DOI:
10.1039/D4RA03962A
(Paper)
RSC Adv., 2024,
14, 19581-19585
Modular access to furo[3,2-c]chromen-4-ones via Yb(OTf)3-catalyzed [3 + 2] annulation of 4-hydroxycoumarins with β-nitroalkenes†
Received
29th May 2024
, Accepted 6th June 2024
First published on 18th June 2024
Abstract
A facile and efficient strategy for modular access to furo[3,2-c]chromen-4-ones using 4-hydroxycoumarin and β-nitroalkenes via Lewis acid-catalyzed formal [3 + 2] annulation protocol is described. This reaction proceeds via cascade Michael addition/nucleophilic addition/elimination in the presence of Yb(OTf)3, which involves the formation of two new σ (C–C and C–O) bonds for the construction of a novel furan ring in a single operation. This protocol affords a variety of functional groups, thereby providing a practical and efficient method for the fabrication of a furo[3,2-c]chromen-4-one framework.
Introduction
Heterocycles featuring a chromen-4-one scaffold are abundantly found in natural products,1 biologically active intermediates2 and functional materials3 with significant pharmaceutical and chemosensing properties. In particular, furan-fused chromen-4-ones display a range of activities in biological investigations and drug explorations,4 for example, antiviral, anticancer, antifungal, antimicrobial, antioxidant, anticoagulant, anti-inflammatory, and Na+ and K+-ATPase inhibitory activities.5 There are two typical regioisomers of furochromen-4-one with respect to the reciprocal orientation of fused furan, namely furo[3,2-c]chromen-4-one and furo[2,3-c]chromen-4-one (Fig. 1a). It was noted that the furo[3,2-c]chromen-4-one isomer expresses exclusive diverse biological properties, such as neo-tanshinlactone, which is a potent phospholipase inhibitor, and norpterophyllin and norisoerlengieafusicol possess anticoagulant and antifungal activities (Fig. 1b).6 Apart from their medicinal properties, furan-fused chromen-4-ones also exhibit other important applications such as chemosensors, photosensitizers, and molecular probes.7
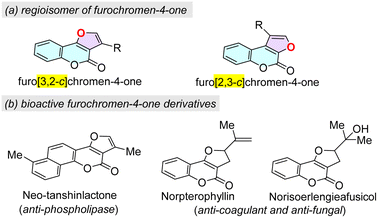 |
| Fig. 1 Regioisomer of furochromen-4-one and the representative bioactive furo[3,2-c]chromen-4-one derivatives. | |
Considering the wide range of applications of furo[3,2-c]chromen-4-ones, over the years, various synthetic methods for their preparation have varied but were extensively integrated with 4-hydroxycoumarin as the starting material.8 Employing aryl ketones as starting materials, Zhang and Phan reported a metal-free protocol for the synthesis of furo[3,2-c]chromen-4-ones (Scheme 1a).9 Later, an improved formal [3 + 2] annulation of 4-hydroxycoumarins with oxime esters for constructing 2-aryl furo[3,2-c]chromen-4-ones was further developed (Scheme 1b).10 Noland's group introduced an iron(III)-catalyzed three-component reaction of 4-hydroxycoumarins, 2-methylfuran, and aldehydes for the one-pot synthesis of functionalized furo[3,2-c]chromen-4-ones (Scheme 1c).11 In 2022, Choudhury and co-workers disclosed a thiol-dependent reaction of arylglyoxal and 4-hydroxycoumarins in the presence of Sc(OTf)3 for the easy access of 2-phenyl-4H-furo[3,2-c]chromen-4-ones (Scheme 1d).12 Very recently, Yang and co-workers established a regioselective Lewis acid-catalyzed cascade annulation of o-hydroxyphenyl propargylamines with 4-hydroxycoumarins to give 2,3-disubstituted furo[3,2-c]chromen-4-ones (Scheme 1e).13 β-Nitroalkenes have recently emerged as an adaptable C2-synthon in the synthesis of countless heterocycles.14 For example, a furan framework could be formed through the condensation reaction between 1,3-dicarbonyl equivalents and nitroalkenes.15 In recent years, β-nitroalkenes have also been used to construct a furocoumarin scaffold with 4-hydroxycoumarins under various catalytic systems.16 In continuation of our interest in investigating fused heterocyclic systems,17 herein, we would like to report a facile strategy for modular access to furo[3,2-c]chromen-4-ones in moderate to good yields via the formal [3 + 2] annulations of 4-hydroxycoumarins with β-nitroalkenes in the presence of Yb(OTf)3 as the Lewis acid (Scheme 1f).
