DOI:
10.1039/D4RA03292F
(Paper)
RSC Adv., 2024,
14, 22981-22987
Synthesis of novel fatty acid 3,4-dihydropyrimidin-2-(1H)-one and antitumoral activity against breast and gastric cancer cells†
Received
4th May 2024
, Accepted 13th July 2024
First published on 22nd July 2024
Abstract
Monastrol is the best-known small compound from the dihydropyrimidinones/thiones (DHPMs) heterocycle family, a cell-permeable molecule recognized as an inhibitor of mitotic kinesin Eg5, that is over-expressed in tumor cells and is a very promising target for the development of new drugs for cancer. The lipophilic properties of the DHPMs have been demonstrated to be of pivotal importance in the design of new molecules. This work describes the synthesis and antitumoral activity of novel C5-substituted fatty-DHPMs against breast and gastric cancer cell lines. The compounds were synthesized via Biginelli multicomponent reaction from oleyl β-ketoester in good yields (40–72%) using a simple approach catalyzed by nontoxic and free-metal sulfamic acid. Among the compounds tested, the compound 10c, derived from 3-hydroxybenzaldehyde and urea, exhibited 77% cellular viability to normal cells (C2C12) and was selected to be evaluated against tumoral breast (MCF-7) and gastric (AGS) cell lines. The results obtained afforded an IC50 of breast cancer cells of 2.3 μM, qualifying the molecule as the most potent, and making it a promising compound for future experiments in vivo.
Introduction
Breast cancer continues to become one of the most pervasive and life-threatening diseases in women, increasing the global cancer burden.1 As per statistics of GLOBOCAN 2022, currently, breast cancer has become the second most incident cancer type in the world, and the fourth in mortality index.2 The projections to 2045 foresee an increase of 36.9% in incidence and 56.8% in mortality related to breast cancer in the world. In females, the spread of breast cancer is by invasive-regional-distant metastasis, in which widespread metastasis is the principal reason for mortality.
The human kinesin-5, also known as Eg5 protein, is a member of the great kinesin family of proteins, that execute functions like mitosis, meiosis, microtubule dynamics, and cell division.3 Kinesins are over-expressed in tumors, breast, lung, pancreatic, ovarian, and bladder cancers with bipolar spindle formation,4 and are traceable in non proliferative tissue. Therefore, they are a promising target for developing new cancer-related drugs. Monastrol (1) is the best-known compound from the dihydropyrimidinones/thiones (DHPMs) heterocycle family of compounds, named Biginelli's compounds after the Biginelli Multicomponent Reaction (Biginelli MCR), developed in 1893.5 This molecular scaffold is associated with several biological activities, like anti-inflammatory, antihypertensive, antibacterial, antifungal, antiviral, antitubercular, antiparasitic, antithyroid, antimuscarinic, antidiabetic, calcium channel modulators, and anti-cancer. Given this large field of activities, DHPMs are considered a paramount drug target in medicinal chemistry.6–10 The first work that described the effect of monastrol as an antitumoral compound was reported in 1999.11 The authors reported the ability of monastrol to interrupt mitosis by inhibiting the motor activity of the kinesin Eg5. Since then, monastrol has been used as an inspiration for the design of new anticancer agents against breast, kidney, colon, melanoma, and ovarian cancer cells.12
The lipophilic properties of the DHPMs have been demonstrated to be of pivotal importance in the design of new molecules. Heterocyclic-fatty acid hybrid derivatives are a new class of heterocyclic compounds with a broad range of biological activities and significance in medicinal chemistry.13 Fatty acids derived from oils and fats of different plant and animal resources are considered one of the most valuable renewable precursors of the synthetic chemical and pharmaceutical industries. On the other hand, heterocyclic compounds are well known nowadays for their constitution of many commercialized drugs due to their unique biological activities. The combination of fatty acids and heterocyclic compounds has made important advances in the construction of valuable biologically relevant molecules in the pharmaceutical industry. Over the last few years, many studies have emphasized the significance of such derivatives.14
Antiproliferative activity on two glioma cell lines (C6 rat and U-138 MG human) of C-5 substituted DHPM-fatty acids, synthesized from Biginelli's MCR, was investigated by Treptow and co-workers.15 The fatty acid hybrid oxo-monastrol-palmitic acid 2 was the most potent, reducing U-138 MG human cell viability by ca. 50% at 10 μM. In another study, De Oliveira and co-workers investigated DHPMs C-6 fatty chain substituted against glioma cancer cells (Fig. 1). The results showed remarkable cytotoxicity with more than twelvefold higher potency as compared to positive control monastrol when screened against rat glioma (C6) cells.16 Structural activity relationship (SAR) studies concluded that increasing lipophilicity at the C-6 position enhances the Eg5 inhibition due to fatty acid binding proteins (FABP), which play a vital role in increasing the transport of molecules through biological barriers.17
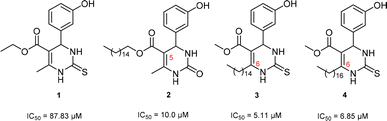 |
| Fig. 1 Monastrol (1) and fatty acid derivatives C5 and C6 substituted studied (2–4) against glioma cell lines. | |
Based on our previous results about the antitumoral activity against glioma cell lines of heterocyclic-fatty acid derivatives,15,17 and in the reported relationship of DHPM heterocycle core in the human kinesin-5 (Eg5), overexpressed in breast cancer, like glioma, this work investigated the antitumoral activity of novel monastrol and oxo-monastrol oleyl chain C5-substituted, against breast and gastric cancer cell lines. The compounds were synthesized by Biginelli MCR reaction, using a simple approach, catalyzed by nontoxic and free-metal sulfamic acid, employing β-ketoester containing oleyl (C18:1) fatty chain, combined to several aromatic aldehydes, urea or thiourea.16
Results and discussion
In previous works of our group, we described the synthesis of fatty-DHPMs C5-substituted by Biginelli MCR, from fatty β-ketoesters and benzaldehyde, 3-hydroxybenzaldehyde, and 4 N,N-dimethylaminobenzaldehyde (Scheme 1(a)). After, their antitumoral activities against glioma C6 rat and U-138 MG human cells were investigated.15 The DHPMs C5-substituted derived from 3-hydroxybenzaldehyde, palmityl and oleyl chains, urea, and thiourea, reduced C6 cell viability by ca. 50% at 10 μM. In addition, the compounds showed a large safety range for neural cells demonstrating non-toxicity to organotypic hippocampal culture. Following this, the fatty DHPMs were also tested in human glioma cells (U-138 M). All compounds reduced cell viability, as in C6 rat cells. The DHPM-fatty acid derived from palmityl and oleyl chains reduced cell viability the most at 50 mM (ca. 70 e 80%). Compound hybrid oxo-monastrol-palmitic acid was the most potent, reducing cell viability by ca. 50% at 10 μM.
