DOI:
10.1039/D3QO01452E
(Research Article)
Org. Chem. Front., 2024,
11, 149-155
Photoredox dearomative β-hydroborylation of indoles for the synthesis of borylated indolines†
Received
8th September 2023
, Accepted 11th November 2023
First published on 15th November 2023
Abstract
A photocatalysed dearomative β-hydroborylation reaction of indole derivatives with NHC-boranes has been accomplished. This protocol shows a broad substrate scope with moderate to excellent yields, providing a novel and efficient route for the preparation of C2- and C3-borylated indolines, which are important structural units in pharmaceuticals, natural products and functional materials. Control experiments elucidated a radical process initiated by photoinduced energy transfer and single-electron transfer for this transformation. Furthermore, gram-scale reactions demonstrated the synthetic value of this method.
Introduction
Indolines are versatile intermediates in organic synthesis and popular motifs in pharmaceuticals, natural products, and some functional materials (Fig. 1).1 In recent decades, considerable research efforts have been made on developing efficient routes for the construction of indolines and their derivatives.2 Among these developed protocols, indole dearomatization reactions have been proved to be powerful approaches for accessing indolines.3 Until now, a great many strategies including hydrogenation of indoles,4 dearomative arylation/cyclizations of indoles,5 transition-metal- or organocatalyst-catalysed dearomative cycloadditions6,7 and some radical-mediated dearomatizations have been well established.8
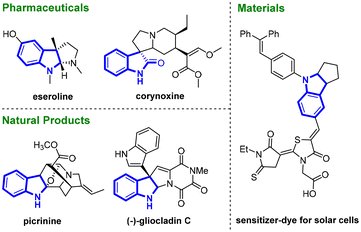 |
| Fig. 1 Examples of indoline-containing compounds. | |
As organoboron compounds are extremely important building blocks in synthetic chemistry, materials science and pharmaceuticals,9–11 recently more efforts have been devoted to the catalytic borylative dearomatization of indoles.12 Among these, transition metal-catalysed dearomative carboboration13 and hydroboration14 have been well developed. For example, the Ito group reported a CuOtBu catalysed dearomative borylation of indole-2-carboxylates, affording a series of C3-borylated chiral indoline derivatives with excellent diastereo- and enantioselectivities (Scheme 1a).14a In 2021, Brown and coworkers disclosed a Ni(DME)Cl2 catalysed regioselective dearomative arylboration of indoles, and various C2- and C3-borylated indolines were prepared (Scheme 1b).14b In 2018, Fontaine and co-workers reported an example of metal-free borylative dearomatization of indoles (Scheme 1c).15 By using ambiphilic aminoborane as a precatalyst, the borylative dearomatization of indoles with pinacolborane (HBpin) was achieved with high conversion and high regio- and diastereoselectivity. However, visible-light-induced borylative dearomatization of indoles has been barely reported so far.
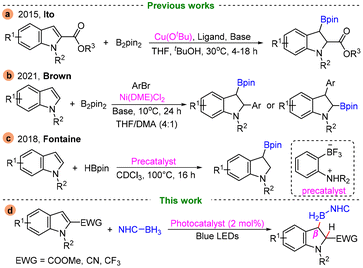 |
| Scheme 1 Dearomative borylation of indoles. | |
In recent years, Lewis base–borane complexes have been demonstrated as a reliable source of boron centered radicals via a hydrogen atom abstraction process in the presence of a radical initiator or a photocatalyst under visible light conditions.16 Due to their low toxicity and ready availability, more and more researchers are starting to use Lewis base–borane as a boron reagent for the construction of C–B bonds, especially N-heterocyclic carbene-boranes (NHC-boranes).17–20 Inspired by previous works on the dearomative hydroborylation of indoles14 and our ongoing research interest in photochemical boron radical chemistry,21 herein we report a visible-light-promoted dearomative β-hydroborylation reaction of indole derivatives and NHC-boranes, which provides an efficient route for preparing C2- or C3-borylated indolines (Scheme 1d).
