DOI:
10.1039/D4OB00859F
(Communication)
Org. Biomol. Chem., 2024,
22, 5734-5738
Enal-azomethine ylides: application in the synthesis of functionalized pyrroles†‡
Received
23rd May 2024
, Accepted 26th June 2024
First published on 27th June 2024
Abstract
Rhodium-catalyzed [3 + 2] annulation of diazoenals and N-alkyl imines resulted in N-alkyl-pyrrole-3-carbaldehyde derivatives. The reaction involves thermal 6π-electrocyclization and aromatization of a new class of enal-azomethine ylides (EAYs). The EAYs derived from dihydroisoquinoline and 2H-azirine gave fused-pyrrole and pyridine derivatives, respectively. The synthetic importance of pyrrole products has been demonstrated by one-step synthesis of the biologically relevant pyrrolo[3,2-c]quinoline scaffold as well as pyrrolo[2,1-a]isoquinoline which is a core structure of lamellarin alkaloids.
The azomethine ylide (AY), originally introduced by Huisgen, is a fundamentally important 1,3-dipole that finds broad applications in the construction of N-heterocycles (Scheme 1a).1–6 AYs have been used as three-atom 4π-synthons in electrocyclizations and [3 + n] cycloadditions leading to the construction of valuable 3-membered and 5–7-membered monocyclic, fused, and bridged nitrogen heterocycles.1–6 Inspired by the synthetic potential of azomethine ylides, herein we report the design of a conceptually new class of enal-functionalized azomethine ylides (Scheme 1b). The synthetic utility of enal-azomethine ylides (EAYs) has been demonstrated in the Rh-catalyzed [3 + 2] annulation of diazoenals and N-alkyl aldimines resulting in one-step synthesis of tetrasubstituted N-alkyl-3-formylpyrroles.7,8 The N-alkyl pyrroles are core structures of many natural products and pharmaceuticals.9
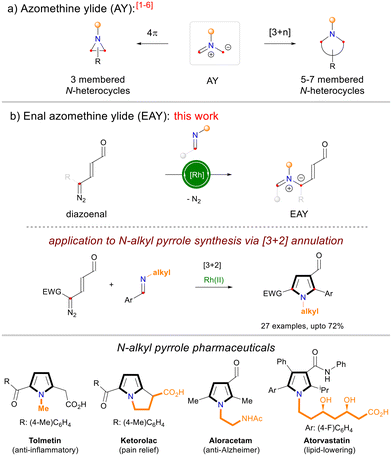 |
| Scheme 1 Construction of N-heterocycles by azomethine ylide cyclizations. | |
Recently, we have introduced a new class of bench-stable enal-functionalized diazo compounds (diazoenals) which serve as precursors to the highly electrophilic Rh-enalcarbenoids.10a Our studies showed that Rh-enalcarbenoids react with a variety of carbon and heteroatom nucleophiles resulting in valuable functionalized enals which are used in the subsequent cyclization reactions to access diverse carbo/heterocycles.10b–h Continuing our studies, we have investigated the reactivity of Rh-enalcarbenoids with N-alkyl imines. Preliminary experiments showed that the reaction of diazoenal 1a with imine 2a in the presence of 3 mol% Rh2(OAc)4 in toluene solvent at 40 °C gives an unstable enal-azomethine ylide (EAY).11 Gratifyingly, at an elevated temperature (110 °C), the reaction gave N-allyl-3-formylpyrrole 3a in 57% yield (Table 1, entry 1) via an overall [3 + 2]-annulation involving 6π-electrocyclization of the EAY and the subsequent in situ oxidation. It is worth mentioning that this is the first report on enal-functionalized azomethine ylides and their application in densely functionalized N-alkyl pyrrole synthesis.
