DOI:
10.1039/D4RA02852J
(Paper)
RSC Adv., 2024,
14, 28915-28926
Magnetic iron-based waterworks sludge modified by chitosan and FeS for aqueous Cr(VI) adsorption and reduction†
Received
17th April 2024
, Accepted 27th July 2024
First published on 11th September 2024
Abstract
Heavy metals have been considered an evolving environmental concern due to their harmful and long-lasting impacts. We synthesized a composite of FeS/CS@MIBWS for aqueous Cr(VI) adsorption and reduction utilizing the iron-based waterworks sludge modified by chitosan and FeS. After determining the optimal conditions for the FeS/CS@MIBWS preparation, its Cr(VI) removal capability was evaluated using material characterisation and static Cr(VI) adsorption assays. Cr(VI) elimination by the composite was a pH-dependent process, with pH 2 being the optimum in the range of 2–10. The adsorption process was befitted a pseudo-second-order model, and the equilibrium results agreed well with the Langmuir model. The thermodynamics investigation showed that Cr(VI) removal by the composite has both spontaneous and endothermic nature. Considering the ionic effects, Cl−, SO42− and PO43− decreased Cr(VI) elimination in the sequence of Cl− < SO42− < PO43−. The key mechanisms for Cr(VI) elimination were physical and chemical adsorption, chelation, and Cr(VI) reduction into Cr(III). Furthermore, FeS/CS@MIBWS demonstrated steady reusability (removal effectiveness of 70% after 5 cycles). FeS/CS@MIBWS's rapid, high-performance, reusable, and easily separable adsorption properties make it a promising choice for heavy metal environmental cleaning.
1 Introduction
The heavy metal pollution in wastewater has attracted widespread attention.1 Chromium appearing in surface water, ground water and soil primarily originates from wide range of modern industries with the dominant forms of Cr(VI) and Cr(III) compounds,2,3 and Cr(VI) has been reported to be carcinogenic, teratogenic, mutagenic and non-biodegradable.4,5 Compared to Cr(III), Cr(VI) has a higher solubility and exists in the forms Cr2O72−, HCrO4−, and CrO42−.6,7 Chronic exposure to Cr(VI) can induce major human disorders and pose a considerable hazard to aquatic creatures even at a very low concentration.8,9 As a result, considering the mutagenic, poisonous, and long-lasting impacts of aqueous Cr(VI), fixing the problem is critical.
Electrochemical approaches, photocatalytic treatment, membrane filtration, ion exchange, adsorption, and other techniques have been developed to control the harm caused by Cr(VI).10–13 In a variety of technologies, adsorption has attracted the most attention due to its benefits such as ease of use, low cost, excellent performance, wide pH range, and so on.14
Iron-based waterworks sludge (IBWS) is a byproduct generated from drinking water treatment plants using iron-based coagulants for water processing. Fe in IBWS mainly exists in amorphous phases, endowing it with porosity and high adsorption capability for a variety of contaminants such as phosphorus and heavy metals, etc..15–18 Additionally, IBWS can be magnetized by calcination or hydrothermal treatment,19,20 resulting in a rapid separation from solution upon adsorption. However, due to the low affinity between Fe and Cr(VI), the adsorption capacity of the IBWS for Cr(VI) removal is poor and may be improved by modification.
Chitosan (CS), a natural polysaccharide, has the world's second biggest yearly production,21 and has been proved to be an effective adsorbent for numerous pollutants22–24 due to its merits including degradability, high reactivity and charge density, biocompatibility, renewability, and harmless.25–27 Given that the form of Cr(VI) in water was oxyanions, existing hydroxyl and amine groups in CS can easily react with Cr(VI) and achieve better adsorption ability.28
Furthermore, because Cr(III) is substantially less toxic than Cr(VI), lowering is a viable and safe technique of eliminating Cr(VI). FeS may be a suitable material for immobilizing Cr(VI), as both Fe2+ and S2− can act as reducing agents. Yang et al., for example, developed nano-FeS and CMC-FeS for Cr(VI) removal, and the two materials efficiently converted Cr2O72− into Cr0.75(OH)3.29 As a result, injecting FeS is thought to achieve simultaneous magnetic and efficient purification of the composite in this investigation.
In this work, we synthesized a novel composite with the aim of immobilizing aqueous Cr(VI). We investigated the physicochemical parameters by characterisation, assessed the composite's reduction ability towards Cr(VI), and proved the process of adsorption. The findings give detailed insight into the manufacture of adsorption materials as well as a reference for lowering Cr(VI) in water.
2 Materials and methods
2.1 Adsorbent preparation
MIBWS utilized in this research was prepared according to the procedures disclosed in our earlier study.3 The adsorbents used in this study were prepared via a modification of the way described in the literature.30 Briefly, 1 g CS and various amounts of MIBWS (0.5, 0.75, 1 and 1.25 g) were added into 100 mL C2H4O2 solution (2.5%, v/v) then stirred and sonicated for 2 h for complete dissolution of CS and uniform dispersion of MIBWS. Then, 50 mL FeSO4·7H2O (20 g L−1) was added into the mixture dropwise. Next, the mixture was agitated for 12 h before 50 mL Na2S was added dropwise under a N2 environment at 60 °C and mixed for 2 h. Following that, 5 mL glutaraldehyde was added to the mixture once it had cooled. After fully aging for 24 hours, the combination was reacted at 60 °C for another 3 h, and its pH was adjusted in succession with NaOH solution. Finally, the mixture was rinsed and dried at 70 °C in a vacuum for 12 h before sieving through 0.15 mm screen mesh to get four FeS/CS@MIBWS adsorbents prepared with varying MIBWS dosage.
The best MIBWS dosage determined in the Cr(VI) adsorption studies with the four adsorbents stated above was used to prepare several types of FeS/CS@MIBWSs with varying S2− and Fe2+ dosages. The S2−
:
Fe2+ mole ratio was kept at 2
:
1 for all the composites, and the other preparing techniques were the same as mentioned earlier.
2.2 Adsorbent characterization
The functional groups of FeS/CS@MIBWS before and after Cr(VI) adsorption were analyzed using Fourier transform infrared spectroscopy (FTIR, Nicolet IS50, Thermo fisher, USA) in the spectra range of 4000–400 cm−1. The Brunauer–Emmett–Teller specific surface area (SBET) and pore diameter distribution was measured using an automatic specific surface area analyzer (BELSORP-max, MicrotracBEL, Japan). The surface element and chemical state of MIBWS, FeS/CS@MIBWS before and after Cr(VI) adsorption were determined by X-ray photoelectron spectroscopy (XPS, Escalab 250Xi+, Thermo Fisher Scientific USA). The crystalline phases of MIBWS and FeS/CS@MIBWS were studied using an X-ray diffractometer (XRD, SmartLab SE, Rigaku, Japan) in the 2θ range of 5–90° with a step size of 0.02°. Magnetic properties of FeS/CS@MIBWS were studied using a vibrating sample magnetometer (VSM, Lake shore 7404, USA). The zero point charge (pHPZC) was determined using a zeta potential meter (Particle Metrix GmbH, Germany). The surface morphology of the composite was recorded using a scanning electron microscope (SEM, Zeiss Genimi500, Germany), and the element content of it was analyzed using a X-ray fluorescence spectrometer (XRF, primus, Japan).