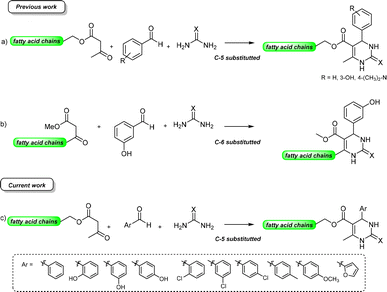 |
| Scheme 1 Previous and current work. (a) Synthesis of fatty acid 5-substituted DHPM from benzaldehyde, 3-hydroxibenzaldehyde, and 4 N,N-dimethylamino benzaldehyde; (b) synthesis of fatty acid DHPMs analogues to monastrol; (c) synthesis of new fatty acid 5-substituted DHPM and study of antitumoral activity against breast cancer. | |
In addition, a series of novel analogs monastrol and oxo-monastrol fatty alkyl C6-substituted were synthesized and their in vitro antitumoral activities were investigated against rat glioblastoma cells (Scheme 1(b)).16 In this work, the effect of the insertion of several fatty chains at the C6 position in the monastrol ring was explored. The results indicated that the compounds derived from palmityl (C16:0) and stearyl (C18:0) chains were the most potent molecules with IC50 values of 5.11 and 6.85 μM, respectively, making them 13 times more potent than monastrol. Besides this, the molecules were also 2.5 times more potent than analog C5-substituted used as a fatty positive control. These results indicated that the hydroxyl group in the aromatic ring and the presence of a long fatty chain are essential for the in vitro antitumoral activities against glioma cell lines.
Considering these previous results in the design of new heterocyclic-fatty chains and the importance that structural variations can have in biological studies, in the present work we explore a large range of aromatic rings in the Biginelli MCR to synthesize C5-substituted new DHPMs (Scheme 1(c)). Here, the oleyl β-ketoester was employed as a 1,3-dicarbonylic component, combined with urea or thiourea which are now being investigated in breast and gastric cancer antitumoral activities.
Initially, the β-ketoesters 5 was prepared by transesterification of methyl acetoacetate with oleyl alcohol (cis-9-octadecen-1-ol, cis-C18:1), with a yield of 80%, following the literature.18
The 1,3-dicarbonylic compound 5 was combined with aromatic aldehydes 6a–j, urea (8), or thiourea (9), in the presence of sulfamic acid as catalyst (20 mol%), methanol as solvent, and reflux for 24 h (Scheme 2).
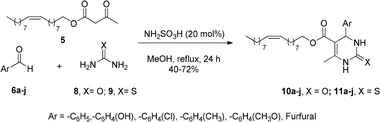 |
| Scheme 2 Synthesis of DHPM 10a–j, 11a–j by Biginelli MCR. | |
The compounds were obtained in good yields (40–72%) from multicomponent Biginelli's reaction (Fig. 2), using sulfamic acid (H2NSO3H, SA) as a green catalyst. Sulfamic acid has been used as a substitute for conventional Brønsted–Lowry and Lewis acid catalysts. It is a relatively stable white crystalline solid, odorless, non-volatile, non-hygroscopic, non-corrosive, and cheap, that is a highly efficient green catalyst for organic reactions.19 The zwitterionic characteristic of SA makes it immiscible with commonly employed non-polar organic solvents, with excellent activity over a vast array of acid-catalyzed organic transformations, including Biginelli's reaction.20
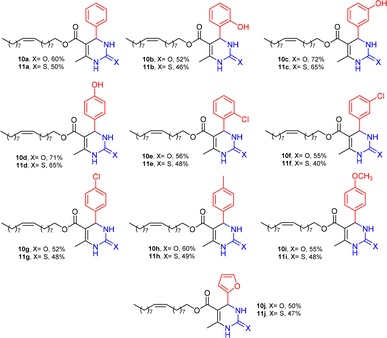 |
| Fig. 2 Fatty chain 5-substituted DHPMs from Biginelli's MCR. | |
The structural diversity of DHPMs and yields are shown in Fig. 2. Twenty compounds were synthesized, of which sixteen are reported for the first time in the literature. All the structures were confirmed by spectroscopic and spectrometric methods (See ESI†).
The fatty 5-substituted DHPM 10a–j, 11a–j were submitted to studies about the antitumoral activity in vitro, against AGS and MCF-7, corresponding to gastric and breast cancer cell lines, respectively. Initially, MTT assays were used to determine the effect of each DHPM on the cell viability of normal myoblast cells (C2C12). The experiments were performed in triplicate (Fig. 3).
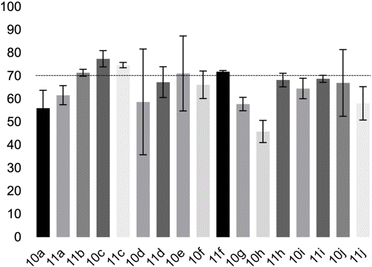 |
| Fig. 3 MTT results of DHPMs (10 μM) in the viability (%) of myoblast cells (C2C12). | |
The compounds 10–11c, 11b, 11f, 11h, 11i showed viability near 70% in this cell type. These compounds were selected for testing with tumoral cell lines AGS (gastric) and MCF-7 (breast). The results are depicted in Table 1. Cells were plated at a density of 5000 cells per mL in 96 well plates. One day after seeding cells were treated with a medium containing the compounds at concentrations ranging from 0 up to 1000 μM for 2 days and finally the MTT reduction assay was carried out.21 Untreated cells were used as controls. Monastrol was used as a reference compound. Normal human myoblasts were used to assess the selectivity of the compounds against cancer cells. The results are expressed as IC50 (μM).
Table 1 IC50 values against myoblast cells (C2C12) and cancer cells (AGS and MCF-7), upon application of DHPM 10c–11b, 11c, 11f, 11h, 11i
Entry |
Compound |
IC50 (μM) |
C2C12 |
AGS |
MCF-7 |
1 |
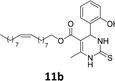 |
88.5 ± 6.4 |
20.2 ± 2.1 |
10.1 ± 1.3 |
2 |
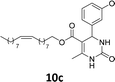 |
18.4 ± 10.5 |
12.1 ± 0.4 |
2.3 ± 0.4 |
3 |
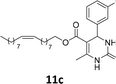 |
446.4 ± 84.2 |
125.4 ± 6.2 |
28.4 ± 7.0 |
4 |
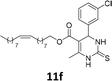 |
307.0 ± 143.3 |
146.2 ± 25.9 |
8.8 ± 8.3 |
5 |
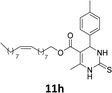 |
362.0 ± 12.6 |
126.2 ± 4.6 |
39.2 ± 4.9 |
6 |
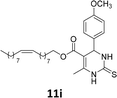 |
166.4 ± 8.9 |
101.8 ± 0.6 |
16.4 ± 2.7 |
7 |
 |
35.5 ± 6.4 |
40.0 ± 6.2 |
25.6 ± 7.3 |
When evaluating the compounds in cancer cells, it can be observed the antitumoral efficacy of the compounds on cancer cells, while in myoblast cells, their cytotoxic effect is achieved at much higher concentrations. The DHPM compounds are described in the literature as selective to Kinesin Eg5, a motor protein overexpressed in this type of cancer cell line.
