DOI:
10.1039/D3RA08272E
(Paper)
RSC Adv., 2024,
14, 700-706
Photocatalytic selective synthesis of (E)-β-aminovinyl sulfones and (E)-β-amidovinyl sulfones using Ru(bpy)3Cl2 as the catalyst†
Received
4th December 2023
, Accepted 17th December 2023
First published on 2nd January 2024
Abstract
Selectively producing a variety of valuable compounds using controlled chemical reactions starting from a common material is an appealing yet complex concept. Herein, a photocatalytic approach for the selective synthesis of (E)-β-aminovinyl sulfones and (E)-β-amidovinyl sulfones from allenamides and sodium sulfinates was established. This reaction exhibits the traits of an eco-friendly solvent and adjustable amide cleavage, and can accommodate a diverse range of substrates with exceptional functional group tolerance. Based on control experiments and deuterium labeling experiments, a plausible radical reaction pathway is proposed.
Introduction
In the field of pharmaceutical and organic chemistry, significant advancements in recent years have paved the way for the recognition of the diverse biological activities of β-amino vinyl sulfone skeletons1 and their potential in organic transformation.2 Consequently, the development of methods for the selective construction of these skeletons is gaining significant attention. Sulfonylation via the addition of sulfonyl radicals to enamides and their derivatives is a prevalent method for chemical transformation (Scheme 1a).3 This process typically involves the use of various aryl sulfonyl halides and sodium sulfinates under specific conditions. Another effective approach to synthesizing aminovinyl sulfones is via C(sp3)–H functionalization of amines (Scheme 1b).4,5 Researchers such as Yuan,4a Xia,4b and Talbot4c have reported direct C(sp3)–H sulfonylation of tertiary amines to β-amidovinyl sulfones via catalysis with excessive oxidants. Visible-light photoredox catalysis has also been used for this purpose by Zheng,5a Rueping,5b and Sun.5c Despite recent advances, there remains a need for developing innovative building blocks to simplify the preparation of β-aminovinyl sulfones. This includes expanding the range of substrates while avoiding expensive catalysts and hazardous additives, which is vital for the safe and efficient practice of organic synthetic chemistry.
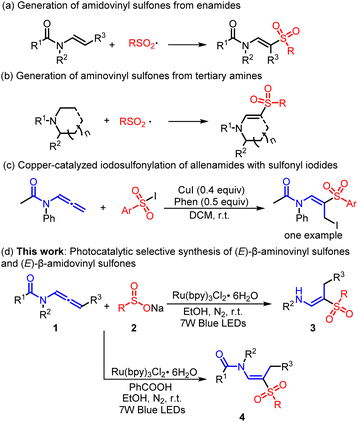 |
| Scheme 1 General strategy for the synthesis of aminovinyl sulfone. | |
As a highly versatile and potent building block, allenamides have garnered extensive usage in the synthesis of aminovinyl derivatives via catalysis by either Brønsted acids or transition metals.6 To the best of our knowledge, there have been limited investigations into the radical reaction of allenamides, particularly the precise chemo-, regio-, and stereoselective radical sulfonylation of allenamides. In 2018, Zhang et al. reported a novel iodosulfonylation of allenamides using sulfonyl iodides, CuI, and 1,10-phenanthroline. The approach resulted in highly regioselective iodination of the allenamide backbone, affording I-α-iodomethyl amidovinyl sulfones.7 At the same time, studies have shown that visible light-induced sulfonylation is an effective method for the synthesis of sulfone-containing compounds.8 However, until now, no reports have described the photoredox-catalyzed hydrosulfonylation of allenamides to efficiently produce β-aminovinyl sulfones or β-aminovinyl sulfones with high regio- and stereoselectivity. Selectively producing a variety of valuable compounds using controlled chemical reactions starting from a common material is an appealing yet complex concept.9 As part of our ongoing research aimed at developing efficient strategies for the synthesis of sulfone-containing compounds,10 we propose a straightforward and generalized photocatalytic method for the production of (E)- β-aminovinyl sulfones and (E)- β-amidovinyl sulfones from allenamides and sodium sulfinates (Scheme 1d).
