DOI:
10.1039/D4RA01681E
(Paper)
RSC Adv., 2024,
14, 14767-14774
Three-component synthesis of pyran-fused biscoumarins: an entry to pyridinone- and pyranone-fused coumarins†
Received
4th March 2024
, Accepted 30th April 2024
First published on 7th May 2024
Abstract
A base-mediated, three-component synthesis of symmetric and unsymmetric pyran-fused biscoumarins via the coupling of 4-hydroxycoumarin with 4-chloro-3-formylcoumarin in ethanol is reported. This methodology is further extended to the construction of various indole/coumarin-substituted symmetric pyran-fused biscoumarins. The unsymmetric pyran-fused biscoumarin can react with amines and 4-hydroxycoumarins under basic and acidic conditions to afford pyridinone-fused coumarins and pyranone-fused coumarins, respectively. The mechanisms of their formation are proposed.
1 Introduction
Coumarin-fused heterocycles are a class of organic compounds that combine the fused-ring structure of coumarins with one or more heterocyclic rings. The fusion of coumarins with heterocycles can result in molecules with a diverse range of biological activities. For instance, the pyran-fused biscoumarin 1 belongs to the class of nonpeptidic HIV protease inhibitors.1 Pyridinone coumarin conjugate 2a is an adenosine receptor,2a and pyridinone coumarin conjugate 2b exhibits mild anti-microbial activity against both Gram positive and negative bacteria.2b Frutinone A (3) is an active ingredient extracted from the lipophilic fraction of the Polygala fruticosa, demonstrating various anti-fungal properties.3 Pyranone coumarin conjugate 4 exhibits good anti-fungal activity against B. cinerea and C. capsica (Fig. 1).4
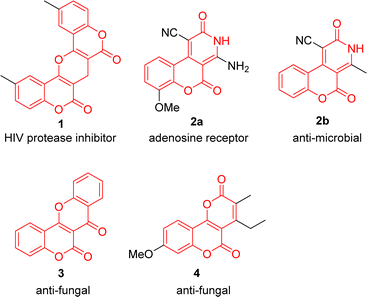 |
| Fig. 1 Representative structures of biologically active coumarin-fused heterocycles 1–4. | |
In addition to their biological activities, coumarin-fused heterocycles also have a wide range of potential applications in organic functional materials. For example, pyranocoumarin 5 possesses photochromic properties.5 Pyrrolocoumarin 6 serves as a light-sensitive organic redox switch.6 Pyrrolocoumarin 7 is electrochromic and changes color when an external potential is applied (Fig. 2).7
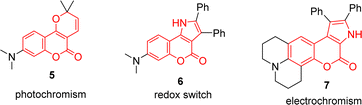 |
| Fig. 2 Representative structures of coumarin-fused heterocycles 5–7 with functional properties. | |
Owing to their potential as therapeutic agents and functional materials, the synthesis of coumarin-fused heterocycles has become an active area of research. While various methodologies for the preparation of pyranocoumarin derivatives have been reported in the literature,8 the development of multi-component synthesis of these compounds from readily available precursors is still needed to facilitate the progress of unearthing their unprecedented properties. Here we report the three-component synthesis of two isomeric pyran-fused biscoumarins via base-promoted reaction of 4-hydroxycoumarin with 4-chloro-formylcoumarin in ethanol. Subsequent investigation of their chemical reactivity toward nucleophilic reagents such as amines and 4-hydroxycoumarins under acidic and basic conditions are also explored.
2 Results and discussion
We began our study by coupling of 4-hydroxycoumarin (8) with 4-chloro-formylcoumarin (9) in the presence of triethylamine as a base in ethanol under room temperature for 24 hours to give compound 10 in 30% yield as a minor product and compound 11 in 45% as a major product, as shown in Scheme 1. The structures of isomeric pyran-fused biscoumarins 10 and 11 could be easily differentiated by their proton NMR spectra. The characteristic singlet absorption peak that appeared at a chemical shift of 5.59 ppm was assigned to the pyran hydrogen for the biscoumarin 10, whereas the singlet absorption peak that appeared at a more downfield shift of 6.53 ppm was assigned to the acetal hydrogen for the biscoumarin 11. The molecular structures of both 10 and 11 were further unambiguously characterized by the X-ray crystallography as shown in Scheme 1.9
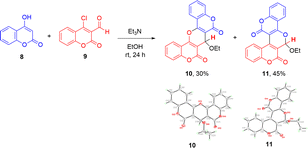 |
| Scheme 1 Three-component synthesis of isomeric biscoumarins 10 and 11, and ORTEP crystal structures of 10 and 11 with atomic displacement shown at 50% probability. | |
Table 1 summarizes the optimization for the regioselective preparation of the biscoumarins 10 and 11 in ethanol at room temperature. When an organic base such as triethylamine was employed, as shown in Scheme 1, a mixture of both isomers 10 and 11 were received (Table 1, entry 1). Interestingly, when DABCO was used as a base, the biscoumarin 10 was obtained as an exclusive product in 35% and 50% yields for 12 and 24 h, respectively (Table 1, entries 2 and 3). On the other hand, biscoumarin 11 was obtained as the major product even though in low yields when K2CO3 or Na2CO3 was employed as the inorganic base (Table 1, entries 4 and 5). When Cs2CO3 was used as a base, to our delight, we observed the selective formation of 11 in 43% for 12 h at room temperature (Table 1, entry 6). Prolonged reaction time from 12 h to 24 h increased the yield of biscoumarin 11 from 43% to 72%, along with only about 5% of biscoumarin 10 (Table 1, entry 7).
Table 1 Optimization for the synthesis of biscoumarins 10 and 11a
Entry |
Base |
Time (h) |
Yield (%) (10) |
Yield (%) (11) |
Reaction conditions: 8 (0.31 mmol, 1.0 equiv.), 9 (0.31 mmol, 1.0 equiv.), base (0.32 mmol, 1.0 equiv.), ethanol (2.5 mL) at rt. |
1 |
Et3N |
24 |
30 |
45 |
2 |
DABCO |
12 |
35 |
— |
3 |
DABCO |
24 |
50 |
— |
4 |
K2CO3 |
24 |
Trace |
15 |
5 |
Na2CO3 |
24 |
Trace |
12 |
6 |
Cs2CO3 |
12 |
Trace |
43 |
7 |
Cs2CO3 |
24 |
5 |
72 |
Scheme 2 outlines the proposed mechanism for the formation of biscoumarins 10 and 11 via this three-component reaction.10 In Path A, it presumably starts with 1,2-addition of 4-hydroxycoumarin (8) with 4-chloro-formylcoumarin (9) to give the alcohol A, and follows by cyclization to generate the intermediate B. The subsequent aromatization of B via elimination of chloride ion and H2O yields the biscoumarin-fused pyrylium ion C. Final 1,4-addition of ethanol to C affords the biscoumarin 10. In Path B, the reaction begins with preferential 1,4-addition of 4-hydroxycoumarin (8) with 4-chloro-formylcoumarin (9) in the presence of Cs2CO3 to give the aldehyde D, and follows by cyclization to furnish the hemiacetal E. The subsequent elimination of chloride ion and H2O of E yields the biscoumarin-fused pyrylium ion F. Final 1,2-addition of ethanol to F furnishes the biscoumarin 11.
