DOI:
10.1039/D4RA03059A
(Paper)
RSC Adv., 2024,
14, 27274-27287
Influence of pomelo pericarp essential oil on the structural characteristics of gelatin-arrowroot tuber flour-based edible films
Received
25th April 2024
, Accepted 20th August 2024
First published on 27th August 2024
Abstract
The present study examined the comprehensive effects of incorporating pomelo pericarp essential oil (PEO) at varying concentrations (0.5, 1.0, 1.5, and 2.0%) into gelatin-arrowroot tuber flour (GEL-ATF) based edible films and evaluated the influence on various structural properties. ATF was prepared from Maranta arundinacea L. tubers using a carefully controlled method to ensure its quality and suitability as a polysaccharide base in film formulations. The results indicated that adding PEO to the GEL-ATF films decreased L, a, and b color values and increased opacity values, especially at higher PEO concentrations. Furthermore, the appearance of both GEL-ATF and GEL-ATF-PEO films exhibited similar characteristics. Incorporating PEO significantly reduced moisture content and water vapor permeability (WVP), indicating enhanced barrier properties against moisture. Additionally, an increase in PEO concentration resulted in decreased film solubility. A decrease in tensile strength (TS) but an increase in elongation at break (EAB) was observed in the GEL-ATF films with higher PEO concentrations (>1% PEO). Slight variations in thermal degradation patterns with increased PEO addition in GEL-ATF were noticed, while X-ray diffractometry (XRD) and Fourier transform infrared spectroscopy (FTIR) results of the tested films provided insights into structural and chemical modifications, indicating changes in crystallinity and molecular interactions upon increased PEO concentration in the film compositions. The microstructural observations confirmed that PEO incorporation led to smoother film surfaces, suggesting a more uniform matrix, which could enhance the film's barrier and mechanical properties. Furthermore, applying PEO into GLE-ATF films exhibited strong antimicrobial activity against Bacillus cereus ATCC 11778. Overall, the present study found that the higher PEO (>1%) concentrations significantly influenced the physical and mechanical properties of GEL-ATF-based edible films. This newly developed edible film could be an effective alternative to inedible polymers for sustainable food packaging solutions.
Introduction
Global plastic production has significantly increased over recent decades, with packaging applications accounting for 40% of this output.1 The global prevalence of synthetic plastic packaging significantly contributes to environmental pollution, underscoring the critical need to explore various alternatives to mitigate its detrimental effects. The apparel industry alone generated 9.2 million tons of plastic pollution in 2019, highlighting the substantial role of packaging in environmental degradation.2 Edible packaging is gaining traction in the food industry for its potential to reduce environmental impact by minimizing plastic waste and promoting sustainability. Incorporating edible films—especially those with natural antimicrobials—into food packaging not only extends the shelf life of perishable goods but also aligns with growing consumer demand for eco-friendly, health-conscious solutions.3 These biopolymer-based films, made from natural polymers like proteins and polysaccharides, offer significant environmental advantages over traditional plastic packaging due to their biodegradability and reliance on renewable resources. They decompose naturally or can be composted, reducing plastic waste and pollution. Additionally, their production often utilizes agricultural by-products, supporting a circular economy. However, challenges remain, such as the environmental costs of biopolymer cultivation and the potential inclusion of non-biodegradable additives in the manufacturing process. To maximize their environmental benefits, it's essential to optimize raw material sourcing and production methods.4
The rise of edible polymer-based packaging, such as films and coatings, marks a significant shift away from traditional plastics and these edible films, particularly those made from proteins and polysaccharides, whether as single materials or composites, are becoming widely recognized in the food industry for their contributions to safety and multifunctional applications.5,6 Edible packaging materials offer more than just an eco-friendly alternative to plastics; they also provide functional benefits such as acting as barriers against moisture, gas, and flavor loss, thereby improving food quality and shelf life. Moreover, these materials play a crucial role in regulating water activity and controlling mass transfer during food processing. Numerous studies have demonstrated the efficiency of edible films and coatings in prolonging the shelf life of perishable goods, such as fruits, vegetables, mushrooms, meat, and fish, by inhibiting microbial growth, enhancing antioxidant properties, and maintaining sensory attributes.7 By reducing weight loss, color changes, and ripening in fresh produce, these innovations address post-harvest spoilage and contribute to decreasing food waste while preserving nutritional integrity.
Studies suggest that biopolymers show potential in the development of edible films, presenting notable benefits in the realm of food packaging. To enhance the efficiency of such films, it is crucial to investigate a broader range of polymers and incorporate additives.8,9 Biopolymers like polysaccharides, proteins, and lipids have been extensively studied for their role in edible film production.10 Gelatin (GEL) is a lustrous, solid biopolymer that is white or yellowish and translucent. It is a partially hydrolyzed product of collagen derived from animal connective tissues, specifically from pork, cow, and poultry. Recently, fish skin has contributed abundant GEL as an alternative source to mammals.11,12 Gelatin is an environmentally friendly polymer, and due to its viscoelastic nature, it has been widely utilized in various industries, including food packaging, pharmaceuticals, cosmetics, etc.1 The viscoelastic nature of GEL is controlled by its molecular weight, amino acid composition, and level of polymerization chain.13 While GEL shows effective gas barrier properties and swells in water, it also demonstrates weaknesses in mechanical strength and water vapor permeability; to overcome these issues, GEL is often combined with other edible polymers and natural additives.14 The effectiveness of GEL in food packaging has been enhanced by mixing it with other biopolymers like chitosan, starch, and glucomannan or natural compounds such as polyphenols and essential oils.9,15–17 Arrowroot (Maranta arundinacea L.), a perennial tuberous plant native to South America's tropical forests, is a member of the Marantaceae family. It contains high amylose content (>35%), exhibits excellent gelling ability and transparency, can form continuous matrices, and is highly suitable for forming edible packaging.18 Arrowroot tuber flour/starch (ATF) has been extensively studied for producing edible films with various additives to enhance their properties. Furthermore, studies have shown that incorporating ATF in edible films can increase water vapor permeability, moisture content, thickness, and decreased solubility in water.19,20
While edible films present numerous benefits, including barrier properties and active food preservation, their widespread adoption is hindered by limitations in mechanical strength, water vapor permeability (WVP), and the consistency of antimicrobial effectiveness.21,22 Generally, adding essential oils (EOs) to edible films is crucial in enhancing the antimicrobial properties of edible films used in food preservation.23 Furthermore, adding EO to the edible film can also improve the mechanical properties by altering their tensile strength (TS) and elongation at break (EAB) values. Essential oil can act as a plasticizer and enhance the flexibility and stretchability of the films.24 Furthermore, the increasing concentration of EO could increase the film matrix's hydrophobicity, thus improving water resistance and moisture barrier properties. Several studies have suggested that adding EO in films made of water-like biopolymers could significantly improve the film's physical properties due to the hydrophobic nature of the EO.25,26 The Citrus grandis L. Osbeck fruit, commonly known as Pomelo, is native to Southern Asia and is found locally in countries like China, Japan, Vietnam, Malaysia, India, and Thailand.27 Pomelo pericarp essential oil (PEO) offers a wide range of chemical components such as limonene, γ-terpinene, α-phellandrene, β-myrcene, 1R-α-pinene, and β-pinene, and exhibits substantial antibacterial, antifungal, and antioxidant activities.28,29 Although several studies have been conducted on PEO's efficacy and functional properties, no study has been conducted on using PEO as an additive to GEL-based biopolymers to test their capacity to improve the edible film's properties. Consequently, the present research aims to develop edible films that combine GEL and ATF, supplemented with PEO at varying concentrations, and to comprehensively evaluate the physical, mechanical, and structural characteristics of the innovative GEL-ATF-PEO-based edible films that have thus been developed.