Table 1 Optimization of the [3 + 2] annulationa
Inspired by the preliminary studies, we further optimized the [3 + 2] annulation. Although, other Rh(II)-catalysts such as Rh2(oct)4, Rh2(esp)2, Rh2(TFA)4, and Rh2(S-DOSP)4 are also effective, Rh2(oct)4, gave the highest yield of 72% (entries 2–5). Rapid decomposition of diazoenal was observed with the highly electrophilic Rh2(TFA)4 leading to a low yield of pyrrole (entry 5). At elevated temperatures, the imine was slowly dissociated to the aldehyde and amine, leading to a reduced yield of pyrrole (entry 7). On the other hand, the yield was diminished upon reducing the temperature due to sluggish reactivity (entries 6 and 8). The reaction was compatible with aromatic solvents whereas non-aromatic solvents are not suitable (entries 9 and 10).
With the optimized conditions (Table 1, entry 2), the scope of the [3 + 2] annulation was studied by varying the imines and diazoenals (Table 2). The reaction was compatible with diverse N-alkyl imines resulting in the corresponding N-substituted pyrroles 4–9 in 49–68% yields. The sterically hindered N-tBu and N-cyclohexyl imines were also tolerated in the reaction (6, 7). Notably, N-Boc-protected 1,2-ethylenediamine derived aldimine successfully delivered pyrrole 9, an analogue of the drug aloracetam in 49% yield, despite the competing NH-insertion reaction of the side chain. N-Benzyl imine gave pyrrole 10 in 65% yield. Gratifyingly, N-propargyl imine furnished the desired pyrrole 11 in 68% yield despite potential cyclopropenation. Interestingly, N-aryl aldimines gave poor yields of annulation products 12–14 (11–36%) presumably due to the sluggish reactivity and competing NH-insertion of the dissociated arylamines at elevated temperatures. Subsequently, aldimines derived from electronically and sterically distinct aryl aldehydes were investigated. The reaction tolerated alkyl, trifluoroalkyl, and halo substituents on the aryl-ring and delivered the C-aryl pyrroles 15–25 in 53–69% yields. Aldimines with electron-rich and electron-deficient aryl groups also smoothly participated in the reaction and gave good yields of pyrroles 26–28. The reaction was not hampered by sterically hindered aryl groups (23–24, 26 and 28). Aldimines derived from naphthaldehyde and pyrenecarboxaldehyde gave π-extended pyrrole products 29 and 30, respectively, in decent yields. With respect to the diazoenals, both keto and ester diazoenals reacted smoothly to give the desired pyrrole products in good yields (e.g.4–7, 10, 17–22).
Table 2 Substrate scope of the [3 + 2] annulationa,b
Reaction was performed using the optimized conditions in Table 1.
Isolated yield.
Yield was calculated after excluding the recovered aldehyde.
Reaction time.
|
|
Next, the synthetic utility of enal-azomethine ylides derived from the cyclic imines was investigated (Scheme 2a and b). A Rh-catalyzed reaction of 3,4-dihydroisoquinoline 2u and diazoenal 1g under standard conditions gave pyrroloisoquinoline 31 in 35% yield via EAY 32 (Scheme 2a). However, 3-phenyl-2H-azirine 2v under the same reaction conditions produced 4-formyl pyridine 33 in 40% yield via an overall [3 + 3] annulation. The reaction is proposed to involve strain-induced ring opening of azirine derived EAY 34, 6π-electrocyclization of the resulting azatriene 35 and the subsequent aromatization of dihydropyridine 36 (Scheme 2b).12
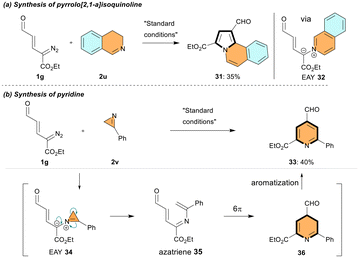 |
| Scheme 2 Reaction with cyclic imines. | |
The tetrasubstituted N-allyl-3-formyl-pyrroles obtained from [3 + 2] annulation are valuable substrates for structural diversification (Scheme 3a and b). The ethyl 1-allyl-4-formyl-5-(2-nitrophenyl)-1H-pyrrole-2-carboxylate 28 was converted to pyrrolo[3,2-c]quinoline 37 in 70% yield via reductive cyclization (Scheme 3a).13 The pyrrolo[3,2-c]quinoline derivatives are known to possess diverse biological properties, including anticancer and anti-Alzheimer activities.14 Next, an intramolecular Heck reaction of ethyl 1-allyl-5-(2-bromophenyl)-4-formyl-1H-pyrrole-2-carboxylate 23 gave pyrrolo[2,1-a]isoquinoline 38 in good yield (55%). Compound 38 has the core structure of the lamellarin D alkaloid (Scheme 3b).15 The N-propargyl pyrrole 11 smoothly participated in the click reaction with benzyl azide to give triazole-tethered pyrrole 39 in 65% yield (Scheme 3c).