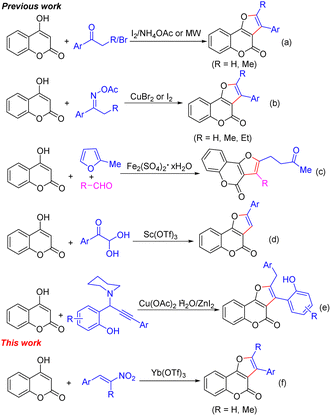 |
| Scheme 1 Diverse strategies for the synthesis of furo[3,2-c]chromen-4-one derivatives. | |
Results and discussion
The reaction of 4-hydroxycoumarin 1a and β-nitroalkene 2a was selected as a model reaction for the optimization of reaction conditions (Table 1). Using 1,2-dichloroethane (1,2-DCE) as the solvent, four different rare-earth metal triflates were screened, and 3a was generally obtained (Table 1, entries 2–5). Yb(OTf)3 was found to be the most efficient catalyst for this reaction, providing the highest yield of the desired product 3a (Table 1, entry 5). No reaction occurred in the absence of the catalyst or when the reaction was performed at room temperature (Table 1, entries 1 and 6). Changing the solvent to toluene, DMF, or THF gave inferior results (Table 1, entries 7–9). Further screening of the catalyst loading amount revealed that 10 mol% was optimal for the reaction, while lower (5 mol%) or higher (20 mol%) loadings all led to no improvement in the yield (Table 1, entries 10 and 11). It was worth mentioning that the reaction is tolerant of moisture and air and could be performed in commercial solvents under open air atmosphere.
Table 1 Screening of the reaction conditionsa

|
Entry |
Catalyst (mol%) |
Solvent |
Temp. (°C) |
Yieldb (%) |
Reaction conditions: 1a (0.5 mmol), 2a (0.5 mmol), solvent (5 mL), reaction time (24 h), and the reaction was monitored using TLC. Yield of the isolated product. |
1 |
No catalyst |
1,2-DCE |
84 |
0 |
2 |
Sc(OTf)3 (10%) |
1,2-DCE |
84 |
55 |
3 |
Y(OTf)3 (10%) |
1,2-DCE |
84 |
57 |
4 |
La(OTf)3 (10%) |
1,2-DCE |
84 |
46 |
5 |
Yb(OTf)3 (10%) |
1,2-DCE |
84 |
62 |
6 |
Yb(OTf)3 (10%) |
1,2-DCE |
25 |
0 |
7 |
Yb(OTf)3 (10%) |
Toluene |
90 |
46 |
8 |
Yb(OTf)3 (10%) |
DMF |
90 |
0 |
9 |
Yb(OTf)3 (10%) |
THF |
66 |
0 |
10 |
Yb(OTf)3 (5%) |
1,2-DCE |
84 |
54 |
11 |
Yb(OTf)3 (20%) |
1,2-DCE |
84 |
60 |
12 |
TfOH (20%) |
1,2-DCE |
84 |
20 |
13 |
TsOH (20%) |
1,2-DCE |
84 |
17 |
With the optimized reaction conditions in hand, we then turned our attention to investigate the substrate scope (Table 2). In general, the reaction proceeded smoothly with a broad spectrum of β-nitroalkenes 2. Electron-rich (–OMe) and electron-deficient (–Cl, –Br) β-nitrostyrenes were well-tolerated, affording the corresponding 2-aryl furo[3,2-c]chromen-4-ones in 45–67% yields. Particularly, no significant effects were observed when altering the substituent to meta- or ortho-position (products 3e–3j). It is notable to show that halogen groups, e.g., –Cl and –Br, remained intact under these reaction conditions (products 3c, 3d, 3f, 3g, 3i, and 3j), which makes this transformation particularly attractive in terms of increasing the molecular complexity through transition metal-catalyzed crossing-coupling protocols. Encouragingly, disubstituted β-nitrostyrene was also successfully coupled with 1a and generated the corresponding product 3k in 67% yield. Subsequently, the suitability of 4-hydroxycoumarins was also tested under the standard conditions. Substituents including electron-withdrawing groups (e.g., –F, –Cl, and –Br) and electron-donating groups (e.g., –Me) at the 6-position of the phenyl ring were well tolerated, giving the corresponding products 3l–3o in 44–68% yields. To our delight, the desired product 3p was successfully obtained in 52% yield when altering the position of the methyl group.