2.3 Cr(VI)-containing wastewater preparation
The Cr(VI)-containing wastewater used in the study was prepared by dissolving K2Cr2O7 into the deionized water. 0.1 M HCl and NaOH solutions were used to adjust the pH of the artificial solution. All the chemical reagents utilized in this study were analytically pure.
2.4 Batch adsorption experiment
0.02 g adsorbent and 50 mL artificial wastewater was placed in a flask in a shaker at 120 rpm, which reacted at fixed time span and temperature. The remnant Cr(VI) in the supernate was detected by a UV-vis spectrophotometer (UV-5100, Yuanxi, China) using the method described in our previous study.3 All the tests were conducted in triplicate, and the average value was used for analysis.
3 Results and discussion
3.1 Adsorbent preparation
Fig. 1a shows the Cr(VI) removal by four FeS/CS@MIBWS prepared with varying MIBWS doses, denoted as FeS/CS@MIBWS1, FeS/CS@MIBWS2, FeS/CS@MIBWS3, and FeS/CS@MIBWS4. The four composites showed Cr(VI) removal capacities of 77.13, 61.92, 50.47 and 41.47 mg g−1, respectively, as the MIBWS increased from 0.5 to 1.25 g, while the corresponding saturation magnetic inductions were 0.46, 7.22, 7.71 and 14.80 emu g−1 (Fig. 1b). FeS/CS@MIBWS1 has the best Cr(VI) removal but the worst magnetism, resulting in poor separability following Cr(VI) removal in a magnetic field. Unlike FeS/CS@MIBWS1, spent FeS/CS@MIBWS2 separated from the aqueous solution in 10 s using a magnetic force, while removing less Cr(VI). As a result, 0.75 g MIBWS was established to be the optimal dosage for FeS/CS@MIBWS preparation, taking into account both Cr(VI) removal and magnetic separation of the spent composite.
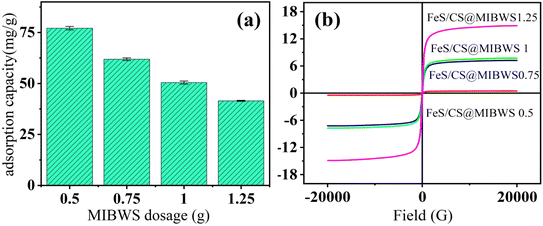 |
| Fig. 1 Adsorption capacity of FeS/CS@MIBWS (a) and VSM (b) variation as function of MIBWS dosage (reaction time = 4 h, C0 = 200 mg L−1, T = 25 °C). | |
As shown in Fig. 2a, Cr(VI) adsorption increased from 47.82 to 66.12 mg g−1 when the FeSO4 dose for the composite preparation increased from 0 to 2 g, demonstrating that FeSO4 may improve Cr(VI) adsorption. Because there was no discernible improvement in Cr(VI) adsorption with increasing FeSO4 dose from 1.5 to 2 g, hence, 1.5 g FeSO4 was determined to be the optimal dosage for FeS/CS@MIBWS preparation. As a result, the best mass ratio for FeS/CS@MIBWS preparation was MIBWS
:
CS
:
FeSO4·7H2O = 0.75
:
1
:
1.5 g. Compared to IBWS, which had a lower Cr(VI) adsorption of 1.23 mg g−1, FeS/CS@MIBWS had a substantially greater Cr(VI) adsorption of 65.72 mg g−1, showing that the approach adopted in this work was effective for IBWS modification (Fig. 2a).
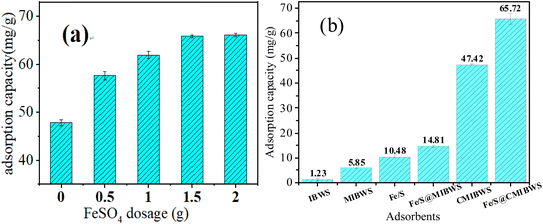 |
| Fig. 2 Adsorption capacity of FeS/CS@MIBWS as a function of FeSO4·7H2O dosage in their ingredients (a); comparison of Cr(VI) adsorption by 6 adsorbents (b) (m = 0.02 g, T = 298 K, reaction time = 4 h, C0 = 50 mg L−1). | |
3.2 Characterization
The phase purity and crystallinity of the MIBWS and FeS/CS@MIBWS samples were analyzed by XRD (Fig. 3). The XRD patterns of MIBWS and FeS/CS@MIBWS were similar to each other, the characteristic XRD peaks at 2θ values of 30.2°, 35.6°, 43.3°, 57.3° and 62.8° were attributed to the (220), (311), (400), (511) and (440) crystal plane of Fe3O4 (JCPDS No. 19-0629).31 Meanwhile, the diffraction pattern was in good agreement with the hexagonal phase of FeS (JCPDS Card No. 75-0602), and the peaks at 30.1°, 33.9°, 43.6°, 53.4° can be indexed to the (100), (101), (102), (110) planes of FeS, respectively.32–36 The broad peak located at 20.40° were indexed to the semi-crystalline polymer of chitosan.37,38
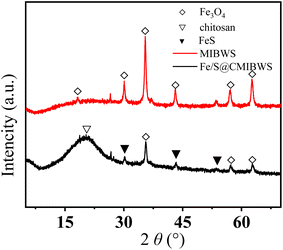 |
| Fig. 3 XRD patterns of MIBWS and FeS/CS@MIBWS. | |
In our previous study,3 pristine IBWS exhibited type IV N2 adsorption–desorption isotherms with the usual type H3 hysteresis loop, whereas FeS/CS@MIBWS had the same kind of N2 adsorption–desorption isotherms and hysteresis loop after modification (Fig. 4a).
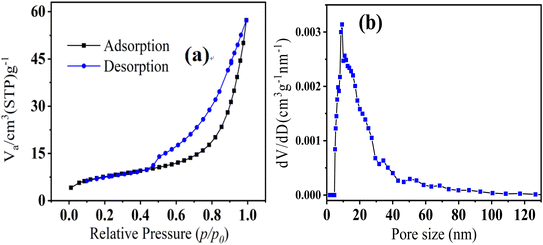 |
| Fig. 4 Nitrogen physisorption–desorption isotherm (a) and pore size distribution (b) of FeS/CS@MIBWS. | |
As demonstrated in our previous study,3 IBWS had SBET of 115.34 m2 g−1, total pore volume (TPV) of 0.28 cm3 g−1, and average pore diameter (APD) of 9.75 nm, whereas, the corresponding parameters of FeS/CS@MIBWS were 27.05 m2 g−1, 0.32 cm3 g−1, and 14.54 nm, respectively (Fig. 4b and Table 1), indicating that modification by CS and FeS significantly reduced the surface area while increased the TPV and APD. IBWS had a greater SBET than FeS/CS@MIBWS, but a lower Cr(VI) adsorption, demonstrating that SBET was not the essential component in the adsorption process.
Table 1 SBET and pore parameters of FeS/CS@MIBWS
Sample |
SBET (m2 g−1) |
TPV (cm3 g−1) |
APD (nm) |
Fe/S@CMIBWS |
27.05 |
0.32 |
14.54 |
FeS/CS@MIBWS had a rough surface due to a high concentration of small particles (a), however there were a considerable number of pores of varied sizes on its surface (b), as seen in Fig. 5. As demonstrated in Table 2, Fe, C, and S were the top three elements in FeS/CS@MIBWS, mostly derived from the IBWS before and after calcination, with FeS employed for modification.