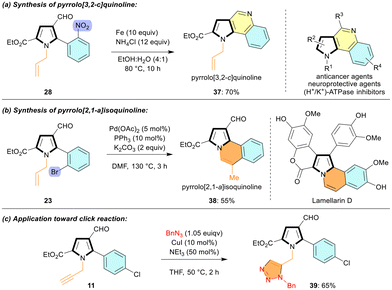 |
| Scheme 3 Synthetic applications. | |
A plausible mechanism for the [3 + 2] annulation reaction is proposed in Scheme 4. The transient Rh-enalcarbenoid 39 generated from diazoenal 1 reacts with N-alkyl aldimine 2 to give metal-bound EAY 40. The release of the Rh-catalyst leads to metal-free EAY 41. The formation of N-benzyl imine derived EAY 41a was detected using the HRMS data. The subsequent 6π-electrocyclization and spontaneous dehydrogenative aromatization7i,16 of dihydropyrrole (±)-42 furnish the N-alkyl-pyrrole-3-carbaldehyde 43.17
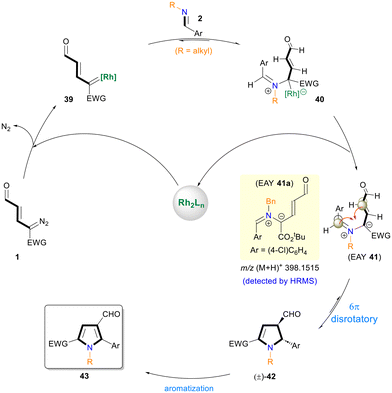 |
| Scheme 4 Plausible mechanism of [3 + 2]-annulation. | |
Conclusions
In conclusion, we have reported a new class of enal-functionalized azomethine ylides (EAYs). The synthetic utility of EAYs has been demonstrated by the Rh-catalyzed [3 + 2] annulation of N-alkyl imines and diazoenals resulting in the tetrasubstituted N-alkyl pyrrole-3-carbaldehyde derivatives. The EAYs derived from cyclic imines dihydroisoquinoline and 2H-azirine gave valuable fused pyrrole and pyridine derivatives. The synthetic utility of N-alkyl pyrrole products was demonstrated by the short synthesis of pyrrolo[3,2-c]quinoline and pyrrolo[2,1-a]isoquinoline scaffolds which are core structures of many biologically important compounds and natural products.
Data availability
The data supporting this article have been included as part of the ESI.‡
Crystallographic data for compound 19 have been deposited at the CCDC under accession number 2213403.
Conflicts of interest
There are no conflicts to declare.
Acknowledgements
This work was supported by the Science & Engineering Research Board (SERB Grants CRG/2018/001684 & CRG/2022/004022) and IISER Bhopal. PKM is the recipient of a research fellowship from the IISER Bhopal. We thank Jyoti Yadav (IISER Bhopal) for helping with the X-ray crystal structure determination. We are grateful to the NMR, X-ray and mass spectrometry facilities of the CIF-IISER Bhopal for the characterization data.
