DOI:
10.1039/D3RA08133H
(Paper)
RSC Adv., 2024,
14, 2369-2379
Novel chalcone-based crown ethers: synthesis, characterization, antioxidant activity, biological evaluations, and wastewater remediation†
Received
28th November 2023
, Accepted 29th December 2023
First published on 11th January 2024
Abstract
Macrocycles play a pivotal and indispensable role within the realms of both medicine and industry. In the course of our research endeavors, we have successfully synthesized five distinct macrocyclic chalcone entities, each showcasing remarkable biological and anti-oxidative properties. Furthermore, these compounds exhibit exceptional promise as potent agents for the removal of dyes in wastewater treatment processes. The synthesis of these key constituents was achieved through the judicious application of the Robinson ether synthesis and Claisen–Schmidt condensation reactions. The structures of compounds 1a–f and 2a–e were characterized by using analytical techniques such as FTIR, 1H NMR, 13C NMR, and DEPT 13C NMR spectroscopy. These macrocycles also underwent in vitro assessments to measure their antibacterial activity using the agar well diffusion method. The results revealed that the macrocyclics were more sensitive to Gram-positive than Gram-negative bacteria. For example, compound 2d exhibited an inhibition zone of 20 mm at 150 ppm. The antioxidant activity as determined via the DPPH method established that all tested compounds showed moderate radical-scavenging ability. Specifically, compound 2e (at 1000 ppm) exhibited antioxidant activity of 79% inhibition of radicals, in comparison to 90% for the standard ascorbic acid. The latter was demonstrated by using methylene blue as an adsorbate under simulated wastewater conditions. Outstandingly, the most effective compounds were 2d and 2c, which achieved removal rates of 96.54% and 92.37%, respectively, for methylene blue dye.
1. Introduction
A macrocycle is a giant cyclic molecule that contains a ring of seven or more atoms.1 Macrocycles play an important role in the field of medicine, and researchers have explored the incorporation of various heteroatoms into their macrocyclic structures. These heteroatoms offer a diverse range of functional groups that enhance the versatility and therapeutic potential of these compounds.2 Macrocycles are also significant in various critical applications due to their potential biological activity,3 anti-cancer,4 anti-oxidation,5 and anti-inflammatory effects,6 and a variety of physical,7 environmental,8 and industrial applications.9
Many macromolecules are composed of bis-aldehydes and bis-ketones. Chalcone moiety macrocycles10 and macrocycles with other functional groups11 have been previously prepared and tested in the aforementioned fields, yielding acceptable results.12 Their potential to address existing challenges in the above-mentioned areas is evident from the magnitude of their impact. To address human health concerns and mitigate the influence of harmful microorganisms, researchers are actively promoting the development and synthesis of novel biologically active compounds. This has become a crucial aspect of scientific research requirements. The exploration of new compounds with beneficial physiological effects, particularly through extensive studies on macrocycles, has led to the emergence of various methods for their preparation.13 Chalcone moiety macrocycles are considered useful organic compounds due to their wide range of applications in the fields of health,14 as anti-inflammatory, anti-bacterial, and anti-viral agents. The most important uses in the environmental field include contaminant removal from wastewater,15 and in industry, they are used as catalysts in chemical reactions.16
Water resource contamination is a highly contested global issue due to its severe and often lasting impact on living organisms.17 Among the various pollutants, the presence of dyes in water is particularly concerning because they can alter the natural appearance of water even at very low concentrations. There is a great deal of environmental pollution of dyes due to the vast array of existing dyes and an annual production reaching approximately 1.6 million tons. Methylene blue (MB) is the most commonly used dye and was first synthesized in 1876 by Heinrich Caro of Badische Anilin and Soda-Fabrik (BASF). Initially created as an aniline-based synthetic dye for coloring cotton in the textile industry, it was soon recognized for its utility in staining and deactivating microbial species.18
Based on this, we created a series of macrocyclic compounds containing two chalcone groups and four ether groups via adducting both Robinson ether synthesis reactions to prepare bis-aldehyde and bis-ketone intermediate linkages.19 The Claisen–Schmidt condensation reaction was used to condense linkages and obtain five macrocycle chalcone moieties as the final products.20 We proceeded to perform a series of tests aimed at assessing their biological activities, antioxidative properties, and their efficacy in purifying water-containing pollutants. To elucidate the chemical structures of the intermediates and final products, a comprehensive array of analytical techniques, including Fourier transform infrared (FTIR), proton (1H) NMR, carbon-13 (13C) NMR, and distortionless enhancement by polarization transfer (DEPT) 13C NMR spectroscopy, was employed.
2. Materials and methods
2.1 General
Melting points (m.p.) were determined using an Electrothermal 9100 instrument (UK) with open capillaries. Compound purity was verified through a recrystallization method. Infrared (IR) spectra were acquired with a Shimadzu IR Affinity-1 spectrophotometer, employing potassium bromide (KBr) as the medium. 1H-NMR and 13C-NMR spectra were recorded on a Brucker DRX-300 (400 MHz) instrument, using tetramethylsilane (TMS) as the internal standard and chloroform (CHCl3) as the solvent. Chemical shifts (δ) are reported in parts per million (ppm). Absorption spectra were collected using a Shimadzu UV-visible double-beam spectrophotometer (model UV1800, Japan) with a fixed 1 nm bandwidth, and a 1 cm quartz cell was used for spectrophotometric measurements.
2.2 Chemistry
2.2.1 Procedure for synthesis of bis-acetophenone and bis-benzaldehyde compounds (1a–f). Through the Robinson ether synthesis, 2 equivalents (20 mmol) of acetophenones or benzaldehydes and 1 equivalent (10 mmol) of dibromo alkanes were refluxed in ethanol in the presence of 3 equivalents of K2CO3 for 8 hours. The completion of the reaction was periodically monitored by FTIR spectroscopy. Afterward, the cooled solution was poured into cold distilled water, resulting in the immediate formation of solid materials. The described product was filtered, washed several times with distilled water and cold ethanol, dried, and then recrystallized in ethanol to obtain white crystals of bis-acetophenones and bis-benzaldehydes with a yield of 81–88%.21,22
2.2.1.1 Synthesis of 2,2′-(hexane-1,6-diylbis(oxy))dibenzaldehyde. (C20H22O4), m.p. (77.1–78.1), yield (81%); IR (cm−1) str., 1681.93 (C
O), 1597 (C
C). 1H-NMR (δ, ppm): 1.59 (p, 4H, H10,10′), 1.09 (p, 4H, H9,9′), 4.09 (t, 4H, H8,8′), 6.98 (combinate dd, 2H, H2,2′), 7.00 (combinate td, 2H, H4,4′), 7.53 (td, 2H, H3,3′), 7.81 (dd, 2H, H5,5′), 10.50 (s, 2H, H7,7′), 13C-NMR (δ, ppm): 25.86: C10,10′, 29.06: C9,9′, 68.26: C8,8′, 112.48: C2,2′, 120.58: C4,4′, 124.87: C6,6′, 128.29: C5,5′, 136.00: C3,3′, 161.46: C1,1′, 189.84: C7,7′. 13C-DEPT-135 (δ, ppm): 25.86: C10,10′, 29.06: C9,9′, 68.26: C8,8′, 112.48: C2,2′, 120.58: C4,4′, 128.29: C5,5′, 136.00: C3,3′, 189.84: C7,7′.
