DOI:
10.1039/D3QO01659E
(Research Article)
Org. Chem. Front., 2024,
11, 74-82
Photoredox-catalyzed intramolecular oxy- and aminoacylation of alkenes with acyl oxime esters: facile synthesis of acylated saturated heterocycles†
Received
9th October 2023
, Accepted 7th November 2023
First published on 17th November 2023
Abstract
In the present paper, an efficient photoredox-catalyzed intramolecular oxy- and aminoacylation of internal alkenes equipped with pendant oxygen- or nitrogen-centered nucleophiles with acyl oxime esters is reported. Under visible-light irradiation, a variety of structurally diverse acylated saturated heterocycles, such as tetrahydrofurans, tetrahydropyrans, δ-valerolactones, tetrahydropyrroles, piperidines, tetrahydro-1,3-oxazepines, etc., were efficiently synthesized from easily accessible unsaturated alcohols, carboxylic acids, tosyl-protected amines, and O-homoallyl benzimidates via an acyl radical-mediated cascade acylation/cyclization process. This light-driven transformation features a broad substrate scope, good functional group compatibility, high regio- and diastereoselectivity, and mild reaction conditions, representing a general and practical procedure toward the construction of acylated saturated heterocycles as well as their derivatives.
Introduction
Functionalized saturated heterocycles are valuable core scaffolds that are ubiquitous in biologically active molecules, pharmaceuticals, agrochemicals, and fine chemicals.1 Consequently, the development of a practical and general synthetic approach toward the construction of such structural scaffolds is of increasing interest to the synthetic chemistry community. In particular, in recent decades, in the promotion of electrochemically enabled2 and photoredox-mediated3 annulation, impressive green and efficient synthetic methodologies toward the preparation of versatile heterocyclic compounds have been developed. Among them, radical-initiated cascade cyclizations of alkenes equipped with pendant heteroatom-containing nucleophiles, which not only allow the synthesis of saturated heterocyclic frameworks but also enable the simultaneous introduction of valuable functional groups via a one-pot process, could provide streamlined and facile synthetic protocols to access structurally diverse heterocycles.4 In this regard, in particular, the acyl radical-triggered transformation has emerged as a hot research topic due to the potential derivatization applications of the acyl substituent in heterocyclic compounds.5 To date, several common carbonyl compounds including aromatic aldehydes,6 carboxylic acids,7 anhydrides,8 acyl chlorides,9 α-ketocarboxylic acids,10 and other acyl sources11 have been widely utilized in a series of acyl-radical-involved transformations. Notably, Xu,12 Li,13 and other groups14 successfully developed the acyl radical-driven cyclization reaction of alkenes towards acyl-containing heterocycles. However, despite the impressive advances in this field, it should be noted that these existing methods still face several challenges, for instance, the use of stoichiometric chemical oxidants, poor compatibility of easily oxidizable functional groups under oxidative conditions, and a narrow alkene substrate scope. Undoubtedly, continuous efforts toward the exploration of the acyl radical-involved cyclization of alkenes under mild and environmentally benign conditions to construct structurally varied heterocycles carrying an acyl group are still highly desired.
In 2019, acyl oxime esters were first employed as acyl radical precursors by Wu and coauthors to react with Michael acceptors (i.e., acrylamide) under visible light irradiation, delivering various heterocyclic 2-oxindole derivatives.15 In this reaction, a visible-light-induced single-electron transfer (SET) from a photocatalyst to oxime esters occurs followed by a fast β-fragmentation, affording acyl radicals for the subsequent transformation. Using this elegant acyl formation approach, such precursors would provide fresh opportunities to expand the potential of acyl radical chemistry with regard to both reactivity and selectivity. Following this seminal work, acyl oxime esters have been widely utilized as acylating reagents for visible-light-mediated difunctionalizations of alkenes to generate diverse linear and cyclic carbonyl compounds (Scheme 1a).16 It is worth mentioning that most of the reported examples focused on terminal alkenes while the use of internal alkene derivatives still remains underexplored and challenging, probably due to their relatively low reactivity and the difficulty in controlling their regioselectivity and diastereoselectivity. More recently, we successfully developed an efficient electrochemical intramolecular amino- and oxysulfonylation of internal alkenes equipped with pendant nitrogen or oxygen-based nucleophiles, producing a variety of sulfonylated N-heterocycles and O-heterocycles with high efficiency and excellent diastereoselectivity (Scheme 1b).17 Inspired by our previous work and other pioneering studies, we wondered whether the acyl oxime esters would be amenable for tandem cyclization of internal alkenes bearing inner nucleophiles toward the synthesis of acyl-substituted heterocyclic building blocks. To the best of our knowledge, there is no report on such a tandem reaction of internal alkenes involving the formation of various heterocycles and simultaneous incorporation of valuable acyl groups using a single procedure. Herein, we present a photoredox-catalyzed intramolecular oxy- and aminoacylation of easily accessible internal alkenes, efficiently furnishing structurally diverse acylated saturated heterocycles, such as tetrahydrofurans, tetrahydropyrans, δ-valerolactones, tetrahydropyrroles, piperidines, and tetrahydro-1,3-oxazepines (Scheme 1c).
