DOI:
10.1039/D4RA04168B
(Paper)
RSC Adv., 2024,
14, 29860-29872
Influences of topography on nitrate export from forested watersheds on Yakushima Island, a Natural World Heritage site†
Received
7th June 2024
, Accepted 3rd September 2024
First published on 19th September 2024
Abstract
In East Asia, high levels of atmospheric nitrogen are deposited onto land. This could elevate the nitrate levels in coastal waters via river runoff, even from areas where anthropogenic sources are minimal. It is important to identify NO3− sources in river water and the mechanisms involved in NO3− runoff. Yakushima Island, Japan, is a Natural World Heritage site featuring numerous watersheds with diverse topography and rivers. The area receives significant precipitation, with up to 10
000 mm in mountainous regions. Its proximity to coastal urban areas in China (∼800 km) leads to substantial atmospheric nitrogen wet and dry deposition in the island's forests. The study aimed to clarify regional water quality characteristics by conducting long-term monitoring of dissolved ion components (Na+, K+, Mg2+, Ca2+, F−, Cl−, NO3−, and SO42−) in river waters, and to determine the effects of NO3− sources and watershed topography on NO3− behavior. Dissolved ion concentrations were obtained from a long-term monitoring (2011–2014) dataset. Cluster analysis classified runoff water from the central mountainous region into three groups: western region, other regions, and groundwater. The average NO3− concentration in the western region was 10.2 μmol L−1, which was higher than the 6.24 μmol L−1 observed in the other regions. Stable isotope analysis in December 2018 showed that river water NO3− (1.39 μmol L−1) in the western region had a high proportion of atmospheric NO3−. Topographic analysis indicated that NO3− and atmospheric NO3− increased in smaller watersheds and steeper terrain. This study conclude that NO3− output is controlled by topography.
1 Introduction
Yakushima Island has a rich natural forest and is a Natural World Heritage site, but there have long been concerns about acidification of the rivers on the island. The island experiences acid wet deposition levels of 43.2 and 35.4 mmol m−2 per year (nss-SO42− and NO3−, respectively), which are significantly higher than those recorded in major cities in Japan (e.g., Tokyo: 17.1 and 25.6 mmol m−2 per year).1 Soils and rivers on the island continue to be affected by groundwater and stream water acidification,2,3 decreasing acid buffering capacities of soils in watersheds,4,5 and the effects of long-term acid deposition from the atmosphere.6 Precipitation on Yakushima Island contains large amounts of sea salt and anthropogenic S- and N-containing compounds.7 The principal cause of acidification is air pollution from continental China, located northwest of the island across the East China Sea.8–10 The transport pathways of pollutants in air mean that the northwest of the island is more affected by air pollution than the southeast.3,11
In unmanaged forest, atmospheric nitrogen deposition accounts for the majority of nitrogen input to the forest nitrogen cycle. Mountain forest ecosystems on islands are more sensitive than inland mountain forest ecosystems to atmospheric deposition. Mountain ecosystems are limited by the supply of N for long periods,12 thus they are particularly sensitive to atmospheric deposition of N.13–15 More N is deposited at high elevations, such as in mountainous areas, than at low elevations.16 Islands with limited ecosystem resources17 are vulnerable to external factors such as N deposition.18 Pristine natural environments tend to have low N inputs, and organisms use limited N.19,20 N deposition from the atmosphere will, therefore, strongly affect pristine natural environments.15,21,22
Anthropogenic emissions of reactive N have dramatically increased the amount of available nitrogen in global ecosystems. Nitrate (NO3−) is water-soluble and one of the most important nutrients for primary production in the aquatic environment. Excess NO3− in river water can cause eutrophication, which can lead to severe ecological and economic problems downstream, such as in lakes, estuaries, and oceans.23–25 Nitrogen oxide concentrations in East Asia have increased rapidly, peaking in approximately 2010, and have since begun to decrease, but atmospheric nitrogen oxide concentrations remain high.26 Nitrogen oxides affect the nitrogen cycle in leeward forests and oceans, regardless of national borders.27,28
In forests with high levels of nitrogen deposition, stream water NO3− is elevated, typically ranging between 100 and 200 μ mol L−1,29,30 providing a nitrogen source for downstream ecosystems.27,31 Interestingly, mountainous islands, even those without direct anthropogenic nitrogen sources, can exhibit high NO3− concentrations in river water due to atmospheric deposition.32 Understanding the mechanisms behind nitrate runoff from forested areas that are free from direct human pollution will provide valuable insights for managing downstream nitrogen levels.
In previous studies, the sources of NO3− have been identified by analyzing nitrogen and oxygen stable isotope ratios (δ15N and δ18O).33,34 Determining δ17O also allows a clear distinction to be made between NO3− deposited from the atmosphere (NO3−atm) and NO3− produced through biological processes (NO3−re).35,36 Δ17O analysis is a powerful tool for investigating the N cycle in forested areas, and has been used to indicate nitrogen saturation,37 N cycling in the European Alps,38 and N cycling in forests.37–45 Furthermore, forested streams with high NO3− concentrations in the stream water have higher Δ17O because atmospheric nitrate is not incorporated into the forest nitrogen cycle, but instead flows out.37,46
The aim of this study was to identify the sources of dissolved NO3− in rivers in forests on Yakushima Island, where most of the N input comes from atmospheric deposition or nitrification. There are many rivers in forested areas with various topographies, meaning that the residence times in different catchments are likely to be different. We identified which rivers were sensitive to atmospheric deposition of nitrogen. First, rivers were classified by region using the results of long-term sampling and analyses of dissolved ionic components. Next, the sources of NO3− in river water in the winter were identified and the influence of the topography of each catchment on the sources of NO3− in river water in the catchment was investigated. We hypothesized that because differences in vegetation are less likely to affect river water quality in winter, more NO3− of atmospheric origin will be deposited into the river water, which is clearly correlated with the topography (especially residence time).
2 Materials and methods
2.1 Site description
Yakushima Island (30.34°N, 130.51°E) is ∼800 km east of Shanghai (China) and ∼60 km south–southwest of Cape Sata in Kagoshima (Japan), and separates the East China Sea and the Pacific Ocean (Fig. 1). The island is circular and has an area of ∼504 km2, with 10 mountain ranges in the center (the Central Mountains Group) with elevations of 1800 m or more, including the highest peak in Kyushu, Mount Miyanoura (1935 m), and 46 mountain ranges47 with elevations of ∼1000 m around the island's edges. The island has subtropical to subarctic climate zones from coast to summit, which is unusual at the latitude of the island. Warm air (from warm oceanic currents) containing large amounts of water vapor rises over the mountains, resulting in large amounts of precipitation. Annual precipitation is ∼4500 mm−1 on the plains and >10
000 mm−1 in the mountains.48 This is one reason why Yakushima Island is also called “the Alpine mountains of the sea”. Yakushima Island is very biodiverse and has a unique range of ecosystems adapted to the humid environment. There are many Yaku cedar (Cryptomeria japonica), which are rare elsewhere in the world and can live for thousands of years. These factors led to ∼20% of Yakushima Island becoming a Natural World Heritage site in 1993.49 The western part of the island has particularly rugged terrain and is a Natural World Heritage site from the coastline to the mountain peaks.50 No people live in the western region and the landscape is forested. In other regions, habitable areas are concentrated in a few flat areas downstream near the coast. Therefore, by selecting the rivers and points to be surveyed, it was possible to eliminate the influence of anthropogenic pollution sources such as domestic wastewater. The sources of nitrogen input to the watershed could be investigated in watersheds where atmospheric nitrogen deposition and nitrogen fixation in the forest were the only sources of nitrogen input.