References
-
(a) R. Huisgen, Proc. Chem. Soc., 1961, 357–369 Search PubMed;
(b) R. Huisgen, Angew. Chem., Int. Ed. Engl., 1963, 2, 565–598 CrossRef;
(c) M. Breugst and H.-U. Reissig, Angew. Chem., Int. Ed., 2020, 59, 12293–12307 CrossRef CAS PubMed.
-
(a)
1,3-Dipolar Cycloaddition Chemistry, ed. A. Padwa, Wiley, New York, 1984, vol. 1 and 2 Search PubMed;
(b)
Synthetic Applications of 1,3-Dipolar Cycloaddition Chemistry Toward Heterocycles and Natural Products, ed. A. Padwa and W. H. Pearson, Wiley, New York, 2002 Search PubMed;
(c) P. A. Wade, Compr. Org. Synth., 1991, 4, 1111–1168 CrossRef;
(d) C. Najera and J. M. Sansano, Curr. Org. Synth., 2003, 7, 1105–1150 CAS.
- Selected reviews of [3 + 2] cycloadditions:
(a) K. V. Gothelf and K. A. Jørgensen, Chem. Rev., 1998, 98, 863–909 CrossRef CAS PubMed;
(b) I. Coldham and R. Hufton, Chem. Rev., 2005, 105, 2765–2809 CrossRef CAS PubMed;
(c) S. Husinec and V. Savic, Tetrahedron: Asymmetry, 2005, 16, 2047–2061 CrossRef CAS;
(d) G. Pandey, P. Banerjee and S. R. Gadre, Chem. Rev., 2006, 106, 4484–4517 CrossRef CAS PubMed;
(e) L. M. Stanley and M. P. Sibi, Chem. Rev., 2008, 108, 2887–2902 CrossRef CAS PubMed;
(f) R. Narayan, M. Potowski, Z.-J. Jia, A. P. Antonchick and H. Waldmann, Acc. Chem. Res., 2014, 47, 1296–1310 CrossRef CAS PubMed;
(g) T. Hashimoto and K. Maruoka, Chem. Rev., 2015, 115, 5366–5412 CrossRef CAS PubMed;
(h) L. Wei, X. Chang and C.-J. Wang, Acc. Chem. Res., 2020, 53, 1084–1100 CrossRef CAS PubMed.
- [3 + 3] cycloadditions:
(a) M.-C. Tong, X. Chen, H.-Y. Tao and C.-J. Wang, Angew. Chem., Int. Ed., 2013, 52, 12377–12380 CrossRef CAS PubMed;
(b) H. Guo, H. Liu, F.-L. Zhu, R. Na, H. Jiang, Y. Wu, L. Zhang, Z. Li, H. Yu, B. Wang, Y. Xiao, X.-P. Hu and M. Wang, Angew. Chem., Int. Ed., 2013, 52, 12641–12645 CrossRef CAS PubMed;
(c) S. Suárez-Pantiga, M. Colas, M. J. Johansson and A. Mendoza, Angew. Chem., Int. Ed., 2015, 54, 14094–14098 CrossRef PubMed;
(d) W.-L. Yang, C.-Y. Li, W.-J. Qin, F.-F. Tang, X. Yu and W.-P. Deng, ACS Catal., 2016, 6, 5685–5690 CrossRef CAS;
(e) S.-M. Xu, L. Wei, C. Shen, L. Xiao, H.-Y. Tao and C.-J. Wang, Nat. Commun., 2019, 10, 5553 CrossRef PubMed;
(f) X. Xu, P. Y. Zavalij and M. P. Doyle, J. Am. Chem. Soc., 2013, 135, 12439–12447 CrossRef CAS PubMed.
- [3 + 4] cycloadditions:
(a) K. Liu, H.-L. Teng and C.-J. Wang, Org. Lett., 2014, 16, 4508–4511 CrossRef CAS PubMed;
(b) W.-L. Yang, T. Ni and W.-P. Deng, Org. Lett., 2021, 23, 588–594 CrossRef CAS PubMed.