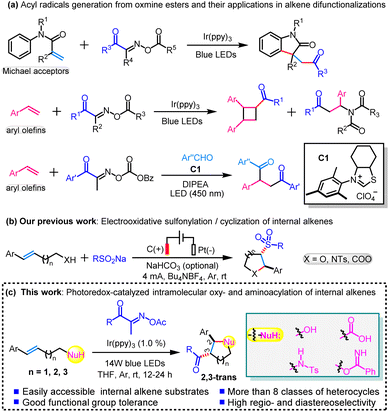 |
| Scheme 1 Research background and summary of this work. | |
Results and discussion
We commenced the reaction optimization using the internal alkene substrate S1 and the easily prepared oxime ester (E)-2-(acetoxyimino)-1-phenylpropan-1-one as the model substrates (Table 1). After efforts toward systematically investigating the reaction conditions, mainly including the photocatalyst, solvent, reaction time, gas atmosphere, etc. (see Table S1† for details of screening studies), we determined the reaction using Ir(ppy)3 (1 mol%) as the catalyst, oxime esters (3.0 equiv.) as the acyl source in tetrahydrofuran under the irradiation of 14 W blue LEDs at room temperature as the standard conditions, which delivered the acylated tetrahydrofuran P1 in 91% NMR yield (Table 1, entry 1). Similar results were obtained when the reaction was conducted in MeCN, acetone, or EtOAc, while the use of MeOH gave a low yield (Table 1, entries 2–5). Other tested photocatalysts, such as Ru(bpy)3Cl2, Ru(bpy)3(PF6)2, Eosin Y, thioxanthone, rhodamine B, and 4CzlPN, were all less effective in promoting the expected reaction compared to the original choice of Ir(ppy)3 (Table 1, entries 6–11). Evaluation of light sources revealed that white light, purple light, red light, and green light could irradiate the reaction to give the expected compound in lower yields (Table 1, entries 12–15). Either O2 or an air atmosphere could dramatically inhibit the expected transformation and caused the decomposition of the starting material S1 to aromatic aldehyde (Table 1, entries 16 and 17). Furthermore, some control experiments showed that both light and photocatalyst were required for this desired transformation, confirming the nature of visible light catalysis (Table 1, entries 18 and 19).
Table 1 Optimization of the reaction conditionsa

|
Entry |
Variation from the standard conditions |
Yieldb (%) |
Reaction conditions: unless mentioned otherwise, all reactions were performed with alkene S1 (0.2 mmol, 1.0 equiv.), (E)-2-(acetoxyimino)-1-phenylpropan-1-one (0.6 mmol, 3.0 equiv.), and Ir(ppy)3 (0.002 mmol, 1 mol%) in 2.0 mL of solvent by using LED irradiation under an argon atmosphere at room temperature.
1H NMR analysis of the reaction mixture determined yields using CH2Br2 as the internal standard.
n.d. = not detected.
|
1 |
None |
91 |
2 |
MeCN instead of THF |
81 |
3 |
Acetone instead of THF |
79 |
4 |
EtOAc instead of THF |
83 |
5 |
MeOH instead of THF |
45 |
6 |
Ru(bpy)3Cl2 instead of Ir(ppy)3 |
17 |
7 |
Ru(bpy)3(PF6)2 instead of Ir(ppy)3 |
10 |
8 |
Eosin Y instead of Ir(ppy)3 |
12 |
9 |
Thioxanthone instead of Ir(ppy)3 |
14 |
10 |
Rhodamine B instead of Ir(ppy)3 |
8 |
11 |
4CzlPN instead of Ir(ppy)3 |
21 |
12 |
White light instead of blue light |
74 |
13 |
Purple light instead of blue light |
65 |
14 |
Red light instead of blue light |
17 |
15 |
Green light instead of blue light |
73 |
16 |
O2 instead of Ar |
41 |
17 |
Air instead of Ar |
64 |
18 |
In the dark |
n.d.c |
19 |
Without Ir(ppy)3 |
n.d.c |
With the optimized conditions in hand, we subsequently investigated the substrate scope of this photochemical transformation. The initial focus was on reacting structurally different unsaturated alcohols with (E)-2-(acetoxyimino)-1-phenylpropan-1-one (Table 2). As exemplified by P1–P17, the reaction worked well over a range of 4-aryl-but-3-en-1-ol to give 3-acyl-substituted tetrahydrofurans in 40%–91% yields with excellent diastereoselectivity. Among these internal alkenes investigated, the obvious electronic effect of the substituents on the aryl ring was observed. In general, internal alkenes with electron-donating groups on the phenyl ring could give much higher yields than those bearing electron-withdrawing groups under the standard conditions. The cyano group and halogen substituents on the phenyl ring of unsaturated alcohols were untouched and endowed the heterocycle with possibilities for further derivatization. On replacing the phenyl group of the 1-aryl-substituted alkene substrate with a naphthyl or a furan substituent, the corresponding cyclized products P16 and P17 were obtained in 83% and 86% yields, respectively. Additionally, when 5-phenylpent-4-en-1-ol and 6-phenylhex-5-en-1-ol were subjected to the photoredox-catalyzed reaction with (E)-2-(acetoxyimino)-1-phenylpropan-1-one, they afforded the acylated tetrahydropyrans and oxepanes, albeit in slightly low yields (P18–P20). Moreover, the reaction was also applicable to 1-diaryl-substituted internal alkene substrates and terminal alkene substrates, affording the corresponding compounds with a quaternary carbon center in moderate to good yields (P21–P27). To further illustrate the synthetic utility of this method, a gram-scale experiment was carried out with (E)-5-(4-butoxyphenyl)pent-4-en-1-ol S1 as an example, affording the desired cyclized product P1 in 70% isolated yield.
Table 2 Substrate scope with different unsaturated alcoholsa
Unless indicated otherwise, all yields are based on the isolated product on a 0.2 mmol scale.