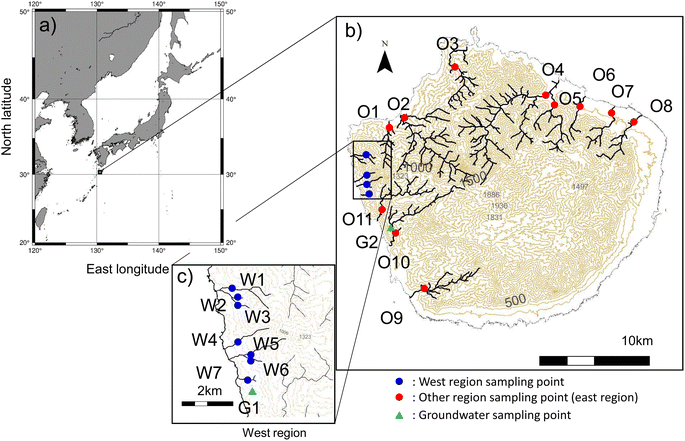 |
| Fig. 1 Maps showing: (a) the location of Yakushima Island, (b) the main rivers in forested areas and groundwater sampling sites, and (c) the rivers in the western region of Yakushima Island from which samples were collected. Blue circles indicate rivers in the western region, red circles indicate rivers in other regions, and green triangles indicate groundwater. | |
This western region has many streams, the headwaters of which are on Mount Kuniwari (1323 m above sea level), only 2 km from the coast. In regions other than the western region, rivers flow from the Central Mountain Range Group to and through the surrounding mountains. Groundwater emerge from many rocky crevices. Samples were collected from 22 sites on the main rivers, shown in Fig. 1. In this study, sampling was carried out on clear days. When it rains in the western region, the roads are closed in case of flooding, making it impossible to carry out surveys. In this area, bridges often wash away, making it unviable to travel around the island. It is dangerous and impossible to conduct surveys at times when it is raining and NO3−atm levels are likely to be high. When we were able to get close to a river, we filled a bottle with river water to collect samples. When we were unable to get close to a river, we used a bucket to collect a river water sample from a bridge. The bucket was lowered into the middle of the river where the water was thought to be well mixed. We grab sampled water from rivers around Yakushima Island over a day of travel. Water samples were collected, and field observations were made along the major rivers at points where no anthropogenic pollution occurred (Table 1). To select the sampling sites, we used GIS to calculate the land use area of the watershed and confirmed on-site that the land use area upstream was forest. The study period was January 2011 to March 2014. Twice monthly samples were collected from the main rivers on Yakushima Island. The surveys conducted during this period aimed to clarify the trends in ionic components of river water in Yakushima Island. An additional study was performed on 19 December 2018 to identify the sources of NO3− in river water during the winter. In this survey, stable isotope ratios were used to estimate the source of NO3− in river water.
Table 1 Land use ratio and topography indicators for the study area. TWI is the topographic wetness index. TWI was calculated using eqn (3). The mean TWI is the average TWI of each watershed
|
|
Forest (%) |
Agricultural land (%) |
Urban (%) |
Area (km2) |
Mean altitude (m) |
Mean slope (°) |
Mean TWI |
Western region |
W1 |
100.0 |
0.0 |
0.0 |
1.94 |
569 |
33.2 |
4.88 |
W2 |
100.0 |
0.0 |
0.0 |
0.29 |
489 |
33.4 |
4.72 |
W3 |
100.0 |
0.0 |
0.0 |
0.29 |
522 |
33.0 |
4.72 |
W4 |
100.0 |
0.0 |
0.0 |
1.14 |
596 |
39.0 |
4.68 |
W5 |
100.0 |
0.0 |
0.0 |
1.44 |
740 |
39.4 |
4.68 |
W6 |
100.0 |
0.0 |
0.0 |
0.14 |
468 |
34.5 |
4.80 |
W7 |
100.0 |
0.0 |
0.0 |
0.36 |
530 |
32.9 |
4.88 |
Other regions |
O1 |
99.5 |
0.5 |
0.0 |
7.06 |
551 |
29.0 |
5.00 |
O2 |
99.3 |
0.1 |
0.0 |
27.93 |
827 |
34.1 |
4.83 |
O3 |
100.0 |
0.0 |
0.0 |
10.54 |
537 |
27.0 |
5.06 |
O4 |
100.0 |
0.0 |
0.0 |
37.16 |
747 |
32.5 |
4.92 |
O5 |
100.0 |
0.0 |
0.0 |
12.81 |
684 |
29.0 |
5.03 |
O6 |
98.0 |
2.0 |
0.0 |
3.70 |
496 |
28.6 |
5.09 |
O7 |
98.7 |
1.3 |
0.0 |
5.64 |
528 |
30.7 |
5.01 |
O8 |
97.5 |
2.5 |
0.0 |
7.62 |
406 |
26.8 |
5.23 |
O9 |
98.3 |
1.5 |
0.2 |
11.82 |
535 |
26.0 |
5.15 |
O10 |
100.0 |
0.0 |
0.0 |
12.07 |
820 |
25.9 |
5.18 |
O11 |
100.0 |
0.0 |
0.0 |
12.36 |
928 |
25.4 |
5.18 |
2.2 Chemical analysis
After collection, each river or groundwater sample was transported to the laboratory and immediately passed through a 0.45 μm membrane disc filter (DISMIC-25CS; Advantec, Tokyo, Japan) and then stored at 4 °C in a dark place. Dissolved ions were determined using a Compact IC 761 ion chromatograph (Metrohm, Herisau, Switzerland). The main dissolved ions (Na+, K+, NH4+, Mg2+, Ca2+, Cl−, NO3−, and SO42−) were determined. The measurement errors were ±4.22 μmol L−1 for Na+, ±3.90 μmol L−1 for K+, ±2.61 μmol L−1 for Mg2+, ±2.37 μmol L−1 for Ca2+, ±6.89 μmol L−1 for Cl−, ±2.31 μmol L−1 for NO3−, and ±3.14 μmol L−1 for SO42−. The NH4+ concentrations in the samples were <2.00 μmol L−1.
The Δ17O, δ18O, and δ15N values for NO3− in the water samples collected in December 2018 were determined after converting the NO3− into N2O using the cadmium reduction method.51–53 Oxygen isotopes in NO3− were determined if the NO3− concentration in a sample was >0.8 μmol L−1. The N2O produced was introduced into a gold wire oven (Agilent 6890; Agilent Technologies, Santa Clara, CA, USA) held at 780 °C to pyrolyze N2O to give N2 and O2. The O2 produced was then analyzed to give the 16O, 17O, and 18O isotope composition using a Finnigan MAT252 isotope ratio mass spectrometer (Thermo Fisher Scientific, Waltham, MA, USA).54,55 The instrument was calibrated using international nitrate standards (USGS-34 and USGS-35).45,52,56 The measurement errors for the samples were ±0.38‰ for δ15N, ±0.5‰ for δ18O, and ±0.2‰ for Δ17O.