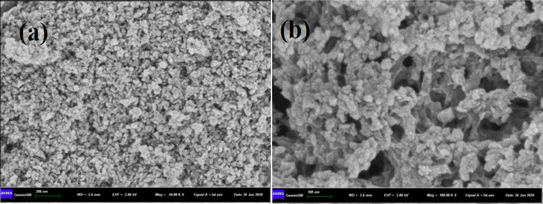 |
| Fig. 5 SEM morphologies of FeS/CS@MIBWS magnified by 50 000 (a) and 100 000 times (b). | |
Table 2 The main element content of FeS/CS@MIBWS
Element |
Content (%) |
Element |
Content (%) |
Fe |
24.30 |
Mo |
0.31 |
C |
11.00 |
Na |
0.24 |
S |
7.02 |
K |
0.16 |
Si |
1.73 |
Mg |
0.15 |
Al |
0.81 |
Ti |
0.10 |
Ca |
0.36 |
Mn |
0.05 |
3.3 Effect of working solution pH
Cr(VI) adsorption by FeS/CS@MIBWS reduced from 163.0 to 15.2 mg g−1 with the increasing pH from 2 to 10 (Fig. 6a), suggesting acidic environment was favorable for Cr(VI) elimination, which matched the previous studies.39,40
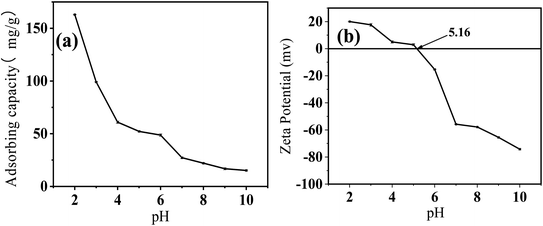 |
| Fig. 6 Influence of the initial solution pH on Cr(VI) adsorption by FeS/CS@MIBWS (a), zeta potential analysis of FeS/CS@MIBWS (b). | |
Fig. 6b shows that the pHpzc of FeS/CS@MIBWS was around pH 5.16. Protonation at pH < 5.16 positively charged the surface of FeS/CS@MIBWS, facilitating the electrostatic adsorption of the negatively charged oxyanions of Cr(VI) such as HCrO4−, Cr2O72−, and CrO42−, whereas, negatively charged surface at pH > 5.16 repulsed the oxyanions in the solution, resulting in decreased Cr(VI) removal. Furthermore, the conflict between the oxyanions and hydroxyl groups for active points on the surface of FeS/CS@MIBWS reweakened Cr(VI) adsorption.41,42
3.4 Kinetic study
Fig. 7a shows that both of the two Cr(VI) adsorption processes increased as contact duration and Cr(VI) concentration raised. Furthermore, the two processes had initial rapid phases that were virtually at equilibrium at 360 minutes, and no additional substantial Cr(VI) removal was seen during the ensuing slow period. Pseudo-firstorder, pseudo-secondorder and Elovich models (eqn (1)–(3))43,44 was utilized to further analyze the experimental data. Fig. 7b–d shows the linear fitting using the three models. |
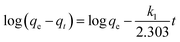 | (1) |
|
 | (2) |
|
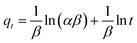 | (3) |
where qe (mg g−1) and qt (mg g−1) were the Cr(VI) uptake at equilibrium and time t, respectively. k1 (min−1) and k2 (g mg−1 min−1) are the pseudo-first order and pseudo-second order rate constants, respectively. α (mg g−1 min−1) and β (g mg−1) are the initial adsorption rate and the desorption constant, respectively.
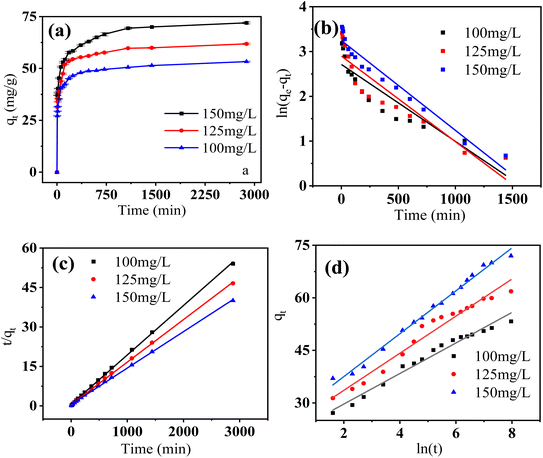 |
| Fig. 7 Effect of contact time on Cr(VI) adsorption (a), linear fitting pseudofirst order kinetics (b), pseudosecond order kinetics (c) and Elovich model (d) (m = 0.02 g, pH = 4, T = 298 K). | |
As shown in Table 3, the correlation coefficient (R2) of the pseudo-second order model was higher than those of the other two models, and was closest to 1, suggesting that it was the optimal model to depict Cr(VI) adsorption on FeS/CS@MIBWS, and chemisorption was the rate-limiting step for the adsorption process in which electrons sharing or exchange between Cr(VI) and FeS/CS@MIBWS was involved.
Table 3 Kinetic parameters of Cr(VI) adsorption by FeS/CS@MIBWS
C0 mg L−1 |
Pseudo-first order |
Pseudo-second order |
Elovich |
qe |
k1 |
R2 |
qe |
k2 |
R2 |
α |
β |
R2 |
100 |
15.06 |
0.00172 |
0.825 |
53.16 |
0.000634 |
0.999 |
531.53 |
0.2293 |
0.9765 |
125 |
18.16 |
0.00191 |
0.835 |
61.84 |
0.000566 |
0.999 |
406.05 |
0.1887 |
0.9647 |
150 |
25.67 |
0.00127 |
0.948 |
72.20 |
0.000366 |
0.998 |
379.58 |
0.1634 |
0.9915 |
3.5 Isotherms and thermodynamics study
Langmuir and Freundlich models (eqn (4) and (5))45 were used to further understand the interaction between Cr(VI) and FeS/CS@MIBWS. |
 | (4) |
|
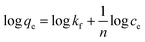 | (5) |
where ce (mg L−1) was the Cr(VI) concentration at equilibrium, qm (mg g−1) was the theoretical saturated Cr(VI) adsorption calculated from the Langmuir equation, b (L mg−1) is the Langmuir constant, kf and n are the Freundlich constant and exponent, respectively.
As shown in Fig. 8a, qe was greater at higher temperatures than at lower temperatures for all the three reaction temperatures, indicating that higher temperatures resulted in improved Cr(VI) adsorption. Fig. 8b and c present the linearized fitting results from the two adopted models.