Experimental
Materials, chemicals, and media
Commercial fish skin gelatin was purchased from JR F&B Co., Ltd. in Bangkok, Thailand. Glycerol was obtained from J. T. Baker, NJ, USA. The essential oil derived from pomelo peel (Citrus maxima (Burm.) Merr.) utilized in this study was procured from Thai China Flavors and Fragrances Industry Co., Ltd., in Nonthaburi, Thailand. All the media for microbial analysis were purchased from HiMedia, located in Mumbai, India.
Preparation of arrowroot tuber flour
Arrowroot tubers (Maranta arundinacea L.) obtained at 12 months of maturity were procured from a contracted farmer located in the Sai Yok district of Kanchanaburi province, situated in western Thailand. Arrowroot tuber flour was carefully processed in accordance with the methodology described by Venkatachalam et al.18 Prior to processing, the arrowroot tubers underwent a thorough washing with distilled water to eliminate any surface impurities, following which the moisture present on the arrowroot surface was desiccated at room temperature overnight. Subsequently, the tubers were homogenized using a blender (WF-20B, Thai grinder, Bangkok, Thailand), with the addition of distilled water at a ratio of 1
:
2. The resultant mixture was then filtered through a muslin cloth to remove any chunky particles and facilitate the settling of the homogenate at the bottom. The specific procedure was duplicated twice, after which the aqueous part containing the arrowroot tuber flour was decanted, with the solid residue being dried in a hot air oven (Binder, model FD 115, Tuttlingen, Germany) at 60 °C for 4 h. The dried residue was subsequently pulverized using a hammer mill (Retsch, model ZM 1, Burladingen, Germany), sifted through a 100-mesh screen, and designated as ATF.
Formation of edible film
Film-forming solutions were prepared in accordance with the methods described by Loo and Sarbon,30 with slight modifications. Arrowroot tuber flour at a concentration of 1% (w/v) was dispersed in distilled water and heated to 85 ± 2 °C on a hot plate with a magnetic stirrer, continuously stirring for 30 min. Concurrently, fish skin gelatin at 3% (w/v) was dissolved in distilled water at 60 °C until a clear solution formed over 30 min. This solution was then combined with the gelatinized ATF at 60 °C and stirred for 30 min. Glycerol at 1% (w/v) was subsequently incorporated, with constant stirring for another 30 min. Pomelo pericarp essential oil was added to the film-forming solutions in varying concentrations of 0.5, 1.0, 1.5, and 2.0% (w/v of the film solution), each stirred for 30 min. The resulting mixtures (15 mL each) were poured into sterile Petri dishes (9 cm in diameter) and air-dried for 7 h at 50 °C. After drying, the films were carefully peeled off and conditioned for 48 h at 25 °C with 55 ± 2% relative humidity before further analysis. Films made by GEL and ATF without adding PEO served as the control samples.
Characterization and properties of testing
Color characteristics, opacity, and appearance. The color characteristics of the tested films, including lightness (L), redness (a), and yellowness (b) values, were assessed using a Hunter Lab colorimeter (model WR18, Jedto, Pathum Thani, Thailand).The opacity of the films was determined by measuring their absorbance at a wavelength of 600 nm using a UV-Vis spectrophotometer (Shimadzu UV-1800, Shimadzu Corporation, Kyoto, Japan). The opacity values were calculated using the following equation.
In the equation,
A denotes the absorbance of the film at 600 nm, while
X represents the film thickness in mm. The film opacity is thus expressed as
A600/mm.
The appearance of the tested edible films was documented using a digital image taken with a handheld digital camera (Coolpix B500, Nikon, Tokyo, Japan).
Moisture content
The moisture content of the tested films was assessed by initially determining the weight (W0) of a film sample measuring 50 × 20 mm using a digital analytical balance (Sartorius, BSA2245-CW, Göttingen, Germany). Subsequently, the film underwent drying at 105 °C until reaching a consistent weight (W1). The moisture content of the film was then calculated using the subsequent equation and presented as a percentage:
Water vapor permeability
Water vapor permeability assessments were carried out in accordance with the methodology outlined by Zhao et al.,31 with some modifications. Specimens of dried film measuring 4 × 4 cm were prepared, and each specimen was positioned atop a clean and dry plastic cup containing 15 g of silica gel (Catalog no. C0707, Preeco Engineering Sdn. Bhd., Selangor, Malaysia). Subsequently, each receptacle was assessed for weight and positioned within a desiccator immersed in distilled water at ambient temperature. The specimens were subject to hourly weight measurements with a precision of ±0.0001 g throughout an 8 h timeframe. The WVP value was determined by utilizing a specific formula predicated on the alterations in weight observed over time.
WVP (g mm m−2 h−1 Pa−1) = W × X/A × t × (P2 − P1) |
where X is the average initial thickness of the film in mm, A is the permeation area of the film in square meters, t denotes the time in hours, and P2 − P1 is the difference in partial pressure between the atmosphere containing silica desiccant and the atmosphere over pure water. The differential partial pressures based on temperature were determined using a vapor pressure of water chart.32
Film solubility
Film solubility was evaluated using the method described by Zhao et al.31 Film fragments were cut (2 × 2 cm), dried at 70 °C for 24 h, and weighed. These specimens were then submerged in a 0.01% (w/w) sodium benzoate solution with 20 mL of distilled water enclosed in a sealed tube for 24 h. After immersion, the mixture was filtered using Whatman no. 4 filter paper, rinsed with 10 mL of distilled water, and the films were dried again at 70 °C for another 24 h to determine their final dry mass. This process was replicated three times for each formulation. Film solubility was calculated based on the initial and final dry masses using the following formula:
Film thickness
The thickness of the examined films was measured using a portable digital micrometer (Mitutoyo 293-340-30 External Micrometer, Kawasaki, Japan), adhering to the methods described by Venkatachalam et al.33 Each treatment involved assessing ten films, with the thickness of each film measured at six random locations. The obtained results are reported in mm.
Mechanical properties
The mechanical properties of the films, specifically TS and EAB, were evaluated using a Universal Testing Machine (Instron 3369, MA, USA) by following the procedure described by Sutharsan et al.34 Film samples were prepared in rectangular shapes measuring 1 cm × 10 cm and tested using a double clamp setup with an initial grip separation of 50 mm and a cross-head speed of 60 mm min−1. The TS and EAB values were calculated using the following equations:
where F represents the maximum tensile force of film (N), W and T are the width and thickness of film, respectively.
where L1 represents the film's length after stretching, L0 is the film's original length.
Thermogravimetric analysis
Thermogravimetric analysis (TGA) was performed to measure the weight reduction of the film sample using a thermogravimetric analyzer (SDT Q-600, TA Instruments, Artisan Technology Group, Champaign, IL, USA) by following the method of Cao et al.35 Each film sample, with a mass between 5–10 mg, was exposed to a temperature range from 25 to 500 °C at a consistent heating rate of 10°C min−1. The results are expressed in weight (%).
X-ray diffractometry
The crystal structures of the films were analyzed using an X-ray diffractometer (D2 Phaser, Bruker, Germany), following a modified method of Cao et al.35 The analysis utilized a copper source at a voltage of 30 kV and a current of 10 mA, with a wavelength of λ = 1.5406 Å. The diffraction spectrum's intensity was measured over an angular range of 2θ (1–60°) at a scanning rate of 2° per min. The results are expressed in intensity (a.u.).
Fourier transform infrared spectroscopy
Fourier transform infrared spectroscopic (FTIR) (PerkinElmer, Spectrum Two, Bucks, UK) analysis of the examined edible films followed the method of Cao et al.35 The films were combined with potassium bromide at a ratio of 1
:
100, pulverized to a fine consistency, and compacted into pellets. The spectroscopic analysis aimed to detect potential interactions among the constituents of the films, specifically GEL, ATF, glycerol, and PEO, and this was accomplished by scanning the specimens 16 times over a 400–4000 cm−1 wavelength with an 8 cm−1 resolution for each spectrum recorded. The results are expressed in transmittance (%).
