DOI:
10.1039/D4RA03987D
(Paper)
RSC Adv., 2024,
14, 29282-29287
A synthetic method for lanthanum hydroxychloride suitable for industrialization and its thermal decomposition properties†
Received
30th May 2024
, Accepted 16th July 2024
First published on 16th September 2024
Abstract
A reactive crystallization method for the synthesis of lanthanum hydroxychloride (La(OH)2Cl) was developed to provide a new low-carbon route for the purification of rare earth elements in hydrometallurgy. The synthetic method reported herein represents a unique low-temperature route, short preparation time and inexpensive materials compared with previous methods, thus making it promising for industrial applications. The key factors controlling the product's phase were determined using single factor tests involving temperature, concentration of NH4Cl in the base solution and the molar ratio of NH4OH/La3+ added per minute into the base solution. Otherwise, the outcome is lanthanum hydroxide (La(OH)3). Subsequently, the synthesized products were characterized by employing X-ray diffraction (XRD), Fourier transform infrared (FT-IR) spectroscopy, transmission electron microscopy (TEM), and energy dispersive spectroscopy (EDS). Finally, the thermal decomposition behavior of La(OH)2Cl was assessed using thermogravimetry (TG) and differential scanning calorimetry (DSC). Lanthanum oxychloride (LaClO) was obtained at about 360 °C. The thermal transformation from LaClO to La2O3 started at 400 °C and was a slow oxidation process. Pure La2O3 could be obtained when the temperature increased to 1500 °C.
1. Introduction
Rare earth ions are extracted via ammonium bicarbonate precipitation in the rare earth hydrometallurgy process.1,2 However, massive amounts of carbon dioxide are released in this mainstream technology.3 Some carbon dioxide is produced during the reaction, and the rest arises from thermal decomposition to obtain rare earth oxide. However, weather patterns have become more volatile, and extremes have become more common as global warming continues.4 Therefore, low-carbon development in all sectors is put forward to solve this problem.5,6 Therefore, low-carbon reforms in the purification process of rare earth elements is imperative.
Lanthanide hydroxychloride (Ln(OH)2Cl) has a monoclinic structure in which the adjacent layers are predominantly held by O–H⋯Cl hydrogen bonds.7–9 It can be decomposed into lanthanum oxychloride through calcination, as per the equation: Ln(OH)2Cl → LnOCl + H2O (↑).7,10,11 Moreover, Ln(OH)2Cl can be further decomposed into rare earth oxides in an air atmosphere at over 1050 °C.12,13 Water and chlorine are the byproducts produced during this thermal decomposition process. In other words, thermal decomposition is advantageous over the current practice of preparing rare earth oxides from rare earth carbonates as it can significantly reduce carbon emissions. Moreover, no carbon dioxide is produced during Ln(OH)2Cl precipitation. It presents a distinctive and transparent approach for advancing a low-carbon economy in the metallurgical processing of rare earth elements.
However, currently, there are limited methods available for the synthesis of Ln(OH)2Cl. The hydrothermal method is the most commonly used (Table 1), but it requires specialized equipment to reach high temperatures and pressures, and it can be hardly applied to the industrial process. Thus, in this work, we developed a reactive crystallization method to synthesize lanthanum hydroxychloride without using any surfactant, chelating agent, gelating agent or extreme conditions. The operation is simple, convenient, and fit for industrialization. We selected lanthanum for the experiments because of its sufficient reserves and single valence and investigated the necessary factors for synthesizing La(OH)2Cl. Finally, we explored the thermal decomposition properties of lanthanum hydroxychloride.
