DOI:
10.1039/D3QO00599B
(Research Article)
Org. Chem. Front., 2024,
11, 142-148
Direct electrochemical difluorination and azo-fluorination of gem-difluorostyrenes†
Received
25th April 2023
, Accepted 13th September 2023
First published on 22nd November 2023
Abstract
Herein, a direct electrolysis protocol for the chemo- and regio-selective difluorination, azo-fluorination, and fluoro-alkoxylation of gem-difluorostyrenes under mild conditions is demonstrated. The reaction enables the selective synthesis of a distinct type of long-chain perfluoroalkyl aromatics involving Et3N·3HF as the fluorine source with/without other nucleophiles. The reaction exhibits good functional group tolerance, and is amenable to gram-scale synthesis. Moreover, an electrochemical reduction of the benzyl C–F bond was also achieved.
The introduction of fluorine atoms and fluorinated building blocks into organic molecules is increasingly being exploited as fluoro-organics show special importance in pharmaceuticals, agrochemicals, and functional materials owing to their unique physical, chemical, and biological properties.1 Monofluorinated (–CFH–), difluoroakylated (–CF2–), and trifluoromethylated (–CF3) aromatic compounds have gained significant synthetic effort on account of their applications in drug discovery.2 However, practical and reliable methods for the longer-chain perfluoroalkylated aromatics are still limited, thus only a few biologically active compounds that contain the perfluoroalkyl group with different types of fluorine distribution have been reported to date.3 Therefore, the development of effective synthetic protocols for the generation of aromatics with the structurally versatile fluoroalkyl group is highly desirable in drug library design and drug discovery.
gem-Difluoroalkenes were found to be an attractive source of fluorinated building blocks for the generation of fluoro-organics via mono-defluorination, fluorine retention and mono-fluorine introduction functionalization.4 To date, the methods for employing an additional fluorine source to gem-difluoroalkenes are merely the fluorine-addition reactions to the synthesis of CF3-containing molecules (Scheme 1a). In 1997, the nucleophilic fluorination of gem-difluoroalkenes to generate α-trifluorocarbanion was disclosed by Burton and co-workers.5 Subsequently, this protocol was elegantly expanded to palladium-catalyzed allylic alkylation and the transformation involving carbanion intercepted by electrophiles.6 Later, Hu and co-workers demonstrated, for the first time, the AgF-mediated fluorometalation of gem-difluoroalkenes to form a C–AgI bond and its rapid homolysis to gain a radical intermediate, which was further applied to palladium-catalyzed fluorinative allylation/arylation and radical trapping process.7 Recently, Feng et al. disclosed a photoredox initiated fluoride anion nucleophilic addition to oxidized gem-difluoroalkenes for delivering α-CF3 benzyl radical intermediate.8 Another method for incorporating fluorine into gem-difluoroalkenes is employing a F+ source, which was developed by Wang and Hu groups for the generation of an α-trifluorocation participating Ritter-type amination and fluoro-hydroxylation reactions, respectively.9 Given the general demand for organofluorides, we explored novel and green protocols to deliver various perfluoroalkyl molecules by using readily available gem-difluorostyrenes.
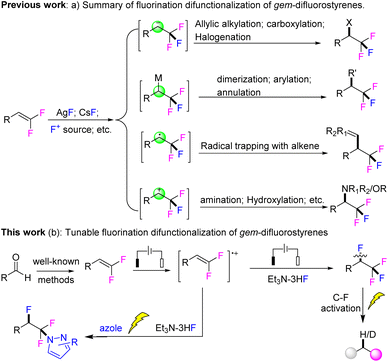 |
| Scheme 1 Overview of methodologies for fluorination difunctionalization of gem-difluorostyrenes. | |
Recently, electrosynthesis has been rediscovered as a sustainable and practical alternative to traditional reagent-based organic transformations with numerous advantages, such as mild and green reaction conditions, high functional group tolerance, and innate scalability.10 As the ongoing interest of our group in the direct anodic oxidative functionalization of arenes and development of green methods to generate fluorosynthons,11 we describe the realization of a novel electrooxidative fluorination-difunctionalization strategy for selectively synthesizing versatile fluoroalkyl aromatics from gem-difluoroalkenes (Scheme 1b). In addition, an electrochemical reduction of the C–F bond was also disclosed.