Microstructural analysis
The microstructure of the films was examined using a scanning electron microscope (SEM) (SU3900, Hitachi, Ltd., Tokyo, Japan) by following a procedure described by Venkatachalam et al.,33 with minor modifications. The film samples were prepared in two stages: surface analysis and cross-sectional analysis. Both specimens were cryo-fractured in liquid nitrogen and coated with a thin layer of gold for 5 min using a sputter coater. The prepared samples were then analyzed under the SEM at an acceleration voltage of 15 kV and a magnification level of 1000×.
Antimicrobial activity
The antibacterial effectiveness of the films was individually evaluated against two Gram-positive bacteria (Bacillus cereus ATCC 11778 and Staphylococcus aureus ATCC 25923) and two Gram-negative bacteria (Escherichia coli ATCC 25922 and Pseudomonas aeruginosa ATCC 27853) using a modified disc surface spreading method as described by Al-Hashimi et al.36 To elaborate, bacterial suspensions were prepared at turbidity equivalent to a McFarland 0.5 standard, reaching 108 CFU mL−1. These adjusted bacterial suspensions were spread on Mueller–Hinton agar, followed by the placement of round film discs (0.6 mm in diameter) in direct contact with the inoculated agar medium, and then the incubation was carried out at 37 °C for 24 h. The antimicrobial effectiveness was examined in triplicate by measuring the diameter (including the diameter of film discs) of the inhibition zone formed around the film. The results are expressed in the inhibition zone (mm).
Statistical analysis
All analysis data are presented as the mean values with their respective standard deviations (n = 3). The study employed a one-way analysis of variance (ANOVA) along with the Duncan multiple range test, with a significance level set at 0.05, to detect any notable discrepancies among the mean values. The statistical analysis in this study was carried out using the SPSS statistical software (v6, for window, from IBM, Armonk, NY, USA). Furthermore, the graphical abstract figure was made with BioRender.
Results and discussion
Color characteristics, opacity, and appearance
The characteristics of films, particularly their color, opacity, and appearance, significantly influence consumer purchasing decisions.37,38 This study investigated the effects of varying concentrations of PEO on the color characteristics of films made from GEL and ATF, with the color properties detailed as L, a, and b values presented in Fig. 1. Incorporating PEO into the film matrix resulted in observable changes in color values, which generally decreased with the addition of PEO, and the intensity of this decrease became more pronounced with increasing PEO concentration in the film matrix. The control films exhibited the highest L, a, and b values of 94.23, 1.36, and −1.24, respectively, while films with added PEO showed a range of color values: 86.54 to 93.43 for L, −0.80 to −1.21 for a, and 0.41 to 1.33 for b. Notably, films with 2.0% PEO concentration exhibited the lowest color values among all PEO-added films, precisely 86.54 for L, 1.36 for b, and −1.24 for a. The study found that changes in the color appearance of the films were closely linked to the composition of their components. This is in accordance with the study of Choo et al.,39 where they found that adding essential oil into starch-based film adversely affected the film color. Gelatin-arrowroot tuber flour composite edible film did not negatively impact the film's color characteristics, as evidenced by the high values of L, a, and b. However, adding PEO at various concentrations had a minor effect on these values, except for the films with 2.0% PEO, which showed a significant loss in color values compared to the control. Incorporating EO into the protein–starch-based composite films could lead to a decrease in color values due to alterations in properties such as water vapor barrier, moisture content and water solubility, and these changes can impact the film's visual appearance and color stability.5,40 The variation in color values of EO-incorporated films may be attributed to light scattering and changes in the film matrix, thus negatively impacting color, and this phenomenon is likely due to the interaction between essential oils and film-forming materials altering the film's optical properties.10,15 Opacity is a crucial optical property in edible films as it significantly influences the appearance of food products. The opacity of edible films can vary based on the materials used in their formulation.9 The opacity results exhibited slight variations among the films, as indicated in Fig. 1. Incorporating PEO into the compositions of GEL-ATF films increased the opacity level, which was directly proportional to the concentration of PEO. Nevertheless, films containing lower levels of GEL-ATF-PEO did not display significant differences in opacity compared to the control film. Only at higher concentrations of PEO (>1.5%) did a noticeable disparity in opacity values become evident when compared to the control films. Increasing the concentration of EO in the film composition typically results in elevated levels of hydrophobic components within the film. These components play a significant role in establishing an impermeable matrix in the film by the oil droplets, consequently enhancing light scattering and raising opacity levels.41 In terms of appearance, there was no significant difference among the films tested, as depicted in Fig. 2. All films exhibited a similar appearance, and the addition of PEO did not alter the overall look, which was slightly translucent with a grayish silver color.
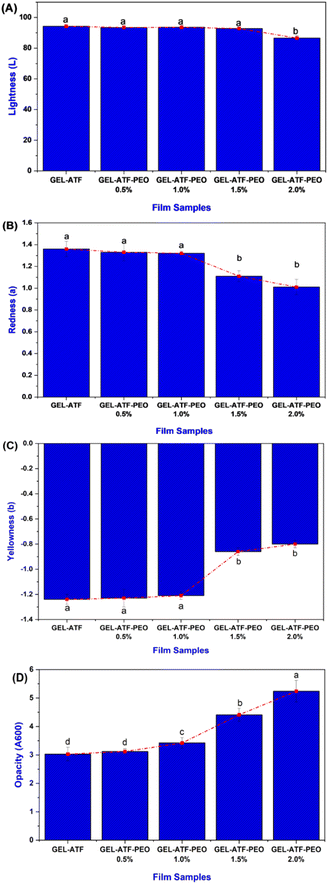 |
| Fig. 1 Differences in lightness (A), redness (B), yellowness (C), and opacity (D) of gelatin-arrowroot tuber flour (GEL-ATF)-based edible films with and without added pomelo pericarp essential oil (PEO) at varying concentrations. Different letters in the graph indicate significant differences between the means (P < 0.05). | |
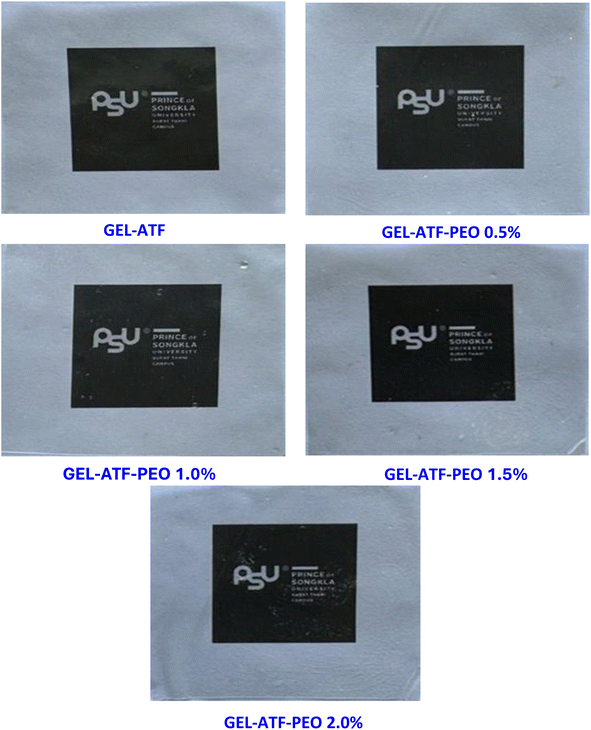 |
| Fig. 2 Differences in appearance of gelatin-arrowroot tuber flour (GEL-ATF)-based edible films with and without added pomelo pericarp essential oil (PEO) at varying concentrations. | |
Moisture content, water vapor permeability and film solubility