Table 1 The contrast between previously reported methods and our method for the synthesis of La(OH)2Cl
Quote |
Method |
Temperature |
Time |
Materials |
Carter et al., 1969 (ref. 8) |
Hydrothermal method |
500 °C |
2–5 days |
NH4Cl, AlCl3·6H2O, HNO3, HCl |
Jouve et al., 2002 (ref. 14) |
Hydrothermal method |
200 °C |
2 days |
Sm2O3, NH4Cl |
Mahajan et al., 2008 (ref. 15) |
Thermally assisted hydrolysis |
130 °C |
32 h |
EuCl3·6H2O, [CH3(CH2)7]3PO, NaOH |
Lee et al., 2008 (ref. 7) |
Solvothermal method |
600 °C |
10 h |
LaCl3·7H2O, TmCl3, ethanol |
Kim et al., 2017 (ref. 16) and Soto-Donoso et al., 2023 (ref. 17) |
Liquid preparation method |
90 °C |
12 h |
LaCl3, NaCl, ethylenediamine |
Dunn et al., 2020 (ref. 18) |
Solvothermal method |
240 °C |
24 h |
LnCl3·7H2O, polyethylene glycol |
Fellner et al., 2021 (ref. 19) |
Microwave solvothermal method |
150/200 °C |
≤1 h |
LuCl3, benzyl alcohol, Eu(Ac)3 |
This work |
Homogeneous preparation method |
60–80 °C |
4 h |
LaCl3, NH4OH, NH4Cl, water |
2. Experiment
2.1 Materials and chemical analysis methods
An aqueous solution of lanthanum chloride was offered by China Northern Rare Earth (Group) High-Tech Co., Ltd, and its chemical composition is listed in Table 2. The total rare earth content was measured using the EDTA (ethylene diamine tetraacetic acid) complexometric titration method, while the ion impurities were measured by the ICP (Inductively Coupled Plasma) method. Other chemical agents used in our experiments were of analytical grade and used as received without further purification.
Table 2 Chemical compositions of an aqueous solution of lanthanum chloride (g L−1)
Elements |
REO |
MgO |
Al2O3 |
Na2O |
CaO |
ZnO |
Fe2O3 |
Concentration |
303.7 |
0.023 |
0.022 |
<0.005 |
0.039 |
0.680 |
<0.005 |
2.2 Preparation procedure
In this experiment, a parallel flow feeding mode was utilized to decrease the supersaturation of this reaction system (Fig. 1). It is helpful to form large and uniform crystal size. To achieve the desired concentration (100 g L−1) of lanthanum chloride solution, a 66 mL rare earth solution was diluted with 154 mL of distilled water. The reason why we used rare earth chloride is because it is the extraction product in the rare earth hydrometallurgy process. The precipitant was prepared by diluting 45 mL of commercial ammonia (13.38 M) with 105 mL of distilled water. Subsequently, these solutions were simultaneously added dropwise to ammonium chloride aqueous solutions with varying concentrations and temperatures. Ammonium chloride aqueous can provide an abundance of chloride ions. Also, a certain temperature can not only offer the energy of product formation but also decrease the supersaturation. Notably, we investigated the effect of the molar ratio of NH4OH/La3+ dropped per minute into the base solution by adjusting the feeding rate of ammonia. We found three major factors, namely, temperature, concentration of NH4Cl in the base solution and the molar ratio of NH4OH/La3+ dropped per minute into the base solution, through a great number of experiments. In this paper, they were examined and are summarized in Table 3. The resulting products were promptly collected under the aforementioned conditions using a Buchner funnel, washed three times with distilled water, and dried in an oven at 70 °C. Parallel experiments were performed to ensure the accuracy of the experimental result.
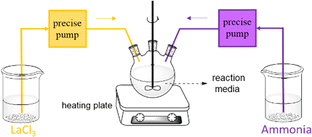 |
| Fig. 1 Schematic of the reaction setup. | |
Table 3 Major experimental conditions for the preparation
Sample no. |
Temperature (°C) |
Concentration of NH4Cl (M) |
Feeding rate of ammonia (mL min−1) |
Molar ratio of NH4OH/La3+ dropped per minute |
1 |
80 |
1.0 |
0.38 |
3.0 |
2 |
80 |
1.8 |
0.38 |
3.0 |
3 |
80 |
2.0 |
0.38 |
3.0 |
4 |
80 |
3.0 |
0.38 |
3.0 |
5 |
80 |
4.0 |
0.38 |
3.0 |
6 |
70 |
1.0 |
0.38 |
3.0 |
7 |
70 |
2.0 |
0.38 |
3.0 |
8 |
70 |
2.8 |
0.38 |
3.0 |
9 |
70 |
3.0 |
0.38 |
3.0 |
10 |
70 |
4.0 |
0.38 |
3.0 |
11 |
60 |
3.0 |
0.38 |
3.0 |
12 |
60 |
4.0 |
0.38 |
3.0 |
13 |
80 |
2.0 |
0.37 |
2.9 |
14 |
80 |
2.0 |
0.39 |
3.1 |
15 |
80 |
4.0 |
0.39 |
3.1 |
16 |
80 |
1.0 |
0.21 |
2.0 |
2.3 Instrumentation
Powder X-ray diffraction (XRD) patterns were collected on a DX-27 mini X-ray diffractometer (Dandong Haoyuan Instrument Co., Ltd, China) using Cu Kα radiation with a step size of 0.02° from 5 to 90°. Fourier transform infrared (FT-IR) spectra were recorded using KBr pellets on a Nicolet iS 10 spectrophotometer (Thermo Fisher Scientific Inc., USA). Transmission electron microscopy (TEM) images and high-resolution TEM (HRTEM) images were obtained on a Talos F200i transmission electron microscope (Thermo Fisher Scientific Inc., USA) with an accelerating voltage of 200 kV. Thermogravimetry-differential scanning calorimetry (TG-DSC) analyses were carried out using an STA449 F3 simultaneous thermal analyzer (NETZSCH, Germany) in an air atmosphere in the temperature range from room temperature to 800 °C, and the heating rate was 5 °C min−1. Field emission-scanning electron microscopy (FE-SEM) images were collected on a Sigma 500 electron microscope electron microscope (Carl Zeiss, Germany) operating at 15 kV.