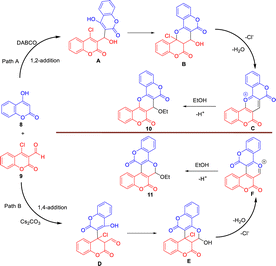 |
| Scheme 2 Proposed mechanism for the formation of compounds 10 and 11. | |
With biscoumarins 10 and 11 in hand, we then investigated their chemical reactivity toward some common nucleophilic agents. When biscoumarin 10 was reacted with 4-hydroxycoumarin in the presence of triethylamine as a base in toluene under reflux conditions for 24 h, the coumarin-substituted pyran-fused biscoumarin 12a was obtained in 68% yield (Scheme 3). Later, we found that this 12a could also be prepared under the same reaction conditions via a pseudo three-component reaction of two equivalents of 4-hydroxycoumairn with 4-chloro-formylcoumarin (9) in 78% yield. Scheme 4 lists the structures and yields of the prepared pyran-fused biscoumarins 12a–e.11 In addition to 4-chloro-3-formylcoumarin (9), 3-chloro-3-phenylacrylaldehyde could also serve as a valid substrate to give the corresponding products 12c and 12e in good yields. The molecular structures of compounds 12a and 12d were further confirmed by the X-ray crystal analysis as shown in Scheme 4.9 While it has been previously documented that compound 12a can also be synthesized via the same two substrates under acidic conditions in aqueous media,11b here we provide alternate basic conditions for its preparation and further extend the substrate scope from 4-chloro-3-formylcoumarin to 3-chloro-3-phenylacrylaldehyde derivatives.
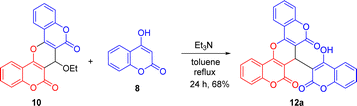 |
| Scheme 3 Synthesis of pyran-fused biscoumarin 12a. | |
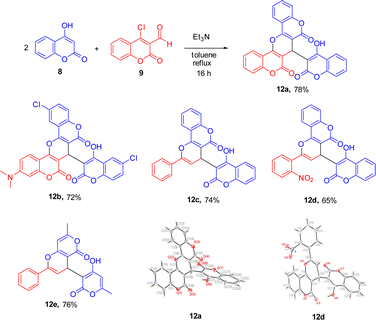 |
| Scheme 4 Synthesis of pyranocoumarins 12a–e. | |
In an effort to expand the scope of this reaction from a pseudo three-component reaction to a three-component one, indole (13) was introduced as a third substrate for this potential multi-component reaction (MCR). To our delight, the biscoumarin 14a was obtained via triethylamine-mediated MCR of 4-hydroxycoumairn (8), 4-chloro-3-formyl-7-methoxycoumarin (9a) and indole (13) in 71% yield. Scheme 5 lists the structures and yields of the prepared pyran-fused biscoumarins 14a–d along with the X-ray crystal structure of compound 14b.9 The formation mechanism of this MCR product presumably follows the reaction steps outlined in Scheme 2, except that the final pyrylium ion is captured by indole instead of ethanol, giving the product 14.
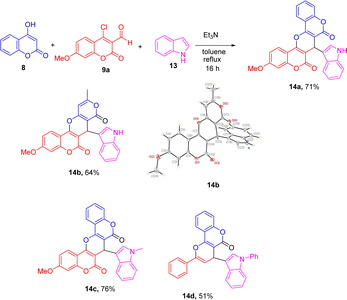 |
| Scheme 5 Synthesis of pyranocoumarins 14a–d, and X-ray crystal structure of 14b. | |
Interestingly, when biscoumarin 11 was reacted with amines (15) under the same conditions as the preparation of compounds 12, the novel pyridinone-fused coumarins 16 were isolated in fair to excellent yields (Scheme 6). On the proton NMR spectra, intramolecular hydrogen bonding absorption peaks appearing at 11.87–11.75 ppm and singlet pyridinone hydrogen absorption peaks appearing at 9.02–8.77 ppm were observed for all prepared compounds 16. Scheme 6 lists the structures and yields of the prepared pyridinone-fused coumarins 16a–e along with the X-ray crystal structure of 16e.9 While both aniline and benzylamine could serve as valid nucleophilic agents to react with compound 11, the yield of the former was found to be much better than that of the latter.
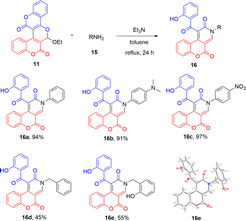 |
| Scheme 6 Preparation of pyridinone-fused coumarins 16a–e, and the X-ray crystal structure of 16e. | |
Scheme 7 outlines the proposed mechanism for the formation of 16a from 11 and aniline. It begins with the elimination of ethoxide ion from 11 to form the pyrylium ion G. The 1,2-addition of aniline nitrogen onto the pyrylium ion G yields the pyranoamine H, which then undergoes ring opening to give the imine I. The subsequent enol–keto tautomerization of the imine I generates the keto J. The [1,5] shift of C-3 hydrogen on coumarin to the imine nitrogen of J furnishes the enamine K. Final intramolecular lactamization of K affords the product 16a. Note that formation of proposed intermediate I appeared to be favored when aniline was used as the nucleophile, presumably due to the favorable extended conjugation. This may explain why using aniline as the nucleophile in this reaction gives better yields than benzylamine.