In general, edible films are designed to act as a barrier against moisture, gas, and other external factors.42 The moisture content of edible films, influenced by the type and concentration of plasticizers and additives in their formulation, critically impacts their properties and performance.43,44 Fig. 3A presents the moisture content of the GEL-ATF films, with or without PEO. The findings indicated a notable moisture content level in the control films; conversely, the GEL-ATF-PEO films exhibited lower moisture content. The elevation of PEO concentration led to a considerable reduction in moisture levels in the tested films. Film compositions with low PEO concentrations did not differ significantly from the control; however, a noticeable distinction was observed when PEO concentrations exceeded 1.5%. This is in accordance with the study of Venkatachalam and Charoenphun,45 who also found that adding PEO in the rice flour-based edible film decreases moisture content. Bhatia et al.46 reported that an increase in EO concentration within the film composition enhances the film's hydrophobic characteristics and thus induces a reduction in the film matrix's affinity for water molecules, decreasing moisture content. The permeability of water vapor plays a pivotal role in determining the effectiveness of food packaging, given that the barrier film employed must inhibit or reduce the passage of water molecules between the food product and its surrounding atmosphere.47 Water vapor permeability of biopolymer-based films is a complex phenomenon influenced by factors including temperature, membrane thickness, and the incorporation of additives.48 The results of the WVP in GEL-ATF films with or without PEO exhibited a similar trend to the moisture content results (Fig. 3B). The increase in PEO concentration from 0.5% to 2.0% within the formulation of the GEL-ATF films led to a proportional decrease in the WVP rate of the tested edible films. Film samples containing higher concentrations of PEO demonstrated a lower level of WVP than other tested films. The decrease in the WVP rate of edible films with higher concentrations of EO can be attributed to enhanced hydrophobicity, reduced availability of hydrophilic groups, and the creation of empty spaces within the film matrix. Essential oils are commonly known for their ability to lower WVP in edible polymer films due to their hydrophobic characteristics.49 The solubility data of GEL-ATF films, with or without the presence of PEO, is presented in Fig. 3C. The results demonstrated a decreasing trend with PEO inclusion in GEL-ATF films. The control films showed a solubility efficiency of 41.98%, whereas GEL-ATF films incorporated with PEO revealed solubility levels ranging between 36.56% and 30.86%, with PEO concentrations of 0.5% and 2.0%. Compared to the other tested films, the GEL-ATF-PEO 2.0% formulation exhibited superior control over solubility. The decreased solubility in protein–starch-based films that are incorporated with EOs is attributed to the formation of protein–amylose–lipid complexes. These complexes form tight helical structures through hydrophobic interactions, which limit the exposure and exploit the hydrophilic nature of the film matrix. Consequently, a lower film solubility is observed. This is in accordance with the study of Al-Hashimi et al.36 and López et al.50
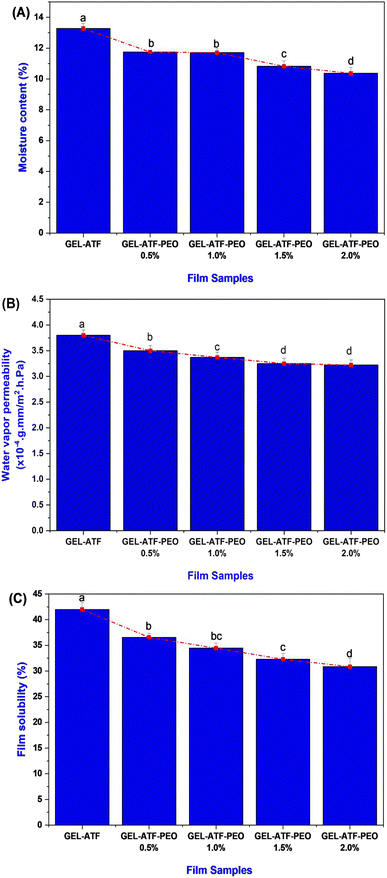 |
| Fig. 3 Differences in moisture content (A), water vapor permeability (B), and film solubility (C) of gelatin-arrowroot tuber flour (GEL-ATF)-based edible films with and without added pomelo pericarp essential oil (PEO) at varying concentrations. Different letters in the graph indicate significant differences between the means (P < 0.05). | |
Thickness, tensile strength, and elongation at break
The incorporation of EO can impact the physical and mechanical properties of the films.51 The film thickness of GEL-ATF-based film incorporated with or without PEO is presented in Fig. 4A. Despite the film variables, non-significant differences were noticed in the tested films. A slight increment was observed in the PEO-incorporated GEL-ATF films; however, the differences were minimal between the PEO-incorporated samples. The increased PEO concentration influences the thickness value slightly among the PEO film samples. Leiva et al.52 reported that incorporating EO in the edible film matrix may act as a plasticizer and, thus, increase the film thickness. Sanyang et al.53 found that when plasticizers are added to the film composition, they interact with polymer chains to create more free space within the structure, resulting in a looser arrangement of these chains and a thicker film. Increasing the concentration of EO in the edible film composition can amplify the plasticizer effect, resulting in changes in film thickness, mechanical properties, and functional characteristics.54 The TS of GEL-ATF-based edible films incorporated with or without PEO is presented in Fig. 4B. Adding PEO into the GEL-ATF film matrix significantly altered the structural properties, thus reflected in the TS. Among the film samples, the GEL-ATF films exhibited a high level of TS, whereas the increasing concentration of PEO adversely decreased the TS (P < 0.05). The higher PEO concentration (>1.5%) exhibited a severe loss of TS than the other tested samples. On the other hand, the EAB of GEL-ATF-based edible film incorporated with PEO had shown higher values than the control samples (Fig. 4C). Furthermore, the increased concentration of PEO in the GEL-ATF composition significantly improved the EAB levels in the tested films. Among the samples, EAB was predominantly high in the GEL-ATF-PEO 2.0% compared to the control, which exhibits the lowest EAB levels. Venkatachalam et al.33 found that incorporating EOs into edible polymer film compositions significantly affects TS and EAB. However, the impact varies depending on the type of EO and concentrations used. Higher concentrations of EOs can lead to more pronounced effects on both TS and EAB in the edible film. Szram et al.55 found that adding a higher concentration of EO in the composite edible film composition had inversely affected the tensile strength; higher concentration led to lower TS. On the other hand, increasing EO concentration in the edible film composition can enhance the EAB, improving the film's flexibility and stretchability.56
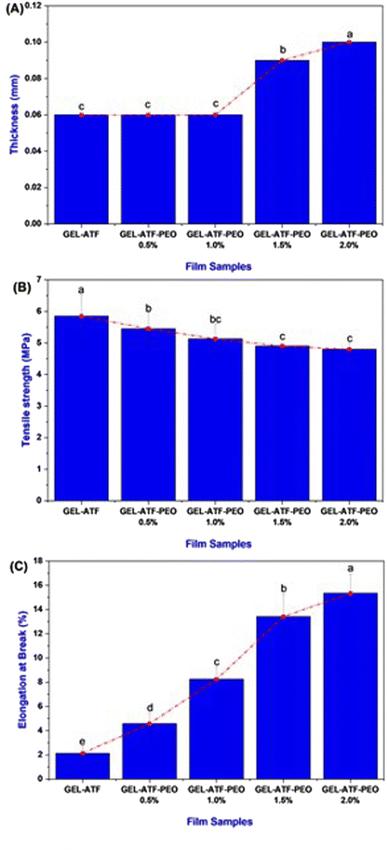 |
| Fig. 4 Differences in thickness (A), tensile strength (B), and elongation at break (C) of gelatin-arrowroot tuber flour (GEL-ATF)-based edible films with and without added pomelo pericarp essential oil (PEO) at varying concentrations. Different letters in the graph indicate significant differences between the means (P < 0.05). | |
Thermogravimetric analysis, X-ray diffractometry and Fourier transform infrared spectroscopy