Table 2 Substrate scope of 4-hydroxycoumarin with β-nitrostyrenesa
Reaction conditions: 4-hydroxycoumarin 1 (0.5 mmol), β-nitrostyrenes 2 (0.5 mmol), and Yb(OTf)3 (0.05 mmol) in 1,2-DCE (5 mL) at reflux under air atmosphere for 24 h. Isolated yields were reported. |
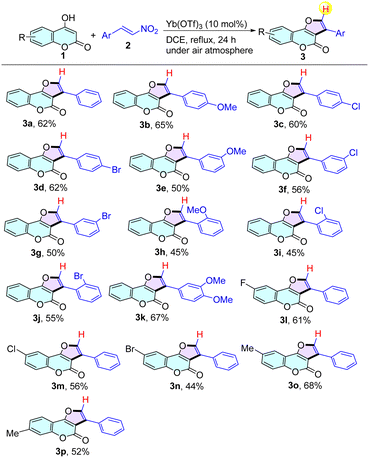 |
We next examined the structural diversity of various β-2-nitrophenylpropenes for the formation of 2,3-disubstituted furo[3,2-c]chromen-4-ones (Table 3). Substituents including electron-neutral (–Me), electron-rich (–OMe) and electron-deficient (–F, –Cl, –Br) β-2-nitrophenylpropenes at the para- and meta-positions of the benzene ring were well-tolerated, generating the desired products 5b–5g in 36–58% yields. Moreover, the structure of the products 5d (CCDC 2354856) was additionally confirmed by X-ray crystallographic analysis (see the ESI† for details).
Table 3 Substrate scope of 4-hydroxycoumarin with β-2-nitrophenylpropenesa
Reaction conditions: 4-hydroxycoumarin 1 (0.5 mmol), β-2-nitrophenylpropenes 2 (0.5 mmol), and Yb(OTf)3 (0.05 mmol) in 1,2-DCE (5 mL) at reflux under air atmosphere for 24 h. Isolated yields were reported. |
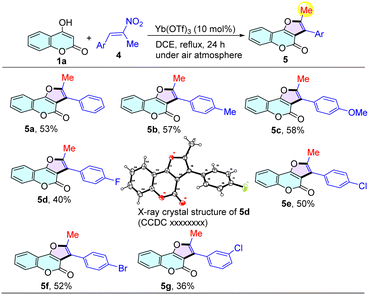 |
To further explore the practical utility of this methodology as a synthetic tool, a scale-up synthesis of the products 3a and 5a was carried out under the standard conditions (Scheme 2). Encouragingly, this transformation could be scaled easily to 10.0 mmol to afford the desired products 3a and 5a without significantly compromising the yield, which showed promise for this synthetic strategy as a useful protocol in practical synthetic contexts.
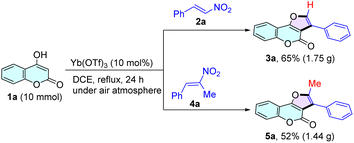 |
| Scheme 2 Scale-up synthesis of 3a and 5a. | |
Based on previous results obtained by other research groups,18 a plausible mechanism for the Yb(OTf)3-catalyzed cyclization reaction of 4-hydroxycoumarin with β-nitrostyrenes was proposed (Scheme 3). Initially, the intermediate A was generated from the Michael addition of 4-hydroxycoumarin 1a to β-nitrostyrene 2a in the presence of Yb(OTf)3 and further underwent the keto–enol tautomerization to give the intermediate B. Subsequently, intramolecular nucleophilic addition of B would produce the intermediate C. Finally, the desired product 3a was formed through the dehydration and elimination of hyponitrous acid (HNO).