With 1.2 g of the alkene substrate S1.
|
|
To further test the generality of this light-driven cascade acylation/cyclization reaction, diverse acyl oxime esters and internal alkenes bearing different nucleophilic pendants were also examined (Table 3). As exemplified by P28–P36, various aryl and aliphatic acyl precursors could react well with (E)-5-(4-butoxyphenyl)pent-4-en-1-ol, affording the corresponding 3-acyl-substituted tetrahydrofurans in moderate to high yields with excellent diastereoselectivity. As for the aryl acyl subunit of oxime esters, the obvious electronic property and steric effect on aryl substitutions were not observed. Acyl oxime esters bearing electron-donating (p-OMe, m-Me, and o-Me) and electron-withdrawing (p-Br, m-Cl, and o-F) substituents at different positions on the phenyl ring displayed good reactivity to reassemble into the internal alkene. On replacing the phenyl group of the oxime ester substrate with a furan substituent, the corresponding product P35 was obtained in 87% yield. Meanwhile, the structure and relative configuration of compound P35 were unambiguously confirmed by single-crystal X-ray diffraction analysis (CCDC 2286313†). Besides unsaturated alcohols, unsaturated carboxylic acids, tosyl-protected amines, and O-homoallyl benzimidate were also suitable for the photo-catalyzed transformation. These substrates containing 1-aryl-substituted alkene, 1,1-diaryl-substituted alkene, and terminal alkene could react well with (E)-2-(acetoxyimino)-1-phenylpropan-1-one to furnish the corresponding 3-acyl δ-valerolactones (P37–P41), oxepan-2-one (P42), tetrahydropyrroles (P43, P44 and P46), piperidines (P45), and tetrahydro-1,3-oxazepines (P47–P50) in 42%–88% yields.
Table 3 Photoredox-catalyzed synthesis of 3-acyl tetrahydrofurans, tetrahydropyrans, δ-valerolactones, tetrahydropyrroles, piperidines, and tetrahydro-1,3-oxazepinesa
All yields are based on the isolated yield on a 0.2 mmol scale.
|
|
The synthetic utility of the products was demonstrated by the derivatization of the newly-formed acyl group and a Pd-catalyzed Suzuki coupling reaction (Scheme 2). As shown in Scheme 2a, using some well-developed procedures of ketone transformation, the ketocarbonyl of compound P1 could be easily converted to the oxime (P51), amine (P52), alcohol (P53 and P54), and methylene (P55) group, respectively, in acceptable yields. In addition, the halogenated compound P24 could react smoothly with arylboronic acids via a Pd-catalyzed Suzuki coupling reaction to further extend the carbon chain of heterocycles (Scheme 2b). Importantly, the parent heterocycle is untouched in the above-mentioned transformations, which could endow the photoredox-catalyzed acylation/cyclization with more synthetic potential for the construction of structurally complex and diverse heterocycles.
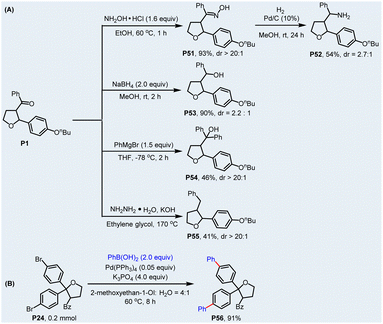 |
| Scheme 2 Synthetic transformations of acyl heterocycles. | |
To better understand the reaction process, several mechanism experiments were carried out, as depicted in Scheme 3. First, we observed that the E-alkene S1 could undergo isomerization to give the thermodynamically less stable Z-alkene in an almost quantitative isolated yield under light irradiation (Scheme 3A). When the reaction was performed in 1 h, 2 h, and 24 h under the standard conditions, both the Z-alkene substrate and the E-alkene substrate could deliver the desired product P1 with similar yields and diastereoselectivities. Meanwhile, the use of E-alkene substrate could generate a trace amount of the Z-alkene at the initial stage of the reaction, which was gradually consumed as the reaction progressed (Scheme 3B). These results showed that the isomerization reaction of partial E-alkene followed by subsequent transformation could be involved in the visible light-mediated reaction. Second, when a radical scavenger (TEMPO, BHT, and 1,1-diphenyethylene) was added into the reaction system, all these reactions were dramatically inhibited under the otherwise standard conditions, resulting in a trace amount of compound P1 and the simultaneously delivery of adducts of the acyl radical (Scheme 3C). These control experiments revealed that the acyl radical could be involved in the light-mediated transformation. Next, a light on–off experiment was conducted. It was found that the reaction could not proceed in the absence of light, revealing that the reaction may proceed through a catalytic radical reaction instead of a radical chain pathway (Scheme 3D).