2.3 Nitrate in the atmosphere calculations
NO3−re generated through nitrification is produced from decomposing organic matter and the fixation of the released nitrogen. Most of the NO3−atm produced in the atmosphere is generated through photochemical reactions between atmospheric NO and O3. The NO3−atm formation process can be characterized from anomalies in 17O enrichment.36,57 NO3−, including NO3−atm, is produced through an assimilation, decomposition, and nitrification cycle and is converted into NO3−re. The Δ17O–NO3− value (the magnitude of the excess 17O produced in the atmosphere) was calculated using eqn (1),58 which was used to distinguish between NO3−atm (Δ17O > 0‰) and NO3−re (Δ17O = 0‰).37 |
Δ17O = ((1 + δ17O)/(1 + δ18O)β) − 1
| (1) |
The value of β was 0.5279.58 Eqn (2) was used to calculate the ratio between the contributions of atmospheric nitric acid and regenerative nitric acid to the NO3− concentrations in the river and stream water samples.
|
NO3−atm/NO3−in river = Δ17Oin rain/Δ17Oin river
| (2) |
This allowed Δ17O–NO3− for stream water to be calculated. The Δ17O–NO3− value for precipitation in the Northern Hemisphere is +26.6 ± 0.9 (std) ‰.36,57 The Δ17O–NO3− values for NO3− in precipitation in December 2018 on Yakushima Island were +23.0‰ (16 December 2018) and +28.3‰ (19 December 2018), which supported the Δ17O values of nitrate in precipitation reported in previous studies (Table 2). The NO3−atm concentration could be calculated from eqn (2), thus the NO3−re concentration could be calculated by subtracting the NO3−atm concentration from the NO3− in stream water concentration.
Table 2 NO3− concentrations and stable isotope ratios in precipitation during the survey period 16–19 December 2019
Sampling date |
NO3− (μmol L−1) |
δ15N (‰) |
δ18O (‰) |
Δ17O (‰) |
16 December 2018 |
11.3 |
−2.94 |
74.23 |
17.98 |
19 December 2018 |
15.83 |
−0.03 |
90.81 |
22.26 |
2.4 Topographic wetness index analysis
In rugged terrain, such as in mountainous areas, each catchment will have a complex topography with various gradients. Detailed analysis taking spatially heterogeneous landforms into consideration can shed light on complex bio-geoscientific processes that occur over large areas in such terrain.59,60 The catchment area, mean catchment slope, and topographic wetness index (TWI) were used as indicators of water retention related to the catchment topography (Table 1). The TWI indicates ponding of surface runoff and local variations in the groundwater table in TOPMODEL, which is a widely used precipitation runoff model for the small watershed scale, such as for watersheds in forests.61 Locations with the same TWI value respond hydrologically in the same way and are, therefore, hydrologically similar. The TWI is also used to investigate the N cycle through identifying hydrologically similar areas.62–66 Using the TWI value, it is possible to quantify the similarity of residence times between sampling watersheds. The catchment area, slope, and topographic wetness index (TWI) for each study site were calculated using ArcGIS (version 10.4.1) using data from a digital elevation model67 with a resolution of 20 m × 20 m. The TWI was calculated using eqn (3).63,68 |
TWI = ln(α/tan β)
| (3) |
In eqn (3), α is the upstream watershed area per contour unit discharged from a given point and β is the slope at a given point. The digital elevation model data had a resolution of 20 m × 20 m. One value was calculated for each 20 m × 20 m square. The TWI for each sampling site was calculated by calculating the mean of several TWIs for 20 m × 20 m squares in the catchment.
2.5 Statistical processing
Statistical processing involved calculating K-means, Welch's t test and performing linear regression analyses using R (version 4.1) software. The K-means results were classified using cluster,69 which is an open source library in R. The K-means were calculated by performing 500 iterations using the Hartigan–Wong algorithm.
3 Results and discussion
3.1 Characteristics of Yakushima Island river water determined by long-term monitoring
The Na+, K+, Mg2+, Ca2+, F−, Cl−, NO3−, and SO42− concentrations found in the 4-year long-term samples of all river water and groundwater were 340 ± 148, 12.3 ± 6.81, 36.8 ± 23.5, 27.4 ± 19.7, 2.57 ± 2.79, 312 ± 151, 7.56 ± 7.21, and 49.3 ± 20.3 μmol L−1, respectively (Fig. S1†). Yakushima Island is surrounded by the sea, thus the river water contains many components derived from sea salt.6 These ionic components change significantly depending on the season. The dissolved ion analysis results were subjected to cluster analysis, which divided the major rivers and groundwater into three groups (Fig. 2). Component 1 contained the Na+, K+, Ca2+, Mg2+, F−, Cl−, NO3−, and SO42− components, and as the component value increased, the concentration of each ionic component increased. Component 2 contained the F− and NO3− components, and as the component value increased, the F− concentration increased, but the NO3− decreased. Ground water tended to have a higher rock content owing to its longer residence time, and the sea salt content tended to be higher in the western region closer to the coast compared with other regions. The cluster analysis results are shown in Table 3. Group 1 contained “other regions” (n = 469), group 2 contained the western region (n = 575), and group 3 contained groundwater (n = 103). These groups together accounted for ∼90% of the samples (Table 3). There were 0 samples in groundwater classified as group 1, and 1 sample in other regions classified as group 3. This demonstrated that the other regions and groundwater had different ionic compositions. The water quality of rivers on Yakushima Island was found to be more affected by differences between the survey sites than by seasonal changes. In the next section, we identify trends for the different ions in the three groups (other regions, the western region, and groundwater).