In this sense, the compounds 11b and 10c (Table 1, entries 1–2, respectively) derived from hydroxybenzaldehyde showed a significant cytotoxic effect against the tumoral cells, compared to Monastrol (Table 1, entry 7). The compound 11b, derived from 2-hydroxibenzaldehyde and thiourea, showed IC50 values for breast cancer (MCF-7) and gastric cancer cell lines (AGS) of 10.1 ± 1.3 μM and 20.2 ± 2.1 μM, respectively, keeping the viability of normal cells at approximately 70% (Fig. 3). On the other hand, compound 11c, derived from 3-hydroxibenzaldehyde and thiourea (Table 1, entry 3), presented IC50 to MCF-7 and AGS of 28.4 ± 7.0 μM and 125.4 ± 6.2 μM, respectively. Finally, compound 10c, which differs from 11c only from urea used in its synthesis, resulting in the presence of sulfur in the DHPM core, showed significant differences in the IC50 values observed (IC50 = 2.3 ± 0.4 μM for breast, 12.1 ± 0.4 μM for gastric, and 77% of viability of normal cells). Other compounds 11f and 11i showed an IC50 of 8.8 ± 8.3 μM and 16.4 ± 2.7 μM for breast cancer cell (MCF-7), respectively.
Conclusions
In conclusion, this work described the synthesis of novel C-5-substituted DHPMs-fatty acids, in good yields (40–72%) from the multicomponent Biginelli reaction, including monastrol and oxo-monastrol analogs. The in vitro antitumoral activities of the compounds were investigated against AGS and MCF-7, corresponding to gastric and breast cancer cell lines, respectively. Besides, the cellular viability with normal (C2C12) was investigated, using MTT assay. Among the compounds tested, the DHPM derived from hydroxybenzaldehyde showed cellular viabilities superior to 70%, both with oxo or thio DHPM-fatty acids. The compound 10c, derived from 3-hydroxybenzaldehyde and urea, exhibited 77% cellular viability to normal cells (C2C12) and was selected to be evaluated against tumoral breast (MCF-7) and gastric (AGS) cell lines. Despite the antitumoral activity, monastrol (1) is recognized as an antimitotic agent, with action in Kinesin Eg5, a motor protein overexpressed in cancer cell lines. The results obtained with the insertion of lipophilic alkyl chains, derived from vegetable oils, as an oleyl fatty acid chain, demonstrated a substantial improvement of the biological activity in vitro for the new fatty acid DHPM derivatives. The compounds 11b, 10c, 11f and 11i showed IC50 values in the range of 2.3–16.4 μM for the breast cancer cells, compared to 25.6 μM obtained with monastrol. In addition, the results with the gastric cancer cell, the compounds 11b and 10c were the most active, with IC50 values 20.2 ± 2.1 μM and 12.1 ± 0.4 μM, respectively, in comparison to 40.0 ± 6.2 μM observed in Monastrol. The compound 10c, derived from 3-hydroxybenzaldehyde and urea, afforded an IC50 against breast cancer cells of 2.3 ± 0.4 μM, qualifying the molecule 10c as the most potent, and making it a promising compound for future experiments in vivo.
Experimental
General
All commercially available reagents were used without further purification. Sulfamic acid (98%), urea, and thiourea were purchased from Sigma-Aldrich Chemical. The solvents employed in extraction and purification (hexane and ethyl acetate) were distilled before use. The reactions were monitored by TLC (thin-layer chromatography) using pre-coated silica gel 60 F254 plates. The visualization was accomplished with UV light, or stained with iodine. Flash chromatography was performed using Merck silica gel with a pore size of 60 Å, 230–400 mesh, with the indicated eluent system. The NMR spectra (1H and 13C) were obtained on a Bruker Nuclear Ascend 400 spectrometer. The spectra were obtained either in CDCl3 or in DMSO-d6 solution. The chemical shifts (δ) are reported in ppm and referenced to tetramethylsilane (TMS) as the internal standard. The coupling constants (J) are reported in hertz (Hz). Hydrogen coupling patterns are described as singlet (s), doublet (d), doublet of doublets (dd), doublet of doublet of doublets (ddd), doublet of triplets (dt), triplet (t), triplet of doublets (td), doublet of quartets (dq), quartet (q), quintet (quint), sextet (sext), broad signal (br), and multiplet (m). The melting points were obtained on an MP90 melting point system.
Cell lines
A C2C12 (myoblast; CRL-1772, ATCC, Gaithersburg, MD, USA) cell line was used, while AGS (gastric adenocarcinoma; CRL-1739, ATCC) and MCF-7 (mammary carcinoma; HTB-22, ATCC) were used as cancer cell lines. C2C12 and MCF7 cells were cultured in DMEM (Dulbecco's Modified Eagle Medium) medium (HyClone, Thermo Scientific USA); MCF-7’s medium was supplemented with human insulin (10 μg mL−1; Gibco, Thermo Scientific, USA) and AGS cells were cultured in RPMI-1640 medium (HyClone, Thermo Scientific), both medium were supplemented with 10% fetal bovine serum (FBS) (Hyclone™, GE Healthcare Life Science), 0.25 μg mL−1 amphotericin B (Hyclone™, GE Healthcare Life Science), and 25 μg mL−1 gentamicin (Millipore). Cells were maintained in an incubator at 37 °C, 5% CO2.
Cell viability (MTT) and antiproliferative activity assays
5000 cells per well were seeded in a 96-well plate (SPL Life Sciences, Korea) for 12 hours, and each DHPM compound was applied at 10 μM as a treatment for 48 hours. The cells were washed with 100 μl of 1X PBS for 3 minutes, and then 100 μl of 0.5 mg mL−1 MTT/PBS 1X solution was added. The plate was then incubated for 90 minutes at 37 °C in an incubator. After discarding the MTT solution, 100 μl DMSO was loaded per well and kept stirring for 30 minutes. The plate was agitated for 30 min in darkness, and absorbance was read at 570 nm using a spectrophotometer. For half maximal inhibitory concentration (IC50) the same previous procedure was followed, but the following concentrations of all the compounds were measured; 1000 μM, 100 μM, 10 μM, 1 μM and 0.1 μM. Data were saved in an Excel template (Microsoft®, Version 2108) and analyses were performed in GraphPad Prism 8.0.1 (Graphpad Software, San Diego, CA).