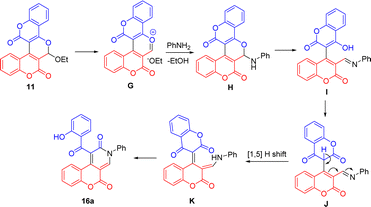 |
| Scheme 7 Proposed mechanism for the formation of compound 16a. | |
On the other hand, when compound 11 was reacted with 4-hydroxycoumarin (8) and 7-N,N-dimethylamino-4-hydroxycoumarin (8a) in the presence of one equivalent of camphorsulfonic acid in wet acetonitrile under reflux conditions for 8 h, the major products isolated were found to be the benzopyrano[3,2-c]coumarin-linked pyranone-fused coumarins 17a and 17b, respectively (Scheme 8). The characteristic singlet absorption peaks for pyranone hydrogens of 17a and 17b that appeared at chemical shifts of 8.76 and 8.68 ppm were clearly observed on the proton NMR spectra. The molecular structure of the pyranone-fused coumarin 17a was further confirmed by the X-ray crystallography as shown in Scheme 8.9
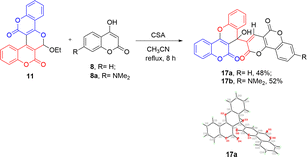 |
| Scheme 8 Synthesis of pyranone-fused coumarins 17a and 17b, and X-ray crystal structure of 17a with atomic displacement shown at 50% probability. | |
Scheme 9 depicts the proposed mechanism for the formation of 17a from 11 and 8. It begins with protonation of acetal oxygen of 11 to give the oxonium ion L. The elimination of ethanol from L yields the pyrylium salt M. The subsequent nucleophilic attack of 4-hydroxycoumarin to the oxonium carbon of M generates the pyran N. The electrocyclic reaction to open up the pyran ring of N furnishes the α,β-unsaturated keto P, which is subjected to the conjugate addition by H2O to give the alcohol Q. Final intramolecular cyclization of Q via elimination of one molecule of H2O affords the compound 17a. This reaction provides quick access to compounds with the linkage of two biologically active molecular scaffolds; that is, benzopyrano[3,2-c]coumarin and pyranone-fused coumarin. Since hybridization of two potent pharmacophores into a single molecule is one of the current strategies to synthesize compounds with improved pharmacological activity,12 the discovery of biological activity from these readily available coumarin-fused heterocycles is highly anticipated.
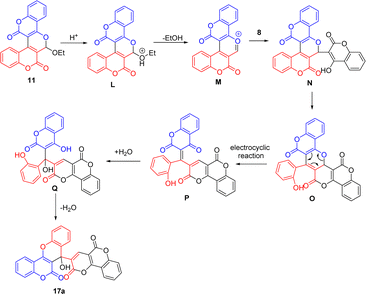 |
| Scheme 9 Proposed mechanism for the formation of compound 17a. | |
3 Conclusions
In summary, we have developed a base-mediated, three-component reaction for the construction of symmetric and unsymmetric pyran-fused biscoumarins via the coupling of 4-hydroxycoumarin with 4-chloro-3-formylcoumarin in ethanol. The symmetric and unsymmetric pyran-fused biscoumarins could serve as precursors to react with different nucleophilic agents including 4-hydroxycoumarins, indoles, and amines to afford products such as substituted biscoumarins, pyridinone-fused coumarins, and pyranone-fused coumarins. The biological activities of those prepared compounds are currently under investigation.
4 Experimental section
4.1. General information
Melting points were determined on a Mel-Temp melting point apparatus in open capillaries and are uncorrected. Infrared (IR) spectra were recorded using 1725XFT-IR spectrophotometer. High resolution mass spectra (HRMS) were obtained on a Thermo Fisher Scientific Finnigan MAT95XL spectrometer using magnetic sector analyzer. 1H NMR (400 MHz) and 13C NMR (100) spectra were recorded on a Bruker 400 spectrometer. Chemical shifts were reported in parts per million on the scale relative to an internal standard (tetramethylsilane, or appropriate solvent peaks) with coupling constants given in hertz. 1H NMR multiplicity data are denoted by s (singlet), d (doublet), t (triplet), q (quartet), m (multiplet). Analytical thin-layer chromatography (TLC) was carried out on Merck silica gel 60G-254 plates (25 mm) and developed with the solvents mentioned. Visualization was accomplished by using portable UV light, ninhydrin spray, or iodine chamber. Flash chromatography was performed in columns of various diameters with Merck silica gel (230–400 mesh ASTM 9385 kieselgel 60H) by elution with the solvent systems. Solvents, unless otherwise specified, were reagent grade and distilled once prior to use. All new compounds exhibited satisfactory spectroscopic and analytical data.
4.2. Procedure for preparation of compound 10
To a 10 mL round bottom flask equipped with a magnetic stirrer was charged with 4-hydroxycoumarin (8, 0.31 mmol, 1.0 equiv.), 4-chloro-3-formylcoumarin (9, 0.31 mmol, 1.0 equiv.) and DABCO (0.32 mmol, 1.0 equiv.) in ethanol (2.5 mL) at room temperature. The resulting mixture was then stirred at that temperature for 24 h. Upon completion of the reaction (monitored by TLC) and the solvent was concentrated under reduced pressure. The residue was purified by column chromatography with ethyl acetate and hexane as the eluent to afford a pale yellow solid of compound 10 (56 mg; yield 50%). Rf = 0.4 (2% MeOH/DCM); mp 231–233 °C; 1H NMR (CDCl3, 400 MHz): δ 8.07 (d, J = 7.2 Hz, 2H), 7.68 (t, J = 7.2 Hz, 2H), 7.47–7.43 (m, 4H), 5.57 (s, 1H), 4.27 (q, J = 7.2 Hz, 2H), 1.20 (t, J = 7.2 Hz, 3H); 13C{1H} NMR (100 MHz, CDCl3): δ 161.1, 155.0, 152.9, 133.5, 124.9, 122.7, 117.4, 113.5, 105.1, 70.4, 62.1, 15.9; IR νmax (neat): 2924, 1727, 1713, 1670, 1610, 1398, 1192, 1059, 1044, 889, 759 cm−1; HRMS (EI) m/z: [M]+ calcd for C21H14O6 362.0790; found 362.0801.