- [3 + 6] cycloadditions:
(a) M. Potowski, J. O. Bauer, C. Strohmann, A. P. Antonchick and H. Waldmann, Angew. Chem., Int. Ed., 2012, 51, 9512–9516 CrossRef CAS PubMed;
(b) H. Liu, Y. Wu, Y. Zhao, Z. Li, L. Zhang, W. Yang, H. Jiang, C. Jing, H. Yu, B. Wang, Y. Xiao and H. Guo, J. Am. Chem. Soc., 2014, 136, 2625–2629 CrossRef CAS PubMed;
(c) H.-L. Teng, L. Yao and C.-J. Wang, J. Am. Chem. Soc., 2014, 136, 4075–4080 CrossRef CAS PubMed;
(d) Q.-H. Li, L. Wei and C.-J. Wang, J. Am. Chem. Soc., 2014, 136, 8685–8692 CrossRef CAS PubMed;
(e) G. Bertuzzi, D. McLeod, L.-M. Mohr and K. A. Jørgensen, Chem. – Eur. J., 2020, 26, 15491–15496 CrossRef CAS PubMed.
- Pyrroles from azomethine ylides:
(a) M. Ohno, M. Komatsu, H. Miyata and Y. Ohshiro, Tetrahedron Lett., 1991, 32, 5813–5816 CrossRef CAS;
(b) M. Reisser and G. Maas, J. Org. Chem., 2004, 69, 4913–4924 CrossRef CAS PubMed;
(c) M. P. Doyle, M. Yan, W. Hu and L. S. Gronenberg, J. Am. Chem. Soc., 2003, 125, 4692–4693 CrossRef CAS PubMed;
(d) K. Kawashima, M. Hiromoto, K. Hayashi, A. Kakehi, M. Shiro and M. Noguchi, Tetrahedron Lett., 2007, 48, 941–944 CrossRef CAS;
(e) C. V. Galliford and K. A. Scheidt, J. Org. Chem., 2007, 72, 1811–1813 CrossRef CAS PubMed;
(f) Z. Wang, Y. Shi, X. Luo, D.-M. Han and W.-P. Deng, New J. Chem., 2013, 37, 1742–1745 RSC;
(g) I. Yavari, M. Naeimabadi and M. R. Halvagar, Tetrahedron Lett., 2016, 57, 3718–3721 CrossRef CAS;
(h) R. Robles-Machín, A. López-Pérez, M. González-Esguevillas, J. Adrio and J. C. Carretero, Chem. – Eur. J., 2010, 16, 9864–9873 CrossRef PubMed;
(i) S. Wang, X. Zhu, Z. Chai and S. Wang, Org. Biomol. Chem., 2014, 12, 1351–1356 RSC;
(j) V. A. Motornov, A. A. Tabolin, Y. V. Nelyubina and S. L. Ioffe, Org. Biomol. Chem., 2021, 19, 3413–3427 RSC;
(k) S. Sayebani, H. Eshghi and M. Naeimabadi, Mol. Divers., 2022, 26, 2221–2230 CrossRef CAS PubMed.