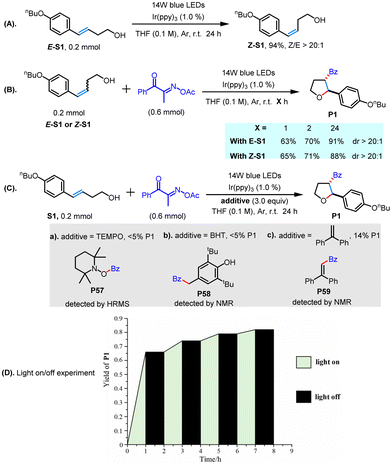 |
| Scheme 3 Mechanistic study. | |
On the basis of the mechanistic experiments presented above and related studies in this field,15–17 a possible mechanism for this photoredox-catalyzed intramolecular oxy- and aminoacylation of internal alkenes with oxime esters is proposed, as depicted in Scheme 4. First, under visible-light irradiation, IrIII(ppy)3 is excited to the photoexcited catalyst. The latter could not only drive the isomerization of the thermodynamic E-alkene to the less stable Z-isomer via an energy transfer pathway but also reduce the acyl oxime esters (E1/2 IrIV/IrIII* = −1.73 vs. SCE for Ir(ppy)3, Ered1/2 = −1.67 V vs. SCE for aliphatic acyl oxime ester (E)-3-(acetoxyimino)butan-2-one, Ered1/2 = −1.42 V vs. SCE for aryl acyl oxime ester (E)-2-(acetoxyimino)-1-phenylpropan-1-one)16avia single-electron transfer to deliver the radical anion I and the IrIV intermediate. Second, the fragmentation of the N–O bond in the radical anion I formed the nitrogen-based radical intermediate II along with an acetoxyl anion species, which could serve as a base in the following transformation. The radical intermediate II could undergo fast C–C bond homolysis to generate the acyl radical III and the acetonitrile molecule. Third, the highly active acyl radical III preferentially attacks the C2 position of the E-alkene or the Z-alkene and generates the stabilized benzylic radical IV, which could be further oxidized viaIrIV(Path A) or an acyl oxime ester (Path B) to the benzylic carbocation V. During the production of intermediate V, the newly-formed photocatalyst IrIII(Path A) or anion I(Path B) could be used for subsequent transformation. Considering that the reaction could not proceed further in the absence of light in the above light on–off experiment, path B may be ruled out. Next, the benzylic carbocation intermediate V was intramolecularly attacked by the nitrogen or oxygen-centered nucleophiles to give the acyl-substituted saturated heterocycles.
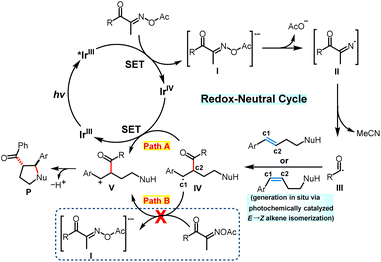 |
| Scheme 4 Proposed reaction mechanism. | |
Conclusions
In summary, we have developed a photoredox-catalyzed intramolecular oxy- and aminoacylation of internal alkenes equipped with pendant nucleophiles with acyl oxime esters. Using acyl oxime esters as the precursor of acyl radicals and bases, this light-induced cascade acylation/cyclization process proceeds smoothly under mild conditions without stoichiometric amounts of chemical redox reagents and bases. Through the use of this methodology, a series of acylated saturated O-heterocycles and N-heterocycles, such as tetrahydrofurans, tetrahydropyrans, δ-valerolactones, tetrahydropyrroles, piperidines, tetrahydro-1,3-oxazepines, etc., were prepared in moderate to good yields with excellent regio- and diastereoselectivity from easily accessible unsaturated alcohols, carboxylic acids, tosyl-protected amines and O-homoallyl benzimidates. In addition, the gram-scale preparation and derivatization experiments of products demonstrated the practicality of this light-driven method. As a beneficial complement to the construction of functionalized heterocycles, this method features broad substrate specificity, good functional group compatibility, excellent regio- and diastereoselectivity, and mild reaction conditions, and will provide a general and practical procedure for the synthesis of acylated five-to-seven saturated heterocycles as well as their derivatives.
Conflicts of interest
There are no conflicts to declare.
Acknowledgements
We acknowledge financial support from the Natural Science Foundation of China (22161056), the Guizhou Science and Technology Department (QKHJC-ZK [2021]-037, QKHPTRC-GCC [2022]032-1, QKHPTRC-CXTD [2022]012), the Zunyi Science and Technology Department (ZSKRPT-2020-5, ZSKRPT-2021-5), and the Zunyi Medical University (202210661194, S202210661093, and ZYDC202301036).
References
-
(a) A. R. Katritzky, Heterocyclic Chemistry: An Academic Subject of Immense Industrial Importance, Chem. Heterocycl. Compd., 1992, 28, 241–259 CrossRef;
(b) Y. Sanemitsu and S. Kawamura, Studies on the Synthetic Development for the Discovery of Novel Heterocyclic Agrochemicals, J. Pestic. Sci., 2008, 33, 175–177 CrossRef CAS;
(c) R. D. Taylor, M. MacCoss and A. D. G. Lawson, Rings in Drugs, J. Med. Chem., 2014, 57, 5845–5859 CrossRef CAS PubMed;
(d) E. Vitaku, D. T. Smith and J. T. Njardarson, Analysis of the Structural Diversity, Substitution Patterns, and Frequency of Nitrogen Heterocycles among U.S. FDA Approved Pharmaceuticals, J. Med. Chem., 2014, 57, 10257–10274 CrossRef CAS PubMed;
(e) F. G. Medina, J. G. Marrero, M. Macías-Alonso, M. C. González, I. Córdova-Guerrero, A. G. T. García and S. Osegueda-Robles, Coumarin Heterocyclic Derivatives: Chemical Synthesis and Biological Activity, Nat. Prod. Rep., 2015, 32, 1472–1507 RSC;
(f) D. G. Brown and J. Boström, Analysis of Past and Present Synthetic Methodologies on Medicinal Chemistry: Where Have All the New Reactions Gone, J. Med. Chem., 2016, 59, 4443–4458 CrossRef CAS PubMed.
-
(a) M. Yan, Y. Kawamata and P. S. Baran, Synthetic Organic Electrochemical Methods Since 2000: On the Verge of a Renaissance, Chem. Rev., 2017, 117, 13230–13319 CrossRef CAS PubMed;
(b) Y. Jiang, K. Xu and C. Zeng, Use of Electrochemistry in the Synthesis of Heterocyclic Structures, Chem. Rev., 2018, 118, 4485–4540 CrossRef CAS;
(c) G. M. Martins, G. C. Zimmer, S. R. Mendes and N. Ahmed, Electrifying Green Synthesis: Recent Advances in Electrochemical Annulation Reactions, Green Chem., 2020, 22, 4849–4870 RSC;
(d) N. Chen and H.-C. Xu, Electrochemical Generation of Nitrogen-Centered Radicals for Organic Synthesis, Green Synth. Catal., 2021, 2, 165–178 CrossRef;
(e) M.-J. Luo, Q. Xiao and J.-H. Li, Electro-/Photocatalytic Alkene-Derived Radical Cation Chemistry: Recent Advances in Synthetic Applications, Chem. Soc. Rev., 2022, 51, 7206–7237 RSC.