3. Results and discussion
3.1 Effect of the key factors on the phase of the product
Fig. 2 compares the wide-angle XRD patterns of samples 1 and 5, which were selected as representatives due to their extreme synthesis conditions. The others are shown in the ESI.† As shown in Fig. 2a, the diffraction peaks centered at 2θ = 13.96°, 16.02°, 24.69°, 27.59°, 27.19°, 33.52°, 36.21°, and 36.30° correspond to the reflection planes of (001), (−101), (101), (−111), (110), (111), (012), and (−211), respectively, for La(OH)2Cl. These peaks are consistent with the monoclinic phase structure of La(OH)2Cl, with the space group of P21/m. Also, no additional diffraction peaks of other impurity phases are observed. Besides, the positions and relative intensities of the diffraction peaks in Fig. 2b are indexed to the hexagonal structure of La(OH)3 with a space group of P63/m.
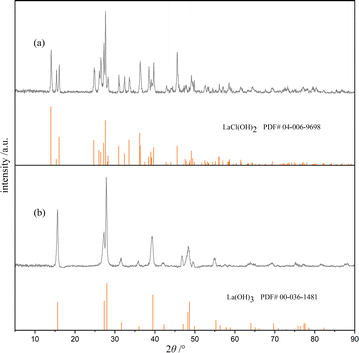 |
| Fig. 2 XRD patterns of (a) sample 5 and (b) sample 1. | |
Table 4 displays the phase of the product formed, determined from the XRD patterns of samples 1–16 (detailed XRD patterns for each sample are provided in the ESI,† as shown in Fig. S2 to S17†). The results indicate that the product obtained at 80 °C is La(OH)2Cl if the concentration of NH4Cl in the base solution is equal to or greater than 2 mol L−1. On the other hand, if the concentration of NH4Cl is less than 2 mol L−1, the resulting product is La(OH)3 instead of La(OH)2Cl. This result shows that the concentration of chloride ion is significant for the production of La(OH)2Cl. At a lower reaction temperature of 70 °C, the concentration of NH4Cl must increase to 3 mol L−1 to obtain La(OH)2Cl. However, at a reaction temperature of 60 °C, La(OH)2Cl precipitation cannot be obtained under any conditions. This indicates that the formation of La(OH)2Cl needs enough energy to go over the barrier. It is important to note that the above conclusions are valid only if the molar ratio of NH4OH/La3+ dropped per minute into the base solution is less than or equal to 3. From the results of samples 14 and 15, it is inferred that when the molar ratio of NH4OH/La3+ exceeds 3, the resulting product is La(OH)3. This outcome suggests that it is not conducive to form La(OH)2Cl when the reaction system is too alkaline. Additionally, the results of sample 16 reveal that if the concentration of NH4Cl does not meet the required standards, we cannot obtain the desired product. Again, the concentration of chloride ion is a crucial effect factor. To sum up, the production of La(OH)2Cl needs sufficient energy, sufficient concentration of chloride ion and lower alkaline reaction system. Also, it is necessary to control the several experimental parameters simultaneously to meet the formation conditions.