Results and discussion
Our investigation began by evaluating the model reaction between N-phenyl-N-(propa-1,2-dien-1-yl) acetamide (1a)11 and sodium p-toluenesulfinate (2a)12 using 3 mol% eosin Y as catalyst and dried ethanol (EtOH) as solvent. After 8 hours of irradiation with 7 W blue LEDs at room temperature, we isolated (E)-N-(2-tosylprop-1-en-1-yl) aniline 3a with a yield of 37% (Table 1, entry 1). Encouraged by this result, we screened other photocatalysts such as Na2–eosin Y, 4-CzIPN, (Ir[p-Fppy]2(dtbpy))PF6 and Ru(bpy)3Cl2·6H2O to improve the conversion rate (entries 2–5). Interestingly, all of these catalysts showed better catalytic efficiency than before, with Ru(bpy)3Cl2·6H2O showing the highest yield of 92%. However, when we changed the solvent from EtOH to other alcohol solvents like methanol (MeOH), isopropyl alcohol (i-PrOH), and tert-butanol (t-BuOH), we observed lower conversions (entries 6–8). Likewise, other solvents like trifluoroethanol (TFE), and 1,1,1,3,3,3-hexafluoro-2-propanol (HFIP) completely inhibited the reaction (entries 9 and 10). Consequently, we selected additives such as bases (K2HPO4) or acids (CH3COOH). The 3a yield did not increase when excess base amounts were added (entry 11). However, when we added acetic acid as an additive, the product 4a was selectively generated whereas the formation of 3a was completely inhibited (entry 12). We further screened other Brønsted acids, including formic acid (HCOOH), trifluoroacetic acid (TFA), p-toluenesulfonic acid (TsOH), and benzoic acid (PhCOOH), and identified benzoic acid as exhibiting good selectivity, with yields of up to 75% for 4a (entries 13–16). Significantly, no desired product was detected in the absence of either Ru(bpy)3Cl2·6H2O or the irradiation of visible light, which both play an essential role in the generation of the sulfonyl radical (entries 18 and 19).
Table 1 Optimization of reaction conditionsa

|
Entry |
Photocatalyst |
Solvent |
Additive |
Yieldb 3a (%) |
Yieldb 4a (%) |
Reaction conditions are as follows: 1a (0.2 mmol), 2a (0.4 mmol), cat. (3 mol%), additive (0.4 mmol), solvent (2 mL), under 7 W blue LEDs, at room temperature, N2, 8 h. Isolated yield based on 1a. Cat. (1 mol%). 2a (0.5 mmol), additive (0.5 mmol). 2a (0.3 mmol), additive (0.3 mmol). N.D., not detected. TFE, 2,2,2-trifluoroethanol. TFA, trifluoroacetic acid. |
1 |
Eosin Y |
EtOH |
— |
37 |
N.D. |
2 |
Na2–eosin Y |
EtOH |
— |
46 |
N.D. |
3 |
4-CzIPN |
EtOH |
— |
64 |
N.D. |
4 |
(Ir[p-Fppy]2(dtbpy))PF6 |
EtOH |
— |
85 |
N.D. |
5 |
Ru(bpy)3Cl2·6H2O |
EtOH |
— |
92 |
N.D. |
6 |
Ru(bpy)3Cl2·6H2O |
MeOH |
— |
88 |
N.D. |
7 |
Ru(bpy)3Cl2·6H2O |
i-PrOH |
— |
74 |
N.D. |
8 |
Ru(bpy)3Cl2·6H2O |
t-BuOH |
— |
70 |
N.D. |
9 |
Ru(bpy)3Cl2·6H2O |
TFE |
— |
N.D. |
N.D. |
10 |
Ru(bpy)3Cl2·6H2O |
HFIP |
— |
N.D. |
N.D. |
11 |
Ru(bpy)3Cl2·6H2O |
EtOH |
K2HPO4 |
71 |
N.D. |
12 |
Ru(bpy)3Cl2·6H2O |
EtOH |
CH3COOH |
N.D. |
54 |
13 |
Ru(bpy)3Cl2·6H2O |
EtOH |
HCOOH |
N.D. |
56 |
14 |
Ru(bpy)3Cl2·6H2O |
EtOH |
TFA |
N.D. |
66 |
15c |
Ru(bpy)3Cl2·6H2O |
EtOH |
TsOH |
N.D. |
57 |
16d |
Ru(bpy)3Cl2·6H2O |
EtOH |
PhCOOH |
N.D. |
75 |
17e |
Ru(bpy)3Cl2·6H2O |
EtOH |
PhCOOH |
N.D. |
64 |
18 |
— |
EtOH |
PhCOOH |
N.D. |
N.D. |
19 |
— |
EtOH |
— |
N.D. |
N.D. |
After optimizing the photoredox conditions, the scope and limitations of the developed method for hydrosulfonylation and amide cleavage of allenamides with sodium sulfinates were examined (Scheme 2). Initially, we examined the impacts of various amides on the reaction, including acetamide, benzamide, and p-toluenesulfonamide. Out of these, the acetamide group exhibited superior selectivity, yielding up to 92% for 3a. Conversely, the p-toluenesulfonamide group failed to produce the intended final product. Next, sodium p-toluenesulfinate (2a) was reacted with allenamides (1) featuring variegated