1
:
1 ratio with L-ascorbic acid. Heat and stir the solution at 400 rpm overnight. Then, add Ti3C2Tx MXene at various concentrations (0.1 wt%, 0.5 wt%, 1.0 wt%) to the solution and sonicate for 30 minutes to disperse evenly.
5.3 Characterization
Adobe Photoshop software was used for quantitative analysis of hair brightness. Three points were randomly selected from the digital image of the hair sample, and the RGB average value (l = lR + lG + lB/3) was calculated. Based on the RGB average value (l0) of uncoated hair (0 wt%), the brightness was calculated as l/l0 × 100. For SEM imaging of hair samples, 1 cm hair samples coated with MXene dye (1.0 wt%) were coated with platinum for the 80 s at 30 mA before imaging. SEM images were taken with a HITACHI (SU3800) scanning electron microscope at 15.0 kV. 4 cm-sized MXene dye-coated hair samples were prepared to measure the tensile strength. Tensile tests were performed with the SurTA STD system. Raman spectroscopy measurements were performed on a Raman spectrometer (Raman touch) under the 785 nm laser excitation. The morphological analysis involved the utilization of field emission scanning electron microscope (FE-SEM, JSM-7610F (JEOL, Japan)) and transmission electron microscope (TEM, JEM-2100F (JEOL, Japan)). An environmental chamber (PL-1KP, TABAI ESPEC CORP.) was used for the 85/85 test. To compare the heating and cooling characteristics of the coated hair samples, the thermal properties were measured on a hotplate set at room temperature (23 °C) and 37 °C. First, the heating properties were measured by placing the hair samples on a hot plate set at 37 °C. Cooling properties were measured by transferring heated hair samples to a hotplate set to room temperature. Thermal images were taken with an IR camera (FLIR E6) at 5 second intervals. Electromagnetic interference (EMI) shielding appraisals were carried out using a Keysight E5071C network analyzer (USA) across the frequency span of 8.2–12.4 GHz, employing the waveguide methodology. Colorimetric qualities of dyed human specimens were quantified through a Gretag Macbeth Color-Eye 3100 Spectrophotometer (USA), encompassing CIE Lab values (L*, a*, b*, c*, and h°). The X-ray photoelectron spectroscopy (XPS) evaluations were executed using a K Alpha+ spectrometer (Thermo VG, USA), which was equipped with a monochromatized Al Kα (hν = 1486.6 eV) radiation source. Zeta potentials were gauged at 25 °C using an ELSZ-2000 apparatus (Otsuka Electronics, Japan). To measure the surface roughness of hair coated with MXene dye and MXene@Col dye, the coated hair was cut into 3 cm pieces using a 3D Optical profiler (Zygo Corp, Zegage™ Pro), and the surface roughness of the coated hair was measured.
5.4 Surface modification of MXene with collagen and preparation of MXene@Col hair dyes
To modify the surface of Ti3C2Tx MXene, MXene was dispersed in 5 mL of distilled water and sonicated for 30 minutes to make it uniform. Collagen type 1 at a concentration of 10 mg mL−1 was then added, and the mixture was stirred overnight at 400 rpm. The liquid was separated using a centrifuge (13
000 rpm) for 10 minutes, and the surface-modified MXene was obtained by washing it 2–3 times with deionized water to remove unbound collagen. As previously explained, MXene@Col hair dye is produced by uniformly mixing MXene surface-modified with collagen with a 1
:
1 mixture of chitosan and ascorbic acid at 10 mg mL−1 concentration.
5.5 Hair dyeing with MXene and surface modified-MXene
Before hair dyeing, the samples were completely washed with water to remove the existing surface coating. The MXene dye was transferred to a 20 mL spray bottle, and the dye was sprayed on the hair samples at intervals of 5 cm. Spread evenly and then combed to ensure a visually even coating. Then dry in a 60 °C oven for 30 minutes.
5.6 Antistatic test
Hair samples were rubbed against a rubber balloon 10 times to induce an electrostatic charge. The induced static voltage was measured with a static meter (CR-2) at a distance of 1 inch from the hair sample. Measurements were repeated 3 times.
5.7 Durability test
The hair samples were completely submerged in a 50 mL conical tube containing 5 v/v% shampoo in 40 mL water. Then, vortex (AVM-10) the conical tube containing the hair sample and shampoo solution for 30 seconds. Then, the hair samples were rinsed with water and dried in a 60 °C oven for 10 minutes. This process was repeated a total of 30 times for each sample.