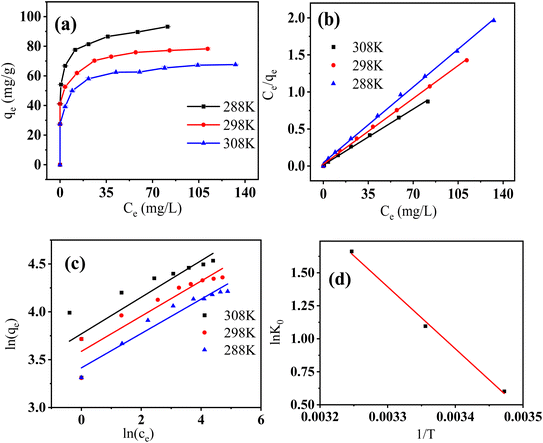 |
| Fig. 8 The relationship between ce and qe under three reaction temperatures (a), linear fitting using Langmuir model (b), Freundlich model (c), and the relationship between 1/T and ln K0 for Cr(VI) adsorption by FeS/CS@MIBWS (d) (reaction time = 24 h, pH = 4). | |
Table 4 shows that all the three correlation coefficients (R2) of Langmuir model exceeded 0.99, whereas the highest correlation coefficient of Freundlich model was 0.939, suggesting Langmuir model was better to describe the Cr(VI) adsorption by FeS/CS@MIBWS, which is a monolayer adsorption process.
Table 4 Isotherm parameters of Cr(VI) adsorption by FeS/CS@MIBWS at different temperatures
T (K) |
Langmuir parameters |
Freundlich parameters |
Thermodynamic constants |
qm |
b |
R2 |
kf |
n |
R2 |
ΔG0 |
ΔH0 |
ΔS0 |
288 |
68.49 |
3.071 |
0.999 |
30.39 |
5.57 |
0.939 |
−1.40 |
39.41 |
0.14 |
298 |
78.74 |
1.806 |
0.999 |
36.11 |
5.44 |
0.853 |
−2.80 |
308 |
92.68 |
1.251 |
0.998 |
43.36 |
5.22 |
0.707 |
−4.21 |
The influence of reaction temperature on Cr(VI) adsorption by FeS/CS@MIBWS and the feasibility of the process was studied using three thermodynamics including standard free energy change (ΔG0), standard entropy change (ΔS0), and standard enthalpy change (ΔH0), which were obtained from eqn (6)–(8).3
|
ΔG0 = −RT ln KL
| (6) |
|
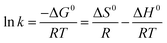 | (8) |
where
KL was a constant from Langmuir isotherm (L mol
−1); Δ
S0 and Δ
H0 were calculated from the relationship of ln
KL and 1/
T shown in
Fig. 8d,
R is the ideal gas constant (8.314 J (mol
−1 K
−1)),
T was the adsorption temperature (K). As shown in
Table 4, Δ
G0 values for all the three reactions were negative and declined with the increasing temperatures, indicating the spontaneous nature of the adsorption process. The endothermic nature of the adsorption process was proved by the positive values of Δ
H0 and Δ
S0.
Table 5 gives Cr(VI) adsorption capacities calculated from the Langmuir model for the modified chitosan adsorbents used in the existing documents and this study. The composite used in this study exhibited the fourth largest Cr(VI) uptake among the 9 composite, suggesting the adsorbent was an excellent material for Cr(VI) removal from water.
Table 5 Comparison of monolayer Cr(VI) adsorption capacities of FeS/CS@MIBWS
Adsorbents |
Moments (emu g−1) |
Qm (mg g−1) |
Conditions (K & pH) |
Ref. |
Chitosan/polymethylmethacrylate |
— |
67.06 |
298 & 3 |
27 |
Magnetic zeolite/chitosan composites |
18.67 |
28.47 |
303 & 3 |
46 |
Ethylamine modified chitosan carbonized rice husk composite beads |
— |
52.7 |
298 & 2 |
47 |
FCN-500 |
54.3 |
52.63 |
308 & 3 |
48 |
Magnetic activated carbon for |
11.07 |
45.3 |
318 & 2 |
49 |
Magnetic zeolite/chitosan composite |
16.83 |
21.25 |
288 & — |
50 |
23.76 |
303 & — |
24.61 |
318 & — |
Ethylenediamine-modified cross-linked magnetic chitosan resin |
— |
51.81 |
293 & — |
51 |
48.78 |
303 & — |
45.87 |
313 & — |
MnO2/CS nanocomposite |
— |
61.56 |
298 & 2 |
52 |
FeS/CS@MIBWS |
7.22 |
68.49 |
288 & 4 |
Current study |
78.74 |
298 & 4 |
92.68 |
308 & 4 |
3.6 Effect of co-existing anions
The effect of common anions in water including Cl−, SO42− and PO43− on Cr(VI) adsorption by FeS/CS@MIBWS was studied. As shown in Fig. 9, all the three anions impaired Cr(VI) uptake in the sequence of Cl− < SO42− < PO43− with their increasing concentration, which was consistent with our prior findings and other recent research.53 Due to the superimposition effect, the coexistence of the three anions resulted in lower Cr(VI) absorption than the solo effect of each anion.
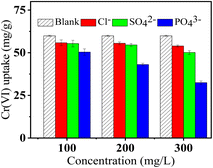 |
| Fig. 9 The effect of coexisting ions on adsorption of Cr(VI) by FeS/CS@MIBWS (T = 298 K, pH = 4, C0 = 50 mg L−1). | |
3.7 Regeneration and reusability
Acetic acid and hydrochloric acid were used for FeS/CS@MIBWS regeneration (Fig. 10a). In comparison to 1 M C2(H2O)2, all the three concentrations of HCl exhibited greater regeneration effect, with 1 M HCl being the best for FeS/CS@MIBWS regeneration. As a results, 1 M HCl was used for FeS/CS@MIBWS regeneration.
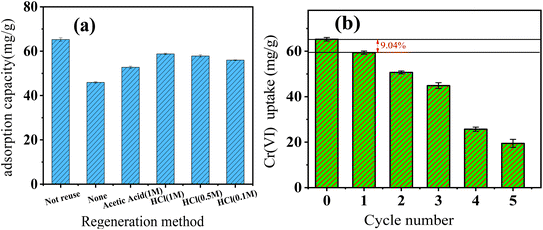 |
| Fig. 10 Determination of regeneration reagent (a) and Cr(VI) uptake variation with reuse cycles (b). | |
As shown in Fig. 10b, there was 9.04% drop in Cr(VI) uptake after the first regeneration, and Cr(VI) uptake decreased by 70.2% after regeneration for 5 times, indicating the FeS/CS@MIBWS can be reused for times.
3.8 Mechanism exploration
As shown in Fig. 11, the bands at around 3369.0 cm−1, 2924.0 cm−1, 1712.5 cm−1, 1648.8 cm−1,1376.0 cm−1 for FeS/CS@MIBWS belonged to NH2 stretching,54 symmetric CH vibration,55 C
O stretching, symmetrical C
O stretching,56 C–H bending,57 respectively. The above-mentioned bands shifted to 3389.2 cm−1, 2925.2 cm−1, 1716.2 cm−1, 1649.9 cm−1and 1377.6 cm−1, respectively, indicating their involvement into Cr(VI) elimination. The bands at 1031.7 cm−1, 612.3 cm−1 and 477.3 cm−1 belonged to the primary amine C–N in chitosan,58 α-Fe2O3 (ref. 59) and Fe–O asymmetric stretching,60 respectively.
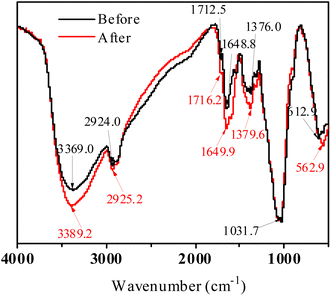 |
| Fig. 11 FTIR spectra of FeS/CS@MIBWS before and after Cr(VI) adsorption. | |
As shown in Fig. 12, the energy bands of Fe 2p, N 1s and S 2p appeared at the wide-scan spectrum of FeS/CS@MIBWS, indicating the chitosan and FeS were successfully loaded. The energy band of Cr 2p emerged after Cr(VI) adsorption, demonstrating the Cr(VI) was adsorbed on the surface of FeS/CS@MIBWS.