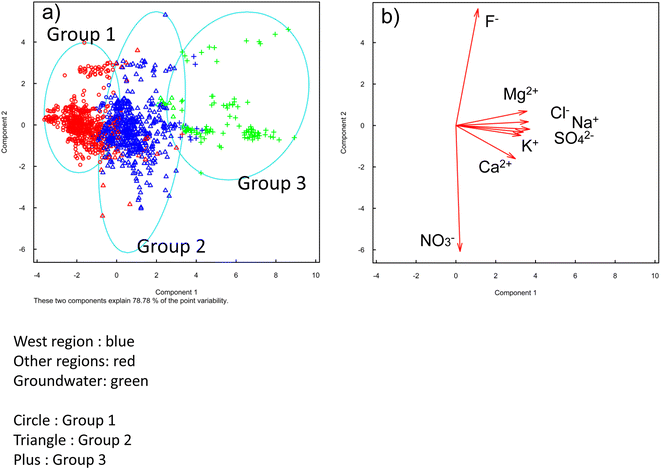 |
| Fig. 2 Results of principal component analysis of the main ionic components determined in the long-term monitoring (2011–2014) study with two explanatory variables. (a) Sample components are shown in blue for the western region, red for the other regions, and green for groundwater. K-Means classification resulted in three classes (plus symbols: group 1, triangles: group 2, and circles: group 3). (b) The eight ion components Na+, K+, Ca2+, Mg2+, F−, Cl−, NO3−, and SO42− are grouped into two components. Components 1 and 2 explained 79% of the ionic components. | |
Table 3 Matrix of the K-means classification results shown in Fig. 2. The K-means were used to divide the ionic components of groundwater in the western and eastern regions of Yakushima Island into three classes, and the number of samples in each class is shown
|
Group 1 |
Group 2 |
Group 3 |
Western region |
28 |
469 |
13 |
Other regions |
575 |
41 |
1 |
Groundwater |
0 |
11 |
103 |
The concentrations of ions in the other regions, western region, and groundwater are shown in Table 4. The dissolved ion concentrations except the NO3− concentration decreased in the order groundwater, western region, and other regions. The NO3− concentration decreased in the order western region (10.2 ± 8.39 μmol L−1), other regions (6.24 ± 5.74 μmol L−1), and groundwater (3.31 ± 3.57 μmol L−1) (Fig. 3 and S1†). The Na to Cl ratio was 1.20 for other regions, 1.04 for the western region, and 1.14 for groundwater, with the ratio for the western region being significantly lower than the ratios for the other groups (p < 0.001) (Fig. 3a). Yakushima Island is composed of uniform granite except in coastal areas, and differences between dissolved ion concentrations at the different study sites were not considered to be caused by differences in the compositions of the base rocks.9
Table 4 Major ionic component concentrations determined in the long-term monitoring study (2011–2014)
|
Na+ (μmol L−1) |
K+ (μmol L−1) |
Mg2+ (μmol L−1) |
Ca2+ (μmol L−1) |
Cl− (μmol L−1) |
NO3− (μmol L−1) |
SO42− (μmol L−1) |
Western region |
392 ± 72.9 |
12.5 ± 3.03 |
41.6 ± 11.9 |
29.8 ± 13.3 |
380 ± 86.4 |
10.2 ± 8.39 |
62.1 ± 7.03 |
Other regions |
236 ± 58.7 |
9.59 ± 3.32 |
23.1 ± 9.72 |
18.1 ± 11.2 |
200 ± 55.3 |
6.24 ± 5.74 |
31.5 ± 6.73 |
Groundwater |
683 ± 95.7 |
24.6 ± 7.99 |
89.7 ± 30.9 |
67.8 ± 25.7 |
614 ± 137 |
3.31 ± 3.57 |
88.2 ± 8.35 |
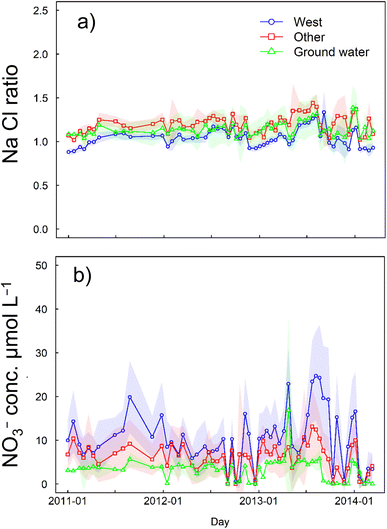 |
| Fig. 3 (a) Na Cl ratios and (b) NO3− concentrations in each area determined in the long-term monitoring study. Blue lines indicate rivers in the western region, red lines indicate rivers in the other regions, and green lines indicate groundwater. | |
The NO3− concentrations in the samples collected between 2011 and 2014 were similar to concentrations found between 1996 and 2011 (11.2 ± 3.09 μmol L−1 for other regions and 14.6 ± 5.16 μmol L−1 in the western region, as shown in Fig. S2†) and in a study performed in 2001 (14.6 ± 5.16 μmol L−1 for the western region and 11.2 ± 3.09 μmol L−1 for other regions).6 A survey conducted in December 2023 still showed high values in the western region (17.6 ± 8.00 μmol L−1 for the western region and 12.8 ± 11.8 μmol L−1 for other regions). We collected samples from rivers in catchment areas >97% covered by forest (Table 1). Agriculture land was only found in some places, with an area of less than 0.2 km2. Field investigations confirmed that the drainage channels did not flow into the rivers at the study sites. Furthermore, no agricultural land and urban areas were found in the western region where NO3− was high. The contribution of anthropogenic NO3−, e.g., from agriculture, was therefore considered to be negligible. The main sources of NO3− in river water were thought to be atmospheric deposition (NO3−atm) and organic matter decomposed by forest organisms (NO3−re), because sea salt contains little NO3−.
The amount of wet nitrogen deposition (NO3− and NH4+) from the atmosphere observed during the survey period is shown in Fig. S4.† The annual average value from 2011 to 2014 was 9.46 ± 1.76 kg N ha−2 per year, and deposition tended to be lower in the summer (0.364 ± 0.276 kg N ha−2 month−1 from July to September). This value is similar to that of Fukuoka City in Kyushu, Japan (9.7 kg N ha−2 per year),46 which is relatively close to Yakushima Island. High NO3− are observed in forest river water around Fukuoka City.27 Such high NO3− in forest river water is commonly observed in suburban forests in the United States,42,70 Japan,29,30 and China.39,71 In forest rivers with a large amount of nitrogen atmospheric deposition, NO3− in river water tended to be high (120 μmol L−1),29 but on Yakushima Island it was very low (14.6 or 11.2 μmol L−1). The annual rainfall in Fukuoka is 1600 mm, while that in Yakuhsima is 4000 mm. This large difference in rainfall had a significant impact on deposition and NO3− in river water.
The NO3− concentration tended to be higher in the western region than the other regions because of enrichment caused by atmospheric fallout in the western region. Higher non-sea-salt-derived SO42− and NO3− concentrations originating in continental Asia were found in the western region than the other regions.3 The topography of the western region causes atmospherically transported substances to accumulate. The terrain in the western region causes fog to form because the mountains are close to the sea and the slopes are covered with forests. Air rich in water vapor rises up the mountain slopes because of valley winds and fog and mizzle forms when the dew point is reached. Pollutants are deposited in relatively large amounts through dry deposition, particularly in winter when fog tends to form.6 Atmospheric components tend to dissolve more readily in fog and mizzle than rain because fog and mizzle droplets have large surface areas.72,73 Fog is an important nitrogen deposition pathway in forest ecosystems, particularly in the canopy.74 Similarly, the NO3− concentration tended to be markedly higher in western Yakushima Island, where fog was more likely to occur, than other areas. The Na and Cl ratios for river water from the western region suggested that atmospheric deposition was an important source of various components. The western region is strongly affected by anthropogenic pollutants in winter because of the prevailing westerly winds.9 It is, therefore, very likely that river water in the western region contains large amounts of NO3− derived from atmospheric nitrogen, particularly in winter. We investigated the source of NO3− in the rivers in the forests of Yakushima Island in winter using stable isotope ratios.
3.2 NO3− concentrations and stable isotope ratios in major rivers in winter 2018
The NO3− concentrations in the river water samples collected in December 2018 are shown in Fig. 4a. The NO3− concentrations in river water from the other and western regions were 14.2 and 19.1 μmol L−1, respectively. Similar to the concentrations found between 1996 and 2014 (Fig. S2†), the NO3− concentration was higher in the western region than other regions. The NO3− concentration in groundwater was 10.3 μmol L−1. NO3− concentrations of 11.3 and 15.8 μmol L−1 were found in precipitation during the study period (Table 2). The concentrations of other ions followed similar trends from 2011 to 2014 (Fig. S3†).