Table 4 Experimental phase of the product formed and yield
Sample no. |
Phase |
Yield |
Sample no. |
Phase |
Yield |
1 |
La(OH)3 |
— |
9 |
La(OH)2Cl |
97.14% |
2 |
La(OH)3 |
— |
10 |
La(OH)2Cl |
96.34% |
3 |
La(OH)2Cl |
97.55% |
11 |
La(OH)3 |
— |
4 |
La(OH)2Cl |
97.10% |
12 |
La(OH)3 |
— |
5 |
La(OH)2Cl |
99.16% |
13 |
La(OH)2Cl |
96.73% |
6 |
La(OH)3 |
— |
14 |
La(OH)3 |
— |
7 |
La(OH)3 |
— |
15 |
La(OH)3 |
— |
8 |
La(OH)3 |
— |
16 |
La(OH)3 |
— |
Fig. 3 presents a schematic illustration that aims to explain the cooperative effect of the three experimental factors mentioned above on the phase of the product. The gray area, including the borderline, represents the only region where the La(OH)2Cl product is obtained. The dashed line in this illustration implies that the molar ratio of NH4OH/La3+ dropped per minute in the base solution is equal to 3 and can move left and right. The area to the left of the dashed line indicates that the molar ratio is greater than 3. Furthermore, as the line moves from the left to the right, the region to the left of the dashed line turns white, implying the formation of La(OH)3.
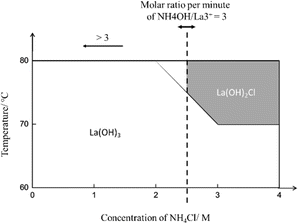 |
| Fig. 3 Schematic of the cooperative effect of the molar ratio of NH4OH/La3+ added per minute, temperature, and concentration of NH4Cl on the phase of the product. | |
3.2 Morphologies of La(OH)2Cl
The surface morphologies and crystal structures of the as-prepared La(OH)2Cl were depicted through the TEM images. The TEM images of samples 3–5 are presented in Fig. 4a–c. Noticeably, at an NH4Cl aqueous solution concentration of 2 mol L−1, the morphology of the product consists of rectangular-column and rod-shaped particles. This outcome suggests that the crystallinity is low, and the rod-shaped particles separate from the rectangular-column-shaped particles due to a partial hydrogen bond fracture between the layers. However, the monocrystals have spindle and more complete rectangular-column shapes at concentrations of 3 mol L−1 and 4 mol L−1. Beside, these results also reveal the possible assembly process of the bulk crystalline La(OH)2Cl during this synthesis method. The structural effects of La(OH)2Cl result in the 0D structures undergoing oriented attachment, producing a single elongated structure in the initial stage. During the process of crystal growth, the rod-shaped crystals aggregate into bundles, which gradually evolve into spindle-shaped and then rectangular-column-shaped crystals, mirroring the evolution observed during the synthesis of nanostructured Eu(OH)2Cl from Eu2O3 nanocrystals. Hence, based on the above conclusion, the optimal experimental conditions are as follows: a temperature of 80 °C, a base solution with an NH4Cl concentration of 4 mol L−1, and a molar ratio of NH4OH/La3+ dropped per minute of 3. Moreover, Fig. 5d displays the lattice spacings from the HRTEM image of the local area marked by the white box in Fig. 4a, revealing the highly crystalline quality of La(OH)2Cl. The measured interplanar distance is approximately 0.633 nm, corresponding to the (100) plane of monoclinic La(OH)2Cl. Further, Fig. 4e depicts the selected area electron diffraction (SAED) pattern obtained from the sample in Fig. 5c, indexed to the monoclinic phase of La(OH)2Cl, as confirmed by the XRD data.
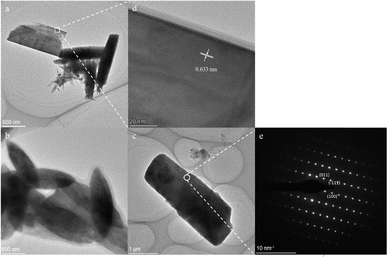 |
| Fig. 4 TEM images of sample 3 (a), sample 4 (b), and sample 5 (c). (d) The HRTEM image of the selected area in figure. (a). (e) SAED pattern of the selected area marked in (c). | |
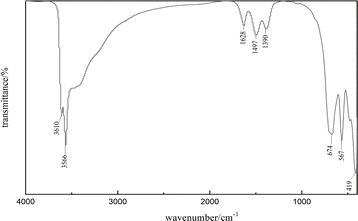 |
| Fig. 5 FT-IR spectra of the as-synthesized La(OH)2Cl (sample 5). | |
3.3 Composition of La(OH)2Cl
Fig. 5 illustrates the use of FTIR spectroscopy for examining the chemical bonding on the surface of the as-synthesized La(OH)2Cl (sample 5). The sharp peaks observed at 3565 cm−1 and 3610 cm−1 are associated with the vibration of O–H bonds in La(OH)2Cl,20 while the broad absorption at about 3500 cm−1 is ascribed to the stretching vibration of water.12 The spectrum displays two absorption peaks of O–H bonds resulting from OH⋯Cl hydrogen bonds. The weak peaks at 1390 cm−1, 1496 cm−1, and 1628 cm−1 correspond to the carbonate species absorbed from the atmosphere.21 Moreover, the band at 674 cm−1 is identified as the stretching vibration of La–O bonds.22,23 Finally, the strong peaks at 419 cm−1 and 567 cm−1 are attributed to the stretching vibration and normal vibrational mode of the La–O bond, respectively.21,24 These results provide strong evidence for the formation of La(OH)2Cl, which is in accordance with the XRD results (Fig. 2a).