2.2.1.2 Synthesis of (E)-2,2′-(but-2-ene-1,4-diylbis(oxy))dibenzaldehyde. (C18H16O4), m.p. (137.7–138.7), yield (73%); IR (cm−1) str., 1697.36 (C
O), 1602.85 (C
C). 1H-NMR (δ, ppm): 4.72 (q, 4H, H8,8′), 6.16 (p, 2H, H9,9′), 6.99 (dd 2H, H2,2′), 7.05 (td, 2H, H4,4′), 7.55 (td, 2H, H3,3′), 7.85 (dd, 2H, H5,5′), 10.50 (s, 2H, H7,7′), 13C-NMR (δ, ppm): 67.98: C8,8′, 112.76: C2,2′, 121.10: C4,4′, 125.10: C6,6′, 127.86: C9,9′, 128.71: C5,5′, 135.92: C3,3′, 160.67: C1,1′, 189.64: C7,7′. 13C-DEPT-135 (δ, ppm): 67.98: C8,8′, 112.76: C2,2′, 121.10: C4,4′, 127.86: C9,9′, 128.71: C5,5′, 135.92: C3,3′, 189.64: C7,7′.
2.2.1.3 Synthesis of 2,2′-(butane-1,4-diylbis(oxy))dibenzaldehyde. (C18H18O4), m.p. (112.9–113.9), yield (80%); IR (cm−1) str., 1676.14 (C
O), 1600.92 (C
C). 1H-NMR (δ, ppm): 2.09 (p, 4H, H9,9′), 4.18 (t, 4H, H8,8′), 6.98 (dd, 2H, H2,2′), 7.02 (td, 2H, H4,4′), 7.53 (td, 2H, H3,3′), 7.80 (dd, 2H, H5,5′), 10.49 (s, 2H, H7,7′). 13C-NMR (δ, ppm): 25.93: C9,9′, 67.85: C8,8′, 112.39: C2,2′, 120.77: C4,4′, 124.84: C6,6′, 128.51: C5,5′, 136.03: C3,3′, 161.17: C1,1′, 189.59: C7,7′. 13C-DEPT-135 (δ, ppm): 25.93: C9,9′, 67.85: C8,8′, 112.38: C2,2′, 120.77: C4,4′, 128.50: C5,5′, 136.04: C3,3′, 189.60: C7,7′.
2.2.1.4 Synthesis of 1,1′-((hexane-1,6-diylbis(oxy))bis(5-fluoro-2,1-phenylene))bis(ethan-1-one). (C22H24F2O4), m.p. (120.9–121.9), yield (78%); IR (cm−1) str., 1668.43 (C
O), 1612.49 (C
C). 1H-NMR (δ, ppm): 1.58 (p, 4H, H11,11′), 1.89 (p, 4H, H10,10′), 2.62 (s, 6H, H8,8′), 4.04 (t, 4H, H9,9′), 6.89 (d, 2H, H2,2′), 7.13 (dd, 2H, H3,3′), 7.45 (d, 2H, H5,5′). 13C-NMR (δ, ppm): 25.99: C11,11′, 29.17: C10,10′, 31.95: C8,8′, 68.92: C9,9′, 113.55: C5, 113.63: C5′, 116.41: C2, 116.65: C2′, 119.95: C3, 120.18: C3′, 128.93: C6, 128.98: C6′, 154.63: C4, 154.65: C4′, 155.43: C1, 157.82: C1′, 198.39: C7, 198.40: C7′. 13C-DEPT-135 (δ, ppm): 25.99: C11,11′, 29.17: C10,10′, 31.96: C8,8′, 68.92: C9,9′, 113.55: C5, 113.62: C5′, 116.41: C2, 116.65: C2′, 119.96: C3, 120.18: C3′.
2.2.1.5 Synthesis of 1,1′-((hexane-1,6-diylbis(oxy))bis(2,1-phenylene))bis(ethan-1-one). (C22H26O4), m.p. (112.8–113.3), yield (85%); IR (cm−1) str., 1662.64 (C
O), 1593.20 (C
C). 1H-NMR (δ, ppm): 1.59 (p, 4H, H11,11′), 1.90 (p, 4H, H10,10′), 2.62 (s, 6H, H8,8′), 4.07 (t, 4H, H9,9′), 6.94 (combine dd, 2H, H2,2′), 6.97 (combine td, 2H, H4,4′), 7.43 (td, 2H, H3,3′), 7.73 (dd, 2H, H5,5′). 13C-NMR (δ, ppm): 26.02: C11,11′, 29.17: C10,10′, 32.08: C8,8′, 68.24: C9,9′, 112.24: C2,2′, 120.47: C4,4′, 128.27: C6,6′, 130.40: C5,5′, 133.70: C3,3′, 158.39: C1,1′, 199.93: C7,7′. 13C-DEPT-135 (δ, ppm): 26.02: C11,11′, 29.17: C10,10′, 32.08: C8,8′, 68.24: C9,9′, 112.24: C2,2′, 120.47: C4,4′, 130.41: C5,5′, 133.70: C3,3′.
2.2.1.6 Synthesis of 1,1′-((ethane-1,2-diylbis(oxy))bis(5-fluoro-2,1-phenylene))bis(ethan-1-one). (C18H16F2O4), m.p. (115.9–116.7), yield (81%); IR (cm−1) str., 1670.35 (C
O), 1610.56 (C
C).