Results and discussion
Initially, we chose N-Cbz-protected methyl indole-2-carboxylate 1a (1.2 equiv.) as a model substrate to react with NHC-BH32a (1.0 equiv.) under photocatalysis. When Ir(ppy)2(dtbbpy)PF6 was used as the photocatalyst, BnSH as the additive, and Na2CO3 as the base, the dearomative β-hydroborylation product 3a was produced in 86% NMR yield (Table 1, entry 1). Other photocatalysts such as Ru(bpy)3Cl2·6H2O were found to be less effective (Table 1, entry 2). The results of the control experiments confirmed the necessity of Ir(ppy)2(dtbbpy)PF6 and light irradiation (Table 1, entries 3 and 4). However, in the absence of BnSH or Na2CO3, the yield was slightly diminished (Table 1, entries 5 and 6). Next, various bases such as NaOAc, NaOH and DBU were screened (Table 1, entries 7–9), and the NMR yield of 3a was improved slightly to 88% in the presence of NaOAc (Table 1, entry 7). To our satisfaction, when changing the amounts of substrate 1a to 1.0 equiv. and 2a to 1.2 equiv., we obtained product 3a in 96% NMR yield (isolated yield, 95%) with 2.9
:
1 dr (Table 1, entry 10). (For details, please see the ESI.†)
Table 1 Optimization of the reaction conditionsa
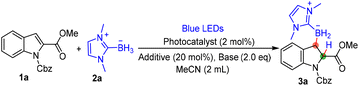
|
Entry |
Photocatalyst |
Additive |
Base |
Yieldb (%) |
Reaction conditions: 1a (1.2 equiv.), 2a (0.2 mmol), photocatalyst (2 mol%), BnSH (20 mol%), base (2.0 equiv.), MeCN (2 mL), 30 W blue LEDs (λ = 440–450 nm), rt, 12 h, N2.
Yields were determined by 1H NMR with triphenyl methane as the internal standard.
In the dark.
1a (0.2 mmol), 2a (1.2 equiv.).
Isolated yield. Ru(bpy)3Cl2·6H2O = tris(2,2′-bipyridyl)ruthenium(II) chloride hexahydrate and Ir(ppy)2(dtbbpy)PF6 = (4,4′-di-tert-butyl-2,2′-bipyridine)bis[(2-pyridinyl)phenyl]iridium(III) hexafluorophosphate. |
1 |
Ir(ppy)2(dtbbpy)PF6 |
BnSH |
Na2CO3 |
86 |
2 |
Ru(bpy)3Cl2·6H2O |
BnSH |
Na2CO3 |
20 |
3 |
— |
BnSH |
Na2CO3 |
nr |
4c |
Ir(ppy)2(dtbbpy)PF6 |
BnSH |
Na2CO3 |
nr |
5 |
Ir(ppy)2(dtbbpy)PF6 |
— |
Na2CO3 |
76 |
6 |
Ir(ppy)2(dtbbpy)PF6 |
BnSH |
— |
80 |
7 |
Ir(ppy)2(dtbbpy)PF6 |
BnSH |
NaOAc |
88 |
8 |
Ir(ppy)2(dtbbpy)PF6 |
BnSH |
NaOH |
41 |
9 |
Ir(ppy)2(dtbbpy)PF6 |
BnSH |
DBU |
79 |
10d |
Ir(ppy)2(dtbbpy)PF6 |
BnSH |
NaOAc |
96(95e) |
With the optimized reaction conditions for the dearomative hydroborylation reaction in hand, a series of indole derivatives and some other heteroaromatic compounds were tested as reaction substrates (Scheme 2). A set of indole-2-carboxylates protected by different substituents including Boc, isopropyl carbonyl and Me were all tolerated, producing the desired borylated indoline derivatives 3b–3d in 67%–97% yields. Gratifyingly, unprotected indole-2-carboxylate 1e also exhibited good reactivity, giving product 3e in 46% yield. The introduction of either electron-withdrawing or electron-donating groups at the ortho, meta, or para position of the benzene ring was tolerated, and the corresponding products 3f–3n were achieved in 30%–95% yields. It was found that when 1m reacted with 2a under the standard conditions, part of the protecting group Cbz on 1m was removed, which was the main reason for the relatively lower yield of 3m. Other types of ester groups like CO2Et and CO2iPr at the C2-position of indoles were all compatible, giving products 3o–3t in good to excellent yields. Remarkably, the borneol-, geraniol-, glucose- and stigmasterol-derived indoles were demonstrated to be amenable substrates, furnishing products 3u–3x in 34%–79% yields, which showcased the late-stage functionalization of bioactive pharmaceuticals and natural products. Subsequently, the effect of other electron-withdrawing groups instead of carboxylates at the C2-position such as CF3 and CN was tested; good applicability was observed and the corresponding products 3y and 3z were achieved in 51%–75% yields. Because of the lack of a stereodirecting group, some of the desired products were achieved as a mixture of diastereomers. Fortunately, the structures of the diastereomers of 3z were confirmed by X-ray diffraction. To further investigate the reactivity, heteroaromatic compounds such as azaindole, benzothiophene and benzofuran were investigated. The expected dearomative hydroborylation products 3aa–3ac were obtained in fair to excellent yields (47%–96%). Compared to 1aa and 1ab, benzofuran 1ac exhibited a more sluggish reactivity, and even when the reaction time was prolonged, there were still lots of benzofuran 1ac remaining. In addition, NHC-boranes bearing iPr, Bn, or tBu on the nitrogen atom were also found to be suitable for this reaction, affording the corresponding products 3ad–3af in good to excellent yields. However, the presence of a large steric hinderance group on NHC-boranes was unfavourable for the reaction; the desired product 3ag could be isolated only in 29% yield. Other types of NHC-boranes such as benzimidazole- or triazole-based boranes could also react with 1a smoothly, offering the corresponding borylated indolines 3ah and 3ai in good yields.