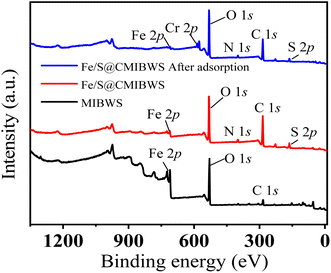 |
| Fig. 12 The full XPS spectra of MIBWS, FeS/CS@MIBWS before and after Cr(VI) adsorption. | |
As shown in Fig. 13a, C 1s XPS spectrum of FeS/CS@MIBWS before Cr(VI) adsorption contained three functional groups with peaks at 283.7, 285.08 and 286.38 eV, corresponding to C
C, C–N and C–O, respectively.61 Despite the fact that the bands of the three peaks did not altered after Cr(VI) adsorption, the peak area of C–N declined from 40.89% to 31.28%, and the peak area of C
C rose from 31.25% to 40.00% (Fig. 13b), respectively, indicating the participation of the carbon functional group into the Cr(VI) adsorption.
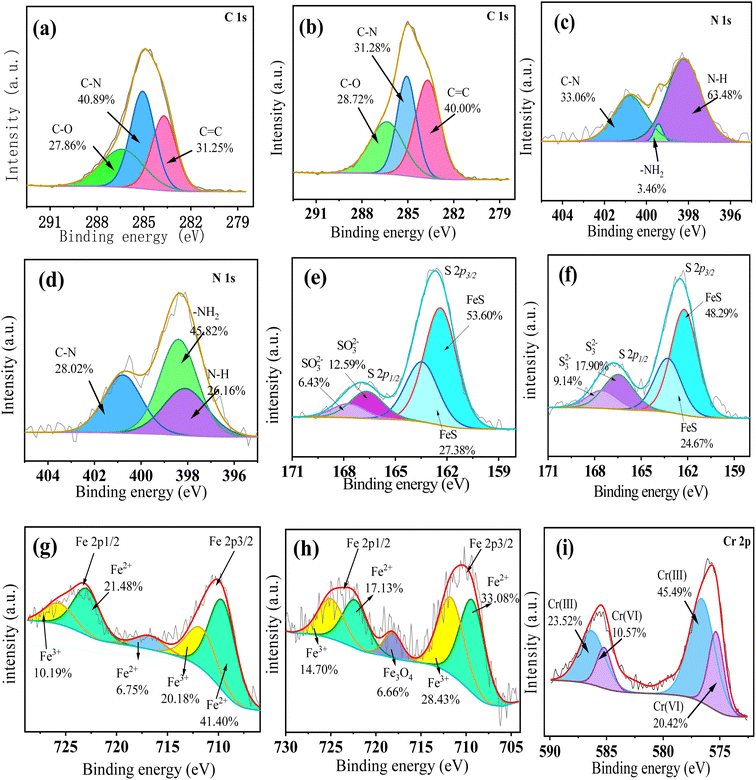 |
| Fig. 13 The detail survey of C before (a) and after (b) Cr(VI) adsorption, N before (c) and after (d) Cr(VI) adsorption, S before (e) and after (f) Cr(VI) adsorption, Fe before (g) and after (h) Cr(VI) adsorption, Cr 2p after Cr(VI) adsorption (i). | |
Fig. 13c shows that N 1s spectra contained three peaks at 398.1, 399.4 and 400.8 eV, respectively, which were attributed to –NH, –NH2 and C–N, respectively,62 indicating the presence of chitosan. After Cr(VI) adsorption, the peak area of –NH decreased from 63.48% to 26.16%, while the peak area of –NH2 rose from 3.46% to 45.82%, respectively (Fig. 13d), indicating the nitrogen-containing functional group were protonated and participated in the Cr(VI) adsorption, which can be described by eqn (9)–(11).63
|
−NH3+ + HCrO4− → NH3+⋯HCrO4−
| (11) |
S 2p1/2 and S 2p3/2 had peaks at approximately 166.8 eV and 162.5 eV, respectively (Fig. 13e). The binding energy of S 2p1/2 consisted two peaks at 162.3 eV and 163.3 eV, corresponding to FeS,64 whereas, the binding energy of S 2p3/2 of SO32− was found at 166.5 eV and 167.6 eV.65 After Cr(VI) adsorption, the peak area of FeS fell from 80.98% to 72.96%, whereas the peak area of SO32− increased from 19.20% to 27.04% (Fig. 13f), indicating the FeS formed during the participated into the Cr(VI) removal as electron donor, and the reaction can be expressed by equation.66
Fig. 13g shows the Fe 2p high resolution XPS spectra of FeS/CS@MIBWS before Cr(VI) adsorption. After Cr(VI) adsorption, the peaks attributed to both Fe3+ and Fe2+ shifted, and a new satellite peak emerged. Meanwhile, peak regions for Fe3+ increase from 30.37 to 43.13%, whereas, peak areas of Fe2+ declined from 69.63 to 56.87% (Fig. 13h). As a result, in conjunction with the study of Cr(VI) conversion, it is possible to assume that the Fe2+ in Fe3O4 in FeS/CS@MIBWS acted as reductant to reduce Cr(VI) into Cr(III), as stated by the following eqn (12)–(16).45
|
FeS + H+ → Fe2+ + HS−
| (12) |
|
3Fe2+ + HCrO4− + 7H+ → 3Fe3+ + 2Cr3+ + 4H2O
| (14) |
|
3HS− + 8HCrO4− + 29H+ → 3SO42− + 8Cr3+ + 20H2O
| (15) |
|
3S2− + 2HCrO4− + 14H+ → 3S + 2Cr3+ + 8H2O
| (16) |
As shown in Fig. 13i respectively, suggesting that Cr was adsorbed on the surface of FeS/CS@MIBWS.67 The peaks at 575.3 eV and 585.3 eV corresponded to Cr 2p3/2 and Cr 2p1/2 of Cr(VI), and accounted for only 30.99% of the total peak area of Cr(VI). Peaks of 576.6 eV and 585.5 eV corresponded to Cr 2p3/2 and Cr 2p1/2 of Cr(III),68 and its peak areas increased from 0 to 69.01% after Cr(VI) adsorption.
It can be concluded from the above analysis, physical and chemical adsorption, chelation and reduction of Cr(VI) into Cr(III) was participated the reaction.
4 Conclusion
In this study, MIBWS-based composite, namely FeS/CS@MIBWS was prepared by magnetized iron-based waterworks sludge, chitosan and FeS. The composite was adopted for a series of static Cr(VI) removal studies to investigate its Cr(VI) adsorption ability. Cr(VI) adsorption by the composite rose as the pH rose from 2 to 10. The pseudo-second order equation was more suitable for the description of Cr(VI) adsorption, and the Langmuir model fitted the experimental data better than the Freundlich model, the maximum uptake of aqueous Cr(VI) was 92.68 mg g−1 in 308 K. The main mechanism include physical and chemical adsorption, mainly the chelation and reduction of Cr(VI) into Cr(III). The composite could be used to purify Cr(VI)-containing effluent.