electronic properties and aryl substitution positions. The photocatalytic transformations were successfully accomplished with all of them. Different substituents on the p-position of the aromatic ring, such as methoxy (1b), methyl (1c), fluoro (1d), chloro (1e), and bromo (1f) groups, were compatible with this photocatalytic strategy, furnishing corresponding products 3b–3f in 62–74% yields. Overall, neither the electron-withdrawing groups nor electron-donating groups had a particularly significant impact on this photochemical process. Additionally, the steric effect of the substituent at the ortho- or meta-position of the arene ring was briefly investigated. Representative functional groups such as Me, MeO, and Cl at the ortho-position were accommodated, and the target (E)-β-aminovinyl sulfones were generated with moderate yields (3i–3k). Unfortunately, when we used internal allenamide 1l as the substrate, the reaction did not proceed, demonstrating that a terminal allenyl is crucial for this transformation. Next, a brief survey of the possible structural variations of sodium sulfinates 2 was conducted by combining allenamide 1a as model components. As shown in Scheme 2b, a series of sodium arylsulfinates were engaged in this photocatalytic process to form the desired (E)-β-aminovinyl sulfones (3m–3v). All tested sodium arylsulfinates 2m–2v, whether electronically rich (e.g. methoxy and tert-butyl) or poor (e.g. fluoro, chloro, bromo, and trifluoromethyl) groups were attached to the aromatic ring, could be converted to the corresponding products 3m–3v in 53–85% yields. It is worth noting that in sodium sulfinate, the ortho or meta-substituents of arene ring seem to behave less positively when undergoing photochemical processes, resulting in a lower yield of (E)-β-amino vinyl sulfone (3s–3u, 32–36%). We speculate that this may be due to the large steric resistance of the ortho-substituent, which affects reaction progress. Additionally, heterocyclic sodium sulfinate 2v was also compatible with this transformation in an acceptable yield. To our delight, aliphatic sodium sulfinate starting materials such as sodium methylsulfinate (2w) and sodium ethylsulfinate (2x) also successfully provided the desired products 3w and 3x in moderate yields.
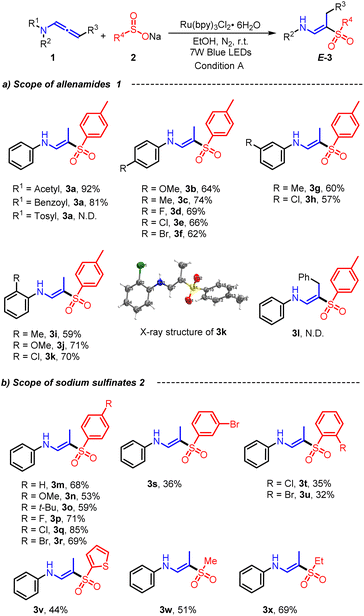 |
| Scheme 2 Substrate scope of (E)-β-aminovinyl sulfones 3. Reaction condition A: 1 (0.2 mmol), 2 (0.4 mmol), Ru(bpy)3Cl2·6H2O (3 mol%), EtOH (2 mL), under 7 W blue LEDs, at room temperature, N2, 8 h. | |
To achieve regio- and stereoselective hydrosulfonylation of allenamides for synthesizing (E)-β-amidovinyl sulfones under benzoic acid conditions, we conducted additional investigation into the photocatalytic process of varied allenamides with sodium sulfinates (Scheme 3). Substrates 1 exhibited good tolerance for functional substituents, including methyl, methoxyl, fluoro, chloro, and bromo, located on the aromatic ring of allenamides, resulting in the delivery of the corresponding E-amidovinyl sulfones 4a–4k with yields ranging from 55% to 86%. Subsequently, various alkyl substituents were introduced onto the nitrogen atom of allenamides to enhance their synthetic versatility, including benzyl (Bn), n-pentyl and methyl (Me) groups. The resulting products (4n–4r) were obtained in yields ranging from 60% to 70%. It is noteworthy that the internal allenamides (1s and 1t) underwent successful conversion to their respective products in acidic conditions. Furthermore, when allenamide is substituted with p-toluenesulfonamide, it can also readily undergo the reaction, although precise control of its stereoselectivity remains elusive (4u). In addition, various sodium arylsulfinate salts and sodium aliphaticsulfinate salts with 1a were converted to the corresponding (E)-β-amidovinyl sulfones in moderate to good yields (4v–4ah).