2.2.2 Procedure for synthesis of macrocyclic chalcone-moiety compounds (2a–e). Through a hybrid of high dilution and the Claisen–Schmidt procedure, an equivalent amount (2.5 mmol) of bis-acetophenone and bis-benzaldehyde was refluxed overnight in 250 mL of a mixture of 75% CH3OH and 25% H2O in the presence of 10 equivalents of KOH. The reaction mixture was stirred at room temperature for an additional 7 days. The completion of the reaction was monitored through FTIR spectroscopy at various time points. The volume was reduced to approximately 50 mL using a rotary evaporator, and then filtered and washed several times with distilled water. It was then dried and recrystallized in xylene.23,24
2.2.2.1 Synthesis of (15E,31E)-19,28-difluoro-6,7,8,9,23,24-hexahydro-17H,30H-tetrabenzo[e,j,r,w][1,4,12,17]tetraoxacyclotetracosine-17,30-dione. (C36H30F2O6), m.p. (167.7–168.5), yield (72%); IR (cm−1) str., 1653.00 (C
O), 1587.42 (C
C). 1H-NMR (δ, ppm): 1.85 (p, 4H, H18,18′), 3.95 (p, 4H, H17,17′), 4.21 (t, 4H, H16,16′), 6.69 (dd, 2H, H2,2′), 6.76 (td, 2H, H4,4′), 6.91 (d, 2H, H14,14′), 7.04 (td, 2H, H3,3′), 7.13 (dd, 2H, H13,13′), 7.36 (d, 2H, H11,11′), 7.40 (dd, 2H, H5,5′), 7.62 (d, 2H, H8,8′), 7.71 (d, 2H, H7,7′). 13C-NMR (δ, ppm): 26.20: C18,18′, 67.94: C17,17′, 68.56: C16,16′, 111.93: C11,11′, 115.39: C2, 115.47: C2′, 115.98: C14, 116.23: C14′, 117.94: C4, 118.17: C4′, 121.07: C13,13′, 123.33: C10,10′, 127.92: C8,8′, 130.51: C3,3′, 131.46: C6,6′, 132.07: C5,5′, 141.65: C7,7′, 152.41: C15,15′, 155.96: C1,1′, 158.03: C12,12′, 193.95: C9,9′. 13C-DEPT-135 (δ, ppm): 26.20: C18,18′, 67.93: C17,17′, 68.55: C16,16′, 111.92: C11,11′, 115.39: C2, 115.46: C2′, 115.99: C14, 116.23: C14′, 117.95: C4, 118.18: C4′, 121.07: C13,13′, 127.91: C8,8′, 130.52: C3,3′, 132.08: C5,5′, 141.65: C7,7′.
2.2.2.2 Synthesis of (17E,33E)-21,30-difluoro-6,7,8,9,10,11,25,26-octahydro-19H,32H-tetrabenzo[e,j,t,y][1,4,12,19]tetraoxacyclohexacosine-19,32-dione. (C38H34F2O4), m.p. (182.5–183.5), yield (74%); IR (cm−1) str., 1651.07 (C
O), 1595.13 (C
C). 1H-NMR (δ, ppm): 1.49 (p, 4H, H19,19′), 2.73 (p, 4H, H18,18′), 3.96 (t, 4H, H17,17′), 4.29 (t, 4H, H16,16′), 6.75 (td, 2H, H4,4′), 6.83 (dd, 2H, H2,2′), 6.87 (d, 2H, H14,14′), 6.94 (td, 2H, H3,3′), 7.24 (dd, 2H, H13,13′), 7.29 (d, 2H, H11,11′), 7.45 (dd, 2H, H5,5′), 7.53 (d, 2H, H8,8′), 7.82 (d, 2H, H7,7′). 13C-NMR (δ, ppm): 26.23: C19,19′, 28.84: C18,18′, 68.33: C17,17′, 68.70: C16,16′, 112.03: C11,11′, 115.21: C2, 115.29: C2′, 116.71: C14, 116.95: C14′, 118.60: C4, 118.83: C4′, 120.56: C13,13′, 123.69: C10,10′, 127.51: C8,8′, 130.20: C3,3′, 131.18: C6,6′, 131.71: C5,5′, 140.72: C7,7′, 153.13: C15,15′, 156.06: C1,1′, 158.25: C12,12′, 191.77: C9,9′. 13C-DEPT-135 (δ, ppm): 26.24: C19,19′, 28.84: C18,18′, 68.33: C17,17′, 68.69: C16,16′, 112.02: C11,11′, 115.20: C2, 115.27: C2′, 116.72: C14, 116.96: C14′, 118.61: C4, 118.84: C4′, 120.56: C13,13′, 127.50: C8,8′, 130.20: C3,3′, 131.71: C5,5′, 140.72: C7,7′.
2.2.2.3 Synthesis of (18Z,26Z,34Z)-6,7,8,9,10,11,25,28-octahydro-17H,36H-tetrabenzo[a1,g,l,v][1,6,14,21]tetraoxacyclooctacosine-17,36-dione. (C40H38O6), m.p. (191.7–192.5), yield (70%); IR (cm−1) str., 1664.57 (C
O), 1598.99 (C
C). 1H-NMR (δ, ppm): 1.21 (p, 4H, H20,20′), 1.47 (p, 4H, H19,19′), 3.94 (t, 4H, H18,18′), 4.62 (d, 4H, H16,16′), 5.97 (t, 2H, H17,17′), 6.89 (dd, 4H, H2,2′,14,14′), 6.95 (td, 2H, H4,4′), 7.01 (td, 2H, H3,3′), 7.31 (td, 2H, H12,12′), 7.40 (m, 4H, H8,8′,13,13′), 7.55 (dd, 4H, H5,5′,11,11′), 7.79 (d, 2H, H7,7′). 13C-NMR (δ, ppm): 25.68: C18,18′, 28.73: C17,17′, 68.04: C16,16′, 68.35: C19,19′, 112.46: C2,2′, 112.74: C14,14′, 120.59: C12,12′, 121.16: C4,4′, 124.47: C6,6′, 127.94: C8,8′, 128.64: C11,11′, 129.65: C3,3′, 130.02: C10,10′, 130.06: C20,20′, 131.35: C13,13′, 132.38: C5,5′, 138.90: C7,7′, 157.36: C15,15′, 157.46: C1,1′, 194.73: C9,9′. 13C-DEPT-135 (δ, ppm): 25.68: C18,18′, 28.73: C17,17′, 68.04: C16,16′, 68.35: C19,19′, 112.46: C2,2′, 112.74: C14,14′, 120.59: C12,12′, 121.16: C4,4′, 127.94: C8,8′, 128.64: C11,11′,129.66: C3,3′, 130.06: C20,20′, 131.35: C13,13′, 132.39: C5,5′, 138.90: C7,7′.