4.3. Procedure for preparation of compound 11
To a 10 mL round bottom flask equipped with a magnetic stirrer was charged with 4-hydroxycoumarin (8, 0.31 mmol, 1.0 equiv.), 4-chloro-3-formylcoumarin (9, 0.31 mmol, 1.0 equiv.) and Cs2CO3 (0.32 mmol, 1.0 equiv.) in ethanol (2.5 mL) at room temperature. The resulting mixture was then stirred at that temperature for 24 h. Upon completion of the reaction (monitored by TLC) and the solvent was concentrated under reduced pressure. The residue was purified by column chromatography with ethyl acetate and hexane as the eluent to afford a pale yellow solid of compound 11 (81 mg; yield 72%). Rf = 0.6 (2% MeOH/DCM); 51 mg; yield 46%; mp 194–196 °C; 1H NMR (CDCl3, 400 MHz): δ 8.05 (dd, J = 7.6, 1.2 Hz, 1H), 8.02 (dd, J = 8.4, 1.2 Hz, 1H), 7.71 (td, J = 8.4, 1.2 Hz, 1H), 7.57 (td, J = 8.4, 1.2 Hz, 1H), 7.46–7.38 (m, 3H), 7.30 (td, J = 8.4, 1.2 Hz, 1H), 6.55 (s, 1H), 4.04–3.91 (m, 2H), 1.23 (t, J = 7.2 Hz, 3H); 13C{1H} NMR (100 MHz, CDCl3): δ 164.1, 158.9, 158.8, 154.1, 153.8, 139.6, 134.6, 132.5, 128.8, 124.9, 123.9, 123.8, 117.3, 117.1, 115.2, 114.9, 114.4, 101.2, 96.5, 66.3, 15.2; IR νmax (neat): 2990, 1709, 1608, 1533, 1453, 1403, 1061, 1036, 913, 862, 748, 737 cm−1; HRMS (EI) m/z: [M]+ calcd for C21H14O6 362.0790; found 362.0796.
4.4. Procedure for preparation of compound 12a via two-component reaction
To a stirred solution of 4-hydroxycoumarins (8, 0.27 mmol, 1.0 equiv.) and 7-ethoxy-6H,7H,8H-pyrano[3,2-c:5,6-c′]dichromene-6,8-dione (10, 0.27 mmol, 1.0 equiv.) in toluene was added triethylamine (0.13 mmol, 0.5 equiv.) dropwise at room temperature. The resulting mixture was then heated to reflux for 24 h. Upon completion of the reaction (monitored by TLC), the reaction mixture was cooled to room temperature. The resulting solid was filtered and washed with hexanes and diethyl ether (5 mL × 3 times each), and the solid was dried under vacuum to get the desired product.
4.5. General procedure for preparation of compound 12a–e via pseudo-3-component reaction
To a stirred solution of 4-hydroxycoumarins (8, 0.48 mmol, 2.0 equiv.) and 4-chloro-3-formylcoumarins or 3-chloro-3-phenylacrylaldehyde (0.24 mmol, 1.0 equiv.) in toluene was added triethylamine (0.12 mmol, 0.5 equiv.) dropwise at room temperature. The resulting mixture was then heated to reflux for 16 h. Upon completion of the reaction (monitored by TLC), the reaction mixture was cooled to room temperature. The resulting solid was filtered and washed with hexanes and diethyl ether (5 mL × 3 times each), and the solid was dried under vacuum to get the desired product.
4.5.1 7-(4-Hydroxy-2-oxo-2H-chromen-3-yl)-6H-pyrano[3,2-c:5,6-c′]dichromene-6,8(7H)-dione (12a). Rf = 0.3 (5% MeOH/CH2Cl2); white solid; 90 mg; yield 78%; mp > 300 °C; 1H NMR (DMSO-d6, 400 MHz): δ 12.35 (brs, 1H), 8.39 (dd, J = 7.6, 1.2 Hz, 2H), 8.04 (d, J = 8.0 Hz, 1H), 7.77 (td, J = 8.8, 1.6 Hz, 2H), 7.61 (td, J = 8.8, 1.6 Hz, 1H); 7.56–7.50 (m, 4H), 7.37 (t, J = 7.6 Hz, 1H), 7.31 (d, J = 8.0 Hz, 1H), 5.56 (s, 1H); 13C{1H} NMR (100 MHz, DMSO-d6): δ 161.6, 160.1 (3C), 155.2, 152.8, 152.4 (2C), 133.5 (3C), 132.8, 125.4 (3H), 124.4, 123.6 (3H), 117.0 (3H), 116.7, 113.5 (3H), 102.9, 25.8; IR νmax (KBr): 3357, 3191, 2920, 2850, 1715, 1667, 1609, 1519, 1372, 1186, 1044, 759 cm−1; HRMS (EI) m/z: [M]+ calcd for C28H14O8 478.0689; found 478.0681.
4.5.2 2-Chloro-7-(6-chloro-4-hydroxy-2-oxo-2H-chromen-3-yl)-11-(dimethylamino)-6H-pyrano[3,2-c:5,6-c′]dichromene-6,8(7H)-dione (12b). Rf = 0.3 (5% MeOH/CH2Cl2); brown solid; 103 mg; yield 72%; mp > 300 °C; 1H NMR (DMSO-d6, 400 MHz): δ 9.77 (brs, 1H), 8.12 (d, J = 2.0 Hz, 1H), 8.00 (d, J = 8.8 Hz, 1H), 7.63–7.59 (m, 2H), 7.39 (d, J = 8.8 Hz, 1H), 7.33 (dd, J = 8.8, 2.4 Hz, 1H) 7.06 (d, J = 8.8 Hz, 1H), 6.62 (d, J = 8.8 Hz, 1H), 6.40 (d, J = 2.0 Hz, 1H), 5.16 (s, 1H), 2.96 (s, 6H); 13C{1H} NMR (100 MHz, DMSO-d6): δ 163.0, 160.8 (3C), 159.7, 154.8, 154.2, 153.0, 152.9, 152.6, 150.6 (2C), 132.0, 129.7, 129.1, 126.3, 125.1, 124.2, 124.1, 122.2, 118.6, 117.9, 115.5, 109.2, 105.4, 102.1, 98.6, 97.3, 40.1, 25.1; IR νmax (KBr): 3350, 2946, 2912, 1659, 1657, 1615, 1519, 1399, 1374, 1182, 795 cm−1; HRMS (EI) m/z: [M]+ calcd for C30H17Cl2NO8 589.0331; found 589.0326.