General procedure for the synthesis of DHPMs 10a–j, 11a–j
Oleic acetoacetate 5 (1 mmol), aromatic aldehyde 6a–j (1 mmol), urea 8 (1 mmol) or thiourea 9 (1 mmol), and sulfamic acid (0.2 mmol) were charged in a round bottom flask with MeOH (5 mL) and stirred refluxed for 24 h. Then, the methanol was removed under reduced pressure and the crude was purified by column chromatography, using mixtures of hexane and ethyl acetate as eluent.
(Z)-Octadec-9-enyl 6-methyl-4-phenyl-2-oxo-1,2,3,4-tetrahydropyrimidine-5-carboxylate (10a)15. MM = 482.35 g mol−1; white solid; M. P.: 81–83 °C; Yield: 60%; 1H NMR (400 MHz, DMSO-d6) δ (ppm): 9.18 (s, 1H), 7.71 (s, 1H), 7.33–7.21 (m, 5H), 5.34–5.32 (m, 2H), 5.13 (d, 1H, 3J = 3.3 Hz), 3.97–3.87 (m, 2H), 2.26 (s, 3H), 1.98–1.95 (m, 4H), 1.44 (quint, 2H, 3J = 7.0 Hz), 1.28–1.23 (m, 22H), 0.85 (t, 3H, 3J = 6.6 Hz); 13C NMR (100 MHz, DMSO-d6) δ (ppm): 165.8, 152.5, 149.0, 145.2, 130.1, 128.8, 127.7, 126.7, 99.5, 63.6, 54.4, 31.7, 29.5, 29.3, 29.1, 29.0, 28.9, 28.6, 27.0, 25.8, 22.5, 18.2, 14.4; IR (max, cm−1): 3243; 3114; 2919; 2815; 1708; 1652; 1469; 1229; 1096; 798.
(Z)-Octadec-9-enyl 6-methyl-4-phenyl-2-thioxo-1,2,3,4-tetrahydropyrimidine-5-carboxylate (11a)15. MM = 498.86 g mol−1; yellow oil; yield: 55%; 1H NMR (400 MHz, DMSO-d6) δ (ppm): 10.32 (s, 1H), 9.63 (s, 1H), 7.33–7.20 (m, 5H), 5.34–5.31 (m, 2H), 5.16 (d, 1H, 3J = 3.6 Hz), 3.99–3.89 (m, 2H), 2.30 (s, 3H), 1.97 (m, 4H), 1.44 (quint, 2H, 3J = 7.0 Hz), 1.23–1.15 (m, 22H), 0.85 (t, 3H, 3J = 7.01 Hz); 13C NMR (100 MHz, DMSO-d6) δ (ppm): 174.5, 165.6, 145.7, 143.8, 130.1, 128.9, 128.1, 126.8, 100.9, 63.9, 54.5, 31.7, 29.5, 29.3, 29.1, 29.0, 28.9, 27.0, 25.8, 22.5, 17.6, 14.4; IR (max, cm−1): 3169; 2925; 2851; 1712; 1652; 1597; 1467; 1318; 1190; 1096; 759.
(Z)-Octadec-9-enyl 4-(2-hydroxyphenyl)-6-methyl-2-oxo-1,2,3,4-tetrahydropyrimidine-5-carboxylate (10b). MM = 498.35 g mol−1; white solid; M. P.: 102–105 °C; yield: 52%; 1H NMR (400 MHz,DMSO-d6) δ (ppm): 7.58 (s, 1H), 7.23–7.20 (m, 1H), 7.18 (m, 1H), 7.17 (m, 1H), 6.90 (ddd, 3J = 7.48 Hz; 3J = 7.31 Hz; 4J = 1.0 Hz, 1H), 6.78–7.76 (m, 1H), 5.36–5.32 (m, 2H), 4.45 (dd, 3J = 4.7 Hz; 4J = 3.0 Hz, 1H), 4.15–4.05 (m, 2H), 1.99–1.94 (m, 4H), 1.73 (s, 3H), 1.60 (quint, 2H), 1.27–1.23 (m, 22H) 0.85 (t, 3H, 3J = 6.8 Hz); 13C NMR (100 MHz, DMSO-d6) δ (ppm): 168.9, 154.9, 151.1, 130.5, 130.1, 129.7, 129.1, 125.9, 120.9, 117.0, 83.5, 64.9, 48.2, 44.4, 32.4, 31.7, 29.5, 29.3, 29.1, 29.0, 28.9, 28.5, 25.7, 24.4, 22.5, 14.4; IR (max, cm−1): 3235; 2921; 2853; 1714; 1687; 1652; 1465; 1229; 1079; 780.
(Z)-Octadec-9-enyl 4-(2-hydroxyphenyl)-6-methyl-2-thioxo-1,2,3,4-tetrahydropyrimidine-5-carboxylate (11b). MM = 514.36 g mol−1; yellow oil; yield: 46%; 1H NMR (400 MHz, DMSO-d6) δ (ppm): 9.16 (s, 1H), 7.22–7.17 (m, 2H), 6.97–6.94 (m, 1H), 6.83 (d, 3J = 7.9 Hz, 1H), 5.35–5.30 (m, 1H), 4.57–4.55 (m, 1H), 4.13–4.07 (m, 2H), 1.99–1.91 (m, 4H), 1.78 (s, 3H), 1.60–1.57 (m, 2H), 1.28–1.24 (m, 22H) 0.85 (t, 3H, 3J = 5.9 Hz); 13C NMR (100 MHz, DMSO-d6) δ (ppm): 176.9, 168.3, 150.9, 130.5, 130.1, 129.1, 124.2, 121.2, 116.8, 81.7, 65.1, 48.6, 42.8, 32.4, 31.7, 29.5, 29.3, 29.2, 29.1, 29.0, 28.5, 27.0, 25.7, 23.2, 22.5, 14.4; IR (max, cm−1): 3198; 2925; 2853; 1738; 1560; 1506; 1459; 1246; 1174; 1092; 913; 753.