2.2.2.4 Synthesis of (6E,24E)-3,28-difluoro-13,14,15,16,17,18,32,33,34,35,36,37-dodecahydro-5H,26H-tetrabenzo[c1,i,n,x][1,8,16,23]tetraoxacyclotriacontine-5,26-dione. (C42H42F2O6), m.p. (159.2–160.2), yield (72%); IR (cm−1) str., 1651.07 (C
O), 1587.42 (C
C). 1H-NMR (δ, ppm): 1.17 (p, 4H, H21,21′), 1.39 (p, 4H, H18,18′), 1.52 (p, 4H, H20,20′), 1.80 (p, 4H, H17,17′), 3.72 (t, 4H, H19,19′), 4.04 (t, 4H, H16,16′), 6.75 (dd, 2H, H2,2′), 6.87 (d, 2H, H14,14′), 6.91 (d, 2H, H13,13′), 7.10 (td, 2H, H4,4′), 7.21 (td, 2H, H3,3′), 7.24 (d, 2H, H8,8′), 7.32 (d, 2H, H11,11′), 7.51 (dd, 2H, H5,5′), 7.83 (d, 2H, H7,7′). 13C-NMR (δ, ppm): 25.68: C18,18′, 25.89: C21,21′, 28.79: C17,17′, 28.87: C20,20′, 68.24: C16,16′, 69.22: C19,19′, 112.30: C11,11′, 113.92: C2, 114.00: C2′, 116.27: C14, 116.51: C14′, 118.35: C4, 118.58: C4′, 120.53: C13,13′, 123.85: C10,10′, 127.39: C8,8′, 129.00: C3,3′, 130.98: C6,6′, 131.69: C5,5′, 139.63: C7,7′, 153.52: C15,15′, 154.53: C1,1′, 157.92: C12, 158.15: C12′, 192.93: C9,9′. 13C-DEPT-135 (δ, ppm): 25.68: C18,18′, 25.89: C21,21′, 28.79: C17,17′, 28.87: C20,20′, 68.24: C16,16′, 69.22: C19,19′, 112.30: C11,11′, 113.92: C2, 113.99: C2′, 116.27: C14, 116.51: C14′, 118.36: C4, 118.59: C4′, 120.53: C13,13′, 127.39: C8,8′, 129.00: C3,3′, 131.69: C5,5′, 139.63: C7,7′.
2.2.2.5 Synthesis of (18E,34E)-2,15-difluoro-6,7,8,9,10,11,25,26,27,28-decahydro-17H,36H-tetrabenzo[a1,g,l,v][1,6,14,21]tetraoxacyclooctacosine-17,36-dione. (C40H38F2O6), m.p. (193.2–193.9), yield (78%); IR (cm−1) str., 1651.07 (C
O), 1579.70 (C
C). 1H-NMR (δ, ppm): 1.18 (p, 4H, H18,18′), 1.42 (p, 4H, H17,17′), 1.96 (p, 4H, H20,20′), 3.76 (t, 4H, H16,16′), 4.10 (t, 4H, H19,19′), 6.75 (dd, 2H, H2,2′), 6.85 (d, 2H, H14,14′), 6.88 (d, 2H, H13,13′), 7.04 (td, 2H, H4,4′), 7.22 (td, 2H, H3,3′), 7.24 (d, 2H, H8,8′), 7.31 (d, 2H, H11,11′), 7.49 (dd, 2H, H5,5′), 7.81 (d, 2H, H7,7′). 13C-NMR (δ, ppm): 25.87: C18,18′, 26.21: C17,17′, 28.88: C20,20′, 68.27: C16,16′, 69.26: C19,19′, 112.15: C11,11′, 113.85: C2, 113.92: C2′, 116.39: C14, 116.62: C14′, 118.49: C4, 118.72: C4′, 120.68: C13,13′, 123.79: C10,10′, 127.38: C8,8′, 128.97: C3,3′, 130.98: C6,6′, 131.80: C5,5′, 139.19: C7,7′, 153.60: C15,15′, 154.54: C1,1′, 157.92: C12,12′, 192.62: C9,9′. 13C-DEPT-135 (δ, ppm): 25.87: C18,18′, 26.21: C17,17′, 28.88: C20,20′, 68.27: C16,16′, 69.25: C19,19′, 112.15: C11,11′, 113.84: C2, 113.92: C2′, 116.39: C14, 116.63: C14′, 118.49: C4, 118.72: C4′, 120.68: C13,13′, 127.38: C8,8′, 128.97: C3,3′, 131.80: C5,5′, 139.19: C7,7′.
2.3 Antioxidant activity
The experimental procedure was conducted following a previously published protocol,25 with minor adjustments. The assessment of the antioxidant properties of compounds and vitamin C was conducted by measuring their ability to scavenge free radicals using the stable radical DPPH. In a 96-well plate, 40 μL of various concentrations (125, 250, 500, and 1000 μg mL−1) of compounds was mixed with 160 μL of freshly prepared 1.0 mM DPPH solution in methanol. The mixtures were then vigorously vortexed. Subsequently, the solution was subjected to incubation at ambient temperature under conditions of darkness for a duration of 30 minutes. The measurement of absorbance was conducted at a wavelength of 517 nm using an ELISA reader (800 TS, BioTek, USA). In this experiment, the DPPH reagent, excluding the sample, was employed as a control, whereas methanol was used as a blank solution. The assessment of free radical scavenging activity was quantified as the percentage of inhibition, as calculated by the application of the subsequent formula:
where AC denotes the absorbance of the control, and AS denotes the absorption of compounds.
2.4 Antimicrobial activity
The agar well diffusion test was carried out according to previously published protocols26,27 to assess the antibacterial activity of the compounds against Staphylococcus aureus ATCC 25923 as a Gram-positive bacterium and Escherichia coli ATCC 11229 as a Gram-negative bacterium. The bacteria were uniformly cultured on Mueller–Hinton agar plates by means of a sterile cotton swab inoculated with saline solution containing bacterial strains (adjusted to 0.5 McFarland standards, which are equal to 1 × 108 CFU mL−1). After the plates absorbed the excess fluid, a sterile cork borer, 8 mm in diameter, was used to create 4 mm-deep wells in the sealed agar medium. Next, 150 μL of each compound at different concentrations (125, 250, 500, and 1000 μg mL−1) was added to the wells in the plates via a micropipette. Positive controls (ciprofloxacin at 5 μg μL−1) as well as sterile distilled water, which served as a negative control, were equally filled in the wells. The plates were incubated at 37 °C for 24 h. The diameters of the inhibition zones for each sample, including the wells, were measured in mm with calipers, and the results were recorded. All assays were performed in triplicate.
2.5 Color-removing ability of macrocycles
Batch adsorption experiments to remove methylene blue dye from solution were carried out with 10 mg of the prepared macrocyclic compound, which was added to a 20 mL aqueous solution of dye (5.0 mg L−1) by use of an appropriate amount of dye stock solutions. The mixtures were stirred using a water-bath shaker at 200 rpm, 30 °C (room temperature); the pH was normal (8.0–9.0). After the desired contact time, the adsorbent and adsorbate were centrifugally separated. The residual methylene blue dye concentrations in the supernatant clear solutions were spectrophotometrically determined at λmax = 664 nm.28
The removal percent was calculated using the below equation:
where
C0 and
Ct denote the concentration of dye at time 0 and
t, respectively; and
A0 and
At describe the initial absorbance and the absorbance of the dye sample at time
t, respectively.