5.8 Cytotoxicity test
The cytotoxicity of MXene dye was investigated using a live/dead assay on HaCaT cells. The hair sample was prepared by coating it with MXene dye and then using a sterilizer. Two to three hair samples coated with different dye concentrations were placed in the upper chamber of Transwell® (SPL, 37012) and seeded at 5 × 104 cells per well in the lower chamber. The cells were cultured in’ Dulbecco's modified’ Eagle's media (DMEM) (Stemcell), which contained 10% fetal bovine serum and 1% penicillin–streptomycin–glutamine (100×) (Gibco). The medium was replaced every 2–3 days, and the cells were stained with a live/dead assay kit (Invitrogen, L3224) on days 1, 4, and 7 of the culture. Images were captured using a fluorescence microscope (Zeiss, Axio Observer 3).
5.9 Immunofluorescence (IF) staining
Samples were fixed with 4% paraformaldehyde (PFA) for 10 minutes, permeabilized, and blocked with 2% BSA and 0.5% PBST (0.5% Triton X-100 in PBS) for 1 hour. After washing with PBS, the cells were incubated with primary antibody KRT5 (1:500, ab52635, Abcam) or KRT14 (1:500, ab7800, Abcam) for 1 hour at RT. After washing with PBST, secondary antibody Alexa Fluor® 488 (1:300, ab150077, Abcam) or Alexa Fluor® 594 (1:300, ab150116, Abcam) was incubated at RT for 1 hour. Finally, after washing with PBST, counterstaining with DAPI (1:3000, D1306, Invitrogen™) was observed under a fluorescence microscope. Relative intensity was quantified using J software.
5.10 Gene expression profile
Using Trizol (Trizol®, Life Technology, USA), total RNAs were isolated from the cell, and the SuperScript Synthesis System (Invitrogen™, USA) was used to reverse-transcribe the RNAs into cDNA. SYBR Green PCR Master-mix was used for real-time PCR in accordance with the ABI StepOnePlusTM real-time PCR equipment manufacturer's instructions (Applied Biosystems, USA). The reference gene used was GAPDH, and cDNA samples were examined to identify the genes of interest (KRT5 and KRT14). Next, each target's gene expression level was computed as −2ΔΔCt.46 For the purpose of finding the relevant gene, each sample underwent at least three analyses. The target genes were chosen to be KRT5 (Hs00361185_m1, ThermoFisher) and KRT14 (Hs00265033_m1, ThermoFisher).
5.11
In vivo UV protection analysis
All animal procedures were approved and followed by the Institutional Animal Care and Use Committee at the Seoul National University (SNU-230915-3). All animal procedures were conducted in compliance with the Korean government law (Animal Protection Act, APA, and the Laboratory Animal Act, LAA). BALB/c-nu mice obtained from NARA Biotech were used in this study. Balb-c/nu was divided into a group without dye applied and a group with MXene and MXene@Col dye applied and irradiated with UV-A (395 nm) wavelength for 40 minutes at 5 cm intervals. Mice were euthanized using excessive CO2, and skin was harvested. After washing the skin with PBS, skin samples were fixed with 4% paraformaldehyde. Histological analysis was assessed by H&E and TUNEL staining. Stratum corneum thickness and apoptosis cells were quantified using Image J software.
5.12 Statistical analysis
All experiments were performed in at least three replicates. Statistics for all data are presented as mean values and corresponding standard deviations (SD). Statistical significance was assessed using one-way ANOVA.
Author contributions
H. D. K. and H. P. Designed the study. H. D. K. and H. P. prepared samples, led the experiments, analyzed the data, produced the figures, and wrote the manuscript. H. D. K. contributed to validating the data analysis. H. P., Y. H. P., G. K., S. K., G. M., N. S. H., and I. I. contributed to performing the experiments and editing the manuscript.
Conflicts of interest
The authors declare no competing interest.
Acknowledgements
This work was supported by the Ministry of Science and ICT of Korea (NRF-2021R1C1C2004576). This research was also supported by the Korean Fund for Regenerative Medicine (KFRM) grant funded by the Korean government (the Ministry of Science and ICT, the Ministry of Health & Welfare). (Code: KFRM 22A0105L1-11). This work was also supported by the Basic Research Laboratory grant funded by the National Research Foundation of Korea (RS-2023-00222737).
References
- D. Zhou, K. Ota, C. Nardin, M. Feldman, A. Widman, O. Wind, A. Simon, M. Reilly, L. R. Levin, J. Buck, K. Wakamatsu, S. Ito and J. H. Zippin, Sci. Signaling, 2018, 11, eaau7987 CrossRef PubMed.
- W. J. Pavan and R. A. Sturm, Annu. Rev. Genomics Hum. Genet., 2019, 20, 41–72 CrossRef CAS PubMed.