As a prelude to our studies in the electrochemical fluoro-functionalization of gem-difluorostyrenes, we chose 4-(2,2-difluorovinyl)-1,1′-biphenyl (1a) as the model substrate to explore the difunctionalization process. As outlined in Table 1, the reaction conditions were initially set by utilizing Et3N·3HF (1.0 mL, from Energy Chemical) as the fluorine source, PhCF3/HFIP mixture (4
:
1) as solvent and 0.50 mmol of 1a. The electrolysis reaction gave the difluorinated 3a in 65% in an undivided cell equipped with graphite as both anode and cathode under 3.5 V constant cell potential and air atmosphere (Table 1, entry 1). When hydrogen fluoride-pyridine was applied as fluoride source, the reaction became slow giving only 53% yield in 4.0 h (entry 2). Both decreasing and increasing the amount of Et3N·3HF gave a slightly lower yield of 3a (entries 3 and 4). Adding additional electrolyte shows negative influence to the reaction (entry 5). The electrode material turned out to be a critical parameter of the electrochemical transformation.12 The influence on the reaction outcome with various electrode combinations was investigated, such as Cgr(graphite)(+)/Pt(−), Pt(+)/Pt(−), Cgl(glassy carbon)(+)/Cgl(−) (entries 6–10). Graphite as anode always exhibits better yield than the other anode materials. Further exploring different solvent systems revealed that the electrolysis transformation could occur for giving relevant low yield of 3a in pure PhCF3 or HFIP than that in the mixture of the two solvents in a proper ratio (entries 11 and 12). The yield of 3a did not show notable increase when a N2 atmosphere was applied to the reaction (entry 13). The control experiment without applied current shows no conversion of 1a, indicating that the anodic oxidation of the gem-difluorostyrenes is crucial for the fluorofunctionalizaton (entry 14).
Table 1 Optimization of reactions conditiona
The substrate scope of the electrochemical difunctionalization of gem-difluorostyrenes was investigated under the optimized conditions and the results are tabulated in Table 2. It was found that the substrates with an electron-rich arene motif generally result in good yield of products (3b, 61%; 3c, 68%; 3d, 71%; 3g, 75%). Electrically neutral aryl difluoroalkene delivered the difluorination product in relatively lower yield (3d, 55%) and the tetra substituted one also achieved the difluorination reaction with better yield (3f, 65%). Spontaneous nucleophilic fluorination occurred in a reversible manner for electron-poor gem-difluorostyrenes, which resulted in a low yield of the electrochemical difunctionalization product (3h, 31%), and the formation of 4-(2,2,2-trifluoroethyl)benzonitrile as byproduct.13 On extending the aromatic conjugated structure, the electron-withdrawing groups, such as CF3, OCF3, and fluorine, are well tolerated and the electrolysis transformation resulted good yields of products (3i–3k). Thiophene substituted aryl difluoroalkene was also compatible and successfully delivered the fluorination product 3l in 35% yield. Notably, ibuprofen derivatives with an electrochemically fragile benzyl position were well compatible for this reagent-free electrochemical difluorination protocol delivering 3m in 55% yield.14 Moreover, the substrate scope can be expanded to heteroaryl gem-difluoroalkenes and the difluoro motif decorated with a functional thiophene ring could conveniently transform to polyfluorinated products in 45% and 30% yield, respectively (3n–3o). In addition, when two gem-difluorostyrenes units were located in a molecule, the reaction could be tunable to react with one or two difluoroalkenes in good yields via adjustment of the added amount of Et3N·3HF (3p–3q).
Table 2 Substrate scope for electro-oxidative difluoronation gem-difluoroalkenea
Reactions were carried out with 1 (0.5 mmol) in the indicated solvent mixture PhCF3/HFIP (4 mL : 1 mL) under air at room temperature by using graphite plate as cathode and anode (1.0 cm × 1.0 cm × 2.0 mm) until the disappearance of 1, about 3.0 h.
Byproduct of 4-(2,2,2-trifluoroethyl)benzonitrile was isolated in 7%.
2.0 mL 2 was used. Yield of the isolated product.