4.5.3 4-(4-Hydroxy-2-oxo-2H-chromen-3-yl)-2-phenylpyrano[3,2-c]chromen-5(4H)-one (12c). Rf = 0.5 (5% MeOH/CH2Cl2); off-white solid; 96 mg; yield 74%; mp 231–233 °C; 1H NMR (DMSO-d6, 400 MHz): δ 8.12 (dd, J = 8.0, 1.2 Hz, 1H), 8.00 (d, J = 7.6 Hz, 1H), 7.86 (d, J = 7.6 Hz, 1H), 7.70 (td, J = 7.6, 1.2 Hz, 1H), 7.61 (td, J = 8.0, 1.2 Hz, 1H), 7.52–7.38 (m, 5H), 7.35 (d, J = 8.4 Hz, 1H), 5.93 (d, J = 4.4 Hz, 1H), 5.16 (d, J = 4.4 Hz, 1H); 13C{1H} NMR (100 MHz, DMSO-d6): δ 161.8, 161.5, 161.0, 156.9, 152.7, 152.5, 147.4, 132.9, 132.7, 132.6, 129.5, 129.2, 129.0, 125.1, 124.7, 124.4, 124.0, 123.1, 117.0, 116.7, 114.4, 105.9, 101.4, 100.6, 27.1; IR νmax (KBr): 3418, 3252, 3070, 2952, 2929, 1718, 1627, 1610, 1395, 1207, 760 cm−1; HRMS (EI) m/z: [M]+ calcd for C27H16O6 436.0947; found 436.0943.
4.5.4 4-(4-Hydroxy-2-oxo-2H-chromen-3-yl)-2-(2-nitrophenyl)pyrano[3,2-c]chromen-5(4H)-one (12d). Rf = 0.5 (5% MeOH/CH2Cl2); off-white solid; 74 mg; yield 65%; mp 247–249 °C; 1H NMR (DMSO-d6, 400 MHz): δ 8.04–8.01 (m, 2H), 7.76–7.60 (m, 5H), 7.55 (dd, J = 8.0, 1.2 Hz, 1H), 7.45–7.34 (m, 4H), 5.79–5.77 (m, 1H), 5.17–5.16 (m, 1H); 13C{1H} NMR (100 MHz, DMSO-d6): δ 161.8, 160.8 (2C), 156.9, 152.7, 152.4, 148.2, 146.3, 133.7, 133.1, 132.7, 131.5, 131.3, 127.2, 125.1, 124.7 (2C), 124.4, 124.1, 122.2, 117.1, 116.7 (2C), 113.8, 105.3, 101.3, 27.3; IR νmax (KBr): 3357, 2956, 2917, 2846, 1709, 1657, 1629, 1607, 1528, 1390, 1207, 754 cm−1; HRMS (EI) m/z: [M]+ calcd for C27H15NO8 481.0798; found 481.0792.
4.5.5 4-(4-Hydroxy-6-methyl-2-oxo-2H-pyran-3-yl)-7-methyl-2-phenylpyrano[4,3-b]pyran-5(4H)-one (12e). Rf = 0.5 (5% MeOH/CH2Cl2); off-white solid; 81 mg; yield 76%; mp 174–176 °C; 1H NMR (DMSO-d6, 400 MHz): δ 11.45 (s, 1H), 7.67–7.64 (m, 2H), 7.43–7.36 (m, 3H), 6.30 (s, 1H), 6.00 (s, 1H), 5.65 (d, J = 4.8 Hz, 1H), 4.66 (d, J = 4.8 Hz, 1H), 2.21 (s, 3H), 2.12 (s, 3H); 13C{1H} NMR (100 MHz, DMSO-d6): δ 162.6 (2C), 161.8, 161.6, 161.5 (2C), 132.7, 129.2, 129.0 (2C), 124.5 (2C), 102.3, 100.8, 100.5, 99.3, 98.1, 88.5, 25.3, 19.8, 19.7; IR νmax (KBr): 3192, 2950, 2917, 2846, 1700, 1659, 1640, 1577, 1448, 1404, 1278, 1190; 760 cm−1; HRMS (EI) m/z: [M]+ calcd for C21H16O6 364.0947; found 364.0939.
4.6. General procedure for preparation of compounds 14a–d via three-component reaction
To a stirred solution of 4-hydroxycoumarin (8, 0.31 mmol, 1.0 equiv.), and 7-methoxy-4-chloro-3-formylcoumarin or 3-chloro-3-phenylacrylaldehyde (0.31 mmol, 1.0 equiv.) in toluene was added indole (13a, 0.31 mmol, 1.0 equiv.) followed by triethylamine (0.15 mmol, 0.5 equiv.) dropwise at room temperature. The resulting mixture was then heated to reflux for 16 h. Upon completion of the reaction (monitored by TLC), the reaction mixture was cooled to room temperature. The resulting solid was filtered and washed with hexanes and diethyl ether (5 mL × 3 times each), and the solid was dried under vacuum to get the desired product.
4.6.1 7-(1H-Indol-3-yl)-3-methoxy-6H-pyrano[3,2-c:5,6-c′]dichromene-6,8(7H)-dione (14a). Rf = 0.3 (5% MeOH/CH2Cl2); off-white solid; 102 mg; yield 71%; mp > 300 °C; 1H NMR (DMSO-d6, 400 MHz): δ 11.06 (s, 1H), 8.36 (d, J = 7.6 Hz, 1H), 8.26 (d, J = 8.4 Hz, 1H), 7.72 (t, J = 7.6 Hz, 1H), 7.51 (t, J = 7.6 Hz, 1H), 7.44–7.41 (m, 2H), 7.32–7.30 (m, 2H), 7.07–6.99 (m, 3H), 6.90 (t, J = 7.6 Hz, 1H), 5.10 (s, 1H), 3.87 (s, 3H); 13C{1H} NMR (100 MHz, DMSO-d6): δ 161.6, 160.1 (3C), 155.2, 152.8, 152.4 (2C), 133.5 (3C), 132.8, 125.4 (3H), 124.4, 123.6 (3H), 117.0 (3H), 116.7, 113.5 (3H), 102.9, 25.8; IR νmax (KBr): 3412, 2950, 2912, 1731, 1665, 1615, 1371, 1267, 1171, 1150, 1050, 735 cm−1; HRMS (EI) m/z: [M]+ calcd for C28H17NO6 463.1056; found 463.1051.
4.6.2 7-(1H-Indol-3-yl)-3-methoxy-10-methyl-6H-pyrano[3′,4′:5,6]pyrano[3,2-c]chromene-6,8(7H)-dione (14b). Rf = 0.6 (5% MeOH/CH2Cl2); off-white solid; 108 mg; yield 64%; mp 272–274 °C; 1H NMR (DMSO-d6, 400 MHz): δ 11.02 (s, 1H), 7.90 (d, J = 8.4 Hz, 1H), 7.33 (t, J = 8.4 Hz, 2H), 7.25 (d, J = 2.0 Hz, 1H), 7.08–6.99 (m, 3H), 6.90 (t, J = 7.6 Hz, 1H), 6.60 (s, 1H), 4.95 (s, 1H), 3.85 (s, 3H), 2.25 (s, 3H); 13C{1H} NMR (100 MHz, DMSO-d6): δ 163.5, 162.8, 162.0, 160.5, 158.1, 154.2, 153.8, 136.7, 126.0, 125.6, 124.1, 121.2, 119.2, 118.4, 115.4, 113.3, 112.2, 106.5, 102.8, 102.5, 101.3, 98.6, 56.5, 25.7, 19.9; IR νmax (KBr): 3357, 2956, 2920, 2850, 1723, 1630, 1615, 1377, 1268, 1154, 1027, 742 cm−1; HRMS (EI) m/z: [M]+ calcd for C25H17NO6 427.1056; found 427.1046.