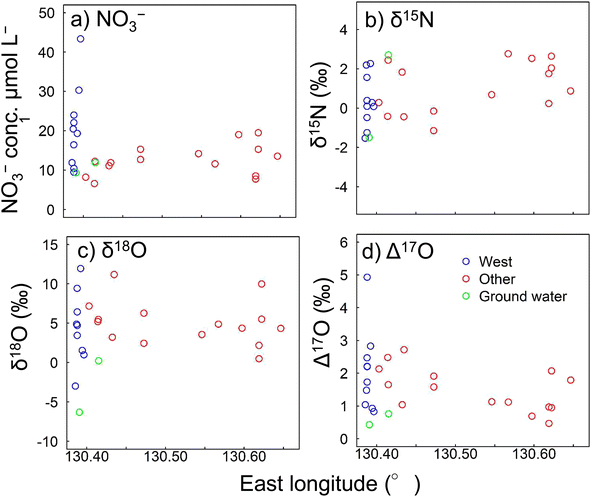 |
| Fig. 4 (a) NO3− concentrations, (b) δ15N values, (c) δ18O values, and (d) Δ17O values found in December 2018. Δ17O was calculated using eqn (1). | |
The δ15N, δ18O, and Δ17O values for NO3− sampled in December 2018 are shown in Fig. 4b–d, respectively. Δ17O was calculated by eqn (1). The δ15N values ranged from −1.15‰ to +3.48‰ in other regions, −1.53‰ to +2.27‰ in the western region, and −1.50‰ to +2.70‰ in groundwater (Fig. 4b). The δ18O values ranged from +0.49‰ to +11.2‰ in other regions, −2.98‰ to +21.2‰ in the western region, and −6.30‰ to +0.23‰ in groundwater (Fig. 4c). These values were within the same ranges as previously found for mountainous areas.75–77 The Δ17O values ranged from +0.7‰ to +2.7‰ in other regions, +0.8‰ to +4.9‰ in the western region, and +0.4‰ to +0.8‰ in groundwater (Fig. 4d). Sampling in this study was conducted on clear days. Therefore, it is possible that storm flow, immediately after rain, could produce an outflow of approximately four times as much NO3−. Furthermore, it is possible that rainfall could increase the Δ17O by five times.78
We next investigated the sources of NO3− in river water in December 2018 using these three stable isotope ratios. The annual mean temperature on Yakushima Island was 20 °C, but the monthly mean temperature for December was 13.7 °C. Microbial activity becomes slower in winter, thus the amount of NO3− supplied to river water through microbial metabolism (NO3−re) decreases.79,80 During periods of winter-type atmospheric pressure patterns, air that has passed over continental Asia reaches Yakushima Island and NO3− derived from the atmosphere (NO3−atm) can be a strong source of NO3− in river water.
The Δ17O values in the rivers in December 2018 were +0.5‰ to +4.9‰, which were comparable with the values previously found for natural surface water (−1.4‰ to +6.8‰).36,42,52,57,78,81 Tsunogai et al.36 studied a mountainous island similar to Yakushima Island, but in a more northern area. NO3−, δ15N and Δ17O showed similar values to other regions, but δ18O was higher on Yakushima Island. Molecular oxygen from the surrounding water also affects the δ18O of oxygen molecules in NO3−.82,83 The amount of precipitation on Yakushima Island is about 10 times that of previous survey site, which is likely to reflect precipitation from river water. The Δ17O in the western region was close to the highest values. Ding et al.46 observed atmospheric deposition of 9.7 kg N ha−1 per year, which is one of the highest in forested areas in Japan, and Yakushima Island showed a similar nitrogen deposition amount (9.3–11.2 kg N ha−1 per year). However, while the stream water in the previous study46 contained high NO3− (110 μmol L−1), the level in the western region was only about one-third that of the previous study. The contributions of NO3−re to total NO3− were 81.3–98.2% for river water and 97.1–98.4% for groundwater. These results indicated that most of the NO3− in river water was supplied through biological nitrification and that very little was supplied from the atmosphere directly. Detailed analysis of the Δ17O values for the rivers indicated that the atmosphere supplied a larger proportion of nitrate in the western region than in other regions. NO3−atm generally enters river water when high levels of precipitation fall and when snow melts.38 Samples were collected during the winter, but no snow fell on Yakushima Island before the sampling period (when the mean temperature was 15.3 °C 60 m above sea level). Less precipitation falls in the western region than in the central part of the island.84 The high Δ17O values in the western region could not, therefore, be attributed to high levels of precipitation or melting snow.
The Δ17O values may have been higher in the western region than the other regions because of differences in water residence times caused by the topographies of the watersheds. There are large differences between watersheds in the western region and other regions. The differences in topography affect the time taken for precipitation to enter the rivers. The longer the water residence time, the more dissolved NO3− in river water will be supplied by organisms. The topography of Yakushima Island is diverse and is particularly different in the western region compared with the other regions. The relationships between topographic indices and the NO3− and NO3−atm concentrations in the river watersheds were, therefore, investigated.
3.3 Effects of topography on variations in the nitrate concentration and sources
Topographic analysis for a wide area was performed to determine why the NO3−atm contribution to the total NO3− concentration was higher in the western region than other regions. NO3−atm was calculated by substituting the value for precipitation in the Northern Hemisphere, +26.6‰,36,57 into eqn (2). Three topography indicators were compared with the NO3− and NO3−atm concentrations. These were the catchment area, mean catchment slope, and mean catchment TWI. The TWI was calculated using eqn (3). Plots of the NO3− and NO3−atm concentrations against the topography indicators are shown in Fig. 5. The catchment area was negatively correlated with the NO3− concentration (Fig. 5a) (NO3−atm = −8.99TWI + 21.7), indicating that the NO3− concentration increased as the catchment area decreased. The TWI was negatively correlated with the NO3−atm concentration (NO3−atm = −2.47TWI + 13.3), indicating that the NO3−atm concentration increased as the TWI decreased (Fig. 5f). The TWI is a useful indicator of the ease with which water (including surface water and groundwater) collects in a catchment.61 The TWI of a watershed is, therefore, widely used as an indicator of microbial metabolism (including denitrification, assimilation processes, and nitrification processes that contribute NO3− to river water).62–65 Hayakawa et al.66 found that NO3− removal from river water through denitrification occurs in catchments with high TWI values.
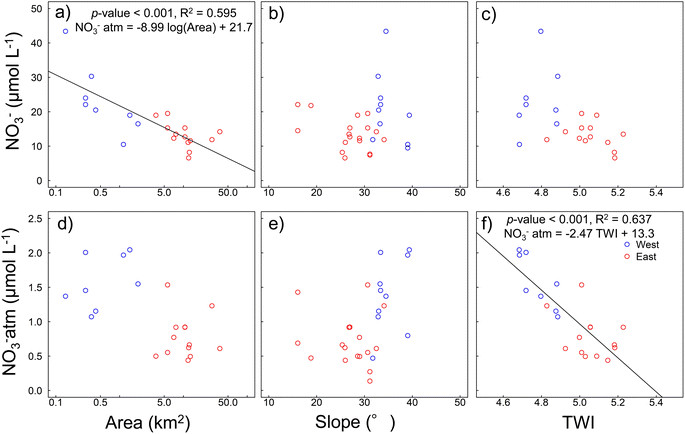 |
| Fig. 5 Relationships between topography parameters (watershed area, watershed mean slope, and topographic wetness index (TWI)) and the (a)–(c) NO3− and (d)–(f) NO3−atm concentrations NO3−atm was calculated by eqn (2). | |
The NO3− concentration tended to be higher in smaller catchments (Fig. 5a). In general, the larger the watershed area, the more dissolution of rock components and metabolism by organisms occurs. However, on Yakushima Island, NO3− concentrations tended to be lower in largely forested areas than elsewhere. River parameters for Yakushima Island reflect precipitation parameters well, and the rivers have short residence times.85 This suggests that in an area with extremely high rainfall, such as Yakushima Island, the ease with which water runs off because of the topography may strongly affect the sources of NO3− in the river water. In a watershed with a relatively large catchment area, the NO3− concentration in river water will probably decrease because NO3− will be used by trees and other organisms. The sampling sites were in catchments dominated by forests, thus it is likely that differences in runoff processes cause the NO3− concentration to increase as the catchment area decreases. However, no clear relationships between the TWI (an indicator of water ponding) and the NO3− concentration was found (R2 = 0.35, p = 0.003), as shown in Fig. 5d.