3. Results and discussion
3.1 Chemistry
The present study is focused on the synthesis and characterization of new macrocycles containing two chalcone moieties and four ether groups, and their evaluation against two types of bacteria: E. coli (Gram-negative) and S. aureus (Gram-positive). This investigation involves the synthesis of a series of novel macrocycles through the combination of various functional groups in di-haloalkanes, aldehydes, and ketones. Common reaction types employed in this synthesis include Williamson ether synthesis, which is used for the benzylation of 2-hydroxybenzaldehydes and 2-hydroxyacetophenones with di-haloalkanes (1a–f), and Claisen–Schmidt condensation of bis-benzaldehydes and bis-acetophenones to yield a series of macrocycles (2a–e), as outlined in Scheme 1. The final step of the synthetic process results in the production of new biologically active macrocycle derivatives.
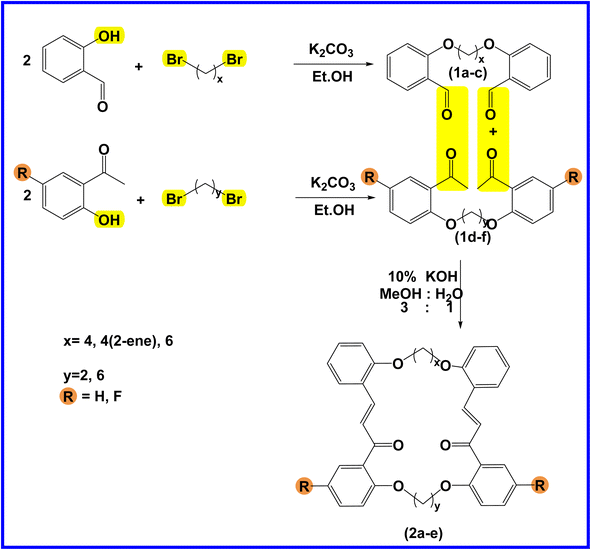 |
| Scheme 1 Stepwise synthesis of a chalcone-based crown ether. | |
The most significant evidence of bis-aldehyde and bis-ketone formation appears in the FTIR spectra of compounds 1a–f. This includes the disappearance of a broad band at 3450–3330 cm−1, which corresponds to the hydroxyl group of hydroxy benzaldehydes and acetophenones, a shift in the absorption band of the carbonyl group to a range of 1681–1662 cm−1, and the presence of intense peaks of CH2 stretching linkages at 2956–2833 cm−1,29 which are absent in mono-aldehydes and mono-ketones. The 1H-NMR spectroscopic analysis confirmed these findings by showing the loss of the aldehydes and the hydroxyl peak of ketones,30 the appearance of peaks of CH2 linkages at 1.5–4.1 ppm,31 and the presence of the C–H peak of aldehydes at approximately 10.5 ppm. Additionally, the 13C-NMR spectroscopic analysis revealed the carbonyl group peak at approximately 189 ppm, along with peaks of CH2 linkages at 25–68 ppm.32 The DEPT charts predicted downward peaks of CH2 linkages at 25–68 ppm and the disappearance of peaks for non-protonated carbons, including the carbonyl of acetophenone and some of the aromatic carbon atoms.
Further evidence of macrocycle formation appeared in the FTIR spectra of compounds 2a–e. This evidence included the disappearance of a band at 2760–2746 cm−1, which corresponded to the C–H stretching of bis-aldehydes, and a shift in the absorption band of carbonyl groups from a range of 1681–1662 cm−1 to the lower range of 1664–1651 cm−1.33,34 The 1H-NMR spectroscopic analysis affirmed this by showing the loss of the aldehydes' C–H peak at approximately 10.5 ppm and the disappearance of the CH3 peak of ketones at approximately 2.6 ppm.35 The 13C-NMR spectroscopic analysis further indicated a shift in the carbonyl group peak to 191–194 ppm.36,37 Predicted DEPT charts demonstrated downward peaks of CH2 linkages at 25–68 ppm and the disappearance of peaks for non-protonated carbons, including carbonyl and some of the aromatic carbon atoms.
3.2 Antioxidant activity
Antioxidants are molecules that generously donate electrons to counteract and neutralize harmful oxidants. These compounds work by stabilizing free radicals within oxidant molecules and replenishing the electrons that free radicals lack, thereby preventing a damaging chain reaction. In a recent research study, the antioxidant potential of five different macrocycles was assessed using the DPPH method.38 The outcomes are detailed in Table 1 and Fig. 1. DPPH, a stable violet-colored free radical, underwent transformation to 1,1-diphenyl-2-picrylhydrazine, which resulted in a change in color. The macrocycles were applied at varying concentrations (125, 250, 500, and 1000 ppm) and displayed diverse degrees of free radical scavenging activity. Previous investigations have demonstrated that macrocycles 2a–e altered the violet DPPH hue to a clear yellow shade due to their hydrogen-donating capabilities.
Table 1 The antioxidant potential of four concentrations of macrocycles 2a–e; the shown numbers are the % inhibition for each macrocycle
|
Macrocycle 2a |
Macrocycle 2b |
Macrocycle 2c |
Macrocycle 2d |
Macrocycle 2e |
Ascorbic acid |
125 ppm |
51 |
49 |
44 |
47 |
50 |
90 |
250 ppm |
52 |
51 |
53 |
48 |
57 |
90 |
500 ppm |
52 |
51 |
55 |
49 |
59 |
90 |
1000 ppm |
52 |
58 |
58 |
53 |
79 |
90 |
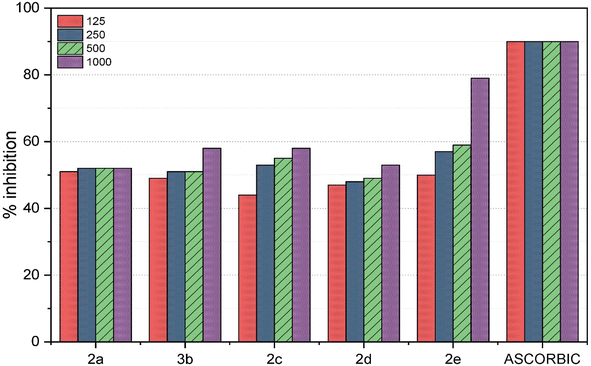 |
| Fig. 1 Inhibition of radicals by 2a–e at 30 minutes as compared to a standard of ascorbic acid. | |
The current study established that the radical-scavenging efficacy of compounds 2a–e is fundamentally rooted in their electron-donating attributes. Dimethyl sulfoxide (DMSO), a polar solvent, was employed39 to attract and inhibit the radicals. The results differed across the various compounds and concentrations tested. Ascorbic acid, renowned for its potent antioxidant properties, was used as a standard in this investigation. Notably, compound 2e (at 1000 ppm) exhibited an antioxidant activity pattern closely resembling that of ascorbic acid, as shown in Fig. 2, with a remarkable 79% inhibition of radicals, indicating significantly lower absorbance compared to the other compounds (2a–e). At different concentrations, the remaining compounds displayed moderate free radical scavenging, exceeding 50% inhibition. However, compounds 2b (at 125 ppm), 2c (at 125 ppm), and 2d (at 125 ppm, 250 ppm, and 500 ppm) demonstrated lower inhibition of free radicals, falling below the 50% threshold.