Thermogravimetric analysis plays a crucial role in the comprehensive characterization of edible films as it enables the examination of their thermal behavior, stability, and degradation patterns.8 In this study, the TGA assesses the thermal stability of the tested edible films by evaluating their thermal behavior across a broad temperature range. Patnode et al.57 have documented that behavioral observations from assessing biopolymer-based edible films in the temperature range of 20 to 500 °C via TGA accentuate their suitability for incorporation in food packaging. Fig. 5A depicts the TGA results for GEL-ATF-based edible films, with and without incorporating PEO. Each tested film sample underwent two phases of thermal degradation. The first phase occurred within the temperature range of 60 to 120 °C, which is attributed to the loss of free and bound water, along with volatile compounds present in the GEL-ATF film matrix, and thus resulted in a weight loss of 6.11% for the control film and 5.81–5.83% for films with added PEO. Wang et al.16 reported that weight loss observed in the films at below <120 °C indicated a lower water absorption from film matrices, and the hydrogen bonds mostly link it. The second phase of thermal degradation occurred between 200 and 400 °C, related to the breakdown of low molecular weight compounds and chemical degradation within the GEL-ATF composition, leading to a weight loss of 62.08% in control and 60.28% in GEL-ATF-PEO 0.5%, with 60.76%, 61.57%, and 59.64% for GEL-ATF-PEO 1.0%, 1.5%, and 2.0%, respectively. Among all the samples, the control film showed slightly less degradation, whereas films incorporating PEO exhibited slightly increased thermal degradation; however, the overall difference in degradation between films with and without PEO was insignificant. Several studies have reported similar findings that adding EO into the edible films did not significantly affect the TGA values.58 X-ray diffractometry (XRD) is widely used to investigate crystallinity, phase composition, and molecular arrangement within films.59 Fig. 5B presents the XRD diffractograms of GEL-ATF-based films with and without PEO incorporation. The overall XRD patterns of the tested films displayed nearly identical configurations, albeit with variations in peak intensities. The results revealed two distinct peaks: a major peak at 21°, consistent across all samples, and a minor peak within the 16–17° range, observed in both GEL-ATF and GEL-ATF-PEO samples. Notably, as the concentration of PEO increased, there was a gradual reduction in the intensity of the smaller peak. This minor peak was absent at a PEO concentration of 2.0%, indicating a significant alteration in the crystalline structure or phase distribution within the film due to the increased PEO content. The increasing concentration of PEO in the GEL-ATF-based edible film composition could have altered the triple helix structure of the gelatin in the composition and thus induced the broad spectrum of XRD in the PEO-treated film samples. Furthermore, Charles et al.60 reported that the film-casting process could transform the starch structure into an amorphous form due to thermal treatment. This transformation is followed by the disruption of intermolecular interactions, specifically hydrogen bonding, between the polymers and additives. Consequently, this process adversely affects the crystallinity pattern of the edible film. Bhatia et al.46 reported that increasing concentrations of spearmint EO in GEL-based edible films significantly altered the films' crystallinity. Fourier transform infrared spectral characteristics are crucial for comprehending the composite material properties of the film, thereby influencing its potential applications in food preservation and sensory attributes.17 Fig. 6 illustrates the FTIR spectrum of the GEL-ATF films incorporated with or without PEO. The FTIR spectrum of the tested samples displayed a wide array of bands. Moreover, the elevation in PEO concentration within the film formulation increased the intensity (% T) of the observed FTIR bands. A prominent broad band appeared within the absorbed band patterns in the PEO-infused GEL-ATF films at 3268 cm−1, indicating –OH stretching and H bonding interaction between GEL and ATF with PEO.61 Similarly, the elevation of PEO concentration in the film blend exhibited varying band intensities observed at 2926 cm−1, representing the C–H group and implying an interaction between the polymers and EO.46 The examined GEL-ATF-based film samples have also revealed numerous distinct absorption bands (550–1610 cm−1) across the spectrum. The band ranging from 1690 to 1550 cm−1 corresponds to C
O stretching vibrations, commonly linked to carboxylic groups in gelatin's amino acids or starch's acetyl groups.62 The region from 1490 to 1400 cm−1 indicates C–H bending vibrations characteristic of alkanes, suggesting the structural elements of starch or GEL.63 Ester functionalities are indicated by C–O stretching vibrations within the 1300 to 1000 cm−1 range, potentially originating from essential oil or esterified starch.64 C
C–H bending in the 980 to 860 cm−1 range denotes vinyl groups, likely constituents of the PEO.65 Aromatic compounds, contributing to the film's odor and potentially its antimicrobial characteristics, are unveiled by the aromatic C–H bending observed from 800 to 700 cm−1.66
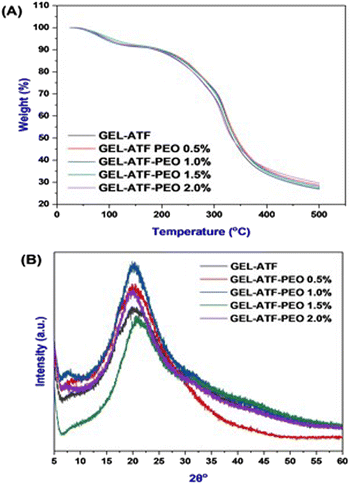 |
| Fig. 5 Differences in thermogravimetric analysis (A), and X-ray diffractometry (B) values of gelatin-arrowroot tuber flour (GEL-ATF)-based edible films with and without added pomelo pericarp essential oil (PEO) at varying concentrations. | |
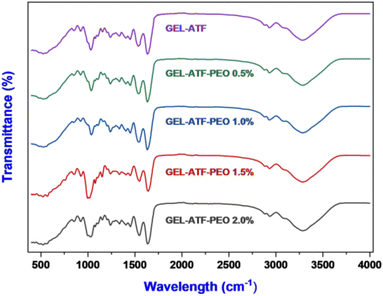 |
| Fig. 6 Differences in Fourier transform infrared spectra of gelatin-arrowroot tuber flour (GEL-ATF)-based edible films with and without added pomelo pericarp essential oil (PEO) at varying concentrations. | |
Microstructural observations
Understanding the microstructure and interactions among film ingredients is crucial for regulating the mechanical and barrier properties of edible films and coatings, which are essential for both material science fundamentals and practical applications.67 Microstructural observations of the GEL-ATF films with and without PEO at various concentrations are depicted in Fig. 7. The overall trend suggests that the GEL-ATF-based film exhibits a rough surface, which becomes smoother with the addition of PEO, and the smoothness increases as the PEO concentration is raised. The control film's surface microstructure shows a relatively rough texture, with densely packed areas interspersed with larger voids or gaps. This morphological observation indicates a semi-continuous GEL and ATF network with potential phase separation. This is in accordance with the study of Firoozmand and Rousseau.68 The pores likely result from dehydration during the film formation, as water molecules exit the matrix. Larger holes may be due to air bubbles or volatiles being released during drying. Wongphan and Harnkarnsujarit69 reported that the observation of rough and aggregation of fine clumps on the surface of the starch-based composite film could be due to miscibility and incompatibility issues. In contrast, films with added PEO present a more homogeneous surface than the control GEL-ATF film, and increasing the PEO concentration further smooths the film surface. Venkatachalam et al.33 found that the application of EO into the starch-based composite film can impact the microstructure differently based on the type and concentration of the EO used, and the EO with smaller droplet size could interact within the polymer matrix more efficiently, resulting in a smoother surface. El Kolli and El Kolli11 found that increased EO concentration in the GEL-based edible film had significantly improved the microstructural characteristics. Films with a higher concentration of GEL-ATF-PEO exhibit no distinct pores or phase separation, suggesting that including PEO leads to a more uniform matrix by probably filling the gaps in the films that would otherwise become pores, potentially enhancing the film's barrier properties and as well as mechanical properties. This is in accordance with the findings of Elma et al.70