- C. Battistella, N. C. McCallum, K. Gnanasekaran, X. Zhou, V. Caponetti, M. Montalti and N. C. Gianneschi, ACS Cent. Sci., 2020, 6, 1179–1188 CrossRef CAS PubMed.
- K. J. Ng, J. Lim, Y. N. Tan, D. Quek, Z. Lim, N. Pantelireis and C. Clavel, Cell Rep., 2022, 40(3), 111100 CrossRef CAS PubMed.
- O. J. X. Morel and R. M. Christie, Chem. Rev., 2011, 111, 2537–2561 CrossRef CAS PubMed.
- H. J. Sung, M. F. Khan and Y. H. Kim, Int. J. Biol. Macromol., 2019, 136, 20–26 CrossRef CAS PubMed.
- Y. Zhang, B. M. Birmann, J. Han, E. L. Giovannucci, F. E. Speizer, M. J. Stampfer, B. A. Rosner and E. S. Schernhammer, BMJ, 2020, 370, m2942 CrossRef PubMed.
- L. He, F. Michailidou, H. L. Gahlon and W. Zeng, Chem. Res. Toxicol., 2022, 35, 901–915 Search PubMed.
- M. Zhang, M. Zhang, Q. Zeng, D. Lin and N. Zhang, Sci. Rep., 2023, 13, 2623 Search PubMed.
- S. Y. Kim, J. H. Kim, Y. Kang, J. W. Yoo, J. Choi and H. J. Lee, J. Cleaner Prod., 2022, 363, 132535 CrossRef CAS.
- P. Walter, E. Welcomme, P. Hallégot, N. J. Zaluzec, C. Deeb, J. Castaing, P. Veyssière, R. Bréniaux, J.-L. Lévêque and G. Tsoucaris, Nano Lett., 2006, 6, 2215–2219 CrossRef CAS PubMed.
- A. C. Santos, A. Panchal, N. Rahman, M. Pereira-Silva, I. Pereira, F. Veiga and Y. Lvov, Nanomaterials, 2019, 9, 903 CrossRef CAS PubMed.
- H. Cui, W. Xie, Z. Hua, L. Cao, Z. Xiong, Y. Tang and Z. Yuan, Molecules, 2022, 27, 8062 CrossRef CAS PubMed.
- A. VahidMohammadi, J. Rosen and Y. Gogotsi, Science, 2021, 372, eabf1581 CrossRef CAS PubMed.
- B. Anasori, M. R. Lukatskaya and Y. Gogotsi, Nat. Rev. Mater., 2017, 2, 16098 CrossRef CAS.
- M. Naguib, M. W. Barsoum and Y. Gogotsi, Adv. Mater., 2021, 33, 2103393 CrossRef CAS PubMed.
- K. Hantanasirisakul and Y. Gogotsi, Adv. Mater., 2018, 30, 1804779 CrossRef PubMed.
- B. Fu, J. Sun, C. Wang, C. Shang, L. Xu, J. Li and H. Zhang, Small, 2021, 17, 2006054 CrossRef CAS PubMed.
- Y.-Z. Zhang, Y. Wang, Q. Jiang, J. K. El-Demellawi, H. Kim and H. N. Alshareef, Adv. Mater., 2020, 32, 1908486 CrossRef CAS PubMed.
- H. Lin, Y. Chen and J. Shi, Adv. Sci., 2018, 5, 1800518 CrossRef PubMed.
- S. Iravani and R. S. Varma, Mater. Adv., 2021, 2, 2906–2917 RSC.
- R. D. Sinclair, J. Invest. Dermatol. Symp. Proc., 2007, 12, 2–5 CrossRef PubMed.
- Y. Tang, Z. Zhang, S. Yang, G. J. Smith and L. Liu, Colloids Surf., B, 2021, 200, 111599 CrossRef CAS PubMed.
- S. S. Adav, R. S. Subbaiaih, S. K. Kerk, A. Y. Lee, H. Y. Lai, K. W. Ng, S. K. Sze and A. Schmidtchen, Sci. Rep., 2018, 8, 1599 CrossRef PubMed.
- H. Hamedi, S. Moradi, S. M. Hudson, A. E. Tonelli and M. W. King, Carbohydr. Polym., 2022, 282, 119100 CrossRef CAS PubMed.
- H. Li, R. Fan, F. Zhang, Z. Cui, J. Li, Y. Cai, L. Kang, X. Zhan, J. Li and D. Tian, Ind. Crops Prod., 2023, 193, 116252 CrossRef CAS.
- K. Rasool, M. Helal, A. Ali, C. E. Ren, Y. Gogotsi and K. A. Mahmoud, ACS Nano, 2016, 10, 3674–3684 CrossRef CAS PubMed.