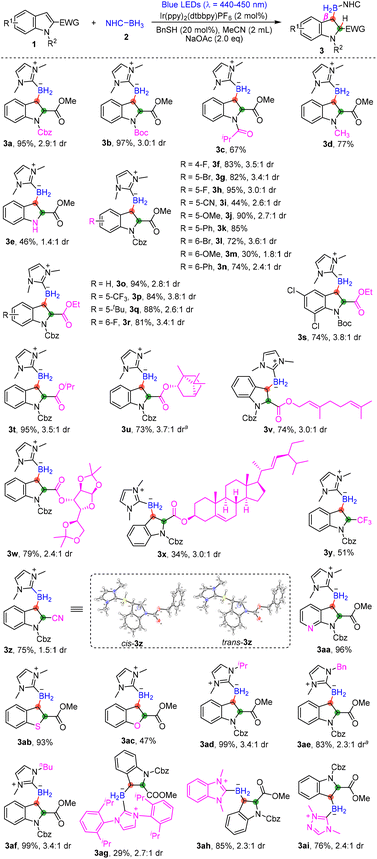 |
| Scheme 2 Substrate scope of 2-substituted indoles. Reaction conditions: 1 (0.2 mmol), 2 (1.2 equiv.), Ir(ppy)2(dtbbpy)PF6 (2 mol%), BnSH (20 mol%), NaOAc (2.0 equiv.), MeCN (2 mL), 30 W blue LEDs (λ = 440–450 nm), rt, 12 h, N2 atmosphere, isolated yields. a The reaction time was 24 h. Cbz = carboxybenzyl, Boc = tert-butyloxycarbonyl. | |
Encouraged by the positive results shown above, we further tested the reactivity of C3-electron-withdrawing-substituted indole derivatives (Scheme 3). Under the standard reaction conditions optimized above, N-Boc-protected methyl indole-3-carboxylate (4a) underwent the dearomative hydroborylation reaction smoothly, leading to the formation of 5a in 95% yield, with a 2.5
:
1 dr. When we performed the reaction in the absence of NaOAc and increased the equivalents of 2a to 2.0, a single diastereoisomer of 5a could be obtained in 99% yield; thus we took these reaction conditions as the optimized ones. To our delight, a variety of C3-carbonyl-functionalized indoles 4 reacted efficiently with NHC-BH32a under the optimal conditions to produce the desired borylated indolines 5b–5n in moderate to excellent yields. It seems that the presence of protective groups can promote the reactions (5a, 5b and 5d–5g). When 4c was employed in this reaction system, it was found that the N-protecting group on 4c could be removed under the standard conditions, and some 4c were converted into 3-CO2Me indole, which could be a reason for the relatively lower yield of 5c. However, the electronic effect of substituent groups on indoles barely had any impact on the reaction efficiency (5h–5n). Other types of electron-withdrawing groups such as CO2Et, CO2tBu, and CN groups at the C3-position of indoles were all tolerated, affording products 5o–5q in excellent yields. Additionally, other C3-carbonyl-functionalized heteroaromatic compounds including azaindole, benzothiophene and benzofuran were also compatible, delivering products 5r–5t in moderate to good yields.