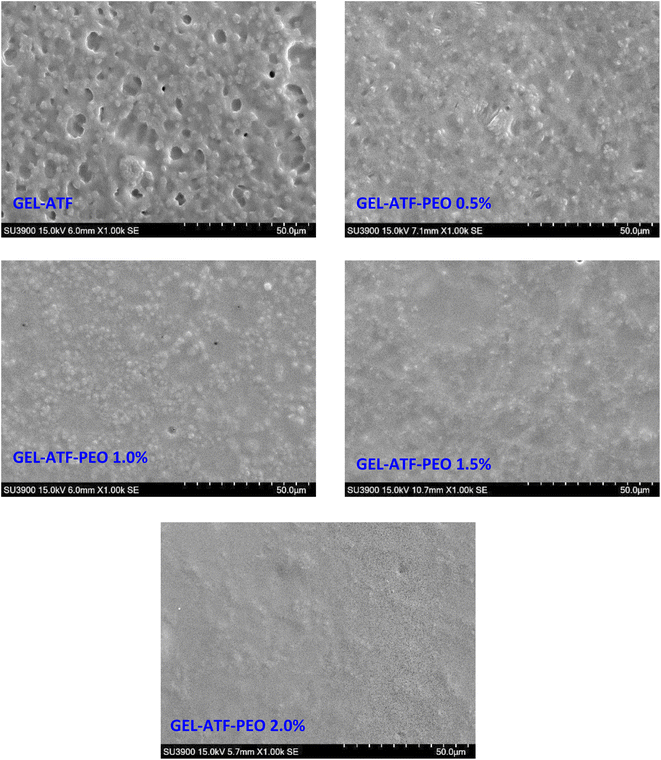 |
| Fig. 7 Differences in microstructural changes of gelatin-arrowroot tuber flour (GEL-ATF)-based edible films with and without added pomelo pericarp essential oil (PEO) at varying concentrations. | |
Antimicrobial properties
The antimicrobial efficacy of GEL-ATF films, both with and without PEO, is presented in Fig. 8. The inhibition zone test was utilized to assess the antimicrobial efficacy of the tested films. Films made of GEL-ATF without PEO exhibited no microbial activity. Generally, biopolymers do not inherently possess antimicrobial properties; however, adding natural additives, particularly EO, can enhance the antimicrobial activity of biopolymer-based edible films.71 The efficacy of EO in controlling microbial growth varied depending on the source, chemical composition, concentration, and the specific bacterial strains targeted.72 Major components such as limonene, α-pinene, thymol, and citral are crucial for their bacteriostatic and bactericidal effects.73 Typically, the mechanism of antimicrobial activity of EOs involves disrupting bacterial cell membranes, inhibiting enzyme activity, and interfering with microbial cell processes. Yumnam et al.27 reported that PEO exhibited strong antimicrobial and antioxidant activities. The primary chemical components, including D-limonene (89–90%), terpinyl acetate (2.8%), α-pinene (2.3%), and terpinolene (0.3%), are involved in inhibiting pathogenic bacteria. In this study, four pathogenic bacteria were tested. The results indicated that the GEL-ATF-based edible films containing PEO exhibited an inhibitory effect solely against Gram-positive bacteria, particularly B. cereus ATCC 1178. Increasing the concentration of PEO in the edible film significantly intensified the inhibition of B. cereus ATCC 1178, as evidenced by the inhibition zones. Specifically, films with 0.5% PEO exhibited a 9.18 mm inhibition zone, while films with PEO concentrations of 1.0%, 1.5%, and 2.0% showed inhibition zones of 9.51 mm, 11.47 mm, and 11.61 mm, respectively. On the other hand, the film samples containing PEO did not inhibit other tested pathogens, even with higher concentrations. In general, the efficacy of EOs in controlling pathogenic bacteria is dose-dependent and varies with different concentrations in controlling microbial activities. Furthermore, the antimicrobial activity of EOs is also influenced by the edible films in which they are encapsulated; these films act as carriers, allowing the EOs to diffuse into the food system and facilitate the inhibition of microbial growth. However, difficulties in releasing the loaded EOs onto the food surface could adversely affect the antimicrobial properties of the EOs. This may explain the lack of an inhibitory effect on the tested pathogenic bacteria in this study. Venkatachalam and Charoenphun45 reported that the antimicrobial activity of PEO in rice flour-based edible films was dose-dependent, and increased concentrations of PEO (>2%) had significant control against Staphylococcus aureus, Listeria monocytogenes, Escherichia coli, and Salmonella Typhimurium. This suggests that the concentration of PEO used in this study was insufficient to inhibit the tested pathogens, and the film matrix did not adequately diffuse the encapsulated PEO into the medium to control the pathogens.
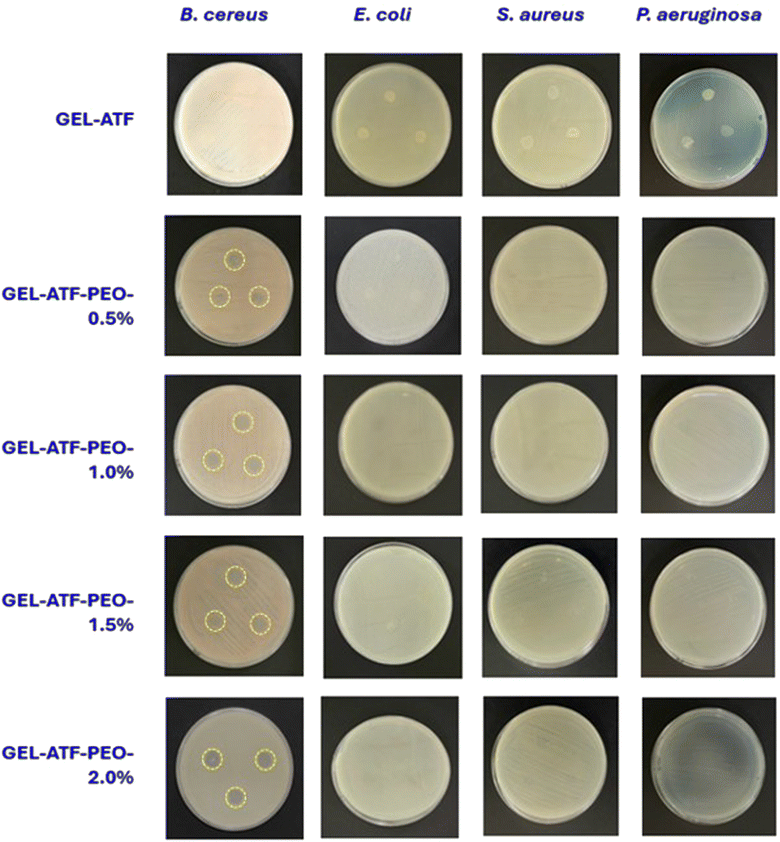 |
| Fig. 8 Differences in antimicrobial activity against selected pathogens by gelatin-arrowroot tuber flour (GEL-ATF)-based edible films with and without added pomelo pericarp essential oil (PEO) at varying concentrations. | |
Conclusion
The present investigation comprehensively examined the influence of pomelo pericarp essential oil on the physical, chemical, and mechanical properties of gelatin-arrowroot tuber flour-based edible films. Incorporating pomelo pericarp essential oil reduced the color values, especially at elevated concentrations; however, the films still maintained a similar appearance among each other and slightly increased opacity despite the increased level of pomelo pericarp essential oil inclusion in the film compositions. The study also illustrated that adding pomelo pericarp essential oil significantly decreased the films' moisture content and water vapor permeability. The diminished solubility of the films with escalating levels of pomelo pericarp essential oil suggested an enhancement in water resistance. Increasing pomelo pericarp essential oil concentrations reduced the tensile strength of the films, while the elongation at break showed an increment with escalating pomelo pericarp essential oil concentration. The addition of pomelo pericarp essential oil had a minimal effect on the thermal stability of the films, showing a slight rise in thermal degradation at higher pomelo pericarp essential oil concentrations. X-ray diffractometry and Fourier transform infrared spectroscopy results demonstrated that elevated pomelo pericarp essential oil concentrations in the film formulation induced changes in crystallinity and molecular interactions. Furthermore, scanning electron microscope observation validated that incorporating pomelo pericarp essential oil resulted in a more polished film surface, suggesting a more consistent and homogeneous matrix. The GEL-ATF-PEO-based film exhibited a strong inhibitory effect against Bacillus cereus ATCC 1178 compared with the other tested pathogens. Overall, this study emphasizes that the presence of pomelo pericarp essential oil in GEL-ATF-based edible films significantly enhanced their physical, mechanical, and structural properties, and increased pomelo pericarp essential oil concentration (>1%) notably improved the film characteristics. The findings of this study suggest new possibilities for developing biodegradable and functional food packaging materials. Future research should explore the long-term stability and biodegradability of GEL-ATF-PEO-based films in various environments and their applications in diverse food systems. Additionally, investigating the integration of pomelo pericarp essential oil with other natural compounds could further enhance the films' antimicrobial and functional properties. It is recommended that new researchers in this field are encouraged to explore the synergistic effects of pomelo pericarp essential oil with other natural polymers or active compounds to design advanced edible films with improved performance characteristics.