Energy dispersive spectroscopy (EDS) analysis was employed to determine the composition of the as-synthesized La(OH)2Cl (sample 5). As shown in Fig. 6, EDS clearly identified that the crystalline substances are composed of Cl, O and La and should therefore be attributed to La(OH)2Cl; H cannot be detected by EDS, thus further confirming the purity of the products. This result is in agreement with the XRD data.
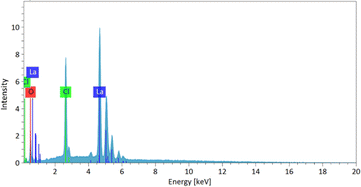 |
| Fig. 6 EDS patterns of as-synthesized La(OH)2Cl (sample 5). | |
3.4 Thermal analysis
Next, the TG curve depicting the thermal transformation of La(OH)2Cl indicates two steps of mass loss (Fig. 7). As shown in Fig. 8a, the XRD pattern of the annealed product at approximately 360 °C is lanthanum oxychloride (LaOCl). The experimental mass loss of nearly 8.68 mass% (La(OH)2Cl to LaOCl) corresponds well with the theoretical values (8.64 mass%). Fig. 8b shows the SEM image of the annealed product. The morphology of LaOCl particles synthesized according to the above steps is a thick platy aggregate. From the TG curve, we also can get that the mass declines slowly from about 480 °C. But there is no obvious peak in the DSC curve between 480 °C and 1500 °C. Lanthanum oxide is expected to form at approximately 480 °C. Increasing the annealing temperature to 1500 °C results in a material with all reflections corresponding to the La2O3 phase (Fig. 8c). From these results, we can infer that the phase transformation of LaOCl to La2O3 is slow. Similar result can be found from Zhu's article.12 The only difference is that pure La2O3 can be obtained when the annealing temperature is increased to 1050 °C. Fig. 8d shows that the morphology of La2O3 is a short rod aggregate.
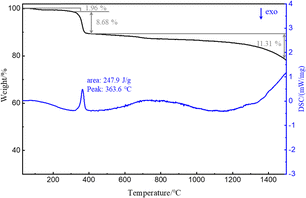 |
| Fig. 7 TG-DSC result of sample 5. | |
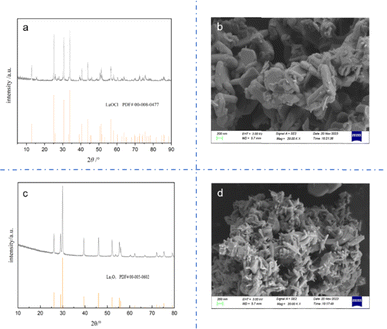 |
| Fig. 8 (a) XRD patterns of the annealed product at approximately 360 °C. (b) SEM image of the annealed product at approximately 360 °C. (c) XRD patterns of the annealed product at 1500 °C. (d) SEM image of the annealed product at 1500 °C. | |
4. Conclusions
To summarize, we explored a reactive crystallization method to synthesize La(OH)2Cl. This method offers the advantages of low-temperature, high yield and short cycle length, making it potentially suitable for industrial applications and representing a novel approach for the low-carbon development of the rare earth metallurgical process. Additionally, we investigated the influence of these main parameters on the product's phase and the importance of controlling them in conjunction to synthesize La(OH)2Cl. The as-synthesized products were characterized using XRD, FTIR, SEM, and TEM techniques. These results showed that the optimal conditions were as follows: the concentration of NH4Cl in the base solution was 4 mol L−1 and the reaction system was 80 °C. At the same time, the molar ratio of NH4OH/La3+ dropped per minute should be controlled to be 3
:
1. Then, the thermal dehydration of La(OH)2Cl was investigated using the DSC/TG studies. It showed that LaOCl could be obtained at about 360 °C. The phase transformation of LaOCl to La2O3 shows slow progress and it starts at about 500 °C. From the SEM images of the annealed products, La(OH)2Cl synthesized by this method offers the possibility of becoming a precursor in the preparation of special morphology LaOCl and La2O3.