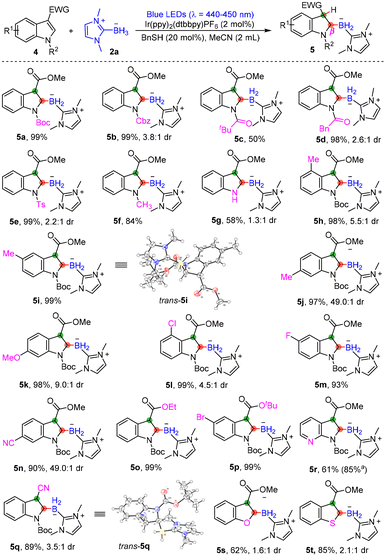 |
| Scheme 3 Substrate scope of 3-substituted indoles. Reaction conditions: 4 (0.2 mmol), 2a (2.0 equiv.), Ir(ppy)2(dtbbpy)PF6 (2 mol%), BnSH (20 mol%), anhydrous MeCN (2 mL), 30 W blue LEDs (λ = 440–450 nm), rt, 12 h, N2 atmosphere, isolated yields. a Yield was determined by 1H NMR with triphenyl methane as the internal standard. | |
In order to gain insight into the mechanism underlying this visible-light-mediated dearomative β-hydroborylation of indole compounds with NHC-boranes, several control experiments were conducted. The reaction was inhibited when TEMPO or 1,1-diphenylethylene was added into the reaction system, and the conjugates (6 and 7) of the radical scavenger and NHC-boryl radical were detected by HRMS (Scheme 4a and b). To find out the source of the H atom at the α-position, isotopic labelling experiments were performed (Scheme 4c and f). The reaction was conducted in CD3CN, and product 3a was obtained in 84% yield without deuteration, which suggests that the solvent CH3CN was not the H source (Scheme 4c). Then we tested the reaction with 10.0 equiv. D2O as an additive under the standard reaction conditions, and the results showed that 3a/3a-d1 was isolated in 83% yield and the H/D ratio was 32
:
68, which indicates that the primary source of the H atom was the residual water in this reaction system (Scheme 4d). Next, NHC-BD3 was synthesized and employed to replace NHC-BH3, and 3a-d2/3a-d3 was isolated in 81% yield with an 87
:
13 H/D ratio, which proves that NHC-BH3 could also be the H atom source (Scheme 4e). Moreover, when BnSD was used instead of BnSH, 3a/3a-d1 was achieved in 95% yield with a 95
:
5 H/D ratio (Scheme 4f), suggesting that BnSH might be a minor source of the H atom. In addition, light/dark experiments showed that product formation occurred only during the period of constant irradiation (Scheme 4g).
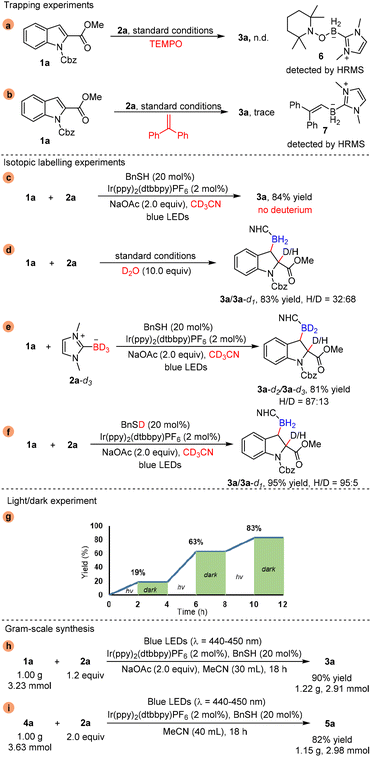 |
| Scheme 4 Mechanism studies and gram-scale synthesis. | |
To illustrate the applicability of this dearomative hydroborylation reaction, gram-scale syntheses of products 3a and 5a were investigated. Two preparative-scale experiments were conducted on a 1.00 g scale of the model reactions (1a, 2a/4a, 2a). To our delight, the reactions proceeded very smoothly to give products 3a and 5a in 90% and 82% yields, respectively (Scheme 4h and i).
Based on the above experimental results and previous reports,17,21 a plausible mechanism for the visible-light-mediated dearomative β-hydroborylation of indole derivatives is proposed, as shown in Fig. 2. Firstly, photoexcitation of the photocatalyst Ir(III) generates its excited state Ir(III)*, which is reductively quenched by NHC-BH32via SET to afford radical cation I′. The deprotonation of the radical cation I′ produces the NHC-boryl radical I. Subsequently, the radical addition of the NHC-boryl radical I at the β-position of indole derivative 1 leads to the formation of radical intermediate II. In path A, intermediate II converts into its anion intermediate IIIvia a single electron reduction. Then protonation of intermediate III affords the desired product 3. In path B, abstraction of the H atom of BnSH by the radical intermediate II takes place to deliver product 3 and the thiyl radical. Then H atom abstraction of NHC-BH32 by the new generated thiyl radical leads to the generation of NHC-boryl radical I. The results of the isotopic experiments (Scheme 4d–f) indicate that path A was the favourable route.