(Z)-Octadec-9-enyl 4-(3-hydroxyphenyl)-6-methyl-2-oxo-1,2,3,4-tetrahydropyrimidine-5-carboxylate (10c)15. MM = 498.35 g mol−1; white solid; M. P.: 103–106 °C; yield: 71%; 1H NMR (400 MHz, DMSO-d6) δ (ppm): 9.30 (s, 1H), 9.09 (d, 1H, 3J = 1.5 Hz), 7.59 (s, 1H), 7.02–6.99 (m, 2H), 6.68–6.66 (m, 2H), 5.34–5.31 (m, 2H), 5.02 (d, 1H, 3J = 3.2 Hz), 3.97–3.87 (m, 2H), 2.24 (s, 3H), 1.99–1.96 (m, 4H), 1.45 (quint, 2H, 3J = 6.8 Hz), 1.28–1.20 (m, 22H), 0.85 (t, 3H, 3J = 6.7 Hz); 13C NMR (100 MHz, DMSO-d6) δ (ppm): 165.9, 157.0, 152.5, 148.4, 146.6, 135.0, 130.1, 127.8, 115.4, 100.0, 63.5, 53.9, 32.4, 31.7, 29.5, 29.3, 29.2, 29.1, 29.0 (2C), 28.6, 27.0, 25.9, 22.5, 18.1, 14.4; IR (max, cm−1): 3237; 3116; 2923; 2851; 1716; 1685; 1648; 1465; 1229; 1079; 778.
(Z)-Octadec-9-enyl 4-(3-hydroxyphenyl)-6-methyl-2-thioxo-1,2,3,4-tetrahydropyrimidine-5-carboxylate (11c)15. MM = 514.36 g mol−1; yellow solid; M. P.: 169–172 °C; yield: 65%; 1H NMR (400 MHz, DMSO-d6) δ (ppm): 10.31 (s, 1H), 9.62 (s, 1H), 9.44 (s, 1H), 7.14–7.06 (m, 1H), 6.65–6.62 (m, 3H), 5.34–5.30 (m, 1H), 5.07 (d, 3J = 3.6 Hz, 1H), 4.02–3.93 (m, 1H), 2.29 (s, 3H), 1.99–1.96 (m, 4H), 1.49–1.43 (m, 2H), 1.23–1.17 (m, 22H) 0.84 (t, 3H, 3J = 6.3 Hz); 13C RMN (100 MHz, DMSO-d6) δ (ppm): 176.9, 168.3, 150.9, 130.5, 130.1, 129.1, 124.2, 121.2, 116.8, 81.7, 65.1, 48.6, 42.8, 32.4, 31.7, 29.5, 29.3, 29.2, 29.1, 29.0, 28.5, 27.0, 25.7, 23.2, 22.5, 14.4; IR (max, cm−1): 3260; 2925; 2853; 1638; 1576; 1459; 1313; 1192; 1120; 702.
(Z)-Octadec-9-enyl 4-(4-hydroxyphenyl)-6-methyl-2-oxo-1,2,3,4-tetrahydropyrimidine-5-carboxylate (10d). MM = 498.35 g mol−1; white solid; M. P.: 103–106 °C; yield: 71%; 1H NMR (400 MHz, DMSO-d6) δ (ppm): 9.30 (s, 1H), 9.09 (d, 1H, 3J = 1.5 Hz), 7.59 (s, 1H), 7.02–6.99 (m, 2H), 6.68–6.66 (m, 2H), 5.34–5.31 (m, 2H), 5.02 (d, 1H, 3J = 3.2 Hz), 3.97–3.87 (m, 2H), 2.24 (s, 3H), 1.99–1.96 (m, 4H), 1.45 (quint, 2H, 3J = 6.8 Hz), 1.28–1.20 (m, 22H), 0.85 (t, 3H, 3J = 6.7 Hz); 13C NMR (100 MHz, DMSO-d6) δ (ppm): 165.9, 157.0, 152.5, 148.4, 146.6, 135.0, 130.1, 127.8, 115.4, 100.0, 63.5, 53.9, 32.4, 31.7, 29.5, 29.3, 29.2, 29.1, 29.0 (2C), 28.6, 27.0, 25.9, 22.5, 18.1, 14.4; IR (max, cm−1): 3237; 3116; 2923; 2851; 1716; 1685; 1648; 1465; 1229; 1079; 778.
(Z)-Octadec-9-enyl 4-(4-hydroxyphenyl)-6-methyl-2-thioxo-1,2,3,4-tetrahydropyrimidine-5-carboxylate (11d). MM = 514.36 g mol−1; yellow solid; M. P.: 134–136 °C; yield: 65%; 1H NMR (400 MHz, DMSO-d6) δ (ppm): 10.23 (s, 1H), 9.54 (s, 1H), 9.39 (s, 1H), 6.99 (d, 2H, 3J = 8.6 Hz), 6.69 (d, 2H, 3J = 8.5 Hz), 5.34–5.31 (m, 1H), 5.05 (d, 1H, 3J = 3.6 Hz), 1.99–1.96 (m, 4H), 1.47–1.43 (m, 2H), 1.28–1.19(m, 22H) 0.84 (t, 3H, 3J = 6.6 Hz); 13C NMR (100 MHz, DMSO-d6) δ (ppm): 174.2, 165.7, 157.4, 145.2, 134.4, 130.1, 128.1, 115.5, 101.3, 63.9, 54.0, 32.4, 31.7, 29.5, 29.3, 29.2, 29.1, 29.0 (2C), 28.5, 27.0, 25.8, 22.5, 17.5, 14.4; IR (max, cm−1): 3198; 2923; 2853; 1716; 1658; 1580; 1513; 1316; 1201; 1084; 751.
(Z)-Octadec-9-enyl 4-(2-chlorophenyl)-6-methyl-2-oxo-1,2,3,4-tetrahydropyrimidine-5-carboxylate (10e). MM = 516.31 g mol−1. white solid; M. P.: 101–103 °C; yield: 56%; 1H NMR (400 MHz, DMSO-d6) δ (ppm): 9.30 (s, 1H), 7.70 (s, 1H), 7.37–7.25 (m, 3H), 5.62 (d, 1H, 3J = 2.8 Hz), 5.35–5.30 (m, 2H), 3.92–3.76 (m, 2H), 2.31 (s, 3H), 1.96 (m, 4H), 1.24–1.13 (m, 24H), 0.85 (t, 3H, 3J = 6.3 Hz); 13C NMR (100 MHz, DMSO-d6) δ (ppm): 165.4, 151.8, 150.1, 142.0, 132.2, 130.5, 130.1, 129.8, 129.4, 129.0, 128.2, 98.1, 63.5, 51.7, 31.7, 29.5, 29.3, 29.1, 29.0, 28.9, 28.5, 27.0, 25.7, 22.5, 18.1, 14.4; IR (max, cm−1): 3235; 3104; 2923; 2851; 1701; 1650; 1465; 1223; 1092; 817.