The survey was carried out in winter when precipitation on Yakushima Island is low, thus the rivers were at base flow. The direct effects of topography on changes in NO3−atm concentrations within watersheds through denitrification and biological processes such as nitrification and uptake will be complex.86 The base rock of Yakushima Island is granite, which has been weathered by long-term acidic deposition,5 and the high precipitation rate tends to cause a short groundwater residence time. Many rivers in the western region have low TWI values (Table 1). As shown in Fig. 5a, b, d and e, the rivers in the western region have low TWI values because they have small catchment areas and steep terrain. This indicates that rain that falls in the forests will remain for a short period in the soil and bedrock, particularly in the western region. The results suggested that, in the western region, water containing NO3− that has not been affected by NO3−re from forests is discharged into streams. This may explain why atmospheric NO3− is affected less by the terrestrial nitrogen cycle in the western region than other regions. The low TWIs may cause both the NO3−atm and NO3− concentrations to be higher in the western region than other regions.
Atmospheric deposition is the main source of nitrogen compounds entering the Yakushima Island ecosystem.87 Trees on Yakushima Island absorb most of the components supplied by the atmosphere.88 In this study, we found a strong negative correlation between the catchment area and NO3− concentration and a strong relationship between the NO3−atm concentration and the TWI (Fig. 5f). NO3−atm deposited in a forest will rapidly become incorporated into the nitrogen cycle within the forest system.31 This indicates that atmospheric deposition of NO3−atm is an important source of nutrients for the forests on Yakushima Island and that the rivers through which NO3−atm is likely to flow to sea are determined by the water retention capacities of the watersheds. The NO3− and NO3−atm concentrations are higher in the western region than other regions because more atmospheric deposition occurs but also the topography facilitates runoff of NO3−atm. Despite the reduction in atmospheric deposition, NO3− in river water remained high in 2023 (Fig. S2†). The results obtained in 2018, therefore, reflect the mechanism of nitrogen cycling in Yakushima's forests.
4 Conclusions
This study has provided insights into the sources of NO3− in the rivers on Yakushima Island, revealing that high NO3− concentrations are primarily driven by atmospheric deposition. By using stable isotope ratios, we were able to differentiate between NO3−atm and NO3−re, allowing for a more accurate quantification of NO3−atm in river water. The rivers on Yakushima Island were categorized into three groups based on the similarity of their dissolved ion compositions: rivers in the western part of the island, rivers in other regions, and groundwater. Notably, NO3− concentrations in the western region were 10.5 μmol L−1 higher than in other areas, including groundwater, with the majority of these watersheds located within the Natural World Heritage site. Stable isotope analysis confirmed that the western region had higher levels of NO3−atm, suggesting that atmospheric nitrate ions contribute more significantly to river water in this area. Interestingly, the Δ17O values in the western region mirrored those found in forests with high nitrate runoff, indicating a similar process might be at play. Despite high levels of wet deposition—comparable with those observed in other Asian regions—the overall NO3− levels in Yakushima's stream water were relatively low, likely because of the island's significant rainfall. Topographic analysis revealed strong correlations between catchment area, NO3− concentration, and TWI, suggesting that smaller watersheds with steep topography are particularly prone to higher NO3−atm inputs in river water. In nitrogen-limited forest ecosystems, NO3− deposited from the atmosphere is typically rapidly assimilated by organisms and converted into NO3−re. However, the unique combination of high precipitation, small watersheds, and steep topography on Yakushima Island appears to limit this biological uptake, allowing a greater proportion of NO3−atm to enter the rivers directly. Given these findings, it is essential to consider that nitrogen deposition from the atmosphere may vary across different regions of Yakushima. In particular, the western region, where fog is more prevalent, may experience higher levels of nitrogen deposition owing to the concentration of atmospheric constituents in wet deposition from fog. Future studies should aim to clarify the impact of different types of wet and occult deposition on NO3− levels in river water and further investigate the relationship between NO3−atm concentrations and topography in other forested regions with similar conditions.
Data availability
Raw data were generated at Gifu University, Fukuoka Institute of Technology and Setsunan University. Derived data supporting the findings of this study are available from the corresponding authors K. S.
Author contributions
K. S., O. N., and S. E. conceived the study; K. S., O. N., and S. E. designed the research; K. S., K. T., N. T., O. N., and S. E. led the field work; K. S., K. N., U. T., and F. N. performed laboratory analysis; K. S. performed data analysis and wrote the paper with input from all co-authors.
Conflicts of interest
There are no conflicts to declare.
Acknowledgements
This work was supported by JSPS KAKENHI (grant numbers 26257301, 18K04413 and 23K14057), the Ministry of the Environment Biodiversity Conservation Promotion Support Project, Yakushima Biodiversity Conservation Council, the Institute for Space–Earth Environmental Research (ISEE), Nagoya University, and the Sasakawa Scientific Research (through a grant from the Japan Science Society). The authors are grateful to the students at each university who taught the analytical methods. We thank Gareth Thomas, PhD, and Leonie Seabrook, PhD, from Edanz (https://jp.edanz.com/ac) for editing a draft of this manuscript.
References
- Ministry of the Environment Government of Japan Acid rain monitoring result, https://www.env.go.jp/air/acidrain/acidrain.html, accessed July 2024.
- S. Agata, M. Ishiki, H. Sakihama, A. Tokuyama, H. Satake and J. Zhang, Characteristics of the chemical composition of groundwater on Tanegashima, Yakushima, and Nakanoshima islands, Kagoshima Prefecture, Japan, Journal of Groundwater Hydrology, 2012, 54, 191–206 CrossRef.
- T. Nakano, M. Okumura, M. Yamanaka and K. Satake, Geochemical Characteristics of Acidic Stream Water on Yakushima Island, a World Natural Heritage Site, Water, Air, Soil Pollut., 2001, 130, 869–874 CrossRef.
- K. Fujii, S. Kanetani and K. Tetsuka, Effects of volcanic parent materials on the acid buffering capacity of forest soils on Yakushima Island, Japan, Soil Sci. Plant Nutr., 2020, 66, 680–692 CrossRef CAS.
- I. Yukihiro, N. Osamu, A. Suguru, Y. Kuriko and Y. Kazuhisa, Study on low alkalinity measurement method - application to low alkalinity stream on Yakushima, Journal of Ecotechnology Research, 2012, 112, 109–112 Search PubMed.
- O. Nagafuchi, S. Akune, K. Yoshimura, A. Kume, S. Ebise and K. Tetsuka, Effect of Acid Deposition on the Water Quality Formation of Mountainous Streams in the Western Part of Yakushima Island, a World Natural Heritage Site, J. Jpn. Soc. Water Environ., 2003, 26, 159–166 CrossRef CAS.