4.6.3 3-Methoxy-7-(1-methyl-1H-indol-3-yl)-6H-pyrano[3,2-c:5,6-c′]dichromene-6,8(7H)-dione (14c). Rf = 0.5 (60% EtOAc/hexanes); off-white solid; 110 mg; yield 76%; mp 298–300 °C; 1H NMR (DMSO-d6, 400 MHz): δ 8.34 (d, J = 7.6 Hz, 1H), 8.24 (d, J = 8.4 Hz, 1H), 7.72 (t, J = 8.0 Hz, 1H), 7.53–7.44 (m, 4H), 7.34–7.28 (m, 1H), 7.10–7.03 (m, 3H), 6.96 (t, J = 7.2 Hz, 1H), 5.06 (s, 1H), 3.87 (s, 3H), 3.67 (s, 3H); 13C{1H} NMR (100 MHz, DMSO-d6): δ 163.6, 160.5, 160.1, 154.3, 153.5, 153.0, 152.3, 136.9, 133.4, 130.0, 126.7, 125.3, 124.6, 123.5, 121.5, 119.4, 119.0, 117, 115.3, 113.6, 113.5, 110.3, 106.5, 106.0, 102.9, 101.2, 56.5, 32.8, 25.8; IR νmax (KBr): 3361, 2956, 2922, 2852, 1731, 1666, 1615, 1369, 1272, 1153, 754 cm−1; HRMS (EI) m/z: [M]+ calcd for C29H19NO6 477.1212; found 477.1219.
4.6.4 2-Phenyl-4-(1-phenyl-1H-indol-3-yl)-4H,5H-pyrano[3,2-c]chromen-5-one (14d). Rf = 0.5 (50% EtOAc/hexanes); off-white solid; 74 mg; yield 51%; mp 154–156 °C; 1H NMR (DMSO-d6, 400 MHz): δ 8.18 (d, J = 8.0 Hz, 1H), 7.87 (d, J = 7.6 Hz, 2H), 7.73–7.68 (m, 2H), 7.63 (s, 1H), 7.55–7.51 (m, 6H), 7.49–7.42 (m, 4H), 7.37–7.34 (m, 1H), 7.17 (t, J = 7.6 Hz, 1H), 7.09 (t, J = 7.6 Hz, 1H), 6.27 (d, J = 4.8 Hz, 1H), 5.00 (d, J = 4.8 Hz, 1H); 13C{1H} NMR (100 MHz, DMSO-d6): δ 161.0, 155.9, 152.6, 145.9, 139.3, 135.9, 132.9, 132.5, 130.2, 129.6, 129.3, 127.8, 127.5, 126.8, 125.1, 124.8, 124.1, 123.3, 122.9, 120.7, 119.8, 119.5, 117.0, 114.5, 111.1, 103.9, 102.8, 27.6; IR νmax (KBr): 3565, 3015, 2957, 2871, 1718, 1629, 1596, 1499, 1456, 1012, 751 cm−1; HRMS (EI) m/z: [M]+ calcd for C32H23NO3 467.1521; found 467.1516.
4.7. General procedure for preparation of compounds 16a–e
To a 10 mL round bottom flask equipped with a magnetic stirrer and a reflux condenser was charged with 11 (0.14 mmol, 1.0 equiv.), amine (0.14 mmol, 1.0 equiv.) and triethylamine (0.14 mmol, 1.0 equiv.) in toluene (3.0 mL) at room temperature. The reaction mixture was heated at 100 °C in an oil bath for 24 h. Upon completion of the reaction (monitored by TLC), the reaction mixture was cooled to room temperature, and the solvent was concentrated under reduced pressure. The residue was purified by column chromatography using ethyl acetate and hexane as the eluent to give the desired products.
4.7.1 1-(2-Hydroxybenzoyl)-3-phenyl-2H-chromeno[3,4-c]pyridine-2,5(3H)-dione (16a). Rf = 0.6 (40% EtOAc/hexanes); white solid; 53 mg; yield 94%; mp 212–214 °C; 1H NMR (CDCl3, 400 MHz): δ 11.82 (s, 1H), 8.86 (s, 1H), 7.59–7.47 (m, 9H), 7.31 (d, J = 8.4 Hz, 1H), 7.14–7.10 (m, 2H), 6.84 (t, J = 7.6 Hz, 1H); 13C{1H} NMR (100 MHz, CDCl3): δ 200.7, 163.1, 160.0, 159.4, 152.8, 146.6, 139.3, 138.6, 138.0, 133.8, 131.6, 130.0, 129.7, 127.9, 126.2, 125.4, 122.2, 120.1, 119.9, 118.86, 118.85, 114.5, 103.6; IR νmax (neat): 3084, 3051, 2337, 1734, 1645, 1621, 1605, 1532, 1193, 1100, 990, 757 cm−1; HRMS (EI) m/z: [M]+ calcd for C25H15NO5 409.0950; found 409.0955.
4.7.2 3-(4-(Dimethylamino)phenyl)-1-(2-hydroxybenzoyl)-2H-chromeno[3,4-c]pyridine-2,5(3H)-dione (16b). Rf = 0.4 (40% EtOAc/hexanes); yellow solid; 57 mg; yield 91%; mp 250–252 °C; 1H NMR (CDCl3, 400 MHz): δ 11.85 (s, 1H), 8.86 (s, 1H), 7.56 (t, J = 8.8 Hz, 2H), 7.49 (t, J = 8.8 Hz, 2H), 7.30 (d, J = 8.8 Hz, 3H), 7.12–7.08 (m, 2H), 6.83 (t, J = 7.6 Hz, 1H), 6.74 (d, J = 8.8 Hz, 2H), 3.01 (s, 6H); 13C{1H} NMR (100 MHz, CDCl3): δ 201.1, 163.1, 160.4, 159.6, 152.8, 151.0, 146.9, 138.9, 137.8, 133.6, 131.8, 127.9, 127.2, 126.7, 125.3, 121.7, 120.02, 120.01, 118.80, 118.77, 114.7, 112.1, 103.1, 40.5; IR νmax (neat): 2919, 1739, 1645, 1615, 1521, 1443, 1338, 1233, 1195, 1098, 990, 741 cm−1; HRMS (EI) m/z: [M]+ calcd for C27H20N2O5 452.1372; found 452.1365.