- L. Zhou, H. Zheng, Z. Liu, S. Wang, Z. Liu, F. Chen, H. Zhang, J. Kong, F. Zhou and Q. Zhang, ACS Nano, 2021, 15, 2468–2480 CrossRef CAS PubMed.
- A. M. Jastrzębska, A. Szuplewska, T. Wojciechowski, M. Chudy, W. Ziemkowska, L. Chlubny, A. Rozmysłowska and A. Olszyna, J. Hazard. Mater., 2017, 339, 1–8 CrossRef PubMed.
- G. Venkatesan, Y. Dancik, A. Sinha, H. M. Kyaw, R. Srinivas, T. L. Dawson, M. Bigliardi, P. Bigliardi and G. Pastorin, J. Hazard. Mater., 2021, 402, 123712 CrossRef CAS PubMed.
- C.-J. Chang, K. M. O’Brien, A. P. Keil, S. A. Gaston, C. L. Jackson, D. P. Sandler and A. J. White, J. Natl. Cancer Inst., 2022, 114, 1636–1645 CrossRef PubMed.
- M. Zheng, X. Wang, Y. Chen, O. Yue, Z. Bai, B. Cui, H. Jiang and X. Liu, Adv. Healthcare Mater., 2023, 12, 2202042 CrossRef CAS PubMed.
- A. Rozmysłowska-Wojciechowska, A. Szuplewska, T. Wojciechowski, S. Poźniak, J. Mitrzak, M. Chudy, W. Ziemkowska, L. Chlubny, A. Olszyna and A. M. Jastrzębska, Mater. Sci. Eng., C, 2020, 111, 110790 CrossRef PubMed.
- K.-H. Kim, E. Kabir and S. A. Jahan, Environ. Int., 2016, 89–90, 222–227 CrossRef CAS PubMed.
- G. Venkatesan, Y. Dancik, Y. R. Neupane, A. V. Karkhanis, P. Bigliardi and G. Pastorin, ACS Sustainable Chem. Eng., 2022, 10, 838–849 CrossRef CAS.
- I. Guryanov, E. Naumenko and R. Fakhrullin, Appl. Surf. Sci. Adv., 2022, 7, 100188 CrossRef.
- S. L. More, E. S. Fung, C. Mathis, A. M. Schulte and D. Hollins, Regul. Toxicol. Pharmacol., 2023, 138, 105331 CrossRef CAS PubMed.
- J.-e Lim, J. Huang, S. J. Weinstein, D. Parisi, S. Männistö and D. Albanes, Sci. Rep., 2023, 13, 3776 CrossRef CAS PubMed.
- L. Li, Y. Cao, X. Liu, J. Wang, Y. Yang and W. Wang, ACS Appl. Mater. Interfaces, 2020, 12, 27350–27360 CrossRef CAS PubMed.
- X. Guan, Z. Yang, M. Zhou, L. Yang, R. Peymanfar, B. Aslibeiki and G. Ji, Small Struct., 2022, 3, 2200102 CrossRef CAS.
- H. Alam, L. Sehgal, S. T. Kundu, S. N. Dalal and M. M. Vaidya, Mol. Biol. Cell, 2011, 22, 4068–4078 CrossRef CAS PubMed.
- Y. Guo, C. J. Redmond, K. A. Leacock, M. V. Brovkina, S. Ji, V. Jaskula-Ranga and P. A. Coulombe, eLife, 2020, 9, e53165 CrossRef CAS PubMed.
- S. Y. An, H.-S. Kim, S. Y. Kim, S. Y. Van, H. J. Kim, J.-H. Lee, S. W. Han, I. K. Kwon, C.-K. Lee, S. H. Do and Y.-S. Hwang, Commun. Biol., 2022, 5, 1270 CrossRef CAS PubMed.
- S. Luo, T. Xiang, J. Dong, F. Su, Y. Ji, C. Liu and Y. Feng, J. Mater. Sci. Technol., 2022, 129, 127–134 CrossRef CAS.
- Y.-D. Zhu, X.-Y. Ma, L.-P. Li, Q.-J. Yang, F. Jin, Z.-N. Chen, C.-P. Wu, H.-B. Shi, Z.-Q. Feng, S.-K. Yin and C.-Y. Li, Adv. Healthcare Mater., 2023, 12(25), 2300731 CrossRef CAS PubMed.
- K. J. Livak and T. D. Schmittgen, Methods, 2001, 25, 402–408 CrossRef CAS PubMed.
|
This journal is © The Royal Society of Chemistry 2024 |
Click here to see how this site uses Cookies. View our privacy policy here.