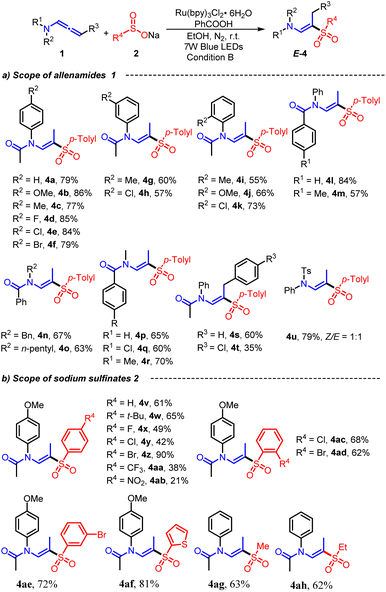 |
| Scheme 3 Substrate scope of (E)-β-amidovinyl sulfones 4. Reaction condition B: 1 (0.2 mmol), 2 (0.4 mmol), PhCOOH (0.4 mmol), Ru(bpy)3Cl2·6H2O (3 mol%), EtOH (2 mL), under 7 W blue LEDs, at room temperature, N2, 8 h. | |
To clarify the working mechanisms, several verification experiments were implemented. Initially, an “on/off” visible light exposure experiment was carried out to highlight the significance of continuous visible light exposure for the effective and managed synthesis of (E)-β-aminovinyl sulfones or (E)-β-amidovinyl sulfones (Scheme S1†). Subsequently, radical inhibition and intermediate trapping experiments were performed in the presence of 2,2,6,6-tetramethylpiperidinyloxy (TEMPO) or 1,1-diphenylethylene (Scheme 4A). Upon adding 3.0 equivalents of TEMPO, it was observed that the reaction process was completely inhibited, and TEMPO adducts 5 were successfully identified through high-resolution mass spectrometry (HRMS), suggesting a possible radical pathway. Additionally, the vinyl-trapped product 6 was also detected and characterized through HRMS. To further elucidate the hydrogen atom source in this process, deuterium labeling experiments were conducted under standard conditions (Scheme 4B). The reaction of allenamide 1a and sodium p-toluenesulfonate 2a in the presence of CD3OD led to the exclusive incorporation of deuterium in the methyl group of the resulting D-3a, while no deuterium was observed in the amino group (Scheme 4c). When D2O was used in stoichiometric amounts, no deuterium was detected in the methyl group (Scheme 4d). However, when the same reaction was conducted in the presence of D2O and CD3OD, the resulting D-3a′ showed 27% deuterium in the N-D group and 100% deuterium in the methyl group, indicating that the hydrogen on the methyl group originated from an alcohol solvent, while incidental traces of water acted as the resource of hydrogen in the medium to construct the N–H bond (Scheme 4e). The deuterium substitution experiment was likewise implemented on product D-4a, confirming that the solvent is the main source of the methyl hydrogen (Scheme 4f). Furthermore, the attempted synthesis of the (E)-β-aminovinyl sulfone under standard conditions using sodium p-toluenesulfonate resulted in the production of only trace amounts of the desired compound, suggesting that amide cleavage may not be initiated by an excess of sodium p-toluenesulfate that may exist in the reaction system (Scheme 4g). To demonstrate the wider applicability of this method, an amplification reaction was conducted under the standard conditions, wherein hydrolysis of 4a in the presence of 2 M aqueous sodium hydroxide solution in THF yielded 3a in 87% yield. It was found that 3a can be separated with a 61% total yield on a 5.0 mmol scale using a two-step reaction in condition B. However, using condition A, 3a can be produced at an 87% yield in a one-step reaction (Scheme 5). These results indicate that this method has significant potential application value in the direct preparation of (E)-β-aminovinyl sulfone.