4.7.3 1-(2-Hydroxybenzoyl)-3-(4-nitrophenyl)-2H-chromeno[3,4-c]pyridine-2,5(3H)-dione (16c). Rf = 0.7 (40% EtOAc/hexanes); yellow solid; 61 mg; yield 97%; mp 239–241 °C; 1H NMR (CDCl3, 400 MHz): δ 11.75 (s, 1H), 8.83 (s, 1H), 8.41 (d, J = 8.8 Hz, 2H), 7.74 (d, J = 8.8 Hz, 2H), 7.58–7.53 (m, 4H), 7.32 (d, J = 8.0 Hz, 1H), 7.15–7.11 (m, 2H), 6.85 (t, J = 7.6 Hz, 1H); 13C{1H} NMR (100 MHz, CDCl3): δ 200.1, 163.2, 159.6, 159.0, 152.9, 148.2, 145.3, 143.4, 139.7, 138.2, 134.3, 131.4, 128.1, 127.6, 125.7, 125.1, 122.6, 120.2, 119.7, 119.1, 119.0, 114.2, 104.5; IR νmax (neat): 2956, 2928, 2856, 1739, 1649, 1621, 1521, 1344, 1231, 1194, 1102, 744 cm−1; HRMS (EI) m/z: [M]+ calcd for C25H14N2O7 454.0801; found 454.0808.
4.7.4 3-Benzyl-1-(2-hydroxybenzoyl)-2H-chromeno[3,4-c]pyridine-2,5(3H)-dione (16d). Rf = 0.6 (40% EtOAc/hexanes); pale yellow solid; 27 mg; yield 45%; mp 260–262 °C; 1H NMR (CDCl3, 400 MHz): δ 11.87 (s, 1H), 8.77 (s, 1H), 7.56–7.41 (m, 9H), 7.28–7.27 (m, 1H), 7.12 (d, J = 8.8 Hz, 1H), 7.08 (t, J = 7.6 Hz, 1H), 6.82 (t, J = 7.6 Hz, 1H), 5.34, 5.18 (ABq, J = 14.0 Hz, 1H each); 13C{1H} NMR (100 MHz, CDCl3): δ 201.0, 163.2, 160.4, 159.3, 152.8, 146.0, 139.1, 138.0, 134.2, 133.7, 131.5, 129.5, 129.3, 129.0, 127.8, 125.4, 121.5, 120.0, 119.9, 119.0, 118.8, 114.6, 103.4, 53.6; IR νmax (neat): 3045, 2940, 1969, 1728, 1620, 1605, 1527, 1457, 1196, 1149, 1109, 750 cm−1; HRMS (EI) m/z: [M]+ calcd for C26H17NO5 423.1107; found 423.1115.
4.7.5 1-(2-Hydroxybenzoyl)-3-(2-hydroxybenzyl)-2H-chromeno[3,4-c]pyridine-2,5(3H)-dione (16e). Rf = 0.4 (40% EtOAc/hexanes); white solid; 34 mg; yield 55%; mp 252–254 °C; 1H NMR (CDCl3, 400 MHz): δ 11.79 (s, 1H), 9.02 (s, 1H), 8.34 (s, 1H), 7.56–7.48 (m, 3H), 7.39 (t, J = 7.2 Hz, 2H), 7.30–7.28 (m, 2H), 7.13 (d, J = 8.4 Hz, 1H), 7.09 (t, J = 7.6 Hz, 1H), 6.95–6.92 (m, 2H), 6.80 (t, J = 7.6 Hz, 1H), 5.29, 5.21 (ABq, J = 14.0 Hz, 1H each); 13C{1H} NMR (100 MHz, CDCl3): δ 199.0, 161.0, 159.9, 158.9, 155.8, 152.3, 147.1, 137.3, 137.2, 133.3, 131.7, 130.9, 129.8, 126.8, 124.8, 122.1, 121.0, 120.9, 119.8, 119.1, 118.4, 118.0, 115.3, 114.7, 102.0, 49.0; IR νmax (neat): 3249, 1731, 1704, 1622, 1529, 1457, 1226, 1147, 749 cm−1; HRMS (EI) m/z: [M]+ calcd for C26H17NO6 439.1056; found 439.1053.
4.8. General procedure for preparation of compounds 17a and 17b
To a 10 mL round bottom flask equipped with a magnetic stirrer and a reflux condenser was charged with 11 (0.14 mmol, 1.0 equiv.), 4-hydroxycoumarins (0.14 mmol, 1.0 equiv.) and camphorsulfonic acid (0.14 mmol, 1.0 equiv.) in acetonitrile (3.0 mL) at room temperature. The resulting reaction mixture was heated at 80 °C in an oil bath for 8 h. Upon completion of the reaction (monitored by TLC), the reaction mixture was cooled to room temperature, and the solvent was concentrated under reduced pressure. The residue was purified by column chromatography using ethyl acetate and hexane as the eluent to give the desired products.
4.8.1 3-(7-Hydroxy-6-oxo-6H,7H-chromeno[4,3-b]chromen-7-yl)-2H,5H-pyrano[3,2-c]chromene-2,5-dione (17a). Rf = 0.5 (40% EtOAc/hexanes); off-white solid; 32 mg; yield 48%; mp 217–219 °C; 1H NMR (CDCl3, 400 MHz): δ 8.76 (s, 1H), 8.21 (d, J = 7.6 Hz, 1H), 7.88 (d, J = 7.6 Hz, 1H), 7.66 (t, J = 7.2 Hz, 2H), 7.51–7.32 (m, 7H), 7.24 (t, J = 6.8 Hz, 1H), 5.40 (s, 1H); 13C{1H} NMR (100 MHz, CDCl3): δ 162.7, 161.3, 159.2, 157.4, 157.3, 153.6, 153.0, 148.8, 135.4, 134.6, 133.3, 132.0, 130.2, 127.7, 125.9, 125.3, 125.0, 124.0, 123.5, 122.2, 117.6, 117.1, 116.8, 114.1, 113.0, 103.8, 100.8, 67.0; IR νmax (neat): 2926, 2854, 1729, 1636, 1563, 1392, 1050, 754 cm−1; HRMS (EI) m/z: [M]+ calcd for C28H14O8 478.0689; found 478.0686.