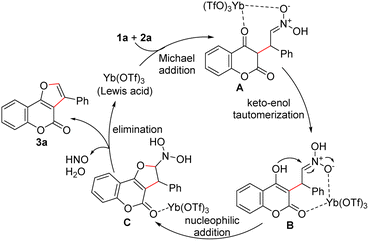 |
| Scheme 3 A possible mechanism for dehydrative annulations. | |
Conclusions
In summary, we have successfully developed an Yb(OTf)3-catalyzed formal [3 + 2] annulation of 4-hydroxycoumarins with β-nitroalkenes for modular access to furo[3,2-c]chromen-4-ones. Through this transformation, the furo[3,2-c]chromen-4-one framework was efficiently constructed, in which two new σ (C–C and C–O) bonds and a new furan ring were formed simultaneously in a single operation. This protocol features broad substrate scope, good functional groups, and without the need for inert atmosphere protection.
Data availability
The data supporting this article have been included as part of the ESI.†
Author contributions
Conceptualization, H. Wang and X. He; methodology, H. Wang and Q. Ma; formal analysis, Y. Sun and S. Zhao; data curation, H. Wang and Y. Sun; writing – original draft preparation, H. Wang and Q. Ma; writing – review and editing, S. Wang and X. He; project administration, S. Wang and X. He; funding acquisition, S. Wang and X. He. All authors have read and agreed to the published version of the manuscript.
Conflicts of interest
There are no conflicts to declare.
Acknowledgements
We thank the Natural Scientific Research Foundations of Anhui Provincial Universities (No. 2022AH050210), the College Students’ Innovation and Entrepreneurship Program of Anhui Province (S201910368095, 202310370018, 202310370284) for financial support.
Notes and references
- For selected reviews, see:
(a) F. G. Medina, J. G. Marrero, M. Macías-Alonso, M. C. González, I. Córdova-Guerrero, A. G. T. García and S. Osegueda-Robles, Nat. Prod. Rep., 2015, 32, 1472–1507 RSC;
(b) Y.-S. Wang, B.-T. Li, S.-X. Liu, Z.-Q. Wen, J.-H. Yang, H.-B. Zhang and X.-J. Hao, J. Nat. Prod., 2017, 80, 798–804 CrossRef CAS PubMed.
- For selected reviews, see:
(a) M. Koley, J. Han, V. A. Soloshonok, S. Mojumder, R. Javahershenas and A. Makarem, RSC Med. Chem., 2024, 15, 10–54 RSC;
(b) H. Wei, J. Ruan and X. Zhang, RSC Adv., 2016, 6, 10846–10860 RSC;
(c) J. Grover and S. M. Jachak, RSC Adv., 2015, 5, 38892–38905 RSC.
- For selected reviews, see:
(a) D. Cao, Z. Liu, P. Verwilst, S. Koo, P. Jangjili, J. S. Kim and W. Lin, Chem. Rev., 2019, 119, 10403–10519 CrossRef CAS PubMed;
(b) M. Tasior, D. Kim, S. Singha, M. Krzeszewski, K. H. Ahn and D. T. Gryko, J. Mater. Chem. C, 2015, 3, 1421–1446 RSC.
- J. L. Rodrigues and L. R. Rodrigues, Nat. Prod. Rep., 2021, 38, 869–879 RSC.
-
(a) A. A. Abu-Hashem and M. El-Shazly, Eur. J. Med. Chem., 2015, 90, 633–665 CrossRef CAS PubMed;
(b) X. Wang, K. F. Bastow, C.-M. Sun, Y.-L. Lin, H.-J. Yu, M.-J. Don, T.-S. Wu, S. Nakamura and K.-H. Lee, J. Med. Chem., 2004, 47, 5816–5819 CrossRef CAS PubMed;
(c) E. S. C. Pôçasa, D. V. S. Lopes, A. J. M. da Silva, P. H. C. Pimenta, F. B. Leitão, C. D. Netto, C. D. Buarque, F. V. Brito, P. R. R. Costa and F. Noël, Bioorg. Med. Chem., 2006, 14, 7962–7966 CrossRef PubMed;
(d) K. V. S. Mani Chandrika and S. Sharma, Bioorg. Med. Chem., 2020, 28, 115398 CrossRef CAS PubMed.