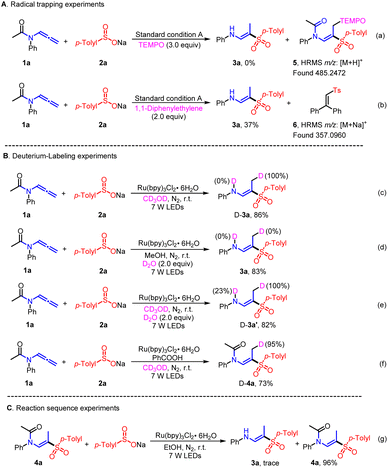 |
| Scheme 4 Control experiments. | |
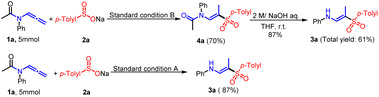 |
| Scheme 5 Synthesis of 3a and 4a using gram scales. | |
Based on the results presented above and in relevant literature,9,10,13 a plausible reaction mechanism for the photocatalytic regio- and stereoselective synthesis of (E)-β-aminovinyl sulfones and (E)-β-amidovinyl sulfones is proposed in Scheme 6. Initially, photoexcitation of Ru(bpy)3Cl2 with visible light generates [Ru2+]*, which is subsequently reductively quenched by free sulfinate to produce an Ru+ ion and a sulfonyl radical. The sulfonyl radical then reacts with the allene moiety of 1a to form a widely delocalized radical intermediate A, which is further reduced to an extensively delocalized carbanion B in the propagating step. There are two possible pathways for the transformation of intermediate B, which depend on the specific reaction conditions. In path a, intermediate B abstracts a proton from EtOH, generating (E)-4a, which undergoes intermolecular nucleophilic addition of the carbonyl group with ethoxy anion to yield intermediate D. Ultimately, proton transfer and selective C–N bond cleavage result in the formation of (E)-β-aminovinyl sulfone 3a, along with a potential byproduct, EtOAc. In path b, intermediate B grabs hydrogen protons from the alcohol to produce (E)-β-amidovinyl sulfone 4a. However, acid–base neutralization between the additive acid and the ethoxy anion inhibits subsequent reactions.
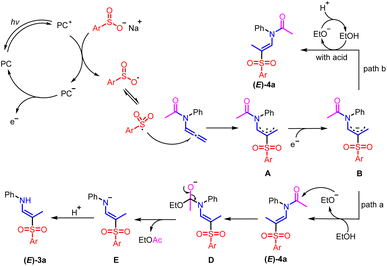 |
| Scheme 6 Proposed pathway toward product 3a and 4a. | |
Conclusions
In summary, we have developed a photochemical hydrosulfonylation method for the selective synthesis of (E)-β-aminovinyl sulfones and (E)-β-amidovinyl sulfones from readily available allenamides and sodium sulfinates. This reaction uses readily available and eco-friendly ethanol as a solvent, achieving significant chemical, regional, and stereoselectivity for the hydrosulfonation of allenamide. Through the regulation of acid additions, selective cleavage of amide bonds can be achieved without the requirement of supplemental transition metal catalysts or stoichiometric quantities of bases. The photocatalytic methodology exhibited a wide range of substrates and enabled the synthesis of diverse (E)-β-aminovinyl sulfones. Its mild conditions and convenient operation underscore the method's advantages for constructing polyfunctionalized aminovinyl sulfone scaffolds, which offer promising applications.
Conflicts of interest
There are no conflicts to declare.
Acknowledgements
We are grateful for financial support from the National Natural Science Foundation of China (NSFC, 21801130) and the Nanjing Polytechnic Institute Key Funded Scheme (NJPI-2021-06).
Notes and references
-
(a) H. Zhang, J. Collins, R. Nyamwihura, O. Crown, O. Ajayi and I. V. Ogungbe, Vinyl sulfone-based inhibitors of trypanosomal cysteine protease rhodesain with improved antitrypanosomal activities, Bioorg. Med. Chem. Lett., 2020, 30, 127217 CrossRef CAS PubMed;
(b) R. Ahmadi and S. Emami, Recent applications of vinyl sulfone motif in drug design and discovery, Eur. J. Med. Chem., 2022, 234, 114255 CrossRef CAS PubMed;
(c) S. Y. Woo, J. H. Kim, M. K. Moon, S. H. Han, S. K. Yeon, J. W. Choi, B. K. Jang, H. J. Song, Y. G. Kang, J. W. Kim, J. Lee, D. J. Kim, O. Hwang and K. D. Park, Discovery of vinyl sulfones as a novel class of neuroprotective agents toward Parkinson's disease therapy, J. Med. Chem., 2014, 57, 1473–1487 CrossRef CAS PubMed;
(d) S. Arastu-Kapur, E. L. Ponder, U. P. Fonović, S. Yeoh, F. Yuan, M. Fonović, M. Grainger, C. I. Phillips, J. C. Powers and M. Bogyo, Identification of proteases that regulate erythrocyte rupture by the malaria parasite Plasmodium falciparum, Nat. Chem. Biol., 2008, 4, 203–213 CrossRef CAS PubMed.