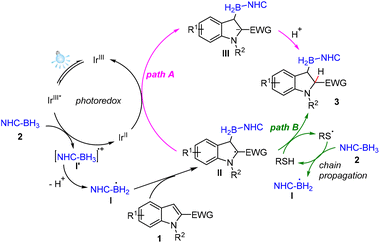 |
| Fig. 2 Proposed mechanism. | |
Conclusions
In conclusion, in this work, we disclosed a visible-light-mediated dearomative β-hydroborylation reaction of indole derivatives with NHC-boranes, offering efficient access to a series of C2- or C3-borylated indolines. A total of 55 examples were developed and large-scale synthesis experiments conducted under the standard conditions were successful, proving the applicability of this protocol. Moreover, a plausible mechanism supported by control experiments and reported works was proposed.
Conflicts of interest
There are no conflicts to declare.
Acknowledgements
The authors gratefully acknowledge the financial support from the National Natural Science Foundation of China (grant no. 22201130) and the Natural Science Foundation of the Jiangsu Higher Education Institutions of China (21KJB150012).
References
-
(a) D. Ni, S. Hu, X. Tan, Y. Yu, Z. Li and L. Deng, Intermolecular formal cycloaddition of indoles with bicyclo[1.1.0]butanes by lewis acid catalysis, Angew. Chem., Int. Ed., 2023, 62, e202308606 Search PubMed
;
(b) D. Li, W. Wang, K. Xu, J. Li, B. Long, Z. Li, G. Tan and X. Yu, Elucidation of a dearomatization route in the biosynthesis of oxysporidinone involving a TenA-like cytochrome P450 enzyme, Angew. Chem., Int. Ed., 2023, 62, e202301976 CrossRef CAS PubMed
;
(c) C. J. Huck, Y. D. Boyko and D. Sarlah, Dearomative logic in natural product total synthesis, Nat. Prod. Rep., 2022, 39, 2231–2291 RSC
;
(d) Y. Wu and W. Zhu, Organic sensitizer from D–π-A to D-A-π-A: effect of the internal electron-withdrawing units on molecular absorption, energy levels and photovoltaic performances, Chem. Soc. Rev., 2013, 42, 2039–2058 RSC
.
-
(a) D. Zhang, H. Song and Y. Qin, Total synthesis of indoline alkaloids: a cyclopropanation strategy, Acc. Chem. Res., 2011, 44, 447–457 CrossRef CAS PubMed
;
(b) G.-J. Mei, W. L. Koay, C. X. A. Tan and Y. Lu, Catalytic asymmetric preparation of pyrroloindolines: strategies and applications to total synthesis, Chem. Soc. Rev., 2021, 50, 5985–6012 RSC
;
(c) K. Nagaraju and D. Ma, Oxidative coupling strategies for the synthesis of indole alkaloids, Chem. Soc. Rev., 2018, 47, 8018–8029 RSC
;
(d) J. Bariwal, L. G. Voskressensky and E. V. Van der Eycken, Recent advances in spirocyclization of indole derivatives, Chem. Soc. Rev., 2018, 47, 3831–3848 RSC
.
-
(a) Y.-Z. Cheng, Z. Feng, X. Zhang and S.-L. You, Visible-light induced dearomatization reactions, Chem. Soc. Rev., 2022, 51, 2145–2170 RSC
;
(b) C. Zheng and S.-L. You, Catalytic asymmetric dearomatization (CADA) reaction-enabled total synthesis of indole-based natural products, Nat. Prod. Rep., 2019, 36, 1589–1605 RSC
.
-
(a) X. Cui, W. Huang and L. Wu, Zirconium-hydride-catalyzed transfer hydrogenation of quinolines and indoles with ammonia borane, Org. Chem. Front., 2021, 8, 5002–5007 RSC
;
(b) C.-B. Yu, J. Wang and Y.-G. Zhou, Facile synthesis of chiral indolines through asymmetric hydrogenation of in situ generated indoles, Org. Chem. Front., 2018, 5, 2805–2809 RSC
;
(c) W. Zhao, Z. Zhang, X. Feng, J. Yang and H. Du, Asymmetric transfer hydrogenation of N-unprotected indoles with ammonia borane, Org. Lett., 2020, 22, 5850–5854 CrossRef CAS PubMed
.
-
(a) W. Zhuang, Y.-Z. Cheng, X.-L. Huang, Q. Huang and X. Zhang, Visible-light induced divergent dearomatization of indole derivatives: controlled access to cyclobutane-fused polycycles and 2-substituted indolines, Org. Chem. Front., 2021, 8, 319–325 RSC
;
(b) X. Fang, S. Gao, Z. Wu, H. Yao and A. Lin, Pd(II)-Catalyzed oxidative dearomatization of indoles: substrate-controlled synthesis of indolines and indolones, Org. Chem. Front., 2017, 4, 292–296 RSC
;
(c) R.-X. Liang, D.-Y. Xu, F.-M. Yang and Y.-X. Jia, A Pd-Catalyzed domino larock annulation/dearomative Heck reaction, Chem. Commun., 2019, 55, 7711–7714 RSC
;
(d) M. Hu, J. Huang, J. Yao, W. Guo, G. Gao, F. Yin, H. Hu, X. Pei, H. Wang and C. Tao, Palladium-catalyzed carbonylative dearomatization of indoles to achieve carbonyl-containing spirocyclic indolenines bearing an all-carbon quaternary center, Org. Lett., 2023, 25, 5951–5956 CrossRef PubMed
.