Data availability
The data supporting the findings of this study are available within the article. Raw data that support the findings of this study are available from the corresponding author, upon reasonable request.
Author contributions
Conceptualization, K. V. and S. L.; methodology, K. V., N. C., P. N., J. P. and S. L.; software, N. C.; validation, K. V., N. C., P. N., J. P. and S. L.; formal analysis and investigation, K. V., J. P. and S. L.; resources, S. L.; data curation, K. V. and S. L.; writing—original draft preparation, K. V., S. L., P. N. and N. C.; writing—review and editing, K. V., N. C., P. N., J. P. and S. L.; visualization and supervision, S. L.; project administration, S. L.; funding acquisition, S. L. All authors have read and agreed to the published version of the manuscript.
Conflicts of interest
There are no conflicts to declare.
Acknowledgements
This research was financially supported by Prince of Songkla University, Surat Thani Campus, 2024. The authors are grateful to Prince of Songkla University, Surat Thani Campus and Burapha University, Chanthaburi Campus, for the resources and support they provided to fulfill this research. In addition, the authors would like to express their gratitude to the Center for Food Innovation and Research Laboratory for providing them with research space and equipment.
References
- B. E. Ştefănescu, C. Socaciu and D. C. Vodnar, Coatings, 2022, 12, 1815 CrossRef.
- W. Courtene-Jones, N. J. Clark and R. C. Thompson, Emerging Top. Life Sci., 2022, 6(4), 435–439 CrossRef.
- T. M. Shivani and M. Sathiavelu, Packag. Technol. Sci., 2023, 36(1), 15–30 CrossRef CAS.
- A. Dirpan, A. F. Ainani and M. Djalal, Polymers, 2023, 15(13), 2781 CrossRef CAS PubMed.
- B. Santoso, T. L. D. Sinaga and G. Priyanto, Food Sci. Technol., 2022, 42, e51020 CrossRef.
- S. Choudhary, K. Sharma, P. K. Mishra, V. Kumar and V. Sharma, Environ. Technol. Innovation, 2023, 29, 103023 CrossRef CAS.
- Y. Feng, H. Xu, Y. Sun, R. Xia, Z. Hou, Y. Li, Y. Wang, S. Pan, Y. Fan, J. Zhu and G. Xin, Packag. Technol. Sci., 2023, 36(8), 629–646 CrossRef.
- J. T. Martins, M. A. Cerqueira, A. I. Bourbon, A. C. Pinheiro and B. W. S. Souza, Food Hydrocolloids, 2012, 29(2), 280–289 CrossRef CAS.
- R. A. Saleha, F. Novitasari, N. P. Andrianita, A. Manab, M. E. Sawitri, R. D. Andriani and P. P. Rahayu, Asian Food Sci. J., 2023, 22(3), 44–60 CrossRef.
- R. G. Puscaselu, A. Lobiuc, I. O. Sirbu and C. Mihai, Gels, 2022, 8(11), 756 CrossRef.
- H. El Kolli and M. El Kolli, Eng. Technol. Appl. Sci. Res., 2021, 11(4), 7489–7494 CrossRef.
- S. Bhatia, A. Al-Harrasi, M. Jawad, Y. A. Shah, M. S. Al-Azri, S. Ullah, M. K. Answer, M. F. Aldawsari, E. Koca and L. Y. Aydemir, Biomimetics, 2023, 8(2), 172 CrossRef CAS.
- A. D. Drozdov and J. deClaville Christiansen, Bioengineering, 2021, 8(6), 73 CrossRef CAS.
- M. S. Ahmad, R. R. Ali, R. A. Majid and Z. Mohamad, Environ. Qual. Manag., 2023, 33(2), 277–284 CrossRef.
- S. S. Roy, S. Min and J. Rhim, J. Compos. Sci., 2023, 7(3), 126 CrossRef CAS.
- K. Wang, W. Wang, R. Ye, J. Xiao, Y. Liu, J. Ding, S. Zhang and A. Liu, J. Sci. Food Agric., 2017, 97(11), 3613–3622 CrossRef CAS PubMed.
- W. Yeddes, A. Chaker and N. Kechaou, J. Food Process. Preserv., 2021, 45(11), e16059 Search PubMed.
- K. Venkatachalam, S. Ieamkheng, P. Noonim and S. Lekjing, Foods, 2023, 12, 3691 CrossRef CAS.
- G. F. Nogueira, B. D. O. Leme, G. R. S. D. Santos, J. V. D. Silva, P. B. Nascimento, C. T. Soares, F. M. Fakhouri and R. A. de Oliveira, Polysaccharides, 2021, 2(2), 373–386 CrossRef CAS.
- F. Matta Fakhouri, G. F. Nogueira, R. A. de Oliveira and J. I. Velasco, Polymers, 2019, 11(10), 1650 CrossRef PubMed.
- W. Y. Weng, Z. Tao, G. M. Liu, W. J. Su, K. Osako, M. Tanaka and M. J. Cao, Packag. Technol. Sci., 2014, 27(1), 37–47 CrossRef CAS.
- J. Kadzińska, M. Janowicz, S. Kalisz, J. Bryś and A. Lenart, Packag. Technol. Sci., 2019, 32(10), 483–495 CrossRef.
- X. Song, L. Cheng and L. Tan, Food Sci. Technol., 2019, 39(4), 971–979 CrossRef.
- V. Oriani, G. Molina, M. Chiumarelli, G. Pastore and M. Hubinger, J. Food Sci., 2014, 79(2), E189–E194 CrossRef CAS PubMed.
- P. K. Binsi, C. N. Ravishankar and T. K. Srinivasa Gopal, J. Food Sci., 2013, 78(4), E526–E534 CrossRef CAS.
- M. A. Rojas-Graü, R. J. Avena-Bustillos, M. Friedman, P. R. Henika, O. Martín-Belloso and T. H. McHugh, J. Agric. Food Chem., 2006, 54(24), 9262–9267 CrossRef.
- M. Yumnam, R. P. Marak, K. A. Gupta, A. M. Rather and P. Mishra, J. Agric. Food Res., 2023, 11, 100472 CAS.
- R. Tocmo, J. T. Peña-Fronteras, K. F. Calumba, M. B. Mendoza and J. J. Johnson, Compr. Rev. Food Sci. Food Saf., 2020, 19(4), 1969–2012 CrossRef CAS PubMed.
- L. Huyen, T. N. H. On, T. T. Y. Nhi, D. T. Phat and M. H. Cang, Pol. J. Chem. Technol., 2021, 23(4), 17–25 CrossRef CAS.
- C. P. Y. Loo and N. M. Sarbon, Food Biosci., 2020, 35, 100589 CrossRef CAS.