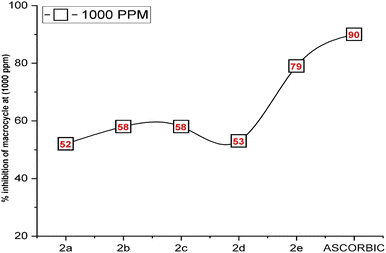 |
| Fig. 2 Inhibition of radicals by 2a–e at the concentration of 1000 ppm as compared to a standard of ascorbic acid. | |
3.3 Antibacterial activity
The antibacterial properties of the macrocyclic compounds were investigated through in vitro testing. Two distinct microorganisms, namely a Gram-positive bacteria (S. aureus) and a Gram-negative bacteria (E. coli), were employed for the study. The inhibition zones produced by each compound were meticulously measured in millimeters after a 24 hours incubation period at 37 °C.40 Impressively, all compounds demonstrated a consistent and substantial order of antibacterial efficacy, with inhibition zones falling within the range of 11 to 21 mm.
To evaluate the antibacterial activity of these macrocyclic compounds, we employed the agar well diffusion method. Notably, the evaluated compounds displayed varying inhibition zones on the culture media against both types of bacteria. This discrepancy in antibacterial activity was attributed to the diverse substituents present on the aromatic rings and linkages of these compounds, as they all share a common core chemical structure.
The comprehensive evaluation results, as presented in Table 2 and Fig. 4, revealed compelling insights. Specifically, compounds 2b (500 ppm), 2b (1000 ppm), and 2d (125 ppm) exhibited the most substantial inhibition zones against S. aureus, as shown in Fig. 5 and 6, while compounds 2a (250 ppm), 2a (1000 ppm), and 2b (500 ppm) displayed exceptional antibacterial activity against E. coli across a wide range of concentrations. Conversely, compounds 2b (250 ppm) and 2e (250 ppm) showed comparatively smaller inhibition zones against S. aureus. Compounds 2a (125 ppm), 2b (125 ppm), 2c (125 ppm), 2c (1000 ppm), 2d (125 ppm), 2d (500 ppm), 2e (250 ppm), and 2e (1000 ppm) exhibited the least significant antibacterial activity against E. coli, when compared to other macrocyclic compounds, across the entire concentration range. These results indicated that the Gram-negative bacteria E. coli displayed greater resistance in comparison to the Gram-positive bacteria S. aureus, as shown in Fig. 3.
Table 2 The antibacterial activity of four concentrations of macrocycles 2a–e; the shown numbers are the inhibition zones in mm
Concentration |
E. coli (Gram-negative) |
S. aureus (Gram-positive) |
125 ppm |
250 ppm |
500 ppm |
1000 ppm |
125 ppm |
250 ppm |
500 ppm |
1000 ppm |
Macrocycle 2a |
11 |
13 |
14 |
13 |
18 |
18 |
19 |
19 |
Macrocycle 2b |
11 |
12 |
13 |
12 |
18 |
17 |
21 |
20 |
Macrocycle 2c |
11 |
12 |
12 |
11 |
17 |
18 |
19 |
18 |
Macrocycle 2d |
11 |
12 |
11 |
12 |
20 |
18 |
19 |
18 |
Macrocycle 2e |
12 |
11 |
12 |
11 |
19 |
17 |
18 |
17 |
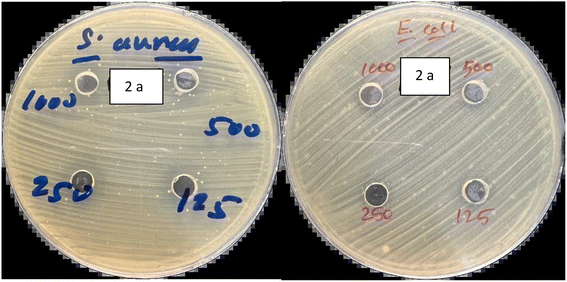 |
| Fig. 3 Antibacterial activity of compound 2a against S. aureus (left) and E. coli (right). | |
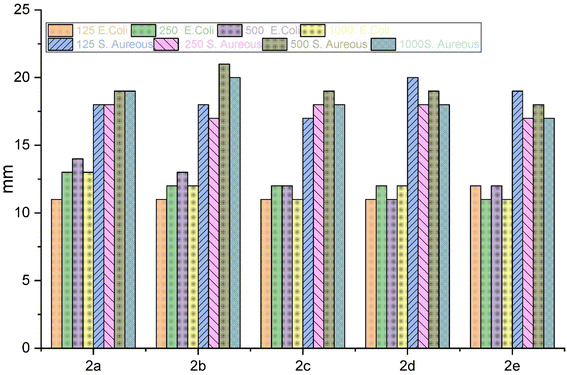 |
| Fig. 4 Antibacterial activity of 2a–e against both microorganisms. | |
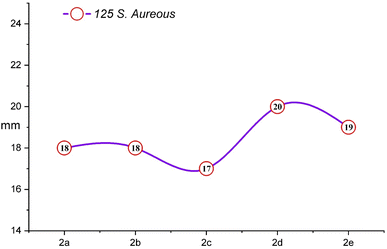 |
| Fig. 5 Inhibition zone (in mm) by 2a–e against S. aureus at 125 ppm. | |
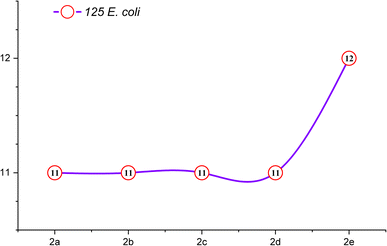 |
| Fig. 6 Inhibition zone (in mm) by 2a–e against E. coli at 125 ppm. | |
3.4 Dye-removing ability of macrocycles
One of the most pressing challenges of our time is water contamination. The physical removal of impurities from polluted water is not only a safer option, but it is also more cost-effective compared to alternative methods such as chemical precipitation, membrane separation, solvent extraction, and physical adsorption.28 This issue is primarily driven by industrial activities, leading to severe health and economic repercussions.