-
(a) H.-F. Tu, X. Zhang, C. Zheng, M. Zhu and S.-L. You, Enantioselective dearomative prenylation of indole derivatives, Nat. Catal., 2018, 1, 601–608 CrossRef CAS
;
(b) C. Li, B. Zhao, G. Mao and G.-J. Deng, Rhodium(III)-catalyzed mild and regioselective dearomative spirocyclization of 2-aryl-3-nitrosoindoles with alkynes, Chem. Commun., 2023, 59, 7044–7047 RSC
;
(c) K. Liu, G. Xu and J. Sun, Gold-catalyzed stereoselective dearomatization/metal-free aerobic oxidation: access to 3-substituted indolines/oxindoles, Chem. Sci., 2018, 9, 634–639 RSC
.
-
(a) Z.-L. Xia, Q.-F. Xu-Xu, C. Zheng and S.-L. You, Chiral phosphoric acid-catalyzed asymmetric dearomatization reactions, Chem. Soc. Rev., 2020, 49, 286–300 RSC
;
(b) F.-T. Sheng, J.-Y. Wang, W. Tan, Y.-C. Zhang and F. Shi, Progresses in organocatalytic asymmetric dearomatization reactions of indole derivatives, Org. Chem. Front., 2020, 7, 3967–3998 RSC
.
-
(a) L. Wu, Y. Hao, Y. Liu, H. Song and Q. Wang, Visible-light-induced dearomative oxamination of indole derivatives and dearomative amidation of phenol derivatives, Chem. Commun., 2020, 56, 8436–8439 RSC
;
(b) M. S. Oderinde, A. Ramirez, T. G. M. Dhar, L. A. M. Cornelius, C. Jorge, D. Aulakh, B. Sandhu, J. Pawluczyk, A. A. Sarjeant, N. A. Meanwell, A. Mathur and J. Kempson, Photocatalytic dearomative intermolecular [2 + 2] cycloaddition of heterocycles for building molecular complexity, J. Org. Chem., 2021, 86, 1730–1747 CrossRef CAS PubMed
;
(c) X.-L. Huang, Y.-Z. Cheng, X. Zhang and S.-L. You, Photoredox-catalyzed intermolecular hydroalkylative dearomatization of electron-deficient indole derivatives, Org. Lett., 2020, 22, 9699–9705 CrossRef CAS PubMed
;
(d) E. Y. K. Tan, A. S. Mat Lani, W. Sow, Y. Liu, H. Li and S. Chiba, Dearomatization of (hetero)arenes through photodriven interplay between polysulfide anions and formate, Angew. Chem., Int. Ed., 2023, 62, e202309764 CrossRef CAS PubMed
.
-
(a) A. J. J. Lennox and G. C. Lloyd-Jones, Selection of boron reagents for Suzuki-Miyaura coupling, Chem. Soc. Rev., 2014, 43, 412–443 RSC
;
(b) L. Xu, S. Zhang and P. Li, Boron-selective reactions as powerful tools for modular synthesis of diverse complex molecules, Chem. Soc. Rev., 2015, 44, 8848–8858 RSC
;
(c) N. Miyaura and A. Suzuki, Palladium-catalyzed cross-coupling reactions of organoboron compounds, Chem. Rev., 1995, 95, 2457–2483 CrossRef CAS
.
-
(a) L. Ji, R. M. Edkins, A. Lorbach, I. Krummenacher, C. Bruckner, A. Eichhorn, H. Braunschweig, B. Engels, P. J. Low and T. B. Marder, Electron delocalization in reduced forms of 2-(BMes2)pyrene and 2,7-bis(BMes2)pyrene, J. Am. Chem. Soc., 2015, 137, 6750–6753 CrossRef CAS PubMed
;
(b) Y. Zheng, J. Xiong, Y. Sun, X. Pan and J. Wu, Stepwise reduction of 9,10-bis(dimesitylboryl)anthracene, Angew. Chem., Int. Ed., 2015, 54, 12933–12936 CrossRef CAS PubMed
.