4.8.2 8-(Dimethylamino)-3-(7-hydroxy-6-oxo-6H,7H-chromeno[4,3-b]chromen-7-yl)-2H,5H-pyrano[3,2-c]chromene-2,5-dione (17b). Rf = 0.4 (40% EtOAc/hexanes); yellow solid; 36 mg; yield 52%; mp 243–245 °C; 1H NMR (CDCl3, 400 MHz): δ 8.68 (s, 1H), 8.20 (d, J = 7.6 Hz, 1H), 7.63 (d, J = 8.8 Hz, 2H), 7.51 (d, J = 7.6 Hz, 1H), 7.46–7.33 (m, 5H), 6.62 (d, J = 8.0 Hz, 1H), 6.49 (s, 1H), 5.41 (s, 1H), 3.10 (s, 6H); 13C{1H} NMR (150 MHz, CDCl3): δ 162.6, 162.3, 160.1, 158.0, 157.0, 156.0, 154.5, 152.8, 148.6, 136.2, 132.9, 129.7, 127.8, 127.5, 125.6, 124.7, 124.4, 123.8, 122.7, 116.9, 116.4, 114.1, 109.9, 101.2, 101.1, 99.2, 97.7, 66.7, 40.2; IR νmax (neat) 3426, 2927, 2854, 2326, 1723, 1716, 1705, 1611, 1566, 1519, 1406, 1183, 1047, 757 cm−1; HRMS (EI) m/z: [M]+ calcd for C30H19NO8 521.1111; found 521.1116.
Conflicts of interest
There are no conflicts to declare.
Acknowledgements
We thank the National Science and Technology Council of Taiwan, for financially supporting this research under Contract No. NSTC 112-2113-M-029-005-MY3, NSTC 109-2113-M-029-010-MY3. Also, the support of the X-ray, MASS, and NMR measurements from The Instrument Center of National Chung Hsing University is greatly acknowledged.
Notes and references
- S. Maiti, S. K. Panja and C. Bandyopadhyay, J. Chem. Res., 2011, 35, 84–86 CrossRef CAS.
-
(a) M. Costa, F. Areias, M. Castro, J. Brea, M. I. Loza and F. Proenca, Org. Biomol. Chem., 2011, 9, 4242–4249 RSC;
(b) K. S. Mohamed and E. E. Elbialy, Heterocycles, 2020, 100, 1035–1047 CrossRef CAS.
- R. S. Thelingwani, K. Dhansay, P. Smith, K. Chibale and C. M. Masimirembwa, Xenobiotica, 2012, 42, 989–1000 CrossRef CAS PubMed.
- R. R. Zhang, J. Liu, Y. Zhang, M. Q. Hou, M. Z. Zhang, F. Zhou and W. H. Zhang, Eur. J. Med. Chem., 2016, 116, 76–83 CrossRef CAS PubMed.
- C. N. Huang, P. Y. Kuo, C. H. Lin and D. Y. Yang, Tetrahedron, 2007, 63, 10025–10033 CrossRef CAS.
- C. H. Lin and D. Y. Yang, Org. Lett., 2013, 15, 2802–2805 CrossRef CAS PubMed.
- C. K. Wu, Z. Weng and D. Y. Yang, Org. Lett., 2019, 21, 5225–5228 CrossRef CAS PubMed.
-
(a) M. Zhang, T. Zhang, S. Yu, H. Qiu, A. Yusuf, X. Xu, Y. Qian and W. Hu, Chem. Sci., 2023, 14, 11850–11857 RSC;
(b) Z. W. Qiu, X. T. Xu, H. P. Pan, Z. S. Jia, A. J. Ma, J. B. Peng, J. Y. Du, N. Feng, B. Q. Li and X. Z. Zhang, J. Org. Chem., 2021, 86, 6075–6089 CrossRef CAS PubMed;
(c) A. Mukherjee, S. Mahato, G. V. Zyryanov, A. Majee and S. Santra, New J. Chem., 2020, 44, 18980–18993 RSC;
(d) J. Zhang, G. Yin, Y. Du, Z. Yang, Y. Li and L. Chen, J. Org. Chem., 2017, 82, 13594–13601 CrossRef CAS PubMed.
- Crystallographic data (excluding structure factors) for 10, 11, 12a, 12d, 14b, 16e, and 17a have been deposited with the Cambridge Crystallographic Data Centre as supplementary publication number CCDC-2264219, -2264217, -2018939, -1985012, -1985024, -2264220, and -2264218, respectively.† These data can be obtained free of charge via www.ccdc.cam.ac.uk/data_request/cif, by emailing data_request@ccdc.cam.ac.uk, or by contacting The Cambridge Crystallographic Data Centre, 12 Union Road, Cambridge CB2 1EZ, UK; fax: +44 1223 336033..
- S. Vyasamudri and D. Y. Yang, Org. Lett., 2020, 22, 3166–3170 CrossRef CAS PubMed.
-
(a) D. Zavrsnik, S. Muratovic, D. Makuc, J. Plavec, M. Cetina, A. Nagl, E. D. Clercq, J. Balzarini and M. Mintas, Molecules, 2011, 16, 6023–6040 CrossRef CAS PubMed;
(b) Z. N. Siddiqui, Tetrahedron Lett., 2014, 55, 163–168 CrossRef CAS;
(c) Z. N. Siddiqui and K. Khan, ACS Sustainable Chem. Eng., 2014, 2, 1187–1194 CrossRef CAS;
(d) S. Balalaie, Y. Doroudian, N. Zarezadeh, H. Z. Tejeneki and F. Rominger, ChemistrySelect, 2019, 4, 8921–8924 CrossRef CAS;
(e) A. N. Nadaf and K. Shivashankar, J. Heterocycl. Chem., 2018, 55, 1375–1381 CrossRef CAS;
(f) C. Bandyopadhyay, K. R. Sur, R. Patra and A. Sen, Tetrahedron, 2000, 56, 3583–3587 CrossRef CAS.
-
(a) A. H. Alkhzem, T. J. Woodman and I. S. Blagbrough, RSC Adv., 2022, 12, 19470–19484 RSC;
(b) M. Szumilak, A. Wiktorowska-Owczarek and A. Stanczak, Molecules, 2021, 26, 2601 CrossRef CAS PubMed;
(c) Shaveta, S. Mishra and P. Singh, Eur. J. Med. Chem., 2016, 124, 500–536 CrossRef CAS PubMed.
|
This journal is © The Royal Society of Chemistry 2024 |
Click here to see how this site uses Cookies. View our privacy policy here.