-
(a) J. M. R. Narayanam and C. R. J. Stephenson, Visible Light Photoredox Catalysis: Applications in Organic Synthesis, Chem. Soc. Rev., 2011, 40, 102–113 RSC;
(b) C. K. Prier, D. A. Rankic and D. W. C. MacMillan, Visible Light Photoredox Catalysis with Transition Metal Complexes: Applications in Organic Synthesis, Chem. Rev., 2013, 113, 5322–5363 CrossRef CAS PubMed;
(c) J.-R. Chen, X.-Q. Hu, L.-Q. Lu and W.-J. Xiao, Visible Light Photoredox-Controlled Reactions of N-radicals and Radical ions, Chem. Soc. Rev., 2016, 45, 2044–2056 RSC;
(d) N. A. Romero and D. A. Nicewicz, Organic Photoredox Catalysis, Chem. Rev., 2016, 116, 10075–10166 CrossRef CAS PubMed;
(e) K. P. S. Cheung, S. Sarkar and V. Gevorgyan, Visible Light-Induced Transition Metal Catalysis, Chem. Rev., 2022, 122, 1543–1625 CrossRef PubMed;
(f) V. Srivastava, P. K. Singh, S. Tivari and P. P. Singh, Visible Light Photocatalysis in the Synthesis of Pharmaceutically Relevant Heterocyclic Scaffolds, Org. Chem. Front., 2022, 9, 1485–1507 RSC.
-
(a) B. Zhao, X. Peng, Y. Zhu, T. A. Ramirez, R. G. Cornwall and Y. Shi, Cu(I)-Catalyzed Diamination of Conjugated Dienes. Complementary Regioselectivity from Two Distinct Mechanistic Pathways Involving Cu(II) and Cu(III) Species, J. Am. Chem. Soc., 2011, 133, 20890–20900 CrossRef CAS PubMed;
(b) R. Zhu and S. L. Buchwald, Copper-Catalyzed Oxytrifluoromethylation of Unactivated Alkenes, J. Am. Chem. Soc., 2012, 134, 12462–12465 CrossRef CAS PubMed;
(c) S. R. Chemler, S. D. Karyakarte and Z. M. Khoder, Stereoselective and Regioselective Synthesis of Heterocycles via Copper-Catalyzed Additions of Amine Derivatives and Alcohols to Alkenes, J. Org. Chem., 2017, 82, 11311–11325 CrossRef CAS;
(d) Z.-L. Li, G.-C. Fang, Q.-S. Gu and X.-Y. Liu, Recent Advances in Copper-Catalysed Radical-involved Asymmetric 1,2-Difunctionalization of Alkenes, Chem. Soc. Rev., 2020, 49, 32–48 RSC;
(e) X.-Y. Yu, J.-R. Chen and W.-J. Xiao, Visible Light-Driven Radical-Mediated C–C Bond Cleavage/Functionalization in Organic Synthesis, Chem. Rev., 2021, 121, 506–561 CrossRef CAS PubMed;
(f) D. Chen, X. Yang, D. Wang, Y. Li, L. Shi and D. Liang, Electrophotocatalytic Tri- or Difluoromethylative Cyclization of Alkenes, Org. Chem. Front., 2023, 10, 2482–2490 RSC.
-
(a) G. V. Maksim and I. N. Gennady, The Chemistry of Acyl Radicals in Solution, Russ. Chem. Rev., 1971, 40, 916 CrossRef;
(b) D. L. Boger, Applications of Free Radicals in Organic Synthesis, Isr. J. Chem., 1997, 37, 119–129 CrossRef CAS;
(c) C. Chatgilialoglu, D. Crich, M. Komatsu and I. Ryu, Chemistry of Acyl Radicals, Chem. Rev., 1999, 99, 1991–2070 CrossRef CAS.
-
(a) S. Esposti, D. Dondi, M. Fagnoni and A. Albini, Acylation of Electrophilic Olefins through Decatungstate-Photocatalyzed Activation of Aldehydes, Angew. Chem., Int. Ed., 2007, 46, 2531–2534 CrossRef CAS;
(b) J. Wang, C. Liu, J. Yuan and A. Lei, Copper-Catalyzed Oxidative Coupling of Alkenes with Aldehydes: Direct Access to α, β-Unsaturated Ketones, Angew. Chem., Int. Ed., 2013, 52, 2256–2259 CrossRef CAS PubMed;
(c) S. A. Moteki, A. Usui, S. Selvakumar, T. Zhang and K. Maruoka, Metal-Free C–H Bond Activation of Branched Aldehydes with a Hypervalent Iodine(III) Catalyst under Visible-Light Photolysis: Successful Trapping with Electron-Deficient Olefins, Angew. Chem., Int. Ed., 2014, 53, 11060–11064 CrossRef CAS;
(d) S. Mukherjee, R. A. Garza-Sanchez, A. Tlahuext-Aca and F. Glorius, Alkynylation of C (O)–H Bonds Enabled by Photoredox-Mediated Hydrogen-Atom Transfer, Angew. Chem., Int. Ed., 2017, 56, 14723–14726 CrossRef CAS;
(e) X. Zhang and D. W. C. MacMillan, Direct Aldehyde C–H Arylation and Alkylation via the Combination of Nickel, Hydrogen Atom Transfer, and Photoredox Catalysis, J. Am. Chem. Soc., 2017, 139, 11353–11356 CrossRef CAS PubMed;
(f) Y. Jiao, M.-F. Chiou, Y. Li and H. Bao, Copper-Catalyzed Radical Acyl-Cyanation of Alkenes with Mechanistic Studies on the tert-Butoxy Radical, ACS Catal., 2019, 9, 5191–5197 CrossRef CAS.