(Z)-Octadec-9-enyl 4-(2-chlorophenyl)-6-methyl-2-thioxo-1,2,3,4-tetrahydropyrimidine-5-carboxylate (11e). MM = 532.29 g mol−1; yellow oil; yield: 48%; 1H NMR (400 MHz, DMSO-d6) δ (ppm): 10.40 (s, 1H), 9.61 (s, 1H), 7.38–7.29 (m, 3H), 5.64 (d, 1H, 3J = 2.8 Hz), 5.35–5.30 (m, 2H), 3.93–3.82 (m, 2H), 2.34 (s, 3H), 1.96 (m, 4H), 1.23–1.13 (m, 24H), 0.82 (t, 3H, 3J = 7.1 Hz); 13C NMR (100 MHz, DMSO-d6) δ (ppm): 174.3, 165.2, 146.4, 140.9, 132.3, 130.1, 129.9, 129.6, 128.3, 100.0, 63.9, 51.9, 31.7, 29.5, 29.3, 29.1, 29.0, 28.9, 28.5, 27.0, 25.7, 22.5, 17.4, 14.4; IR (max, cm−1): 3189; 2927; 1710; 1659; 1473; 1327; 1210; 1090; 754.
(Z)-Octadec-9-enyl 4-(3-chlorophenyl)-6-methyl-2-oxo-1,2,3,4-tetrahydropyrimidine-5-carboxylate (10f). MM = 516.31 g mol−1; white solid. M. P.: 84–87 °C; yield: 55%; 1H NMR (400 MHz, DMSO-d6) δ (ppm): 9.32 (s, 1H), 7.82 (s, 1H), 7.38–7.18 (m, 3H), 5.36–5.31 (m, 2H), 5.15 (d, 2H, 3J = 2.8 Hz), 4.03–3.85 (m, 2H), 2.29 (s, 3H), 2.0–1.98 (m, 4H), 1.45–1.41 (m, 2H), 1.25 (m, 22H), 0.84 (t, 3H, 3J = 6.3 Hz); 13C NMR (100 MHz, DMSO-d6) δ (ppm): 165.6, 152.2, 149.7, 147.6, 133.4, 130.9, 130.1, 127.7, 126.7, 125.3, 98.7, 63.7, 54.1, 31.7, 29.5, 29.3, 29.1, 29.0, 28.9, 28.6, 27.0, 25.9, 22.5, 18.2, 14.4; IR (max, cm−1): 3229; 3102; 2919; 2851; 1710; 1654; 1465; 1227; 1096; 706.
(Z)-Octadec-9-enyl 4-(3-chlorophenyl)-6-methyl-2-thioxo-1,2,3,4-tetrahydropyrimidine-5-carboxylate (11f). MM = 532.29 g mol−1; yellow oil; yield: 40%; 1H NMR (400 MHz, DMSO-d6) δ (ppm): 10.43 (s, 1H), 9.68 (s, 1H), 7.40–7.15 (m, 3H), 5.35–5.30 (m, 2H), 5.17 (d, 1H, 3J = 2.8 Hz), 4.05–3.86 (m, 2H), 2.31 (s, 3H), 1.99–1.96 (m, 4H), 1.47–1.41 (m, 2H), 1.23–1.15 (m, 22H), 0.84 (t, 3H, 3J = 6.5 Hz); 13C NMR (100 MHz, DMSO-d6) δ (ppm): 174.6, 165.4, 146.3, 146.1, 133.5, 131.1, 130.1, 128.1, 126.9, 125.5, 64.0, 54.1, 31.7, 29.5, 29.3, 29.1, 29.0, 28.5, 27.0, 25.8, 22.5, 17.6, 14.4; IR (max, cm−1): 3184; 2923; 2853; 1708; 1654; 1589; 1474; 1324; 1203; 1092; 751.
(Z)-Octadec-9-enyl 4-(4-chlorophenyl)-6-methyl-2-oxo-1,2,3,4-tetrahydropyrimidine-5-carboxylate (10g). MM = 516.31 g mol−1; white solid. M. P.: 86–89 °C; yield: 52%; 1H NMR (400 MHz, DMSO-d6) δ (ppm): 9.24 (s, 1H), 7.75 (s, 1H), 7.37 (d, 2H, 3J = 8.6 Hz), 7.23 (d, 2H, 3J = 8.3 Hz), 5.34–5.31 (m, 2H), 5.13 (d, 2H, 3J = 3.2 Hz), 4.01–3.95 (m, 1H), 3.89–3.85 (m, 1H), 2.26 (s, 3H), 1.99–1.97 (m, 4H), 1.42 (quint, 2H, 3J = 6.6 Hz), 1.28–1.14 (m, 22H), 0.84 (t, 3H, 3J = 6.5 Hz); 13C NMR (100 MHz, DMSO-d6) δ (ppm): 165.6, 152.2, 149.5, 144.1, 132.3, 130.5, 130.1, 128.8, 128.6, 98.9, 63.6, 53.9, 31.7, 29.5, 29.4, 29.3, 29.2, 29.1 (2C), 28.6, 27.0, 25.9, 22.5, 18.2, 14.4; IR (max, cm−1): 3249; 3122; 2925; 2855; 1712; 1652; 1465; 1289; 1229; 1090; 788.
(Z)-Octadec-9-enyl 4-(4-chlorophenyl)-6-methyl-2-thioxo-1,2,3,4-tetrahydropyrimidine-5-carboxylate (11g). MM = 532.29 g mol−1; yellow oil; yield: 48%; 1H NMR (400 MHz, DMSO-d6) δ (ppm): 10.38 (s, 1H), 9.65 (s, 1H), 7.40 (d, 2H, 3J = 8.4 Hz), 7.22 (d, 2H, 3J = 8.4 Hz), 5.33–5.31 (m, 2H), 5.16 (d, 1H, 3J = 3.4 Hz), 4.03–3.97 (m, 1H), 3.91–3.85 (m, 1H), 2.31 (s, 3H), 1.98–1.97 (m, 4H), 1.44–1.41 (m, 2H), 1.24–1.13 (m, 22H), 0.84 (t, 3H, 3J = 6.7 Hz); 13C NMR (100 MHz, DMSO-d6) δ (ppm): 174.5, 165.4, 146.1, 142.7, 132.7, 130.5, 130.1, 129.0, 128.8, 100.4, 63.9, 53.9, 32.4, 31.7, 29.5, 29.4, 29.3, 29.2, 29.1, 29.0, 28.5, 27.0, 25.8, 22.5, 17.6, 14.4; IR (max, cm−1): 3416; 2923; 2853; 1691; 1648; 1463; 1178; 1090; 1026; 827.