|
|
Furthermore, the reaction was successfully applied to the chemo- and regioselective azo-fluorination of gem-difluoroalkenes via the introduction of azole as the second nucleophile after the necessary adjustments of the electrolysis conditions (Table 3) (see ESI† for details). Interestingly, when both Et3N·3HF and azole were applied as nucleophiles, the electrolysis protocol could selectively install the two distinct functionalities on the two ends of gem-difluorostyrene to deliver 1-(1,1,2-trifluoro-2-arylethyl)-1H-azole as a single isomer (5). 2,2-Difluorovinyl arenes containing various functional substituents, such as ester, OCF3, and CN, could transform to the desired fluoroazolation products in moderate yields (5a–5e, 35–51%). The reaction could also selectively react with one difluoroalkene if two such units are located in the same molecule to give the desired product 5f in 45% yield. 2,2-Difluorovinyl arene with a heterocyclic substituent or heteroaryl gem-difluorostyrene gave relatively lower yields in this electrochemical di-functionalization reaction (5g, 28%; 5h, 18%). From the results, it appeared that the reaction was not sensitive to the electronic nature/structure of azoles. Pyrazole with different substituents (F, Cl, Br, I) or benzotriazole show similar reactivity and afford the fluoroazolation products in up to 51% yield (5i–5m). In addition, by replacing the azole with an alcohol, a relatively weak nucleophile, a diminished selectivity of electrochemical difunctionalization was observed due to similar nucleophilicity of the alcohol versus the fluoride ion under slightly adjusted electrochemical conditions (see ESI† for details). The introduction of alcohols (methanol, ethanol, isopropanol, and cyclopentanol) as the second nucleophile resulted in two inseparable isomers with one-to-one ratio in moderate yield [(2,2,2-trifluoro-1-methoxyethyl)arene/(1,2,2-trifluoro-2-methoxyethyl)arene: 5n/5n′, 55%; 5o/5o′, 52%; 5p/5p′, 45%; 5q/5q′, 33%].
Table 3 Substrate scope for electro-oxidative fluoroazolation gem-difluoroalkenea
Reaction condition: a platinum plate as cathode (1.0 cm × 1.0 cm × 0.1 mm) and a graphite plate as anode (1.0 cm × 1.0 cm × 2.0 mm), constant cell potential, 1 (1.0 equiv., 0.5 mmol), 2 (2.0 equiv., 1.0 mmol), 4 (2.0 equiv., 1.0 mmol), nBu4NBF4 (0.1 M), DCM/HFIP (4 mL : 1 mL), room temperature, air, 5 h. Yield of the isolated product.
|
|
To demonstrate the scalability and practicality of the current reaction, the difluorination reaction was performed on gram scale (Scheme 2a) and compound 3a was obtained on a 1.19 g scale with a yield of 47%. Following the similar mechanism of difluorination, dibromination and dichlorination reactions were unsuccessful. However, using similar electrochemical parameters, dichlorination and dibromination could be successfully introduced to gem-difluorostyrenes via an electrocatalytic radical dichlorination/dibromonation process to give compounds 6/7 in 70% and 69% yield, respectively (Scheme 2b).15 We further investigated the regio- and chemo-selective electrochemical di-functionalization with TMSCN and Et3N·3HF as nucleophiles. The possible fluorocyanization product 8 was detected by GC-MS under the current electrolysis conditions (Fig. S1†). Notably, the benzyl C–F bond of compound 3g can be selectively reduced under electrochemical conditions, leading to hydrodefluorination 9 or deuterination-defluorination 10 in 83% and 81% yield, respectively (Scheme 2c).
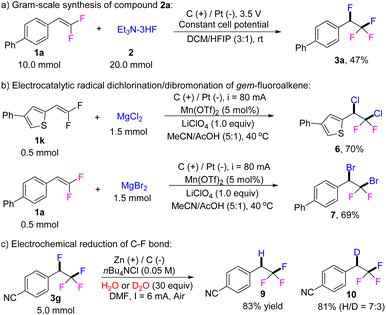 |
| Scheme 2 Further study of this electrochemical transformation. | |
In light of the outstanding versatility of the electro-oxidative fluorination-difunctionalization strategy, we became intrigued with delineating its mode of action. To this end, several control experiments were conducted. Alkyl substituted gem-difluoroalkene 11 as substrate could not give the desired product 12, indicating a conjugated functional group with gem-difluoroalkene is essential in this electrochemical transformation (Scheme 3a). The cyclic voltammetry experiments were performed on the model substrate 1a, revealing the oxidation onset of 1a is 1.15 V vs. SCE (Fig. S1†). Subsequently, two di-fluorination reactions were carried out with 1a at constant anode potential of 1.05 V and 1.30 V versus SCE, respectively (Scheme 3b). The desired product 3a was not detected for electrolysis at 1.05 V, thus supporting the oxidation of gem-difluoroalkene reactant initiates the electrochemical transformation. A radical trapping experiment was further conducted under O2 atmosphere and hydrogen peroxide 13 was detected by GC-MS, which suggested the benzyl radical intermediate of 1a may be involved during the electrochemical di-fluorination process (Scheme 3c). Additionally, a normal styrene derivative, such as 4-vinylbiphenyl, was easy to oxidize in the current electrochemical conditions, while no fluorination reaction could take place (Fig. S4†). On the basis of the current studies and previous reports,8 a plausible pathway for this electrochemical fluorination-difunctionalization is proposed (Scheme 3d). When graphite carbon is used as the working electrode, compound 1 is initially oxidized to give radical cation I. Fluorine anion, as a nucleophile, reacts with Ivia nucleophilic attack at the C1 position to afford benzylic radical II, which is further oxidized to give benzyl cation III. Product 3 can be obtained via subsequent nucleophilic addition by another fluoride ion. On the cathode, hydrogen cations are reduced to provide molecular hydrogen.