Recognizing the gravity of the problem, extensive research has been conducted to address it. In this regard, we evaluated this novel class of synthesized chalcone moiety macrocycles for their efficacy in removing colorants from wastewater, specifically at room temperature, as shown in Table 3. To assess their adsorption capabilities, 5.0 mg L−1 methylene blue was employed as the adsorbate, with compounds 2a–e serving as the adsorbents.
Table 3 Absorbance and percent removal of methylene blue by macrocycles 2a–e
Compound |
Absorbance |
% removed color |
Methylene blue |
0.983 |
0.00 |
2a |
0.207 |
78.94 |
2b |
0.103 |
89.52 |
2c |
0.075 |
92.37 |
2d |
0.034 |
96.54 |
2e |
0.123 |
87.48 |
The results demonstrate the amazing efficiency of these chalcone moiety macrocycles in eliminating contaminants from wastewater. Notably, compounds 2c and 2d emerged as the most effective, achieving removal rates of 92.37% and 96.54% for methylene blue dye, respectively, as shown in Fig. 7 and 8, surpassing the performance of other adsorbents. In contrast, compound 2a exhibited a lower removal efficiency of 78.92%, as seen in Fig. 9. These findings signify a significant step forward in combatting water pollution and highlight the potential of chalcone moiety macrocycles to provide a sustainable solution to this critical issue.
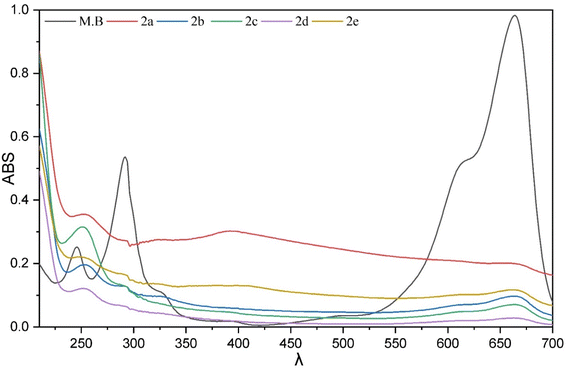 |
| Fig. 7 Absorbance of 2a–e with methylene blue. | |
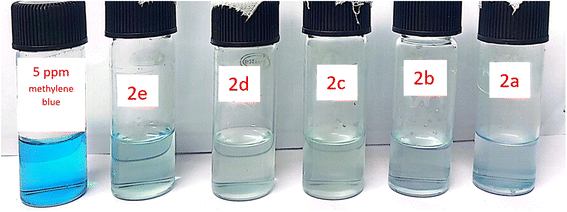 |
| Fig. 8 Ability of macrocycles 2a–e to remove methylene blue dye. | |
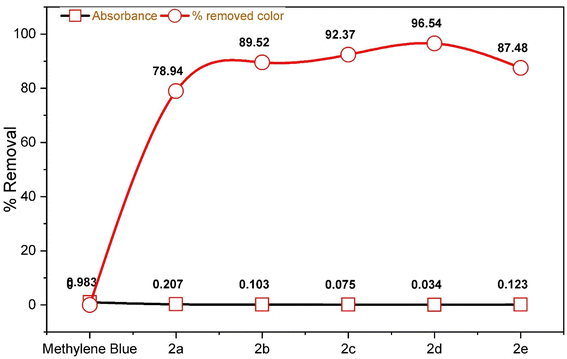 |
| Fig. 9 Absorbance and percent removal of methylene blue by 2a–e. | |
The usefulness of the specific adsorbent depends not only on its adsorptive capacity, but also on the ease of its regeneration and reuse. Four different eluents (ethanol, methanol, nitric acid, and deionized water) were applied as the desorbing agent. The most optimal one was ethanol, and thus, the adsorbent was dried in an oven during regeneration, with each sorption–desorption cycle employing ethanol and subsequent heat treatment.
The removal efficiency of the adsorbents remained constant, and no significant difference in removal efficiency was observed during five adsorption–desorption cycles. These data are encouraging and indicate that there is satisfactory reusability for the adsorbents used in this experimental study.
4. Conclusion
Six bis intermediate linkages and five macrocyclic bis-chalcone moieties were successfully synthesized, yielding symmetric compounds, as confirmed by 13C-NMR and 13C-DEPT charts. However, certain compounds (1f, 2a, 2b, 3d, and 2e) exhibited a distorted symmetric structure, with carbon atoms in slightly different positions, which was attributed to the challenges posed by the increased size of these molecules.
The synthesized compounds demonstrated promising applications, particularly in biological activities against the Gram-positive bacteria S. aureus and the Gram-negative bacteria E. coli. Compounds 2a–e displayed effective results, with a noteworthy observation of greater susceptibility of Gram-negative bacteria (E. coli) as compared to Gram-positive bacteria (S. aureus). Comprehensive evaluation highlighted compound 2b (at 500 ppm) as a potent inhibitor against E. coli, while compound 2a (at 500 ppm) exhibited exceptional antibacterial activity against S. aureus, with the introduction of a halogen atom, such as fluorine, contributing to the enhanced performance against both bacterial types.
In addition to antimicrobial properties, the compounds demonstrated antioxidant activity, with strong antioxidant activity of 79% inhibition of radicals exhibited by compound 2e (at 1000 ppm) that was similar to that of ascorbic acid at 90% inhibition. This result, with significantly lower absorbance compared to the other compounds (2a–e), underscores the potential of these compounds in antioxidant applications.
Furthermore, the macrocycles were evaluated for their ability to remove dye from contaminated water, with all five exhibiting excellent results. Compound 2d, in particular, emerged as the most effective, achieving a remarkable 96.54% removal rate for methylene blue dye, surpassing the performance of other tested adsorbents. These findings collectively highlight the diverse and promising applications of the synthesized compounds in various fields, from antibacterial and antioxidant activities to water purification processes.
Data availability
The data that support this study are available in the article and accompanying online ESI.†
Conflicts of interest
The authors have no conflicts of interest.
Acknowledgements
This work was supported by Salahaddin University – Erbil, Erbil, Kurdistan, Iraq as the PhD program. The authors would like to thank Dr. Mohammed K. Samad, Dr. Aveen F. Jalal, and Dr. Rebwar Muhammad Hamasalih for the excellent technical assistance performed in conducting the in vitro biochemical assays.