(Z)-Octadec-9-enyl 6-methyl-2-oxo-4-(p-tolyl)-1,2,3,4-tetrahydropyrimidine-5-carboxylate (10h). MM = 496.37 g mol−1; white solid; M. P.: 123–125 °C; yield: 60%; 1H NMR (400 MHz, DMSO-d6) δ (ppm): 9.14 (s, 1H), 7.66 (s, 1H), 7.10 (s, 4H), 5.34–5.31 (m, 2H), 5.09 (d, 2H, 3J = 3.3 Hz), 3.96–3.86 (m, 2H), 2.25 (s, 6H), 1.98–1.97 (m, 4H), 1.45–1.43 (m, 2H), 1.24–1.15 (m, 22H), 0.85 (t, 3H, 3J = 6.6 Hz); 13C NMR (100 MHz, DMSO-d6) δ (ppm): 165.8, 152.5, 148.8, 142.3, 136.7, 130.1, 129.3, 126.6, 99.6, 63.5, 54.1, 32.4, 31.7, 29.5, 29.4, 29.3, 29.2, 29.1 (2C), 29.0 (2C), 28.6, 27.0, 25.9, 22.5, 21.1 18.1, 14.4; IR (max, cm−1): 3249; 3116; 2923; 2851; 1726; 1708; 1654; 1465; 1229; 1092; 786.
(Z)-Octadec-9-enyl 6-methyl-2-thioxo-4-(p-tolyl)-1,2,3,4-tetrahydropyrimidine-5-carboxylate (11h). MM = 512.34 g mol−1; yellow oil; yield: 49%; 1H NMR (400 MHz, DMSO-d6) δ (ppm): 10.28 (s, 1H), 9.59 (s, 1H), 7.12–7.08 (m, 4H), 5.32–5.31 (m, 2H), 5.12 (d, 1H, 3J = 3.6 Hz), 4.00–3.95 (m, 1H), 3.92–3.88 (m, 1H), 2.30 (s, 3H), 2.26 (s, 3H), 1.99–1.97 (m, 4H), 1.45–1.42 (m, 2H), 1.28–1.15 (m, 22H), 0.85 (t, 3H, 3J = 6.4 Hz); 13C NMR (100 MHz, DMSO-d6) δ (ppm): 174.4, 165.6, 145.5, 140.9, 137.3, 130.1, 129.5, 126.8, 101.0, 63.9, 54.2, 32.4, 31.7, 29.5, 29.5, 29.4, 29.3, 29.2, 29.1, 29.0, 29.0, 28.6, 27.0, 25.8, 22.5, 21.1, 17.5, 14.4; IR (max, cm−1): 3163; 2925; 2853; 1710; 1652; 1595; 1469; 1276; 1198; 1096; 763.
(Z)-Octadec-9-enyl 4-(4-methoxyphenyl)-6-methyl-2-oxo-1,2,3,4-tetrahydropyrimidine-5-carboxylate (10i). MM = 512.36 g mol−1; white solid; M. P.: 118–120 °C; yield: 55%, 1H NMR (400 MHz, DMSO-d6) δ (ppm): 9.14 (s, 1H), 7.64 (s, 1H), 7.13 (d, 2H, 3J = 8.4 Hz), 6.85 (d, 2H, 3J = 8.7 Hz), 5.33–5.31 (m, 2H), 5.08 (d, 2H, 3J = 3.1 Hz), 3.99–3.93 (m, 1H), 3.90–3.84 (m, 1H), 3.71 (s, 3H), 2.25 (s, 3H), 1.98–1.97 (m, 4H), 1.45–1.42 (m, 2H), 1.28–1.16 (m, 22H), 0.85 (t, 3H, 3J = 6.6 Hz); 13C NMR (100 MHz, DMSO-d6) δ (ppm): 165.8, 158.9, 152.5, 148.7, 137.4, 130.1, 127.8, 114.1, 99.7, 63.5, 55.4, 53.8, 32.4, 31.7, 29.5, 29.4, 29.3, 29.2, 29.1, 29.1, 29.0 (2C), 28.6, 27.0, 25.9, 22.5, 18.1, 14.4; IR (max, cm−1): 3249; 3112; 2925; 2853; 1730; 1706; 1654; 1513; 1229; 1092; 782.
(Z)-Octadec-9-enyl 4-(4-methoxyphenyl)-6-methyl-2-thioxo-1,2,3,4-tetrahydropyrimidine-5-carboxylate (11i). MM = 528.34 g mol−1; yellow oil; yield: 48%; 1H NMR (400 MHz, DMSO-d6) δ (ppm): 10.28 (s, 1H), 9.57 (s, 1H), 7.12 (d, 2H, 3J = 8.7 Hz), 6.88 (d, 2H, 3J = 8.7 Hz), 5.33–5.31 (m, 2H), 5.10 (d, 1H, 3J = 3.5 Hz), 4.00–3.97 (m, 1H), 3.92–3.86 (m, 1H), 3.71 (s, 3H), 2.30 (s, 3H), 1.98–1.97 (m, 4H), 1.46–1.41 (m, 2H), 1.24–1.15 (m, 22H), 0.85 (t, 3H, 3J = 6.6 Hz); 13C NMR (100 MHz, DMSO-d6) δ (ppm): 174.3, 165.6, 159.2, 145.4, 136.0, 130.1, 128.1, 114.3, 101.1, 63.9, 55.5, 53.9, 32.4, 31.7, 29.5, 29.4, 29.3, 29.1, 29.0 (2C), 28.5, 27.0, 25.8, 22.5, 17.5, 14.4; IR (max, cm−1): 3190; 2925; 2853; 1706; 1582; 1511; 1465; 1274; 1182; 1110; 831.
(Z)-Octadec-9-enyl 4-(furan-2-yl)-6-methyl-2-oxo-1,2,3,4-tetrahydropyrimidine-5-carboxylate (10j). MM = 472.33 g mol−1; white solid. M. P.: 122–125 °C; yield: 50%; 1H NMR (400 MHz, DMSO-d6) δ (ppm): 9.24 (s, 1H), 7.75 (s, 1H), 7.53 (m, 1H), 6.33 (dd, 1H, 3J = 3.2 Hz, 3J = 1.9 Hz), 6.06 (d, 1H, 3J = 3.2 Hz), 5.33–5.31 (m, 2H), 5.20 (d, 2H, 3J = 3.4 Hz), 4.02–3.92 (m, 2H), 2.23 (s, 3H), 1.99–1.96 (m, 4H), 1.51–1.48 (m, 2H), 1.24–1.21 (m, 22H), 0.85 (t, 3H, 3J = 6.6 Hz); 13C NMR (100 MHz, DMSO-d6) δ (ppm): 165.5, 156.8, 152.8, 149.9, 142.5, 130.1, 110.7, 105.6, 97.1, 63.6, 48.1, 32.4, 31.7, 29.5, 29.4, 29.3, 29.2, 29.1, 29.0, 28.9, 28.6, 27.0, 25.8, 22.5, 18.1, 14.4; IR (max, cm−1): 3247; 3116; 2923; 2851; 1732; 1710; 1658; 1465; 1237; 1094; 796.