- T. Nakano, Y. Yokoo, M. Okumura, S.-R. Jean and K. Satake, Evaluation of the Impacts of Marine Salts and Asian Dust on the Forested Yakushima Island Ecosystem, a World Natural Heritage Site in Japan, Water, Air, Soil Pollut., 2012, 223, 5575–5597 CrossRef CAS PubMed.
- T. Doi, O. Nagafuchi, K. Yokota, K. Yoshimura, S. Akune, T. Yamanaka and S. Miyabe, Determination of sulfur isotope ratio of sulfate in mountainous streams on Yakushima Island after in-situ collection/concentration of sulfate, 2011, pp. 135–144 Search PubMed.
- O. Nagafuchi, H. Kakimoto, S. Ebise and M. Ukita, Effects of forests on mountain stream water quality, Jpn. J. Limnol., 2002, 63, 11–19 CrossRef CAS.
- T. Ohara, Why is the increase of tropospheric ozone concentration in mountain and island regions in Japan?(〈Feature 2〉Influence of long-range transport of air pollution on the Japanese ecosystems), Jpn. J. Ecol., 2011, 61, 77–81 CAS.
- S. Ebise and O. Nagafuchi, Runoff characteristics of water quality and influence of acid rain on mountainous streamwater on Yakushima Island, Jpn. J. Limnol., 2002, 63, 1–10 CrossRef CAS.
- J. P. Kaye and S. C. Hart, Competition for nitrogen between plants and soil microorganisms, Trends Ecol. Evol., 1997, 12, 139–143 CrossRef CAS PubMed.
- J. S. Baron, H. M. Rueth, A. M. Wolfe, K. R. Nydick, E. J. Allstott, J. T. Minear and B. Moraska, Ecosystem Responses to Nitrogen Deposition in the Colorado Front Range, Ecosystems, 2000, 3, 352–368 CrossRef CAS.
- J. S. Baron, K. R. Nydick, H. M. Rueth, B. M. Lafrançois and A. P. Wolfe, High Elevation Ecosystem Responses to Atmospheric Deposition of Nitrogen in the Colorado Rocky Mountains, ed. U. M. Huber, H. K. M. Bugmann and M. A. Reasoner, Springer Netherlands, Dordrecht, 2005, pp. 429–436 Search PubMed.
- J. S. Baron, C. T. Driscoll, J. L. Stoddard and E. E. Richer, Empirical Critical Loads of Atmospheric Nitrogen Deposition for Nutrient Enrichment and Acidification of Sensitive US Lakes, Bioscience, 2011, 61, 602–613 CrossRef.
- M. W. Williams and K. A. Tonnessen, Critical loads for inorganic nitrogen deposition in the Colorado Front Range, USA, Ecological Applications, 2000, 10, 1648–1665 CrossRef.
- M. van der Velde, S. R. Green, M. Vanclooster and B. E. Clothier, Sustainable development in small island developing states: agricultural intensification, economic development, and freshwater resources management on the coral atoll of Tongatapu, Ecological Economics, 2007, 61, 456–468 CrossRef.
- V. Wasiuta, M. J. Lafrenière, A.-L. Norman and M. G. Hastings, Summer deposition of sulfate and reactive nitrogen to two alpine valleys in the Canadian Rocky Mountains, Atmos. Environ., 2015, 101, 270–285 CrossRef CAS.
- E. J. Hundey, S. D. Russell, F. J. Longstaffe and K. A. Moser, Agriculture causes nitrate fertilization of remote alpine lakes, Nat. Commun., 2016, 7, 10571 CrossRef CAS PubMed.
- L. Nanus, M. W. Williams, D. H. Campbell, E. M. Elliott and C. Kendall, Evaluating Regional Patterns in Nitrate Sources to Watersheds in National Parks of the Rocky Mountains using Nitrate Isotopes, Environ. Sci. Technol., 2008, 42, 6487–6493 CrossRef CAS PubMed.
- W. D. Bowman, J. R. Gartner, K. Holland and M. Wiedermann, Nitrogen Critical Loads For Alpine Vegetation and Terrestrial Ecosystem Response: Are We There Yet?, Ecological Applications, 2006, 16, 1183–1193 CrossRef PubMed.
- L. Nanus, J. A. McMurray, D. W. Clow, J. E. Saros, T. Blett and J. J. Gurdak, Spatial variation of atmospheric nitrogen deposition and critical loads for aquatic ecosystems in the Greater Yellowstone Area, Environ. Pollut., 2017, 223, 644–656 CrossRef CAS PubMed.
- J. N. Galloway and E. B. Cowling, Reactive Nitrogen and the World: 200 Years of Change, Ambio, 2002, 31, 64–71 CrossRef PubMed.
- G. F. McIsaac, M. B. David, G. Z. Gertner and D. A. Goolsby, Nitrate flux in the Mississippi River, Nature, 2001, 414, 166–167 CrossRef CAS PubMed.
- H. W. Paerl, Controlling eutrophication along the freshwater-Marine continuum: dual nutrient (N and P) reductions are essential, Estuaries Coasts, 2009, 32, 593–601 CrossRef CAS.
- J. Kurokawa and T. Ohara, Long-term historical trends in air pollutant emissions in Asia: Regional Emission inventory in ASia (REAS) version 3, Atmos. Chem. Phys., 2020, 20, 12761–12793 CrossRef CAS.
- M. Chiwa, N. Onikura, J. Ide and A. Kume, Impact of N-Saturated Upland Forests on Downstream N Pollution in the Tatara River Basin, Japan, Ecosystems, 2011, 15, 230–241 CrossRef.
- F. Taketani, M. N. Aita, K. Yamaji, T. Sekiya, K. Ikeda, K. Sasaoka, T. Hashioka, M. C. Honda, K. Matsumoto and Y. Kanaya, Seasonal Response of North Western Pacific Marine Ecosystems to Deposition of Atmospheric Inorganic Nitrogen Compounds from East Asia, Sci. Rep., 2018, 8, 1–9 CAS.
- M. Chiwa, Long-term changes in atmospheric nitrogen deposition and stream water nitrate leaching from forested watersheds in western Japan, Environ. Pollut., 2021, 287, 117634 CrossRef CAS PubMed.
- H. Sase, M. Takahashi, K. Matsuda, N. Yamashita, U. Tsunogai, F. Nakagawa, M. Morohashi, H. Yotsuyanagi, T. Ohizumi, K. Sato, J. Kurokawa and M. Nakata, Nitrogen saturation of forested catchments in central Japan - progress or recovery?, Soil Sci. Plant Nutr., 2022, 68, 5–14 CrossRef CAS.
- R. Sugimoto and T. Tsuboi, Seasonal and annual fluxes of atmospheric nitrogen deposition and riverine nitrogen export in two adjacent contrasting rivers in central Japan facing the Sea of Japan, Journal of Hydrology: Regional Studies, 2017, 11, 117–125 Search PubMed.
- S. Ebise, O. Nagafuchi and H. Kawamura, Relationships between Acidic Wet Depositions and Water Quality in Mountain Streams on Solitary Islands and High Mountains Facing the Sea of Japan and the East China Sea, Environ. Sci., 2019, 32, 125–140 Search PubMed.