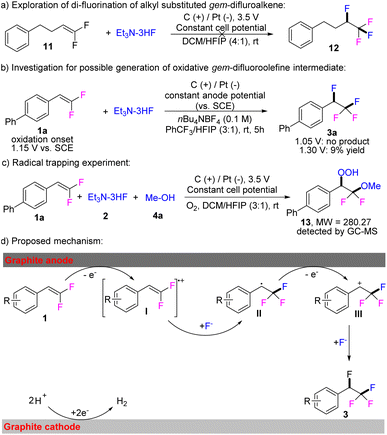 |
| Scheme 3 Mechanism studies. | |
Conclusions
In summary, we present, in this study, a mild and general protocol for the effective fluorination-difunctionalization of gem-difluorostyrene, providing structurally versatile fluoroalkyl building blocks under electrochemical conditions. The reaction exhibits good functional group tolerance, such as halogens, cyano, benzyl, heterocycles, which are synthetically useful handles for further manipulation. In addition, the novel electro-oxidative fluorination-difunctionalization strategies can regio- and chemo-selectively deliver distinct types of long-chain perfluoroalkyl aromatics with different nucleophiles. Moreover, the benzyl C–F bond of the difluorination product can continuously undergo electrochemical modification.
Author contributions
LW conceived the experiment and wrote the first draft of manuscript; BL, ZZ, NZ, and CS synthesized the starting materials and analyzed the data. PF contributed to the conception; PF and HL contributed to the design of experiments and wrote the manuscript.
Conflicts of interest
There are no conflicts to declare.
Acknowledgements
This work was supported by Guangdong Basic and Applied Basic Research Foundation (2023A1515012251), the Key Laboratory of Applied Surface and Colloid Chemistry (Shaanxi Normal University), Ministry of Education, and Jinan University.
Notes and references
-
(a) K. Müller, C. Faeh and F. Diederich, Fluorine in pharmaceuticals: looking beyond intuition, Science, 2007, 317, 1881–1886 CrossRef PubMed
;
(b) S. Purser, P. R. Moore, S. Swallow and V. Gouverneur, Fluorine in medicinal chemistry, Chem. Soc. Rev., 2008, 37, 320–330 RSC
;
(c)
I. Ojima, Fluorine in Medicinal Chemistry and Chemical Biology, Wiley-Blackwell, Chichester, 2009 CrossRef
;
(d) T. Furuya, A. S. Kamlet and T. Ritter, Catalysis for fluorination and trifluoromethylation, Nature, 2011, 473, 470–477 CrossRef CAS
;
(e) Y. Zhou, J. Wang, Z. Gu, S. Wang, W. Zhu, J. L. Acena, V. A. Soloshonok, K. Izawa and H. Liu, Next Generation of Fluorine-Containing Pharmaceuticals, Compounds Currently in Phase II-III Clinical Trials of Major Pharmaceutical Companies: New Structural Trends and Therapeutic Areas, Chem. Rev., 2016, 116, 422–518 CrossRef CAS PubMed
.
-
(a)
T. Hiyama, Organofluorine Compounds: Chemistry and Applications, Springer-Verlag, New York, 2000 CrossRef
;
(b)
P. Kirsch, Modern Fluoro organic Chemistry: Synthesis, Reactivity, Applications, Wiley-VCH, Weinheim, 2004 CrossRef
;
(c) H. Mei, J. Han, S. Fustero, M. Medio-Simon, D. M. Sedgwick, C. Santi, R. Ruzziconi and V. A. Soloshonk, Fluorine-Containing Drugs Approved by the FDA in 2018, Chem. – Eur. J., 2019, 25, 11797 CrossRef CAS
;
(d) O. A. Tomashenko and V. V. Grushin, Aromatic trifluoromethylation with metal complexes, Chem. Rev., 2011, 111, 4475–4521 CrossRef CAS
;
(e) J. Charpentier, N. Frgh and A. Togni, Electrophilic trifluoromethylation by use of hypervalent iodine reagents, Chem. Rev., 2015, 115, 650–682 CrossRef CAS PubMed
.