Data availability
The data supporting this article have been included as part of the ESI.†
Author contributions
Jingxi Tie: conceptualization, methodology, writing – original draft, writing – review & editing; Weipeng Li: data curation, validation, writing – original draft; Xiaohan Duan: validation, visualization, writing – original draft; HuawenWang: investigation, validation; Shuli Liu: investigation, writing – original draft; Weigao Zhao: project administration, supervision, writing – review & editing. The manuscript was written through contributions of all authors. All authors have given approval to the final version of the manuscript.
Conflicts of interest
The authors declare no competing interests.
Acknowledgements
The authors gracefully thank for the final support by Henan Province Science and Technology Research and Development project (242102231015), Key Promotion Project of Henan Province (No. 232102321060) and Key R&D projects of Gansu Province in 2024 (24YFFK001).
References
- A. Behera, S. P. Sahu, S. Kumar-Singh, B. Mahapatra and R. K. Patel, Polypyrrole modified zirconium (IV) phosphate nanocomposite: An effective adsorbent for Cr (VI) removal by adsorption-reduction mechanism, Mater. Chem. Phys., 2022, 290, 126540 CrossRef CAS.
- S. Y. Miao, J. R. Guo, Z. M. Deng, J. X. Yu and Y. R. Dai, Adsorption and reduction of Cr (VI) in water by iron-based metal-organic frameworks (Fe-MOFs) composite electrospun nanofibrous membranes, J. Cleaner Prod., 2022, 370, 133566 CrossRef CAS.
- J. X. Tie, W. P. Li, H. Y. Liu, K. Huang, X. Mi, M. H. Wei and L. J. Hou, Efficient adsorption and reduction of Cr(VI) by a novel polyaniline modified magnetic iron-based waterworks sludge from aqueous solution, Chem. Eng. J., 2023, 451(3), 137673 CrossRef CAS.
- J. J. Chang, J. Zhang, H. Wang, Y. F. Bai, Y. Liu, Y. Z. Bi, H. Z. Zhang, H. H. Chen, S. Barnie and H. J. Xie, Cr (VI) adsorption and reduction by magnetite-humic acid adsorption complexes under mildly acidic conditions: Synergistic/antagonistic mechanism and multi-step reaction model, Chem. Eng. J., 2023, 451, 138648 CrossRef CAS.
- J. C. Han, G. J. Chen, L. P. Qin and Y. Mu, Metal respiratory pathway-independent Cr isotope fractionation during Cr (VI) reduction by shewanella oneidensis MR-1, Environ. Sci. Technol. Lett., 2017, 4(11), 500–504 CrossRef CAS.
- R. S. Cutting, V. S. Coker, N. D. Telling, R. L. Kimber, C. I. Pearce, B. L. Ellis, R. S. Lawson, G. Van der Laan, R. A. D. Pattrick and D. J. Vaughan, Optimizing Cr (VI) and Tc (VII) remediation through nanoscale biomineral engineering, Environ. Sci. Technol., 2010, 44(7), 2577–2584 CrossRef CAS PubMed.
- S. P. Xia, Z. L. Song, P. Jeyakumar, S. M. Shaheen, J. Rinklebe, Y. S. Ok, N. Bolan and H. L. Wang, A critical review on bioremediation technologies for Cr (VI)-contaminated soils and wastewater, Crit. Rev. Environ. Sci. Technol., 2019, 49(12), 1027–1078 CrossRef CAS.
- K. Mukherjee, R. Saha, A. Ghosh and B. Saha, Chromium removal technologies, Res. Chem. Intermed., 2013, 39(6), 2267–2286 CrossRef CAS.
- A. S. Yusuff, M. A. Lala, K. A. Thompson-Yusuff and E. O. Babatunde, ZnCl2-modified eucalyptus bark biochar as adsorbent: preparation, characterization and its application in adsorption of Cr (VI) from aqueous solutions, S. Afr. J. Chem. Eng., 2022, 42, 138–145 Search PubMed.
- N. Abdullah, N. Yusof, W. J. Lau, J. Jaafar and A. F. Ismail, Recent trends of heavy metal removal from water/wastewater by membrane technologies, J. Ind. Eng. Chem., 2019, 76, 17–38 CrossRef CAS.
- C. Pan, L. D. Troyer, P. Liao, J. G. Catalano, W. L. Li and D. E. Giammar, Effect of humic acid on the removal of chromium (VI) and the production of solids in iron electrocoagulation, Environ. Sci. Technol., 2017, 51(11), 6308–6318 CrossRef CAS PubMed.
- P. Sharma, J. Prakash, T. Palai and R. Kaushal, Surface functionalization of bamboo leave mediated synthesized SiO2 nanoparticles: Study of adsorption mechanism, isotherms and enhanced adsorption capacity for removal of Cr (VI) from aqueous solution, Environ. Res., 2022, 214, 113761 CrossRef CAS PubMed.
- Y. Zhao, W. K. Chang, Z. D. Huang, X. G. Feng, L. Ma, X. X. Qi and Z. H. Li, Enhanced removal of toxic Cr (VI) in tannery wastewater by photoelectrocatalysis with synthetic TiO2 hollow spheres, Appl. Surf. Sci., 2017, 405, 102–110 CrossRef CAS.
- C. B. Godiya, Y. H. Xiao and X. L. Lu, Amine functionalized sodium alginate hydrogel for efficient and rapid removal of methyl blue in water, Int. J. Biol. Macromol., 2020, 144, 671–681 CrossRef CAS PubMed.
- S. Yildiz and S. Sevinç, Heavy metal adsorption by dewatered iron-containing waste sludge, Ecol. Chem. Eng. S, 2018, 25(3), 431–456 CAS.
- J. J. Lian, F. J. Zhou, B. Bo Chen, M. Yang, S. S. Wang, Z. L. Liu and S. P. Niu, Enhanced adsorption of molybdenum(VI) onto drinking water treatment residues modified by thermal treatment and acid activation, J. Cleaner Prod., 2020, 244, 118719 CrossRef CAS.
- J. H. Wang, X. J. Han, Y. F. Ji and H. R. Ma, Adsorption of Cr(VI) from aqueous solution onto short-chain polyaniline/palygorskite composites, Desalin. Water Treat., 2015, 56(2), 356–365 CrossRef CAS.
- S. H. Shrestha, J. Kulandaivelu, K. Sharma, G. M. Jiang and Z. G. Yuan, Effects of dosing iron- and alum-containing waterworks sludge on sulfide and phosphate removal in a pilot sewer, J. Cleaner Prod., 2020, 387, 118719 Search PubMed.
- A. Khan, Y. Huo, Z. Qu, Y. W. Liu, Z. H. Wang, Y. Chen and M. X. Huo, A facile calcination conversion of groundwater treatment sludge (GTS) as magnetic adsorbent for oxytetracycline adsorption, Sci. Rep., 2021, 11(1), 5276 CrossRef CAS PubMed.
- S. Y. Zhu, G. Dong, Y. Yu, J. K. Yang, W. Yang, W. Fan, D. D. Zhou, J. C. Liu, L. L. Zhang, M. X. Huo and Y. Wang, Hydrothermal synthesis of a magnetic adsorbent from wasted iron mud for effective removal of heavy metals from smelting wastewater, Environ. Sci. Pollut. Res., 2018, 25, 22710–22724 CrossRef CAS PubMed.