-
(a) G. Bergonzini, C. Cassani and C.-J. Wallentin, Acyl Radicals from Aromatic Carboxylic Acids by Means of Visible-Light Photoredox Catalysis, Angew. Chem., Int. Ed., 2015, 54, 14066–14069 CrossRef CAS PubMed;
(b) M. Zhang, R. Ruzi, J. Xi, N. Li, Z. Wu, W. Li, S. Yu and C. Zhu, Photoredox-Catalyzed Hydroacylation of Olefins Employing Carboxylic Acids and Hydrosilanes, Org. Lett., 2017, 19, 3430–3433 CrossRef CAS PubMed;
(c) M. Zhang, J. Xie and C. Zhu, A General Deoxygenation Approach for Synthesis of Ketones from Aromatic Carboxylic Acids and Alkenes, Nat. Commun., 2018, 9, 3517 CrossRef PubMed;
(d) L. Zhang, S. Chen, H. He, W. Li, C. Zhu and J. Xie, Photoredox/Nickel-Catalyzed Hydroacylation of Ethylene with Aromatic Acids, Chem. Commun., 2021, 57, 9064–9067 RSC;
(e) S.-C. Ren, X. Yang, B. Mondal, C. Mou, W. Tian, Z. Jin and Y. R. Chi, Carbene and Photocatalyst-Catalyzed Decarboxylative Radical Coupling of Carboxylic Acids and Acyl Imidazoles to form Ketones, Nat. Commun., 2022, 13, 2846 CrossRef CAS PubMed.
-
(a) F. Kazemi, A. R. Kiasat and B. Mombaini, Simple Preparation of Symmetrical Carboxylic Acid Anhydrides by Means of Na2CO3/SOCl2, Synth. Commun., 2007, 37, 3219–3223 CrossRef CAS;
(b) G. Bergonzini, C. Cassani, H. Lorimer-Olsson, J. Hörberg and C.-J. Wallentin, Visible-Light-Mediated Photocatalytic Difunctionalization of Olefins by Radical Acylarylation and Tandem Acylation/Semipinacol Rearrangement, Chem. – Eur. J., 2016, 22, 3292–3295 CrossRef CAS PubMed;
(c) S. Dong, G. Wu, X. Yuan, C. Zou and J. Ye, Visible-Light Photoredox Catalyzed Hydroacylation of Electron-deficient Alkenes: Carboxylic Anhydride as an Acyl Radical Source, Org. Chem. Front., 2017, 4, 2230–2234 RSC;
(d) Y. Zhou, L. Zhao, M. Hu, X.-H. Duan and L. Liu, Visible-Light Photoredox-Catalyzed Divergent 1,2-Diacylation and Hydroacylation of Alkenes with Carboxylic Acid Anhydride, Org. Lett., 2023, 25, 5268–5272 CrossRef CAS;
(e) R. Cao, Y. Liu, X. Shi and J. Zheng, Visible-Light Induced Cross-Electrophile Coupling of Imines and Anhydrides to Synthesize α-Amino Ketones, Chem. Commun., 2023, 59, 10668–10671 RSC.
-
(a) Y. Liu, Z. Chen, Q.-L. Wang, P. Chen, J. Xie, B.-Q. Xiong, P.-L. Zhang and K.-W. Tang, Visible Light-Catalyzed Cascade Radical Cyclization of N-Propargylindoles with Acyl Chlorides for the Synthesis of 2-Acyl-9H-pyrrolo[1,2-a] indoles, J. Org. Chem., 2020, 85, 2385–2394 CrossRef CAS;
(b) L. Bao, Z.-X. Wang and X.-Y. Chen, Metal-Free Generation of Acyl Radical via Photoinduced Single-Electron Transfer from Lewis Base to Acyl Chloride, Org. Lett., 2022, 24, 8223–8227 CrossRef CAS PubMed;
(c) H. Zhang, S. Liang, D. Wei, K. Xu and C. Zeng, Electrocatalytic Generation of Acyl Radicals and Their Applications, Eur. J. Org. Chem., 2022, e202200794 CrossRef CAS;
(d) J.-Q. Chen, X. Luo, M. Chen, Y. Chen and J. Wu, Visible-Light-Induced 1,7-Enyne Dicyclization: Synthesis of Ester-Substituted Benzo[j]phenanthridines, Org. Lett., 2023, 25, 1978–1983 CrossRef CAS.