- W. Zhao, L. Yu, Y. Gu, W. Ma, K. Wang, J. Liang and Q. Liu, Food Biosci., 2023, 56, 103279 CrossRef CAS.
- D. R. Lide, CRC Handbook of Chemistry and Physics, CRC Press, Washington, D.C, 2004, vol. 85 Search PubMed.
- K. Venkatachalam, N. Rakkapao and S. Lekjing, Membranes, 2023, 13(2), 161 CrossRef CAS.
- J. Sutharsan, C. A. Boyer and J. Zhao, Carbohydr. Polym. Technol. Appl., 2022, 4, 100232 CAS.
- Q. Cao, Y. Zhang, W. Chen, X. Meng and B. Liu, Int. J. Biol. Macromol., 2018, 106, 1307–1313 CrossRef CAS.
- G. A. Al-Hashimi, B. A. Ammar, G. Lakshmanan, F. Caccilola and N. Lakhssassi, Foods, 2020, 9(2), 184 CrossRef PubMed.
- H. Wang, M. A. A. A. Gani and C. Liu, SAGE Open, 2023, 13(2), 215824402311671 CrossRef.
- S. Phomrak and M. Phisalaphong, J. Nanomater., 2017, 2017, 4739793 Search PubMed.
- K. Choo, M. Lin and A. Mustapha, Food Biosci., 2021, 43, 101287 CrossRef CAS.
- S. Bhatia, A. Al-Harrasi, Y. A. Shah, H. W. K. Altoubi, P. Sharma, M. K. Anwer, D. S. Kaithavalappil, E. Koca and L. Y. Aydemir, Gels, 2023, 9(4), 337 CrossRef CAS PubMed.
- X. Li, Z. C. Tu, X. M. Sha, Y. H. Ye and Z. Y. Li, Food Sci. Nutr., 2020, 8(7), 3099–3109 CrossRef CAS.
- A. Z. Nazreen, J. Jai, S. A. Ali and N. Mohamed Manshor, Sci. Res. J., 2020, 17(2), 222–245 Search PubMed.
- J. A. M. Freitas, G. M. N. Mendonça, L. B. Santos, J. D. Alonso, J. F. Mendes, H. S. Barud and H. M. C. Azeredo, Foods, 2022, 11(15), 2336 CrossRef CAS PubMed.
- B. Girgin and N. Tuğrul, Period. Polytech., Chem. Eng., 2023, 67(2), 193–204 CrossRef CAS.
- K. Venkatachalam and N. Charoenphun, Membranes, 2023, 13(4), 435 CrossRef CAS.
- S. Bhatia, A. Al-Harrasi, S. Ullah, S. M. Al-Azri, E. A. Ahmed Bekhit, L. Karam, M. Albratty, F. M. Aldawsari and K. M. Anwar, Foods, 2023, 12(2), 364 CrossRef CAS.
- V. Siracusa, Int. J. Polym. Sci., 2012, 2012, 302029 Search PubMed.
- W. Xue, J. Zhu, P. Sun, F. Yang, H. Wu and W. Li, Trends Food Sci. Technol., 2023, 136, 295–307 CrossRef CAS.
- A. Acevedo-Fani, L. Salvia-Trujillo, M. A. Rojas-Graü and O. Martin-Belloso, Food Hydrocolloids, 2015, 47, 168–177 CrossRef CAS.
- C. A. López, A. H. de Vries and S. J. Marrink, Carbohydr. Res., 2012, 364, 1–7 CrossRef.
- M. Ghasemlou, N. Aliheidari, R. Fahmi, S. Shojaee-Aliabadi, B. Keshavarz, M. J. Cran and R. Khaksar, Carbohydr. Polym., 2013, 98(1), 1117–1126 CrossRef CAS.
- A. F. R. Leiva, J. Hernández-Fernández and R. Ortega-Toro, Polymers, 2022, 14(23), 5077 CrossRef.
- M. L. Sanyang, S. M. Sapuan, M. Jawaid, M. R. Ishak and J. Sahari, J. Food Sci. Technol., 2015, 53(1), 326–336 CrossRef.
- C. Li, J. Pei, X. Xiong and F. Xue, Coatings, 2020, 10, 784 CrossRef CAS.
- A. Szram, J. Sokołowski, J. Nowak, M. Domarecka and M. Łukomska-Szymańska, Inż. Mater., 2017, 2(216), 103–107 Search PubMed.
- S. Ebrahimzadeh, D. Biswas, S. S. Roy and J. D. McClements, Trends Food Sci. Technol., 2023, 135, 43–56 CrossRef CAS.
- K. Patnode, B. Rasulev and A. Voronov, ACS Appl. Mater. Interfaces, 2022, 14(6), 8384–8393 CrossRef CAS.
- D. Šuput, V. Lazić, L. Pezo, S. Markov, Ž. Vaštag, L. Popović, A. Radulović, S. Ostojić, S. Zlatanović and S. Popović, Pol. J. Food Nutr. Sci., 2016, 66(4), 277–285 CrossRef.
- R. Aguilar-Sánchez, R. Munguía-Pérez, F. Reyes-Jurado, A. Navarro-Cruz, T. Cid-Pérez, P. Hernández-Carranza, S. D. C. Beristain-Bauza, C. E. Ochoa-Velasco and R. Avila-Sosa, Molecules, 2019, 24(12), 2340 CrossRef PubMed.
- L. A. Charles, N. Motsa and A. A. Abdillah, Polymers, 2022, 14(17), 3462 CrossRef.
- A. I. Channa, J. Ashfaq, A. M. Siddiqui, D. A. Chandio, A. M. Shar and A. Alhazaa, Polymers, 2022, 14(22), 5020 CrossRef PubMed.
- E. A. Soliman and M. Furuta, Food Nutr. Sci., 2014, 5(11), 1040–1055 Search PubMed.
- N. Singh, P. Belton and D. M. R. Georget, Int. J. Biol. Macromol., 2009, 45(2), 116–119 CrossRef CAS PubMed.
- T. Hongbo, Z. Qian, L. Yanping and L. Xiaojun, Polym. Bull., 2022, 79, 9485–9501 CrossRef.
- S. Agatonovic-Kustrin, P. Ristivojevic, V. Gegechkori, T. M. Litvinova and D. W. Morton, Appl. Sci., 2020, 10, 7294 CrossRef CAS.
- A. C. S. Valderrama and G. C. R. De, Am. J. Anal. Chem., 2017, 8, 726–741 CrossRef CAS.
- R. Santhosh, J. Ahmed, R. Thakur and P. Sarkar, Sustainable Food Technol., 2024, 2, 307–330 RSC.
- H. Firoozmand and D. Rousseau, Food Hydrocolloids, 2015, 50, 84–93 CrossRef CAS.
- P. Wongphan and N. Harnkarnsujarit, Int. J. Biol. Macromol., 2020, 156, 80–93 CrossRef CAS PubMed.
- M. Elma, E. A. Pradana, N. Sihombing, R. N. Thala'ah, A. Rahma and E. L. A. Rampun, IOP Conf. Ser.: Earth Environ. Sci., 2022, 999(1), 012024 Search PubMed.
- X. Li, Z. C. Tu, X. M. Sha, Y. H. Ye and Z. Y. Li, J. Food Sci. Technol., 2022, 59, 815–824 CrossRef CAS PubMed.
- S. Garzoli, Plants, 2023, 12(4), 800 CrossRef PubMed.
- M. Galgano, P. Capozza, F. Pellegrini, M. Cordisco, A. Sposato, S. Sblano, M. Camero, G. Lanave, G. Fracchiolla, M. Corrente, F. Cirone, A. Trotta, M. Tempesta, D. Buonavoglia and A. Pratelli, Antibiotics, 2022, 11(7), 979 CrossRef CAS PubMed.
|
This journal is © The Royal Society of Chemistry 2024 |
Click here to see how this site uses Cookies. View our privacy policy here.