-
(a) E. Emer, J. Twilton, M. Tredwell, S. Calderwood, T. L. Collier, B. Liegault, M. Taillefer and V. Gouverneur, Diversity-Oriented Approach to CF3CHF-, CF3CFBr-, CF3CF2-, (CF3)2CH-, and CF3(SCF3)CH-Substituted Arenes from 1-(Diazo-2,2,2-trifluoroethyl)arenes, Org. Lett., 2014, 16, 6004–6007 CrossRef CAS PubMed
;
(b) M. Ohashi, N. Ishida, K. Ando, Y. Hashimoto, A. Shigaki, K. Kikushima and S. Ogoshi, CuI-Catalyzed Pentafluoroethylation of Aryl Iodides in the Presence of Tetrafluoroethylene and Cesium Fluoride: Determining the Route to the Key Pentafluoroethyl CuI Intermediate, Chem. – Eur. J., 2018, 24, 9794–9798 CrossRef CAS PubMed
;
(c) N. Taka, H. Koga, H. Sato, T. Ishizawa, T. Takahashi and J. Imagawa, 6-Substituted 2,2-bis(fluoromethyl)-benzopyran-4-carboxamide K+ channel openers, Bioorg. Med. Chem., 2000, 8, 1393–1405 CrossRef CAS PubMed
;
(d) C. Alonso, E. M. de Marigorta, G. Rubiales and F. Palacios, Carbon trifluoromethylation reactions of hydrocarbon derivatives and heteroarenes, Chem. Rev., 2015, 115, 1847–1935 CrossRef CAS PubMed
;
(e) D. M. Ferguson, J. R. Bour, A. J. Canty, J. W. Kampf and M. S. Sanford, Synthesis, Characterization, and Reactivity of Palladium Fluoroenolate Complexes, J. Am. Chem. Soc., 2017, 139, 11662–11665 CrossRef CAS PubMed
.
- For recent advances on transformation of gem-difluoroalkenes, see:
(a) J. P. Sorrentino, D. L. Orsi and R. A. Altman, Acid-Catalyzed Hydrothiolation of gem-Difluorostyrenes to Access α,α-Difluoroalkylthioethers, J. Org. Chem., 2021, 86, 2297–2311 CrossRef CAS PubMed
;
(b) Y. Li, X. Li, X. Li and D. Shi, J. Org. Chem., 2021, 86, 6983–6993 CrossRef CAS PubMed
;
(c) J. Li, S. Liu, R. Zhong, Y. Yang, Y. He, J. Yang, Y. Ma and Z. Wang, Reversal of Regioselectivity in Nucleophilic Difluoroalkylation of α,β-Enones Employing
In Situ-Formed Sterically Encumbered Silylium Catalyst, Org. Lett., 2021, 23, 5859–5864 CrossRef CAS
;
(d) W. Zhu, H. Xi, W. Jiao, L. Huang, L. Wang and J. Wu, Difunctionalization of gem-Difluoroalkenes via Photoredox Catalysis: Synthesis of Diverse α,α-Difluoromethyl-β-alkoxysulfones, Org. Lett., 2022, 24, 720–725 CrossRef CAS PubMed
;
(e) H. Lin, W. Jiao, Z. Chen, J. Han, D. Fang, M. Wang and J. Liao, Enantioselective Cu-Catalyzed Nucleophilic Addition of Fluorinated Reagents: C–C Bond Formation for the Synthesis of Chiral Vicinal Difluorides, Org. Lett., 2022, 24, 2197–2202 CrossRef CAS PubMed
;
(f) D. Bai, Y. Guo, D. Ma, X. Guo and H. Wu, Copper-Catalyzed Hydroamination of gem-Difluoroalkenes Access to Diversified α-Difluoromethyl Amines, Org. Lett., 2023, 25, 533–537 CrossRef CAS
;
(g) Y. T. Liu, Y. H. Fan, Y. Mei, D. J. Li, Y. Jiang, W. H. Yu and F. Pan, Chromium-Catalyzed Defluorinative Reductive Coupling of Aldehydes with gem-Difluoroalkenes, Org. Lett., 2023, 25, 549–554 CrossRef CAS PubMed
;