-
(a) J. Jiang, Y. Wang and X. Zhang, Rhodium-Catalyzed Asymmetric Hydrogenation of β-Acetylamino Acrylosulfones: A Practical Approach to Chiral β-Amido Sulfones, ACS Catal., 2014, 4, 1570–1573 CrossRef CAS;
(b) B. Niu, B. G. Blackburn, K. Sachidanandan, M. V. Cooke and S. Laulhé, Metal-free visible-light-promoted C(sp3)–H functionalization of aliphatic cyclic ethers using trace O2, Green Chem., 2021, 23, 9454–9459 RSC;
(c) J. Long, W. Gao, Y. Guan, H. Lv and X. Zhang, Nickel-Catalyzed Highly Enantioselective Hydrogenation of beta-Acetylamino Vinylsulfones: Access to Chiral beta-Amido Sulfones, Org. Lett., 2018, 20, 5914–5917 CrossRef CAS PubMed.
-
(a) H. Jiang, X. Chen, Y. Zhang and S. Yu, C-H Functionalization of Enamides: Synthesis of β-Amidovinyl Sulfones via Visible-Light Photoredox Catalysis, Adv. Synth. Catal., 2013, 355, 809–813 CrossRef CAS;
(b) T. H. Zhu, X. C. Zhang, X. L. Cui, Z. Y. Zhang, H. Jiang, S. S. Sun, L. L. Zhao, K. Zhao and T. P. Loh, Direct C(sp2)-H Arylsulfonylation of Enamides via Iridium(III)-Catalyzed Insertion of Sulfur Dioxide with Aryldiazonium Tetrafluoroborates, Adv. Synth. Catal., 2019, 361, 3593–3598 CrossRef CAS;
(c) L. Chen, M. Zhou, L. Shen, X. He, X. Li, X. Zhang and Z. Lian, Metal- and Base-Free C(sp2)-H Arylsulfonylation of Enamides for Synthesis of (E)-beta-Amidovinyl Sulfones via the Insertion of Sulfur Dioxide, Org. Lett., 2021, 23, 4991–4996 CrossRef CAS PubMed;
(d) D. Sun and R. Zhang, Transition-metal-free, visible-light-induced oxidative cross-coupling for constructing β-acetylamino acrylosulfones from sodium sulfinates and enamides, Org. Chem. Front., 2018, 5, 92–97 RSC;
(e) X. Tu, J. Huang, W. Xie, T. Zhu and J. Wu, Generation of (E)-β-sulfonyl enamines from sulfur dioxide via a radical process, Org. Chem. Front., 2021, 8, 1789–1794 RSC;
(f) T.-H. Zhu, X.-C. Zhang, K. Zhao and T.-P. Loh, Cu(OTf)2-mediated C(sp2)–H arylsulfonylation of enamides via the insertion of sulfur dioxide, Org. Chem. Front., 2019, 6, 94–98 RSC.
-
(a) J. Lai, L. Chang and G. Yuan, I2/TBHP Mediated C-N and C-H Bond Cleavage of Tertiary Amines toward Selective Synthesis of Sulfonamides and beta-Arylsulfonyl Enamines: The Solvent Effect on Reaction, Org. Lett., 2016, 18, 3194–3197 CrossRef CAS PubMed;
(b) X. Rong, J. Guo, Z. Hu, L. Huang, Y. Gu, Y. Cai, G. Liang and Q. Xia, Iodine-Mediated Coupling of Cyclic Amines with Sulfonyl Hydrazides: an Efficient Synthesis of Vinyl Sulfone Derivatives, Eur. J. Org Chem., 2021, 4, 701–708 CrossRef;
(c) R. J. Griffiths, W. C. Kong, S. A. Richards, G. A. Burley, M. C. Willis and E. P. A. Talbot, Oxidative β-C–H sulfonylation of cyclic amines, Chem. Sci., 2018, 9, 2295–2300 RSC.
-
(a) M. Chen, Z.-T. Huang and Q.-Y. Zheng, Visible light-mediated dehydrogenative β-arylsulfonylation of tertiary aliphatic amines with arylsulfonyl chlorides, Org. Biomol. Chem., 2014, 12, 9337–9340 RSC;
(b) K. Muralirajan, R. Kancherla and M. Rueping, Dehydrogenative Aromatization and Sulfonylation of Pyrrolidines: Orthogonal Reactivity in Photoredox Catalysis, Angew. Chem., Int. Ed., 2018, 57, 14787–14791 CrossRef CAS PubMed;
(c) J. Tong, H. Li, Y. Zhu, P. Liu and P. Sun, Visible-light-induced dehydrogenative sulfonylation of tertiary amines under transition-metal- and photocatalyst-free conditions, Green Chem., 2022, 24, 1995–1999 RSC.