- Selected references for N-alkyl pyrrole synthesis:
(a) W. W. Tan and N. Yoshikai, Chem. Sci., 2015, 6, 6448–6455 RSC;
(b) D.-S. Kim, Y.-S. Seo and C.-H. Jun, Org. Lett., 2015, 17, 3842–3845 CrossRef CAS PubMed;
(c) X.-Y. Zhang, Z.-W. Yang, Z. Chen, J. Wang, D.-L. Yang, Z. Shen, L.-L. Hu, J.-W. Xie, J. Zhang and H.-L. Cui, J. Org. Chem., 2016, 81, 1778–1785 CrossRef CAS PubMed;
(d) X. Zhang, X. Xu, G. Chen and W. Yi, Org. Lett., 2016, 18, 4864–4867 CrossRef CAS PubMed;
(e) J. Peng, Y. Gao, C. Zhu, B. Liu, Y. Gao, M. Hu, W. Wu and H. Jiang, J. Org. Chem., 2017, 82, 3581–3588 CrossRef CAS PubMed;
(f) O. M. P. Zúñigaa, Á. G. Sathicqa, J. J. M. Zambranob and G. P. Romanelli, Curr. Org. Synth., 2017, 14, 1–18 CrossRef;
(g) K. Matsui, M. Shibuya and Y. Yamamoto, Commun. Chem., 2018, 1, 21 CrossRef;
(h) D. Andreou, M. G. Kallitsakis, E. Loukopoulos, C. Gabriel, G. E. Kostakis and I. N. Lykakis, J. Org. Chem., 2018, 83, 2104–2113 CrossRef CAS PubMed;
(i) K. Luo, S. Mao, K. He, X. Yu, J. Pan, J. Lin, Z. Shao and Y. Jin, ACS Catal., 2020, 10, 3733–3740 CrossRef CAS;
(j) L. Zuo, Y. Yang and W. Guo, Org. Lett., 2021, 23, 2013–2018 CrossRef CAS PubMed;
(k) T. Shi, G. Yin, X. Wang, Y. Xiong, Y. Peng, S. Li, Y. Zeng and Z. Wang, Green Synth. Catal., 2023, 4, 20–34 CrossRef CAS.
- Selected references for N-alkyl pyrrole natural products and pharmaceuticals:
(a) F. Fischer, M. Matthisson and P. Herrling, Neurodegener. Dis., 2004, 1, 50–70 CrossRef PubMed;
(b) S. I. Young, P. D. Thornton and A. Thompson, Nat. Prod. Rep., 2010, 27, 1801–1839 RSC;
(c) V. Bhardwaj, D. Gumber, V. Abbot, S. Dhiman and P. Sharma, RSC Adv., 2015, 5, 15233–15266 RSC;
(d) S. P. Adams, M. Tsang and J. M. Wright, Cochrane Database Syst. Rev., 2015, CD008226 Search PubMed;
(e) T. E. Mallinson, J. Paramed. Pract., 2017, 9, 522–526 CrossRef;
(f) N. Singh, S. Singh, S. Kohli, A. Singh, H. Asiki, G. Rathee, R. Chandra and E. A. Anderson, Org. Chem. Front., 2021, 8, 5550–5573 RSC;
(g) K. Seipp, L. Geske and T. Opatz, Mar. Drugs, 2021, 19, 514 CrossRef CAS PubMed;
(h) M. Elsayed, M. O. Aboelez, B. E. Elsadek, H. A. Sarhan, K. A. Khaled, A. Belal, A. Khames, Y. A. Hassan, A. A. Abdel-Rheem and E. B. Elkaeed, Pharmaceutics, 2022, 14, 880 CrossRef CAS PubMed.
- Synthetic applications of enalcarbene:
(a) S. G. Dawande, V. Kanchupalli, J. Kalepu, H. Chennamsetti, B. S. Lad and S. Katukovala, Angew. Chem., Int. Ed., 2014, 53, 4076–4080 CrossRef CAS PubMed;
(b) S. G. Dawande, V. Kanchupalli, B. S. Lad, J. Rai and S. Katukojvala, Org. Lett., 2014, 16, 3700–3703 CrossRef CAS PubMed;
(c) J. Kalepu and S. Katukojvala, Angew. Chem., Int. Ed., 2016, 55, 7831–7835 CrossRef CAS PubMed;
(d) V. Kanchupalli and S. Katukojvala, Angew. Chem., Int. Ed., 2018, 57, 5433–5437 CrossRef CAS PubMed;
(e) B. S. Lad and S. Katukojvala, ACS Catal., 2018, 8, 11807–11814 CrossRef CAS;
(f) V. Kanchupalli, L. A. Thorbole, J. Kalepu, D. Joseph, M. Arshad and S. Katukojvala, Org. Lett., 2022, 24, 3850–3854 CrossRef CAS PubMed;
(g) P. K. Mandal and S. Katukojvala, Chem. – Eur. J., 2024, 30, e202303862 CrossRef CAS PubMed;
(h) P. K. Mandal, R. Chakrawarti and S. Katukojvala, Org. Lett., 2024, 26, 3463–3468 CrossRef CAS PubMed.