-
(a) J. Liu, Q. Liu, H. Yi, C. Qin, R. Bai, X. Qi, Y. Lan and A. Lei, Visible-Light-Mediated Decarboxylation/Oxidative Amidation of α-Keto Acids with Amines under Mild Reaction Conditions Using O2, Angew. Chem., Int. Ed., 2014, 53, 502–506 CrossRef CAS;
(b) L. Chu, J. M. Lipshultz and D. W. C. MacMillan, Merging Photoredox and Nickel Catalysis: The Direct Synthesis of Ketones by the Decarboxylative Arylation of α-Oxo Acids, Angew. Chem., Int. Ed., 2015, 54, 7929–7933 CrossRef CAS;
(c) H. Huang, G. Zhang and Y. Chen, Dual Hypervalent Iodine(III) Reagents and Photoredox Catalysis Enable Decarboxylative Ynonylation under Mild Conditions, Angew. Chem., Int. Ed., 2015, 54, 7872–7876 CrossRef CAS;
(d) H. Tan, H. Li, W. Ji and L. Wang, Sunlight-Driven Decarboxylative Alkynylation of α-Keto Acids with Bromoacetylenes by Hypervalent Iodine Reagent Catalysis: A Facile Approach to Ynones, Angew. Chem., Int. Ed., 2015, 54, 8374–8377 CrossRef CAS;
(e) F. Penteado, E. F. Lopes, D. Alves, G. Perin, R. G. Jacob and E. J. Lenardão, α-Keto Acids: Acylating Agents in Organic Synthesis, Chem. Rev., 2019, 119, 7113–7278 CrossRef CAS;
(f) H.-L. Zhu, F.-L. Zeng, X.-L. Chen, K. Sun, H.-C. Li, X.-Y. Yuan, L.-B. Qu and B. Yu, Acyl Radicals from α-Keto Acids: Metal-Free Visible-Light-Promoted Acylation of Heterocycles, Org. Lett., 2021, 23, 2976–2980 CrossRef CAS;
(g) Y.-Y. Cheng, H.-Y. Hou, Y. Liu, J.-X. Yu, B. Chen, C.-H. Tung and L.-Z. Wu, α-Acylation of Alkenes by a Single Photocatalyst, Angew. Chem., Int. Ed., 2022, 61, e202208831 CrossRef CAS;
(h) X. Ji, Z. Yang, X. Wu, G.-J. Deng and H. Huang, Photoredox Neutral Decarboxylative Hydroxyalkylations of Heteroarenes with α-Keto Acids, J. Org. Chem., 2022, 87, 4168–4182 CrossRef CAS PubMed.
-
(a) M. Ociepa, O. Baka, J. Narodowiec and D. Gryko, Light-Driven Vitamin B12-Catalysed Generation of Acyl Radicals from 2-S-Pyridyl Thioesters, Adv. Synth. Catal., 2017, 359, 3560–3565 CrossRef CAS;
(b) L. Capaldo, R. Riccardi, D. Ravelli and M. Fagnoni, Acyl Radicals from Acylsilanes: Photoredox-Catalyzed Synthesis of Unsymmetrical Ketones, ACS Catal., 2018, 8, 304–309 CrossRef CAS;
(c) G. Goti, B. Bieszczad, A. Vega-Peñaloza and P. Melchiorre, Stereocontrolled Synthesis of 1,4-Dicarbonyl Compounds by Photochemical Organocatalytic Acyl Radical Addition to Enals, Angew. Chem., Int. Ed., 2019, 58, 1213–1217 CrossRef CAS;
(d) Q. Wu, Y.-H. Zhao, C. Lu-Lu, H.-Y. Li and H.-X. Li, Metal-free Photocleavage of the C(non-acyl)–S bond of Thioesters for Regioselective Pyridylthioesterification of Styrenes, Org. Chem. Front., 2022, 9, 2977–2985 RSC;
(e) Z. Zhang, Y. Tian, X. Li, Z. Wang, R. Liu, P. Chen, X. Li, J. Dai and D. Shi, S-Trifluoromethyl Thioesters as Bifunctional Reagents for Acyl-Trifluoromethylthiolation of Alkenes and 1,3-Enynes via Photoredox/Copper Dual Catalysis, Green Chem., 2023, 25, 2723–2729 RSC.
-
(a) S.-M. Xu, J.-Q. Chen, D. Liu, Y. Bao, Y.-M. Liang and P.-F. Xu, Aroyl Chlorides as Novel Acyl Radical Precursors via Visible-Light Photoredox Catalysis, Org. Chem. Front., 2017, 4, 1331–1335 RSC;
(b) Y.-L. Wei, J.-Q. Chen, B. Sun and P.-F. Xu, Synthesis of Indolo[2,1-a] Isoquinoline Derivatives via Visible-Light-Induced Radical Cascade Cyclization Reactions, Chem. Commun., 2019, 55, 5922–5925 RSC;
(c) Q.-S. Zhao, G.-Q. Xu, H. Liang, Z.-Y. Wang and P.-F. Xu, Aroylchlorination of 1,6-Dienes via a Photoredox Catalytic Atom-Transfer Radical Cyclization Process, Org. Lett., 2019, 21, 8615–8619 CrossRef CAS PubMed.
- M.-B. Zhou, R.-J. Song, X.-H. Ouyang, Y. Liu, W.-T. Wei, G.-B. Deng and J.-H. Li, Metal-free Oxidative Tandem Coupling of Activated Alkenes with Carbonyl C(sp2)–H Bonds and Aryl C(sp2)–H Bonds Using TBHP, Chem. Sci., 2013, 4, 2690–2694 RSC.