- E. Cheraghipour and M. Pakshir, Environmentally friendly magnetic chitosan nano-biocomposite for Cu(II) ions adsorption and magnetic nano-fluid hyperthermia: CCD-RSM design, J. Environ. Chem. Eng., 2021, 9(2), 104883 CrossRef CAS.
- A. H. Almarri, Chitosan composites for thionine dye adsorption, Int. J. Environ. Anal. Chem., 2021, 1–12, 1904915 Search PubMed.
- C. D. S. Cardoso and L. Vitali, Chitosan versus chitosan-vanillin modified: An evaluation of the competitive adsorption of five emerging contaminants, Water, Air, Soil Pollut., 2021, 232(5), 1–13 Search PubMed.
- X. L. Yu, J. Zhang and Y. Zheng, Perchlorate adsorption onto epichlorohydrin crosslinked chitosan hydrogel beads, Sci. Total Environ., 2021, 761, 143236 CrossRef CAS PubMed.
- S. L. Fan, Z. Q. Huang, Y. J. Zhang, H. Y. Hu, X. Q. Liang, S. X. Gong, J. Zhou and R. Tu, Magnetic chitosan-hydroxyapatite composite microspheres: Preparation, characterization, and application for the adsorption of phenolic substances, Bioresour. Technol., 2019, 274, 48–55 CrossRef CAS PubMed.
- V. N. Le, T. N. Tu and J. Kim, Facile synthesis of Cu-based metal-organic framework/chitosan composite granules for toluene adsorption, Sep. Purif. Technol., 2023, 306, 122718 CrossRef CAS.
- Z. Y. Li, T. T. Li, L. B. An, P. F. Fu, C. J. Gao and Z. M. Zhang, Highly efficient chromium(VI) adsorption with nanofibrous filter paper prepared through electrospinning chitosan/polymethylmethacrylate composite, Carbohydr. Polym., 2016, 137, 119–126 CrossRef CAS PubMed.
- Y. Yang, Y. H. Zhang, G. Y. Wang, Z. B. Yang, J. R. Xian, Y. X. Yang, T. Li, Y. L. Pu, Y. X. Jia, Y. Li, Z. Cheng, S. R. Zhang and X. X. Xu, Adsorption and reduction of Cr(VI) by a novel nanoscale FeS/chitosan/biochar composite from aqueous solution, J. Environ. Chem. Eng., 2021, 9(4), 105407 CrossRef CAS.
- H. P. Yang, M. Hong and S. Y. Chen, Removal of Cr(VI) with Nano-FeS and CMC-FeS and transport properties in porous media, Environ. Technol., 2020, 41(22), 2935–2945 CrossRef CAS PubMed.
- J. L. Liu, B. Q. Zhou, H. Zhang, J. Ma and W. B. Zhang, A novel biochar modified by chitosan-Fe/S for tetracycline adsorption and studies on site energy distribution, Bioresour. Technol., 2019, 294, 122152 CrossRef CAS PubMed.
- D. D. Shao, X. X. Wang, J. X. Li, Y. S. Huang, X. M. Ren, G. S. Hou and X. K. Wang, Reductive immobilization of uranium by PAAM-FeS/Fe3O4 magnetic composites, Environ. Sci.: Water Res. Technol., 2015, 8, 3155 RSC.
- G. R. Liu, C. F. Song, Z. L. Huang, X. Jin, K. H. Cao, F. Y. Chen, B. H. Jin, R. Li and Q. Huang, Ultrasound enhanced destruction of tetracycline hydrochloride with peroxydisulfate oxidation over FeS/NBC catalyst: Governing factors, strengthening mechanism and degradation pathway, Chemosphere, 2023, 338, 139418 CrossRef CAS PubMed.
- H. Y. Chen, P. F. Lv, Q. M. Liu, P. F. Tian, S. Y. Cao and S. J. Yuan, Bonding iron chalcogenides in a hierarchical structure for high-stability sodium storage, J. Colloid Interface Sci., 2023, 637, 251–261 CrossRef CAS PubMed.
- D. M. Feng, X. Zhang, Y. Sun and T. Y. Ma, Surface-defective FeS2 for electrochemical NH3 production under ambient conditions, Nano Mater. Sci., 2020, 2(2), 132–139 CrossRef.
- C. T. Wei, M. X. Shen, K. L. Ai and L. H. Lu, Transformation from FeS/Fe3C nanoparticles encased S, N dual doped carbon nanotubes to nanosheets for enhanced oxygen reduction performance, Carbon, 2017, 123, 135–144 CrossRef CAS.
- S. C. Ma, Q. Cai, K. Lu, F. Liao and M. W. Shao, Bi-functional Au/FeS (Au/Co3O4) composite for in situ SERS monitoring and degradation of organic pollutants, J. Nanopart. Res., 2016, 18, 1–13 CrossRef CAS.
- S. T. Danalıoğlu, Ş. S. Bayazit, Ö. Kerkez-Kuyumcu and M. A. Salam, Efficient removal of antibiotics by a novel magnetic adsorbent: Magnetic activated carbon/chitosan (MACC) nanocomposite, J. Mol. Liq., 2017, 240, 589–596 CrossRef.
- R. Varma and S. Vasudevan, Extraction, characterization, and antimicrobial activity of chitosan from horse mussel modiolus modiolus, ACS Omega, 2020, 5(32), 20224–20230 CrossRef CAS PubMed.
- X. Zhong, Z. P. Lu, W. Liang and B. W. Hu, The magnetic covalent organic framework as a platform for high-performance extraction of Cr(VI) and bisphenol A from aqueous solution, J. Hazard. Mater., 2020, 393, 122353 CrossRef CAS PubMed.
- Z. M. Liu, G. Chen, L. C. Xu, F. P. Hu and X. Y. Duan, Removal of Cr(VI) from wastewater by a Novel adsorbent of magnetic goethite: Adsorption performance and adsorbent characterisation, ChemistrySelect, 2019, 4, 13817–13827 CrossRef CAS.
- R. Borah, S. Banerjee and A. Kumar, Surface functionalization effects on structural, conformational, and optical properties of polyaniline nanofibers, Synth. Met., 2014, 197, 225–232 CrossRef CAS.
- Z. Lu, H. Zhang, A. Shahab, K. Zhang and H. Ullah, Comparative study on characterization and adsorption properties of phosphoric acid activated bio char and nitrogen-containing modified biochar employing Eucalyptus as a precursor, J. Cleaner Prod., 2021, 12, 127046 CrossRef.
- C. B. Godiya, S. M. Sayed, Y. H. Xiao and X. L. Lu, Highly porous egg white/polyethyleneimine hydrogel for rapid removal ofheavy metal ions and catalysis in wastewater, React. Funct. Polym., 2020, 149, 104509 CrossRef CAS.
- J. Manuel, J. Salguero and R. P. Ramasamy, Synthesis and characterization of polyaniline nanofibers as cathode active material for sodium-ion battery, J. Appl. Electrochem., 2019, 49, 529–537 CrossRef CAS.
- C. B. Godiya, S. Kumarb and Y. H. Xiao, Amine functionalized egg albumin hydrogel with enhanced adsorption potential for diclofenac sodium in water, J. Hazard. Mater., 2020, 393, 122417 CrossRef CAS PubMed.