References
- D. W. Fitzpatrick and H. J. Ulrich, Macrocyclic Chemistry: New Research Developments, Nova Science Pub Inc, 2010 Search PubMed.
- V. Gupta, J. Singh, M. Kinger, A. K. Arora and V. S. Jaswal, Synthesis, 2015, 1, 2 Search PubMed.
- A. S. Surur and D. Sun, Front. Chem., 2021, 9, 659845 CrossRef CAS PubMed.
- A. Martinez De Pinillos Bayona, P. Mroz, C. Thunshelle and M. R. Hamblin, Chem. Biol. Drug Des., 2017, 89, 192–206 CrossRef CAS PubMed.
- J.-S. Lee, I.-h. Song, P. B. Shinde and S. B. Nimse, Antioxidants, 2020, 9, 859 CrossRef CAS PubMed.
- A. Chávez-Riveros, E. Hernández-Vázquez, A. Nieto-Camacho, T. Ramírez-Apan and L. D. Miranda, Org. Biomol. Chem., 2019, 17, 1423–1435 RSC.
- L. B. Zasada, L. Guio, A. A. Kamin, D. Dhakal, M. Monahan, G. T. Seidler, C. K. Luscombe and D. J. Xiao, J. Am. Chem. Soc., 2022, 144, 4515–4521 CrossRef CAS PubMed.
- C. Ropeter, H. Wenzl, H.-P. Beck, E. A. Wehrmann, J. Twidell and D. U. Sauer, The Impact of Microcycles on Batteries in Different Applications, 2001 Search PubMed.
- T. Huang, M. Alyami, N. M. Kashab and S. P. Nunes, J. Mater. Chem. A, 2021, 9, 18102–18128 RSC.
- M. L. Rao, H. Houjou and K. Hiratani, Tetrahedron Lett., 2001, 42, 8351–8355 CrossRef CAS.
- M. Shakir, S. Khatoon, S. Parveen and Y. Azim, Transition Met. Chem., 2007, 32, 42–46 CrossRef CAS.
- H. I. Ugras, I. Basaran, T. Kilic and U. Cakir, J. Heterocycl. Chem., 2006, 43, 1679–1684 CrossRef CAS.
- F. E. Hawaiz, Zanco J. Pure Appl. Sci., 2016, 28, 140–147 Search PubMed.
- W. C. Campbell, Curr. Pharm. Biotechnol., 2012, 13, 853–865 CAS.
- R. N. Dsouza, U. Pischel and W. M. Nau, Chem. Rev., 2011, 111, 7941–7980 CrossRef CAS PubMed.
- N. A. Thiele, S. N. MacMillan and J. J. Wilson, J. Am. Chem. Soc., 2018, 140, 17071–17078 CrossRef CAS PubMed.
- Inamuddin, Int. J. Biol. Macromol., 2019, 121, 1046–1053 CrossRef CAS PubMed.
- F. Mashkoor and A. Nasar, J. Magn. Magn. Mater., 2020, 500, 166408 CrossRef CAS.
- J. S. Sawyer, Tetrahedron, 2000, 56, 5045–5065 CrossRef.
- A. Guida, M. H. Lhouty, D. Tichit, F. Figueras and P. Geneste, Appl. Catal., A, 1997, 164, 251–264 CrossRef CAS.
- M. M. Ayoob, F. E. Hawaiz, A. Hussein, M. K. Samad, F. Hussain and S. K. Mohamed, Egypt. J. Chem., 2020, 63, 2617–2629 Search PubMed.
- F. E. Hawaiz, F. H. S. Hussain and H. J. Azeez, J. Mater. Sci. Eng. A, 2014, 4, 361–366 CAS.
- S. K. Mohamed, M. Akkurt, F. E. Hawaiz, M. M. Ayoob and E. Hosten, Acta Crystallogr., Sect. E: Crystallogr. Commun., 2017, 73, 13–16 CrossRef CAS PubMed.
- D. X. Duc and N. T. Chung, Vietnam J. Sci. Technol., 2020, 58, 1–9 Search PubMed.
- M. Rashid, M. Akter, J. Uddin, S. Islam, M. Rahman, K. Jahan, M. Sarker, M. Rahman and G. Sadik, Clin. Phytosci., 2023, 9, 1–9 CrossRef CAS.
- H. F. S. Akrayi, R. M. H. Salih and P. A. Hamad, ARO, 2015, 3, 35–41 CrossRef.
- M. M. Ayoob and F. E. Hawaiz, Inorg. Chem. Commun., 2023, 158, 111499 CrossRef CAS.
- A. F. Jalal and N. A. Fakhre, PLoS One, 2022, 17, e0275330 CrossRef CAS PubMed.
- C. Lluna-Galán, L. Izquierdo-Aranda, R. Adam and J. R. Cabrero-Antonino, ChemSusChem, 2021, 14, 3744–3784 CrossRef PubMed.
- A. Fayad, W. El Malti, B. Ameduri and A. Alaaeddine, C. R. Chim., 2022, 25, 9–18 CrossRef CAS.
- S. A. Guillén-Castellanos, J. S. Parent and R. A. Whitney, J. Polym. Sci., Part A: Polym. Chem., 2006, 44, 983–992 CrossRef.
- Q. Xu, H. Xie, P. Chen, L. Yu, J. Chen and X. Hu, Green Chem., 2015, 17, 2774–2779 RSC.
- S. Mandge, H. P. Singh, S. D. Gupta and N. Moorthy, Trends Appl. Sci. Res., 2007, 2, 52–56 CrossRef CAS.
- C. Tamuly, I. Saikia, M. Hazarika, M. Bordoloi, N. Hussain, M. R. Das and K. Deka, RSC Adv., 2015, 5, 8604–8608 RSC.
- O. Petrov, Y. Ivanova and M. Gerova, Catal. Commun., 2008, 9, 315–316 CrossRef CAS.
- M. Tiecco, R. Germani and F. Cardellini, RSC Adv., 2016, 6, 43740–43747 RSC.
- A. Hemamalini and T. M. Das, RSC Adv., 2014, 4, 41010–41016 RSC.
- V. Bondet, W. Brand-Williams and C. Berset, LWT – Food Sci. Technol., 1997, 30, 609–615 CrossRef CAS.
- C. Sanmartín-Suárez, R. Soto-Otero, I. Sánchez-Sellero and E. Méndez-Álvarez, J. Pharmacol. Toxicol. Methods, 2011, 63, 209–215 CrossRef PubMed.
- R. C. Goy, S. T. Morais and O. B. Assis, Rev. Bras. Farmacogn., 2016, 26, 122–127 CrossRef CAS.
|
This journal is © The Royal Society of Chemistry 2024 |
Click here to see how this site uses Cookies. View our privacy policy here.