(h) Y. Wang, X. Qi, Q. Ma, P. Liu and G. C. Tsui, Stereoselective Palladium-Catalyzed Base-Free Suzuki–Miyaura Cross-Coupling of Tetrasubstituted gem-Difluoroalkenes: An Experimental and Computational Study, ACS Catal., 2021, 11, 4799–4809 CrossRef CAS
;
(i) M. Nambo, K. Ghosh, J. C. H. Yim, Y. Tahara, N. Inai, T. Yanai and C. M. Crudden, Desulfonylative Coupling of Alkylsulfones with gem-Difluoroalkenes by Visible-Light Photoredox Catalysis, ACS Catal., 2022, 12, 9526–9532 CrossRef CAS
;
(j) C. Liu, C. Zhu, Y. Cai and H. Jiang, Solvent-Switched Oxidation Selectivities with O2: Controlled Synthesis of α-Difluoro(thio)methylated Alcohols and Ketones, Angew. Chem., Int. Ed., 2021, 60, 12038–12045 CrossRef CAS PubMed
;
(k) F. P. Wu, Y. Yuan, J. Liu and X. F. Wu, Pd/Cu-Catalyzed Defluorinative Carbonylative Coupling of Aryl Iodides and gem-Difluoroalkenes: Efficient Synthesis of α-Fluorochalcones, Angew. Chem., Int. Ed., 2021, 60, 8818–8822 CrossRef CAS PubMed
;
(l) Z. Zhu, L. Lin, J. Xiao and Z. Shi, Nickel-Catalyzed Stereo- and Enantioselective Cross-Coupling of gem-Difluoroalkenes with Carbon Electrophiles by C–F Bond Activation, Angew. Chem., Int. Ed., 2022, 61, e202113209 CrossRef CAS PubMed
;
(m) D. Bai, F. Wu, L. Chang, M. Wang, H. Wu and J. Chang, Highly Regio- and Enantioselective Hydrosilylation of gem-Difluoroalkenes by Nickel Catalysis, Angew. Chem., Int. Ed., 2022, 61, e202114918 CrossRef CAS PubMed
;
(n) K. Xu, Z. Xu, M. Zhang, X. G. Mao, T. Wang, Y. Wu and L. Liu, The aerobic oxidative hydroxysulfurization of gem-difluoroalkenes to produce α,α-difluoro-β-hydroxysulfides, Org. Chem. Front., 2021, 8, 5831–5836 RSC
;
(o) X. Liu, J. Lin, C. Zhuang, J. Zhong, D. Song, J. Zhao and H. Cao, Switchable hydroxysulfonyloxylation and defluorination–decarboxylation sulfonylation of gem-difluoroalkenes with sodium sulfinate via aerobic oxidation, Org. Chem. Front., 2021, 8, 6220–6225 RSC
.
- B. V. Nguyen and D. J. Burton, A New Route for the Preparation of Substituted 2,2-Difluorostyrenes and a Convenient Route to Substituted (2,2,2-Trifluoroethyl)benzenes, J. Org. Chem., 1997, 62, 7758–7764 CrossRef CAS
.
-
(a) P. Tian, C. Q. Wang, S. H. Cai, S. Song, L. Ye, C. Feng and T. P. Loh, F− Nucleophilic-Addition-Induced Allylic Alkylation, J. Am. Chem. Soc., 2016, 138, 15869–15872 CrossRef CAS PubMed
;
(b) W. J. Yoo, J. Kondo, J. A. Rodríguez-Santamaría, T. V. Nguyen and S. Kobayashi, Efficient Synthesis of α-Trifluoromethyl Carboxylic Acids and Esters through Fluorocarboxylation of gem-Difluoroalkenes, Angew. Chem., Int. Ed., 2019, 58, 6772–6775 CrossRef CAS
;
(c) T. Song, C. H. Tung and Z. Xu, Org. Chem. Front., 2022, 9, 2926 RSC
;
(d) C. Liu, C. Zhu, Y. Cai, Z. Yang, H. Zeng, F. Chen and H. Jiang, Fluorohalogenation of gem-Difluoroalkenes: Synthesis and Applications of α-Trifluoromethyl Halides, Chem. – Eur. J., 2020, 26, 1953–1957 CrossRef CAS
.