- For generation of azomethine ylides from diazo compounds, see:
(a) A. Padwa and S. F. Hornbuckle, Chem. Rev., 1991, 91, 263–309 CrossRef CAS;
(b)
M. P. Doyle, M. A. McKervey and Y. Tao, Modern Catalytic Methods for Organic Synthesis with Diazo Compounds: From Cyclopropanes to Ylides, Wiley-VCH, Weinheim, 1998, pp. 238–279 Search PubMed.
- N. S. Y. Loy, A. Singh, X. Xu and C.-M. Park, Angew. Chem., Int. Ed., 2013, 52, 2212–2216 CrossRef CAS PubMed.
- S. Choudhary, J. Yadav, Mamta, A. P. Pawar, S. Vanaparthi, N. A. Mir, E. Iype, R. Sharma, R. Kant and I. Kumar, Org. Biomol. Chem., 2020, 18, 1155–1164 RSC.
-
(a) T. H. Brown, R. J. Ife, D. J. Keeling, S. M. Laing, C. A. Leach, M. E. Parsons, C. A. Price, D. R. Reavill and K. J. Wiggall, J. Med. Chem., 1990, 33, 527–533 CrossRef CAS PubMed;
(b) K. Grychowska, G. Satała, T. Koz, A. Partyka, E. Colacino, S. Chaumont-Dubel, X. Bantreil, A. Wesołowska, M. Pawłowski, J. Martinez, P. Marin, G. Subra, A. J. Bojarski, F. Lamaty, P. Popik and P. Zajdel, ACS Chem. Neurosci., 2016, 7, 972–983 CrossRef CAS PubMed;
(c) K. Grychowska, S. Chaumont-Dubel, R. Kurczab, P. Koczurkiewicz, C. Deville, M. Krawczyk, W. Pietruś, G. Satała, S. Buda, K. Piska, M. Drop, X. Bantreil, F. Lamaty, E. Pékala, A. J. Bojarski, P. Popik, P. Marin and P. Zajdel, ACS Chem. Neurosci., 2019, 10, 3183–3196 CrossRef CAS PubMed;
(d) K. Grychowska, U. Lopez-Sanchez, M. Vitalis, G. Canet, G. Satala, A. Olejarz-Maciej, J. Golebiowska, R. Kurczab, W. Pietrus, M. Kubacka, C. Moreau, M. Walczak, K. Blicharz-Futera, O. Bento, X. Bantreil, G. Subra, A. J. Bojarski, F. Lamaty, C. Becamel, C. Zussy, S. Chaumont-Dubel, P. Popik, H. Nury, P. Marin, L. Givalois and P. Zajdel, J. Med. Chem., 2023, 66, 14928–14947 CrossRef CAS PubMed;
(e)
M. Haroum and C. Tratrat, Pyrrolo[3,2-c]quinoline compounds as CK2 inhibitors, U.S. PatentUS11945818B1, 2024 Search PubMed.
- H. Fan, J. Peng, M. T. Hamann and J.-F. Hu, Chem. Rev., 2008, 108, 264–287 CrossRef CAS PubMed.
- J. Barluenga, G. Lonzi, L. Riesgo, L. A. López and M. Tomás, J. Am. Chem. Soc., 2010, 132, 13200–13202 CrossRef CAS PubMed.
- The formyl group was necessary for spontaneous dehydrogenative aromatization. See the ESI for control experiments.‡.
Footnotes |
† Dedicated to Professor Sukh Dev on the occasion of his 100th birthday. |
‡ Electronic supplementary information (ESI) available. CCDC 2213403. For ESI and crystallographic data in CIF or other electronic format see DOI: https://doi.org/10.1039/d4ob00859f |
|
This journal is © The Royal Society of Chemistry 2024 |
Click here to see how this site uses Cookies. View our privacy policy here.