-
(a) L.-L. Wei, H. Xiong and R. P. Hsung, The Emergence of Allenamides in Organic Synthesis, Acc. Chem. Res., 2003, 36, 773–782 CrossRef CAS PubMed;
(b) T. Lu, Z. Lu, Z. X. Ma, Y. Zhang and R. P. Hsung, Allenamides: a powerful and versatile building block in organic synthesis, Chem. Rev., 2013, 113, 4862–4904 CrossRef CAS PubMed;
(c) R. Blieck, M. Taillefer and F. Monnier, Metal-Catalyzed Intermolecular Hydrofunctionalization of Allenes: Easy Access to Allylic Structures via the Selective Formation of C-N, C-C, and C-O Bonds, Chem. Rev., 2020, 120, 13545–13598 CrossRef CAS PubMed.
- N. Lu, Z. Zhang, N. Ma, C. Wu, G. Zhang, Q. Liu and T. Liu, Copper-Catalyzed Difunctionalization of Allenes with Sulfonyl Iodides Leading to (E)-alpha-Iodomethyl Vinylsulfones, Org. Lett., 2018, 20, 4318–4322 CrossRef CAS PubMed.
-
(a) K. Muralirajan, R. Kancherla and M. Rueping, Dehydrogenative Aromatization and Sulfonylation of Pyrrolidines: Orthogonal Reactivity in Photoredox Catalysis, Angew. Chem., Int. Ed., 2018, 57, 14787–14791 CrossRef CAS PubMed;
(b) G. Zhang, L. Zhang, H. Yi, Y. Luo, X. Qi, C.-H. Tung, L.-Z. Wu and A. Lei, Visible-light induced oxidant-free oxidative cross-coupling for constructing allylic sulfones from olefins and sulfinic acids, Chem. Commun., 2016, 52, 10407–10410 RSC;
(c) B. Lipp, L. M. Kammer, M. Kücükdisli, A. Luque, J. Kühlborn, S. Pusch, G. Matulevičiūtė, D. Schollmeyer, A. Šačkus and T. Opatz, Visible Light-Induced Sulfonylation/Arylation of Styrenes in a Double Radical Three-Component Photoredox Reaction, Chem. Eur. J., 2019, 25, 8965–8969 CrossRef CAS PubMed;
(d) Y. Yu, A. Liu, J. He, C. Wang, H. Mei and J. Han, Visible-light-irradiated tandem sulfonylation/cyclization of indole tethered alkenes for the synthesis of tetrahydrocarbazoles, Chin. Chem. Lett., 2022, 33, 4886–4890 CrossRef CAS;
(e) X.-X. Meng, Q.-Q. Kang, J.-Y. Zhang, Q. Li, W.-T. Wei and W.-M. He, Visible-light-initiated regioselective sulfonylation/cyclization of 1,6-enynes under photocatalyst- and additive-free conditions, Green Chem., 2020, 22, 1388–1392 RSC.
- For selected examples, see:
(a) J. Mahatthananchai, A. M. Dumas and J. W. Bode, Catalytic selective synthesis, Angew Chem. Int. Ed. Engl., 2012, 51, 10954–10990 CrossRef CAS PubMed;
(b) J. B. Peng and X. F. Wu, Ligand- and Solvent-Controlled Regio- and Chemodivergent Carbonylative Reactions, Angew Chem. Int. Ed. Engl., 2018, 57, 1152–1160 CrossRef CAS PubMed;
(c) V. Palani, M. A. Perea and R. Sarpong, Site-Selective Cross-Coupling of Polyhalogenated Arenes and Heteroarenes with Identical Halogen Groups, Chem. Rev., 2022, 122, 10126–10169 CrossRef CAS PubMed;
(d) I. P. Beletskaya, C. Najera and M. Yus, Chemodivergent reactions, Chem. Soc. Rev., 2020, 49, 7101–7166 RSC;
(e) A. M. Beauchemin, Site-selective reactions: Exploiting intramolecularity, Nat. Chem., 2013, 5, 731–732 CrossRef CAS PubMed.