-
(a) B. Gao, Y. Zhao, C. Ni and C. J. Hu, AgF-Mediated Fluorinative Homocoupling of gem-Difluoroalkenes, Org. Lett., 2014, 16, 102–105 CrossRef CAS PubMed
;
(b) H. J. Tang, L. Z. Lin, C. Feng and T. P. Loh, Palladium-Catalyzed Fluoroarylation of gem-Difluoroalkenes, Angew. Chem., Int. Ed., 2017, 56, 9872–9876 CrossRef CAS PubMed
;
(c) H. J. Tang, Y. F. Zhang, Y. W. Jiang and C. Feng, F− Nucleophilic-Addition-Induced [3 + 2] Annulation: Direct Access to CF3-Substituted Indenes, Org. Lett., 2018, 20, 5190–5193 CrossRef CAS PubMed
;
(d) P. E. Daniel, C. I. Onyeagusi, A. A. Ribeiro, K. Li and S. J. Malcolmson, Palladium-Catalyzed Synthesis of α-Trifluoromethyl Benzylic Amines via Fluoroarylation of gem-Difluoro-2-azadienes Enabled by Phosphine-Catalyzed Formation of an Azaallyl–Silver Intermediate, ACS Catal., 2019, 9, 205–210 CrossRef CAS PubMed
;
(e) T. Y. Lin, Z. Pan, Z. Y. Tu, S. Zhu, H. H. Wu, Y. Liu, Z. Li and J. Zhang, Design and Synthesis of TY-Phos and Application in Palladium-Catalyzed Enantioselective Fluoroarylation of gem-Difluoroalkenes, Angew. Chem., Int. Ed., 2020, 59, 22957–22962 CrossRef CAS PubMed
;
(f) B. Gao, Y. Zhao and J. Hu, AgF-Mediated Fluorinative Cross-Coupling of Two Olefins: Facile Access to α-CF3 Alkenes and β-CF3 Ketones, Angew. Chem., Int. Ed., 2015, 54, 638–642 CrossRef CAS PubMed
.
- H. Liu, L. Ge, D. X. Wang, N. Chen and C. Feng, Photoredox-Coupled F− Nucleophilic Addition: Allylation of gem-Difluoroalkenes, Angew. Chem., Int. Ed., 2019, 58, 3918–3922 CrossRef CAS PubMed
.
-
(a) L. Yang, W. X. Fan, E. Lin, D. H. Tan, Q. Li and H. Wang, Synthesis of α-CF3 and α-CF2H amines via the aminofluorination of fluorinated alkenes, Chem. Commun., 2018, 54, 5907–5910 RSC
;
(b) J. Hu, Y. Yang, Z. Lou, C. Ni and J. Hu, Fluoro-Hydroxylation of gem-Difluoroalkenes: Synthesis of 18O-labeled α-CF3 Alcohols, Chin. J. Chem., 2018, 36, 1202–1208 CrossRef CAS
.
-
(a) J. B. Sperry and D. L. Wright, The application of cathodic reductions and anodic oxidations in the synthesis of complex molecules, Chem. Soc. Rev., 2006, 35, 605–621 RSC
;
(b) R. Francke and R. D. Little, Redox catalysis in organic electrosynthesis: basic principles and recent developments, Chem. Soc. Rev., 2014, 43, 2492–2521 RSC
;
(c) M. Yan, Y. Kawamata and P. S. Baran, Synthetic Organic Electrochemical Methods Since 2000: On the Verge of a Renaissance, Chem. Rev., 2017, 117, 13230–13319 CrossRef CAS PubMed
;
(d) J.-i. Yoshida, A. Shimizu and R. Hayashi, Electrogenerated Cationic Reactive Intermediates: The Pool Method and Further Advances, Chem. Rev., 2017, 118, 4702–4730 CrossRef PubMed
;
(e) Y. Jiang, K. Xu and C. Zeng, Use of Electrochemistry in the Synthesis of Heterocyclic Structures, Chem. Rev., 2017, 118, 4485–4540 CrossRef PubMed
;
(f) S. Tang, Y. Liu and A. Lei, Electrochemical Oxidative Cross-coupling with Hydrogen Evolution: A Green and Sustainable Way for Bond Formation, Chem, 2018, 4, 27–45 CrossRef CAS
;
(g) K. D. Moeller, Using Physical Organic Chemistry To Shape the Course of Electrochemical Reactions, Chem. Rev., 2018, 18, 4817–4833 CrossRef PubMed
;
(h) P. Xiong and H. C. Xu, Chemistry with Electrochemically Generated N-Centered Radicals, Acc. Chem. Res., 2019, 52, 3339–3350 CrossRef CAS PubMed
;
(i) C. Ma, P. Fang, Z. R. Liu, S. S. Xu, K. Xu, X. Cheng, A. W. Lei, H. C. Xu, C. C. Zeng and T. S. Mei, Recent advances in organic electrosynthesis employing transition metal complexes as electrocatalysts, Sci. Bull., 2021, 66, 2412–2429 CrossRef CAS PubMed
;
(j) C. Zhu, N. W. Ang, T. H. Meyer, Y. Qiu and L. Ackermann, Organic Electrochemistry: Molecular Syntheses with Potential, ACS Cent. Sci., 2021, 7, 415–431 CrossRef CAS PubMed
.