Data availability
The data that support the findings of this study are available from the corresponding authors (Jincheng Guo and Xihong Hao), upon reasonable request.
Conflicts of interest
There are no conflicts to declare.
Acknowledgements
We thank the support from the grants from the National Natural Science Foundation of China (no. 51634005) and the Natural Science Foundation of Inner Mongolia Autonomous Region (no. 2024MS2019). We also thank China Northern Rare Earth (Group) High-Tech Co., Ltd for the aqueous solution of lanthanum chloride from hydrometallurgical process.
References
- R. J. Liu, Z. H. Zhao, X. Y. Sang, Z. Z. Zhang, D. Wei, G. G. Zhang, W. B. Zhang and Y. Gao, Study on Preparation of Lanthanum Carbonate with Mixed Precipitation Agent of Ammonium Bicarbonate and Ammonia, Rare Met. Cem. Carbides, 2017, 45, 51 Search PubMed.
- J. C. Guo, H. Tian, X. Y. Wang, X. J. Zhang, Y. H. Zhang and L. X. Yu, Preparation of Lanthanum Carbonate with Mixed Precipitation Agent and Its Precipitation Crystallization Process, J. Chin. Soc. Rare Earths, 2023, 41, 1126 Search PubMed.
- J. K. Zhang, S. L. Zheng, P. Li, Y. N. Yang, X. D. Guan, J. Y. Zhou and D. Y. Chen, Preparation of High Quality Lanthanum Carbonate and Lanthanum Oxide by Composite Precipitant, J. Chin. Soc. Rare Earths, 2022, 40, 624 Search PubMed.
- B. Chris, A. Max, N. Karsten, J. N. Lars, F. Manfred, L. Stefan, S.-R. Baltazar, D.-R. Amandine, S. Seton, W. Henri, S. Oliver and R. Shahrzad, A review of technology and policy deep decarbonization pathway options for making energy-intensive industry production consistent with the Paris agreement, J. Cleaner Prod., 2018, 187, 960 CrossRef.
- M. A. Quader, S. Ahmed, S. Z. Dawal and Y. Nukman, Present needs, recent progress and future trends of energy-efficient ultra-low carbon dioxide (CO2) steelmaking (ULCOS) program, Renewable Sustainable Energy Rev., 2016, 55, 537 CrossRef.
- T. T. Xu, X. D. Zheng, B. Ji, Z. H. Xu, S. F. Bao, X. Zhang, G. M. Li, J. F. Mei and Z. Y. Li, Green recovery of rare earth elements under sustainability and low carbon: a review of current challenges and opportunities, Sep. Purif. Technol., 2024, 330, 125501 CrossRef CAS.
- S.-S. Lee, C.-H. Joh and S.-H. Byeon, Highly enhanced blue-emission of LnOCl:Tm prepared by dehydration of Ln(OH)2Cl:Tm (Ln = La and Gd), Mater. Sci. Eng., B, 2008, 151, 163 CrossRef CAS.
- F. L. Carter and S. Levinson, The Hydrothermal Preparation, Single-Crystal Lattice Parameters, Decomposition Data for Some Lanthanide Dihydroxy Chlorides the Related Hydroxy Chloride, Yb3O(OH)5Cl2, Inorg. Chem., 1969, 8, 2788 CrossRef CAS.
- R. F. Klevtsova and L. A. Glinskaya, The Crystal Structure of Pr(OH)2Cl, Sm(OH)2Cl, and Gd(OH)2Cl, J. Struct. Chem., 1969, 10, 408 CrossRef.
- S. G. Podkolzin, E. E. Stangland, M. E. Jones, E. Peringer and J. A. Lercher, Methyl Chloride Production from Methane over Lanthanum-Based Catalysts, J. Am. Chem. Soc., 2007, 129, 2569 CrossRef CAS PubMed.