-
(a) X. Wang, K. F. Bastow, C.-M. Sun, Y.-L. Lin, H.-J. Yu, M.-J. Don, T.-S. Wu, S. Nakamura and K.-H. Lee, J. Med. Chem., 2004, 47, 5816–5819 CrossRef CAS PubMed;
(b) I. Cortés, L. J. Cala, A. B. J. Bracca and T. S. Kaufman, RSC Adv., 2020, 10, 33344–33377 RSC.
-
(a) N. M. Sarih, A. Ciupa, S. Moss, P. Myers, A. G. Slater, Z. Abdullah, H. A. Tajuddin and S. Maher, Sci. Rep., 2020, 10, 7421 CrossRef CAS PubMed;
(b) N. Kitamura, S. Kohtani and R. Nakagaki, J. Photochem. Photobiol., C, 2005, 6, 168–185 CrossRef CAS.
-
(a) C.-J. Lee, Y.-J. Jang, Z.-Z. Wu and W. Lin, Org. Lett., 2012, 14, 1906–1909 CrossRef CAS PubMed;
(b) D. Zha, H. Li, S. Li and L. Wang, Adv. Synth. Catal., 2017, 359, 467–475 CrossRef CAS;
(c) X. Chang, X. Zhang and Z. Chen, Org. Biomol. Chem., 2018, 16, 4279–4287 RSC;
(d) W.-Y. Huang, Y.-C. Chen and K. Chen, Chem.–Asian J., 2012, 7, 688–691 CrossRef CAS PubMed;
(e) A. Dey and A. Hajra, Org. Biomol. Chem., 2017, 15, 8084–8090 RSC;
(f) R.-Q. Mei, X.-Y. Xu, L. Peng, F. Wang, F. Tian and L.-X. Wang, Org. Biomol. Chem., 2013, 11, 1286–1289 RSC;
(g) V. Modrocká, E. Veverková, R. Baran and R. Šebesta, ChemistrySelect, 2018, 3, 1466–1471 CrossRef.
-
(a) M. Zhang, R. Zhang, J. Wang, X. Yu, Y. Zhang, Q. Wang and W. Zhang, Chin. J. Chem., 2016, 34, 1344–1352 CrossRef CAS;
(b) P. H. Pham, Q. T. D. Nguyen, N. K. Q. Tran, V. H. H. Nguyen, S. H. Doan, H. Q. Ha, T. Truong and N. T. S. Phan, Eur. J. Org. Chem., 2018, 2018, 4431–4435 CrossRef CAS;
(c) A. R. Kaneria, R. R. Giri, V. G. Bhila, H. J. Prajapati and D. I. Brahmbhatt, Arabian J. Chem., 2017, 10, S1100–S1104 CrossRef CAS.
-
(a) T. A. To, Y. H. Vo, A. T. Nguyen, A. N. Q. Phan, T. Truong and N. T. S. Phan, Org. Biomol. Chem., 2018, 16, 5086–5089 RSC;
(b) Q. T. Pham, P. Q. Le, H. V. Dang, H. Q. Ha, H. T. D. Nguyen, T. Truong and T. M. Le, RSC Adv., 2020, 10, 44332–44338 RSC.
- W. E. Noland, H. V. Kumar, A. Sharma, B. Wei and S. Girmachew, Org. Lett., 2020, 22, 1801–1806 CrossRef CAS PubMed.
- A. Jana, D. Ali, P. Bhaumick and L. H. Choudhury, J. Org. Chem., 2022, 87, 7763–7777 CrossRef CAS PubMed.
- G. S. Sorabad and D.-Y. Yang, J. Org. Chem., 2023, 88, 4730–4742 CrossRef CAS PubMed.
- For recent reviews, see:
(a) A. Z. Halimehjani, I. N. N. Namboothiri and S. E. Hooshmand, RSC Adv., 2014, 4, 31261–31299 RSC;
(b) S. T. Sivanandan, M. J. Jesline, D. K. Nair and T. Kumar, Asian J. Org. Chem., 2023, 12, e202200555 CrossRef CAS;
(c) F. Doraghi, M. M. A. Ashtiani, F. Moradkhani, B. Larijani and M. Mahdavi, RSC Adv., 2024, 14, 14835–14846 RSC.