- X. Q. Liu, Y. Y. Zhang, Y. Liu and T. A. Zhang, Green method to synthesize magnetic zeolite/chitosan composites and adsorption of hexavalent chromium from aqueous solutions, Int. J. Biol. Macromol., 2022, 194, 746–754 CrossRef CAS PubMed.
- S. Sugashini and K. M. M. S. Begum, Column adsorption studies for the removal of Cr(VI) ions by ethylamine modified chitosan carbonized rice husk composite beads with modelling and optimization, J. Chem., 2012, 11, 460971 Search PubMed.
- X. Liu, H. L. Liu, K. P. Cui, Z. L. Dai, B. Wang and R. Weerasooriya, Adsorption-Reduction of Cr(VI) with Magnetic Fe-C-N Composites, Water, 2023, 15(12), 2290 CrossRef CAS.
- Z. Y. Wu, H. Zhang, E. Ali, A. Shahab, H. Y. Huang, H. Ullah and H. H. Zeng, Synthesis of novel magnetic activated carbon for effective Cr(VI) removal via synergistic adsorption and chemical reduction, Environ. Technol. Innovat., 2023, 30, 103092 CrossRef CAS.
- X. Q. Liu, Y. Liu and T. A. Zhang, Preparation of magnetic zeolite/chitosan composite using silane as modifier for adsorption of Cr(VI) from aqueous solutions, J. Vinyl Addit. Technol., 2021, 27, 640–654 CrossRef CAS.
- X. J. Hu, J. S. Wang, Y. G. Liu, X. Li, G. M. Zeng, Z. L. Bao, X. X. Zeng, A. W. Chen and F. Long, Adsorption of chromium (VI) by ethylenediamine-modified cross-linked magnetic chitosan resin: Isotherms, kinetics and thermodynamics, J. Hazard. Mater., 2010, 185(1), 306–314 CrossRef PubMed.
- V. P. Dinh, M. D. Nguyen, Q. H. Nguyen, T. T. T. Do and L. V. Tan, Chitosan-MnO2 nanocomposite for effective removal of Cr (VI) from aqueous solution, Chemosphere, 2020, 257, 127147 CrossRef CAS PubMed.
- B. Mahanty and S. Mondal, Synthesis of Magnetic Biochar Using Agricultural Waste for the Separation of Cr(VI) From Aqueous Solution, Arab. J. Sci. Eng., 2021, 46, 10803–10818 CrossRef CAS.
- J. Monjane, D. Chemane, A. Zimba, P. Dimande and A. Uamusse, Production of chitosan from crab shells using an aqueous extract of wood ash for the deacetylation of chitin: An innovative, eco-friendly, and low-cost method, Am. J. Polym. Sci. Techol., 2021, 7(2), 29–37 Search PubMed.
- N. Ismillayli, S. Hadi, N. K. T. Dharmayani, R. K. Sanjaya and D. Hermanto, Characterization of alginate-chitosan membrane as potential edible film, IOP Conf. Ser. Mater. Sci. Eng., 2020, 833, 012073 CrossRef CAS.
- A. Raghatate, F. D. C. Vega, O. V. Meraz, K. Ahmadi, N. M. Chaudhari, D. S. A. Puthirath, N. Castaneda, P. M. Ajayan, J. M. H. Ramirez, V. Balan and F. C. R. Hernández, Sustainable biocomposites for structural applications with environmental affinity, ACS Appl. Mater. Interfaces, 2022, 14(15), 17837–17848 CrossRef CAS PubMed.
- A. M. Bin, J. J. Lim, K. Shameli, N. A. Ibrahim and M. Y. Tay, Synthesis of silver nanoparticles in chitosan, gelatin and chitosan/gelatin bionanocomposites by a chemical reducing agent and their characterization, Molecules, 2011, 16(9), 7237–7248 CrossRef PubMed.
- D. W. Zhang, J. M. Wang, L. Ren, X. C. Meng, B. Y. Luan and Y. M. Zhang, A novel cationic-modified chitosan flocculant efficiently treats alkali-surfactant- polymer flooding-produced water, Polym. Bull., 2023, 80, 12865–12879 CrossRef CAS.
- O. A. Bulavchenko, A. A. Pochtar, E. Y. Gerasimov, A. V. Fedorov and V. V. Kaichev, Chemical and texture promoters in Cu-Fe-Al oxide nanocomposite catalysts for combustion of solid fuel gasification products, Appl. Catal., A, 2019, 590, 117364 CrossRef.
- A. Ramachandran, P. Anitha and S. Gnanavel, Structural and electronic impacts on corrosion inhibition activity of novel heterocyclic carboxamides derivatives on mild steel in 1 M HCl environment: Experimental and theoretical approaches, J. Mol. Liq., 2022, 359, 119218 CrossRef CAS.
- F. C. De-Godoi, E. Rodriguez-Castellon, E. Guibal and M. M. Beppu, An XPS study of chromate and vanadate sorption mechanism by chitosan membrane containing copper nanoparticles, Chem. Eng. J., 2013, 234, 423–429 CrossRef CAS.
- R. Bhatt, B. Sreedhar and P. Padmaja, Chitosan supramolecularly cross linked with trimesic acid-facile synthesis, characterization and evaluation of adsorption potential for chromium (VI), Int. J. Biol. Macromol., 2017, 104, 1254–1266 CrossRef CAS PubMed.
- L. L. Luo, S. Y. Cheng, L. H. Yue, Z. M. You and J. J. Cai, N-doped biochar from chitosan gel-like solution: Effect of hydrothermal temperature and superior aqueous
Cr (VI) removal performance, Colloids Surf., A, 2022, 641, 128426 CrossRef CAS.
- W. Q. Wu, Y. B. Li, J. Y. Jin, H. M. Wu, S. F. Wang and Q. H. Xia, A novel nonenzymatic electrochemical sensor based on 3D flower-like Ni7S6 for hydrogen peroxide and glucose, Sens. Actuators, B, 2016, 232, 633–641 CrossRef CAS.
- V. A. Chanturiya, I. Z. Bunin and M. V. Ryazantseva, The main features of the natural sulphides surface modification under the impact of high-power electromagnetic pulses, Inz. Miner., 2019, 21(1/2), 123–127 Search PubMed.
- L. Kang, H. P. Yang, J. M. Wu and X. N. Liu, Facile integration of FeS and titanate nanotubes for efficient removal of total Cr from aqueous solution: Synergy in simultaneous reduction of Cr(VI) and adsorption of Cr(III), J. Hazard. Mater., 2020, 398, 122834 CrossRef PubMed.
- X. Y. Lin, X. He, L. Lei, Y. F. Zhao, L. Z. Cui and G. Y. Wu, Development of ionic liquid filled chitosan capsules to remove Cr (VI) from acidic solution: adsorption properties and mechanism, J. Environ. Chem. Eng., 2022, 10(4), 108081 CrossRef CAS.
- C. S. Shen, H. Chen, S. S. Wu, Y. Z. Wen, L. N. Li, Z. J. Jiang, M. C. Li and W. P. Liu, Highly efficient detoxification of Cr (VI) by chitosan-Fe (III) complex: Process and mechanism studies, J. Hazard. Mater., 2013, 244, 689–697 CrossRef PubMed.
|
This journal is © The Royal Society of Chemistry 2024 |
Click here to see how this site uses Cookies. View our privacy policy here.