-
(a) P. Feng, G. Ma, X. Chen, X. Wu, L. Lin, P. Liu and T. Chen, Electrooxidative and Regioselective C–H Azolation of Phenol and Aniline Derivatives, Angew. Chem., 2019, 131, 8488–8492 CrossRef
;
(b) X. Peng, J. Zhao, G. Ma, Y. Wu, S. Hu, Z. Ruan and P. Feng, Electro-oxidative C–H amination of heteroarenes with aniline derivatives via radical–radical cross coupling, Green Chem., 2021, 23, 8853–8858 RSC
;
(c) X. Wu, G. Ma, X. Peng, Z. Ning, Z. Lin, X. Chen, Y. Tang and P. Feng, Photoredox initiated azole-nucleophilic addition: oxo-azolation of gem-difluoroalkenes, Org. Chem. Front., 2021, 8, 4817–4877 Search PubMed
;
(d) Z. Ning, Z. Zhang, Q. Yan, N. Zhou, L. Wen, X. Peng and P. Feng, Electrochemically time-dependent oxidative coupling/coupling-cyclization reaction between heterocycles: tunable synthesis of polycyclic indole derivatives with fluorescence properties, Sci. China: Chem., 2022, 65, 1962–1967 CrossRef CAS
;
(e) X. Peng, L. Wen, Z. Ning, Z. Zhang, C. Sun, Y. Tang and P. Feng, Electrochemically controlled dearomative 2,3-difunctionalization of indoles to synthesize oxoindoline derivatives, Org. Chem. Front., 2022, 9, 3800–3806 RSC
;
(f) Y. Liang, N. Zhou, G. Ma, L. Wen, X. Wu and P. Feng, Tunable alkoxy-nucleophilic addition under photochemical condition: Dioxidation of gem-difluoroalkenes with O2, Mol. Catal., 2022, 528, 112373 CrossRef CAS
;
(g) L. Wen, N. Zhou, Z. Zhang, C. Liu, S. Xu, P. Feng and H. Li, Electrochemical difunctionalization of gem-difluoroalkenes: a metal-free synthesis of α-difluoro(alkoxyl/azolated)methylated ethers, Org. Lett., 2023, 25, 3308–3313 CrossRef CAS PubMed
.
- D. M. Heard and A. J. Lennox, Electrode Materials in Modern Organic Electrochemistry, Angew. Chem., Int. Ed., 2020, 59, 18866–18884 CrossRef CAS PubMed
.
- P. J. Riss and F. I. Aigbirhio, A simple, rapid procedure for nucleophilic radiosynthesis of aliphatic [18F]trifluoromethyl groups, Chem. Commun., 2011, 47, 11873–11875 RSC
.
-
(a) Z. Ruan, Z. Huang, Z. Xu, S. Zeng, P. Feng and P. H. Sun, Late-stage azolation of benzylic C-H bonds enabled by electrooxidation, Sci. China: Chem., 2021, 64, 800–807 CrossRef CAS
;
(b) W. Wei, Y. Zhong, Y. Feng, L. Gao, H. Tang, Y. Pan, X. Ma and Z. Mo, Electrochemically Mediated Direct C(sp3)–H Sulfonylation of Xanthene Derivatives, Adv. Synth. Catal., 2022, 364, 726–731 CrossRef CAS
;
(c) Z. W. Hou, D. J. Liu, P. Xiong, X. L. Lai, J. Song and H. C. Xu, Site-Selective Electrochemical Benzylic C–H Amination, Angew. Chem., Int. Ed., 2021, 60, 2943–2947 CrossRef CAS PubMed
.
- N. Fu, G. Sauer and S. Lin, Electrocatalytic Radical Dichlorination of Alkenes with Nucleophilic Chlorine Sources, J. Am. Chem. Soc., 2017, 139, 15548–15553 CrossRef CAS PubMed
.
|
This journal is © the Partner Organisations 2024 |
Click here to see how this site uses Cookies. View our privacy policy here.