- A. W. A. M. van der Heijden, V. Bellière, L. E. Alonso, M. Daturi, O. V. Manoilova and B. M. Weckhuysen, Destructive Adsorption of CCl4 over Lanthanum-Based Solids: Linking Activity to Acid-Base Properties, J. Phys. Chem. B, 2005, 109, 23993 CrossRef CAS PubMed.
- X. R. Zhu, L. J. Hope-Weeks, D. Ramirez, R. Baghi, V. R. Charles and Y. J. He, Controllable decomposition of lanthanum oxychloride through different annealing conditions, J. Alloys Compd., 2019, 800, 29 Search PubMed.
- Z. W. Shi, X. Zhang, Y. H. Zhao, J. Y. Wang and G. C. Zhu, Kinetics on Chlorination Process of La2O3 and CeO2 by Ammonium Chloride, Chin. J. Process Eng., 2005, 5, 23 Search PubMed.
- C. Jouve, J. Marrot and D. Riou, β-Sm(OH)2Cl: a new lamellar variety, Acta Crystallogr., 2002, 58, 14 Search PubMed.
- S. V. Mahajan, J. Hart, J. Hood, A. Everheart, M. L. Redigolo, D. S. Koktysh, E. A. Payzant and J. H. Dickerson, Synthesis of RE(OH)2Cl and REOCl (RE = Eu, Tb) nanostructures, J. Rare Earths, 2008, 26, 131 CrossRef.
- H. Kim, M. Kim and S. H. Byeon, Ce4+/Ce3+ redox-controlled luminescence “off/on” switching of highly oriented Ce(OH)2Cl and Tb-doped Ce(OH)2Cl, J. Mater. Chem. C, 2017, 5, 444 RSC.
- N. Soto-Donoso, L. Favier, S. F. Villalobos, V. Paredes-García, T. Bataille, J. F. Marco, R. M. Hlihor, E. L. Fur and D. Venegas-Yazigi, Lanthanum hydroxychloride/anatase composite and its application for effective UV-light driven oxidation of the emergent water contaminant cetirizine, Chem. Eng. Res. Des., 2003, 196, 685 CrossRef.
- A. J. A. Dunn, J. W. Annis, J. M. Fisher, D. Thompsett and I. R. Walton, Ce(OH)2Cl and lanthanide-substituted variants as precursors to redox-active CeO2 materials, Dalton Trans., 2020, 49, 14871 RSC.
- M. Fellner, A. Soppelsa and A. Lauria, Heat-Induced Transformation of Luminescent, Size Tuneable, Anisotropic Eu:Lu(OH)2Cl Microparticles to Micro-Structurally Controlled Eu:Lu2O3 Microplatelets, Crystals, 2021, 11, 992 CrossRef CAS.
- M. Mousavi-Kamazani, S. Alizadeh, F. Ansari and M. Salavati-Niasari, A controllable hydrothermal method to prepare La(OH)3 nanorods using new precursors, J. Rare Earths, 2015, 33, 425 CrossRef CAS.
- N. Imanak, T. Masui and Y. Kato, Preparation of the cubic-type La2O3 phase by thermal decomposition of LaI3, J. Solid State Chem., 2005, 178, 395 CrossRef.
- J. Feng, X. H. Li, M. Z. Wang, X. L. Zheng, J. T. Bai, L. Wang and Y. Peng, One-pot, template-free synthesis of hydrophobic single-crystalline La(OH)3 nanowires with tunable size and their d0 ferromagnetic properties, RSC Adv., 2015, 5, 16093 RSC.
- Y. Chen, Q. Qian, X. Liu, L. Xiao and Q. Chen, LaOCl nanofibers derived from electrospun PVA/lanthanum chloride composite fibers, Mater. Lett., 2010, 64, 6 CrossRef CAS.
- S.-S. Lee, H.-I. Park, C.-H. Joh and S.-H. Byeon, Morphology-dependent photoluminescence property of red-emitting LnOCl:Eu (Ln = La and Gd), J. Solid State Chem., 2007, 180, 3529 CrossRef CAS.
Footnotes |
† Electronic supplementary information (ESI) available. See DOI: https://doi.org/10.1039/d4ra03987d |
‡ H. Tian and J. C. Guo contributed to the work equally and should be regarded as cofirst authors. |
|
This journal is © The Royal Society of Chemistry 2024 |
Click here to see how this site uses Cookies. View our privacy policy here.