-
(a) M. Ji, L. Xu, X. Luo, M. Jiang, S. Wang, J.-Q. Chen and J. Wu, Alkoxycarbonyl Radicals from Alkyloxalyl Chlorides: Photoinduced Synthesis of Isoquinolinediones under Visible Light Irradiation, Org. Chem. Front., 2021, 8, 6704–6709 RSC;
(b) D.-Y. Yang, L. Liu, J.-Y. Gu, Y.-H. He and Z. Guan, Photoredox Catalyzed Radical Cascade Aroylation (Sulfonylation)/Cyclization Enables Access to Fused Indolo-pyridones, J. Org. Chem., 2021, 86, 18042–18055 CrossRef CAS PubMed;
(c) J.-Q. Chen, X. Tu, B. Qin, S. Huang, J. Zhang and J. Wu, Synthesis of Ester-Substituted Indolo[2,1-a] Isoquinolines via Photocatalyzed Alkoxycarbonylation/Cyclization Reactions, Org. Lett., 2022, 24, 642–647 CrossRef CAS PubMed;
(d) W.-H. Rao, Q. Li, L.-L. Jiang, Y.-G. Li, P. Xu, X.-W. Deng, M. Li, G.-D. Zou and X. Cao, Photoredox-Catalyzed Acyl Lactonization of Alkenes with Aldehydes: Synthesis of Acyl Lactones, J. Org. Chem., 2022, 87, 14194–14207 CrossRef CAS PubMed;
(e) H. He, Q. Wan, Z.-W. Hou, Q. Zhou and L. Wang, Organoelectrophotocatalytic Generation of Acyl Radicals from Formamides and Aldehydes: Access to Acylated 3-CF3-2-Oxindoles, Org. Lett., 2023, 25, 7014–7019 CrossRef CAS;
(f) W.-H. Rao, Q. Li, Y.-G. Li, L.-L. Jiang, M.-X. Yue, G.-D. Zou and X. Cao, Visible-Light Photoredox-Catalyzed Acyl Lactonization of Alkenes with Acyl Chlorides, Eur. J. Org. Chem., 2023, e202300191 CrossRef CAS;
(g) H.-X. Xiao, P.-P. Li, B. Pan, W. Liang, F. Du and Y. Yu, Photoinduced, Silver(I)-Mediated Synthesis of Ester-Substituted Fused Quinazolinones via Cascade Alkoxycarbonylation/Cyclization of Heterocycles Bearing Unactivated Alkenes, J. Org. Chem., 2023, 88, 5861–5874 CrossRef CAS PubMed.
- X. Fan, T. Lei, B. Chen, C.-H. Tung and L.-Z. Wu, Photocatalytic C–C Bond Activation of Oxime Ester for Acyl Radical Generation and Application, Org. Lett., 2019, 21, 4153–4158 CrossRef CAS.
-
(a) Y.-Y. Cheng, T. Lei, L. Su, X. Fan, B. Chen, C.-H. Tung and L.-Z. Wu, Visible Light Irradiation of Acyl Oxime Esters and Styrenes Efficiently Constructs β-Carbonyl Imides by a Scission and Four-Component Reassembly Process, Org. Lett., 2019, 21, 8789–8794 CrossRef CAS;
(b) P.-Z. Wang, Y. Gao, J. Chen, X.-D. Huan, W.-J. Xiao and J.-R. Chen, Asymmetric Three-Component Olefin Dicarbofunctionalization Enabled by Photoredox and Copper Dual Catalysis, Nat. Commun., 2021, 12, 1815 CrossRef CAS;
(c) Y. Guo, P.-F. Huang, Y. Liu and B.-H. He, Visible-Light-Induced Acylation/Cyclization of Active Alkenes: Facile Access to Acylated Isoquinolinones, Org. Biomol. Chem., 2022, 20, 3767–3778 RSC;
(d) S. Jin, X. Sui, G. C. Haug, V. D. Nguyen, H. T. Dang, H. D. Arman and O. V. Larionov,
N-Heterocyclic Carbene-Photocatalyzed Tricomponent Regioselective 1,2-Diacylation of Alkenes Illuminates the Mechanistic Details of the Electron Donor–Acceptor Complex-Mediated Radical Relay Processes, ACS Catal., 2022, 12, 285–294 CrossRef CAS;
(e) Y.-C. Liu, P. Chen, X.-J. Li, B.-Q. Xiong, Y. Liu, K.-W. Tang and P.-F. Huang, Visible-Light-Induced Dual Acylation of Alkenes for the Construction of 3-Substituted Chroman-4-ones, J. Org. Chem., 2022, 87, 4263–4272 CrossRef CAS;
(f) Z.-T. Luo, J.-H. Fan, B.-Q. Xiong, Y. Liu, K.-W. Tang and P.-F. Huang, Visible-Light-Induced Acylation/Arylation of Alkenes via Aryl Migration/Desulfonylation, Eur. J. Org. Chem., 2022, e202200793 CrossRef CAS.
-
(a) X.-Q. Mou, L.-C. Ren, M. Zhang, M. Wang, Y.-F. Jin, Q.-X. Guan, A. Cai, S.-M. Zhang, H. Ren, Y. Zhang and Y.-Z. Chen, Complementary Copper-Catalyzed and Electrochemical Aminosulfonylation of O-Homoallyl Benzimidates and N-Alkenyl Amidines with Sodium Sulfinates, Org. Lett., 2022, 24, 1405–1411 CrossRef CAS PubMed;
(b) X.-Q. Mou, L.-C. Ren, M. Wang, H.-H. Zhang, A. Cai, K.-X. Wan, S.-M. Zhang, B.-D. Cui, Y. Zhang and Y.-Z. Chen, Electrochemically Enabled Intramolecular Amino- and Oxysulfonylation of Alkenes with Sodium Sulfinates to Access Sulfonylated Saturated Heterocycles, J. Org. Chem., 2023, 88, 3238–3253 CrossRef CAS.
Footnotes |
† Electronic supplementary information (ESI) available: Details of the reaction condition screening, experimental procedures, spectra data of products, and single crystal data of product 35. CCDC 2286313. For ESI and crystallographic data in CIF or other electronic format see DOI: https://doi.org/10.1039/d3qo01659e |
‡ These authors contributed equally. |
|
This journal is © the Partner Organisations 2024 |
Click here to see how this site uses Cookies. View our privacy policy here.