-
(a) R. Smoum, A. Rubinstein, V. M. Dembitsky and M. Srebnik, Boron containing compounds as protease inhibitors, Chem. Rev., 2012, 112, 4156–4220 CrossRef CAS PubMed
;
(b) H. Newman, A. Krajnc, D. Bellini, C. J. Eyermann, G. A. Boyle, N. G. Paterson, K. E. McAuley, R. Lesniak, M. Gangar, F. von Delft, J. Brem, K. Chibale, C. J. Schofield and C. G. Dowson, High-throughput crystallography reveals boron-containing inhibitors of a penicillin-binding protein with di- and tricovalent binding modes, J. Med. Chem., 2021, 64, 11379–11394 CrossRef CAS PubMed
.
- L. Chen, J.-J. Shen, Q. Gao and S. Xu, Synthesis of cyclic chiral α-amino boronates by copper-catalyzed asymmetric dearomative borylation of indoles, Chem. Sci., 2018, 9, 5855–5859 RSC
.
-
(a) C. Shen, N. Zeidan, Q. Wu, C. B. J. Breuers, R.-R. Liu, Y.-X. Jia and M. Lautens, Pd-Catalyzed dearomative arylborylation of indoles, Chem. Sci., 2019, 10, 3118–3122 RSC
;
(b) S. Panda and J. M. Ready, Tandem allylation/1,2-boronate rearrangement for the asymmetric synthesis of indolines with adjacent quaternary stereocenters, J. Am. Chem. Soc., 2018, 140, 13242–13252 CrossRef CAS PubMed
;
(c) S. Das, C. G. Daniliuc and A. Studer, Lewis acid catalyzed stereoselective dearomative coupling of indolylboron ate complexes with donor–acceptor cyclopropanes and alkyl halides, Angew. Chem., Int. Ed., 2018, 57, 4053–4057 CrossRef CAS PubMed
;
(d) S. Panda and J. M. Ready, Palladium catalyzed asymmetric three-component coupling of boronic esters, indoles, and allylic acetates, J. Am. Chem. Soc., 2017, 139, 6038–6041 CrossRef CAS PubMed
.
-
(a) K. Kubota, K. Hayama, H. Iwamoto and H. Ito, Enantioselective borylative dearomatization of indoles through copper(I) catalysis, Angew. Chem., Int. Ed., 2015, 54, 8809–8813 CrossRef CAS PubMed
;
(b) G. L. Trammel, R. Kuniyil, P. F. Crook, P. Liu and M. K. Brown, Nickel-catalyzed dearomative arylboration of indoles: regioselective synthesis of C2- and C3-borylated indolines, J. Am. Chem. Soc., 2021, 143, 16502–16511 CrossRef CAS PubMed
.
- A. Jayaraman, L. C. M. Castro, V. Desrosiers and F.-G. Fontaine, Metal-free borylative dearomatization of indoles: exploring the divergent reactivity of aminoborane C–H borylation catalysts, Chem. Sci., 2018, 9, 5057–5063 RSC
.
-
(a) T.-Y. Peng, F.-L. Zhang and Y.-F. Wang, Lewis base-boryl radicals enabled borylation reactions and selective activation of carbon-heteroatom bonds, Acc. Chem. Res., 2023, 56, 169–186 CrossRef CAS PubMed
;
(b) M. Wang and Z. Shi, Methodologies and strategies for selective borylation of C-het and C-C bonds, Chem. Rev., 2020, 120, 7348–7398 CrossRef CAS PubMed
;
(c) S.-H. Ueng, M. M. Brahmi, É. Derat, L. Fensterbank, E. Lacôte, M. Malacria and D. P. Curran, Complexes of borane and N-heterocyclic carbenes: a new class of radical hydrogen atom donor, J. Am. Chem. Soc., 2008, 130, 10082–10083 CrossRef CAS PubMed
.