-
(a) A. Y. Barkov, V. Y. Korotaev and V. Y. Sosnovskikh, Tetrahedron Lett., 2013, 54, 4181–4184 CrossRef CAS;
(b) M. Ghosh, S. Santra, P. Mondal, D. Kundu and A. Hajra, Chem.–Asian J., 2015, 10, 2525–2536 CrossRef CAS PubMed;
(c) M. Arai, Y. Miyauchi, T. Miyahara, T. Ishikawa and S. Saito, Synlett, 2009, 122–126 CAS;
(d) M. Miyashita, T. Kumazawa and A. Yoshikoshi, J. Org. Chem., 1980, 45, 2945–2950 CrossRef CAS;
(e) R. Ballini, S. Gabrielli and A. Palmieri, Synlett, 2010, 2468–2470 CAS;
(f) V. Mane, T. Kumar, S. Pradhan, S. Katiyar and I. N. N. Namboothiri, RSC Adv., 2015, 5, 69990–69999 RSC;
(g) D. Dauzonne, H. Josien and P. Demerseman, Tetrahedron, 1990, 46, 7359–7371 CrossRef CAS.
-
(a) S. Singh, A. Srivastava, S. M. Mobin and S. Samanta, RSC Adv., 2015, 5, 5010–5014 RSC;
(b) D. I. Brahmbhatt, B. R. Hirani, S. U. Pandya and U. R. Pandya, Indian J. Chem., Sect. B: Org. Chem. Incl. Med. Chem., 2000, 39, 233–235 Search PubMed;
(c) M. Ghosh and A. Hajra, Eur. J. Org. Chem., 2015, 7836–7841 CrossRef CAS.
-
(a) H. Yin, Y. Wu, Y. Jiang, M. Wang and S. Wang, Org. Lett., 2023, 25, 3078–3082 CrossRef CAS PubMed;
(b) J. Duan, X. He, P. Y. Choy, Q. Wang, M. Xie, R. Li, K. Xu, Y. Shang and F. Y. Kwong, Org. Lett., 2021, 23, 6455–6460 CrossRef CAS PubMed;
(c) H. Yin, Y. Wu, X. Gu, Z. Feng, M. Wang, D. Feng, M. Wang, Z. Cheng and S. Wang, RSC Adv., 2022, 12, 21066–21078 RSC;
(d) H. Yin, Q. Ma, Y. Wang, X. Gu, Z. Feng, Y. Wu, M. Wang and S. Wang, RSC Adv., 2021, 11, 19639–19646 RSC;
(e) X. He, R. Li, P. Y. Choy, T. Liu, J. Wang, O. Ying Yuen, M. P. Leung, Y. Shang and F. Y. Kwong, Org. Lett., 2020, 22, 9444–9449 CrossRef CAS PubMed;
(f) M. Shao, Y. Wu, Z. Feng, X. Gu and S. Wang, Org. Biomol. Chem., 2016, 14, 2515–2521 RSC.
-
(a) M. Ghosh, S. Santra, P. Mondal, D. Kundu and A. Hajra, Chem.–Asian J., 2015, 10, 2525–2536 CrossRef CAS PubMed;
(b) S. Singh, A. Srivastava, S. M. Mobin and S. Samanta, RSC Adv., 2015, 5, 5010–5014 RSC;
(c) F. Zhang, C. Li, C. Wang and C. Qi, Org. Biomol. Chem., 2015, 13, 5022–5029 RSC;
(d) R. Gattu, S. Bhattacharjee, K. Mahato and A. T. Khan, Org. Biomol. Chem., 2018, 16, 3760–3770 RSC;
(e) R. Gattu, S. Mondal, S. Ali and A. T. Khan, Org. Biomol. Chem., 2019, 17, 347–353 RSC.
Footnote |
† Electronic supplementary information (ESI) available: Copies of 1H and 13C NMR spectra for newly synthesized compounds, CIF for compound 5d. CCDC 2354856. For ESI and crystallographic data in CIF or other electronic format see DOI: https://doi.org/10.1039/d4ra03962a |
|
This journal is © The Royal Society of Chemistry 2024 |
Click here to see how this site uses Cookies. View our privacy policy here.