-
(a) M.-H. Huang, Y.-L. Zhu, W.-J. Hao, A.-F. Wang, D.-C. Wang, F. Liu, P. Wei, S.-J. Tu and B. Jiang, Visible-Light Photocatalytic Bicyclization of 1,7-Enynes toward Functionalized Sulfone-Containing Benzo[a]fluoren-5-ones, Adv. Synth. Catal., 2017, 359, 2229–2234 CrossRef CAS;
(b) Y. L. Zhai, H. Zhou, Q. Q. Liu, B. R. Leng, Z. Zhang, J. Z. Li, D. C. Wang and Y. L. Zhu, Photocatalytic Markovnikov-type addition and cyclization of terminal alkynes leading to 4-sulfonyl quinoline-2(1H)-ones, Chem. Commun., 2022, 58, 5112–5115 RSC;
(c) Y. L. Zhu, B. Jiang, W. J. Hao, A. F. Wang, J. K. Qiu, P. Wei, D. C. Wang, G. Li and S. J. Tu, A new cascade halosulfonylation of 1,7-enynes toward 3,4-dihydroquinolin-2(1H)-ones via sulfonyl radical-triggered addition/6-exo-dig cyclization, Chem. Commun., 2016, 52, 1907–1910 RSC.
- Allenamides were known and prepared according to the published methods:
(a) I. Chakrabarty, S. M. Inamdar, M. O. Akram, A. B. Gade, S. Banerjee, S. Bera and N. T. Patil, [3+2]-Annulation of platinum-bound azomethine ylides with distal C
C bonds of N-allenamides, Chem. Commun., 2017, 53, 196–199 RSC;
(b) S. Das, A. Azim, S. K. Hota, S. P. Panda, S. Murarka and S. De Sarkar, An organophotoredox-catalyzed redox-neutral cascade involving N-(acyloxy)phthalimides and allenamides: synthesis of indoles, Chem. Commun., 2021, 57, 13130–13133 RSC;
(c) S. Banerjee, B. Senthilkumar and N. T. Patil, Gold-Catalyzed 1,2-Oxyalkynylation of N-Allenamides with Ethylnylbenziodoxolones, Org. Lett., 2018, 21, 180–184 CrossRef PubMed;
(d) W.-F. Zheng, P. P. Bora, G.-J. Sun and Q. Kang, Rhodium-Catalyzed Regio- and Stereoselective [2 + 2] Cycloaddition of Allenamides, Org. Lett., 2016, 18, 3694–3697 CrossRef CAS PubMed. - Aryl Sulfinates were known and prepared according to the published methods:
(a) G. Bogonda, D. V. Patil, H. Y. Kim and K. Oh, Visible-Light-Promoted Thiyl Radical Generation from Sodium Sulfinates: A Radical–Radical Coupling to Thioesters, Org. Lett., 2019, 21, 3774–3779 CrossRef CAS PubMed;
(b) A. U. Meyer, S. Jäger, D. Prasad Hari and B. König, Visible Light-Mediated Metal-Free Synthesis of Vinyl Sulfones from Aryl Sulfinates, Adv. Synth. Catal., 2015, 357, 2050–2054 CrossRef CAS.
-
(a) O. A. Storozhenko, A. A. Festa, G. I. Detistova, V. B. Rybakov, A. V. Varlamov, E. V. Van der Eycken and L. G. Voskressensky, Photoredox-Catalyzed Hydrosulfonylation of Arylallenes, J. Org. Chem., 2020, 85, 2250–2259 CrossRef CAS PubMed;
(b) Z. Xu, J. Jiang, Q. Lu, J. Chen, S. Chen, L. Shi and Z. Yimei, Photoredox Catalyzed Sulfonylation of Multisubstituted Allenes with Ru(bpy)3Cl2 or Rhodamine B, J. Organomet. Chem., 2022, 957, 122125 CrossRef CAS;
(c) J. Zhu, W. C. Yang, X. d. Wang and L. Wu, Photoredox Catalysis in C–S Bond Construction: Recent Progress in Photo-Catalyzed Formation of Sulfones and Sulfoxides, Adv. Synth. Catal., 2017, 360, 386–400 CrossRef;
(d) D. Singla, V. Luxami and K. Paul, Eosin Y mediated photo-catalytic C–H functionalization: C–C and C–S bond formation, Org. Chem. Front., 2023, 10, 237–266 RSC.
Footnotes |
† Electronic supplementary information (ESI) available. CCDC 2303358. For ESI and crystallographic data in CIF or other electronic format see DOI: https://doi.org/10.1039/d3ra08272e |
‡ These authors contributed equally. |
|
This journal is © The Royal Society of Chemistry 2024 |
Click here to see how this site uses Cookies. View our privacy policy here.