(Z)-Octadec-9-enyl 4-(furan-2-yl)-6-methyl-2-thioxo-1,2,3,4-tetrahydropyrimidine-5-carboxylate (11j). MM = 488.31 g mol−1; yellow solid; M. P.: 76–78 °C; yield: 47%; 1H NMR (400 MHz, DMSO-d6) δ (ppm): 10.40 (s, 1H), 9.64 (s, 1H), 7.57–7.56 (m, 1H), 6.36 (dd, 1H, 3J = 3.2 Hz, 3J = 1.8 Hz), 6.11 (d, 1H, 3J = 3.3 Hz), 5.34–5.31 (m, 2H), 5.22 (d, 2H, 3J = 3.7 Hz), 4.04–3.93 (m, 2H), 2.28 (s, 3H), 1.99–1.96 (m, 4H), 1.51–1.50 (m, 2H), 1.24–1.21 (m, 22H), 0.85 (t, 3H, 3J = 6.6 Hz); 13C NMR (100 MHz, DMSO-d6) δ (ppm): 175.3, 165.2, 155.1, 146.5, 143.0, 130.1, 110.9, 106.6, 98.5, 64.0, 48.1, 32.4, 31.7, 29.5, 29.4, 29.3, 29.2, 29.1, 29.0 (2C), 28.5, 27.0, 25.8, 22.5, 17.5, 14.4; IR (max, cm−1): 3184; 3001; 2919; 2851; 1716; 1654; 1591; 1467; 1313; 1188; 1096; 747.
Data availability
The data supporting this article have been included free of charge as ESI.†
Conflicts of interest
The authors declare no competing interests.
Acknowledgements
The authors are thankful for financial support from Coordenação de Aperfeiçoamento de Pessoal de Nivel Superior (CAPES), and Conselho Nacional de Desenvolvimento Científico e Tecnológico (CNPq). Fellowships from CAPES are also acknowledged.
References
- D. Nikam and A. Jain, Results Chem., 2023, 5, 100718 CrossRef CAS.
- International Agency for Research on Cancer, available on https://www.iarc.who.int/[accessed on March, 15th, 2024] Search PubMed.
- G. Woehlke and M. Schliwa, Nat. Rev. Mol. Cell Biol., 2000, 1, 50 CrossRef CAS PubMed.
- O. Rath and F. Kozielski, Nat. Rev. Cancer, 2012, 12, 527 CrossRef CAS.
- P. Biginelli, Ber. Dtsch. Chem. Ges., 1891, 24, 1317 CrossRef.
- C. O. Kappe, Acc. Chem. Res., 2000, 33, 879 CrossRef CAS PubMed.
- Â. de Fátima, T. C. Braga, L. S. da Neto, B. S. Terra, B. G. F. Oliveira, D. L. da Silva and L. V. Modolo, J. Adv. Res., 2015, 6, 363 CrossRef.
- R. Kaur, S. Chaudhary, K. Kumar, M. K. Gupta and R. K. Rawal, Eur. J. Med. Chem., 2017, 132, 108 CrossRef CAS PubMed.
- S. Faizan, T. F. Roohi, R. M. Raju, Y. Sivamani and P. Kumar, J. Mol. Struct., 2023, 1291, 136020 CrossRef CAS.
- Â. Fátima, T. C. Braga, L. S. Neto, B. S. Terra, B. G. F. Oliveira, D. L. Silva and L. V. Modolo, J. Adv. Res., 2015, 6, 363 CrossRef.
- T. U. Mayer, T. M. Kapoor, S. J. Haggarty, R. W. King, S. L. Schreiber and T. J. Mitchison, Science, 1999, 286, 971 CrossRef CAS PubMed.
- D. Russowsky, R. F. S. Canto, S. A. A. Sanches, M. G. M. D'Oca, A. de Fátima, R. A. Pilli, L. K. Kohn, M. A. Antônio and J. E. Carvalho, Bioorg. Chem., 2006, 34, 173 CrossRef CAS PubMed.
- T. A. Majrashi, S. Ahmed, H. A. Abd El Salam, G. H. Al-Ansary, M. Farouk Hamissa and W. M. Eldehna, RSC Adv., 2023, 13, 13655 RSC.
- A. Y. Khormi, R. El-sayed, T. A. Farghaly, M. R. Shaaban and A. M. Farag, Curr. Org. Synth., 2023, 20, 395 CrossRef CAS.
- T. G. M. Treptow, F. Figueiro, E. H. F. Jandrey, A. M. O. Battastini, C. G. Salbego, J. B. Hoppe, P. S. Taborda, S. B. Rosa, L. A. Piovesan, C. R. M. D'Oca, D. Russowsky and M. G. M. D'Oca, Eur. J. Med. Chem., 2015, 95, 552 CrossRef CAS PubMed.
- F. S. De Oliveira, P. M. De Oliveira, L. M. Farias, R. C. Brinkerhoff, R. C. M. A. Sobrinho, T. M. Treptow, C. R. Montes D'Oca, M. A. G. Marinho, M. A. Hort, A. P. Horn, D. Russowsky and M. G. Montes D'Oca, MedChemComm, 2018, 9, 1282 RSC.
- X. Liu, B. Testa and A. Fahr, Pharm. Res., 2010, 28, 962 CrossRef PubMed.
- M. G. M. D'Oca, R. M. Soares, R. R. Moura and V. F. Granjão, Fuel, 2012, 97, 884 CrossRef.
- P. K. P. G. Chopra, T. L. Lambat, S. H. Mahmood, R. G. Chaudhary and S. Banerjee, Chem. Select, 2021, 6, 6867 CAS.
- A. Samiei, M. A. Bodaghifard and M. Hamidinasab, Curr. Green Chem., 2024, 11, 194 CrossRef CAS.
- L. S. Santos, C. Theoduloz, R. A. Pilli and J. Rodriguez, Eur. J. Med. Chem., 2009, 44, 3810 CrossRef CAS PubMed.
|
This journal is © The Royal Society of Chemistry 2024 |
Click here to see how this site uses Cookies. View our privacy policy here.