-
(a) F. Xie, Z. Mao, D. P. Curran, H. Liang and W. Dai, Facile borylation of alkenes, alkynes, imines, arenes and heteroarenes with N-heterocyclic carbene-boranes and a heterogeneous semiconductor photocatalyst, Angew. Chem., Int. Ed., 2023, 62, e202306846 CrossRef CAS PubMed
;
(b) Y.-Q. Miao, X.-Y. Li, Q.-J. Pan, Y. Ma, J.-X. Kang, Y.-N. Ma, Z. Liu and X. Chen, A general photo-induced wide-scope regioselective hydroboration of alkenes without using a photocatalyst or an external initiator, Green Chem., 2022, 24, 7113–7121 RSC
;
(c) G. Li, G. Huang, R. Sun, D. P. Curran and W. Dai, Regioselective radical borylation of α,β-unsaturated esters and related compounds by visible light Irradiation with an organic photocatalyst, Org. Lett., 2021, 23, 4353–4357 CrossRef CAS PubMed
;
(d) C. Zhu, J. Dong, X. Liu, L. Gao, Y. Zhao, J. Xie, S. Li and C. Zhu, Photoredox-controlled β-regioselective radical hydroboration of activated alkenes with NHC-boranes, Angew. Chem., Int. Ed., 2020, 59, 12817–12821 CrossRef CAS PubMed
;
(e) P.-J. Xia, D. Song, Z.-P. Ye, Y.-Z. Hu, J.-A. Xiao, H.-Y. Xiang, X.-Q. Chen and H. Yang, Photoinduced single-electron transfer as an enabling principle in the radical borylation of alkenes with NHC-borane, Angew. Chem., Int. Ed., 2020, 59, 6706–6710 CrossRef CAS PubMed
;
(f) J. Qi, F.-L. Zhang, J.-K. Jin, Q. Zhao, B. Li, L.-X. Liu and Y.-F. Wang, New radical borylation pathways for organoboron synthesis enabled by photoredox catalysis, Angew. Chem., Int. Ed., 2020, 59, 12876–12884 CrossRef CAS PubMed
;
(g) C. Zhu, S. Gao, W. Li and C. Zhu, Photoredox radical borylation of electron-deficient alkenes with NHC-boranes, Chem. Commun., 2020, 56, 15647–15650 RSC
;
(h) Y.-Q. Miao, Q.-J. Pan, Z. Liu and X. Chen, Visible-light-induced 1,2-diphenyldisulfane-catalyzed regioselective hydroboration of electron-deficient alkenes, New J. Chem., 2022, 46, 19091–19094 RSC
.
-
(a) W. Dai, S. J. Geib and D. P. Curran, 1,4-Hydroboration reactions of electron-poor aromatic rings by N-heterocyclic carbene boranes, J. Am. Chem. Soc., 2020, 142, 6261–6267 CrossRef PubMed
;
(b) P. J. Xia, Z.-P. Ye, Y.-Z. Hu, J.-A. Xiao, K. Chen, H.-Y. Xiang, X.-Q. Chen and H. Yang, Photocatalytic C-F bond borylation of polyfluoroarenes with NHC-boranes, Org. Lett., 2020, 22, 1742–1747 CrossRef CAS PubMed
;
(c) K. Takahashi, M. Shimoi, T. Watanabe, K. Maeda, S. J. Geib, D. P. Curran and T. Taniguchi, Revisiting polyfluoroarenes as radical acceptors: radical C−F bond borylation of polyfluoroarenes with N-heterocyclic carbene boranes and synthesis of borane-containing liquid crystals, Org. Lett., 2020, 22, 2054–2059 CrossRef CAS PubMed
;
(d) Z.-L. Chen, C. Empel, K. Wang, P.-P. Wu, B.-G. Cai, L. Li, R. M. Koenigs and J. Xuan, Enabling cyclopropanation reactions of imidazole heterocycles via chemoselective photochemical carbene transfer reactions of NHC-boranes, Org. Lett., 2022, 24, 2232–2237 CrossRef CAS PubMed
.
-
(a) N. Zhou, X.-A. Yuan, Y. Zhao, J. Xie and C. Zhu, Synergistic photoredox catalysis and organocatalysis for inverse hydroboration of imines, Angew. Chem., Int. Ed., 2018, 57, 3990–3994 CrossRef CAS PubMed
;
(b) T. Kawamoto, T. Morioka, K. Noguchi, D. P. Curran and A. Kamimura, Inverse hydroboration of imines with NHC-boranes is promoted by diphenyl disulfide and visible light, Org. Lett., 2021, 23, 1825–1828 CrossRef CAS PubMed
.
- T. Ye, F.-L. Zhang, H.-M. Xia, X. Zhou, Z.-X. Xu and Y.-F. Wang, Stereoselective hydrogen atom transfer to acyclic radicals: a switch enabling diastereodivergent borylative radical cascades, Nat. Commun., 2022, 13, 426–437 CrossRef CAS PubMed
.
- X. Liu, Y. Shen, C. Lu, Y. Jian, S. Xia, Z. Gao, Y. Zheng, Y. An and Y. Wang, Visible-light-driven PhSSPh-catalysed regioselective hydroborylation of α,β-unsaturated carbonyl compounds with NHC-boranes, Chem. Commun., 2022, 58, 8380–8383 RSC
.
|
This journal is © the Partner Organisations 2024 |
Click here to see how this site uses Cookies. View our privacy policy here.