- N. Ohte, I. Tayasu, A. Kohzu, C. Yoshimizu, K. Osaka, A. Makabe, K. Koba, N. Yoshida and T. Nagata, Spatial distribution of nitrate sources of rivers in the Lake Biwa watershed, Japan: controlling factors revealed by nitrogen and oxygen isotope values, Water Resour. Res., 2010, 46, W07505 CrossRef.
- C. Kendall, Isotope Tracers in Catchment Hydrology, ed. C. Kendall and J. J. McDonnell, Elsevier, Amsterdam, 1998, vol. 16, pp. 519–576 Search PubMed.
- U. Tsunogai, S. Daita, D. D. Komatsu, F. Nakagawa and A. Tanaka, Quantifying nitrate dynamics in an oligotrophic
lake using Δ17O, Biogeosciences, 2011, 8, 687–702 CrossRef CAS.
- U. Tsunogai, D. D. Komatsu, S. Daita, G. A. Kazemi, F. Nakagawa, I. Noguchi and J. Zhang, Tracing the fate of atmospheric nitrate deposited onto a forest ecosystem in Eastern Asia using Δ17O, Atmos. Chem. Phys., 2010, 10, 1809–1820 CrossRef CAS.
- F. Nakagawa, U. Tsunogai, Y. Obata, K. Ando, N. Yamashita, T. Saito, S. Uchiyama, M. Morohashi and H. Sase, Export flux of unprocessed atmospheric nitrate from temperate forested catchments: a possible new index for nitrogen saturation, Biogeosciences, 2018, 15, 7025–7042 CrossRef CAS.
- I. Bourgeois, J. Savarino, N. Caillon, H. Angot, A. Barbero, F. Delbart, D. Voisin and J. C. Clément, Tracing the Fate of Atmospheric Nitrate in a Subalpine Watershed Using Δ17O, Environ. Sci. Technol., 2018, 52, 5561–5570 CrossRef CAS PubMed.
- S. Huang, F. Wang, E. M. Elliott, F. Zhu, W. Zhu, K. Koba, Z. Yu, E. A. Hobbie, G. Michalski, R. Kang, A. Wang, J. Zhu, S. Fu and Y. Fang, Multiyear Measurements on Δ17O of Stream Nitrate Indicate High Nitrate Production in a Temperate Forest, Environ. Sci. Technol., 2020, 54, 4231–4239 CrossRef CAS PubMed.
- J. T. Bostic, D. M. Nelson, R. D. Sabo and K. N. Eshleman, Terrestrial Nitrogen Inputs Affect the Export of Unprocessed Atmospheric Nitrate to Surface Waters: Insights from Triple Oxygen Isotopes of Nitrate, Ecosystems, 2022, 25, 1384–1399 CrossRef CAS.
- S. Hattori, Y. N. Palma, Y. Itoh, M. Kawasaki, Y. Fujihara, K. Takase and N. Yoshida, Isotopic evidence for seasonality of microbial internal nitrogen cycles in a temperate forested catchment with heavy snowfall, Sci. Total Environ., 2019, 690, 290–299 CrossRef CAS PubMed.
- L. A. Rose, E. M. Elliott and M. B. Adams, Triple Nitrate Isotopes Indicate Differing Nitrate Source Contributions to Streams across a Nitrogen Saturation Gradient, Ecosystems, 2015, 18, 1209–1223 CrossRef CAS.
- R. D. Sabo, D. M. Nelson and K. N. Eshleman, Episodic, seasonal, and annual export of atmospheric and microbial nitrate from a temperate forest, Geophys. Res. Lett., 2016, 43, 683–691 CrossRef CAS.
- U. Tsunogai, L. Cheng, M. Ito, D. D. Komatsu, F. Nakagawa and H. Shinohara, Remote determinations on fumarole outlet temperatures in an eruptive volcano, Geophys. Res. Lett., 2016, 43, 11620–11627 CrossRef.
- U. Tsunogai, D. D. Komatsu, T. Ohyama, A. Suzuki, F. Nakagawa, I. Noguchi, K. Takagi, M. Nomura, K. Fukuzawa and H. Shibata, Quantifying the effects of clear-cutting and strip-cutting on nitrate dynamics in a forested watershed using triple oxygen isotopes as tracers, Biogeosciences, 2014, 11, 5411–5424 CrossRef.
- W. Ding, U. Tsunogai, F. Nakagawa, T. Sambuichi, M. Chiwa, T. Kasahara and K. Shinozuka, Stable isotopic evidence for the excess leaching of unprocessed atmospheric nitrate from forested catchments under high nitrogen saturation, Biogeosciences, 2023, 20(3), 753–766, DOI:10.5194/bg-20-753-2023.
- G. Oota, Mountains of Yakushima, Yaedake Press, 1993 Search PubMed.
- Kyushu Regional Forest Office homepage, https://www.rinya.maff.go.jp/kyusyu/yakusima_hozen_c/gaiyou/kisyou/index.html, accessed November 2023.
- Yakushima World Heritage Conservation Center web site, https://www.env.go.jp/en/park/yakushima/ywhcc/wh/toroku.html, accessed November 2023.
- UNESCO World heritage list nomination – IUCN summary 662: Yakushima, https://whc.unesco.org/document/153977, accessed November 2023.
- U. Tsunogai, T. Kido, A. Hirota, S. B. Ohkubo, D. D. Komatsu and F. Nakagawa, Sensitive determinations of stable nitrogen isotopic composition of organic nitrogen through chemical conversion into N2O, Rapid Commun. Mass Spectrom., 2008, 22, 345–354 CrossRef CAS PubMed.
- U. Tsunogai, T. Miyauchi, T. Ohyama, D. D. Komatsu, M. Ito and F. Nakagawa, Quantifying nitrate dynamics in a mesotrophic lake using triple oxygen isotopes as tracers, Limnol. Oceanogr., 2018, 63, S458–S476 CrossRef CAS.
- U. Konno, U. Tsunogai, D. D. Komatsu, S. Daita, F. Nakagawa, A. Tsuda, T. Matsui, Y. J. Eum and K. Suzuki, Determination of total N2 fixation rates in the ocean taking into account both the particulate and filtrate fractions, Biogeosciences, 2010, 7, 2369–2377 CrossRef CAS.
- D. D. Komatsu, T. Ishimura, F. Nakagawa and U. Tsunogai, Determination of the 15N/14N, 17O/16O, and 18O/16O ratios of nitrous oxide by using continuous-flow isotope-ratio mass spectrometry, Rapid Commun. Mass Spectrom., 2008, 22, 1587–1596 CrossRef CAS PubMed.
- A. Hirota, U. Tsunogai, D. D. Komatsu and F. Nakagawa, Simultaneous determination of δ15N and δ18O of N2O and δ13C of CH4 in nanomolar quantities from a single water sample, Rapid Commun. Mass Spectrom., 2010, 24, 1085–1092 CrossRef CAS PubMed.
- F. Nakagawa, A. Suzuki, S. Daita, T. Ohyama, D. D. Komatsu and U. Tsunogai, Tracing atmospheric nitrate in groundwater using triple oxygen isotopes: evaluation based on bottled drinking water, Biogeosciences, 2013, 10, 3547–3558 CrossRef.
- U. Tsunogai, T. Miyauchi, T. Ohyama, D. D. Komatsu, F. Nakagawa, Y. Obata, K. Sato and T. Ohizumi, Accurate and precise quantification of atmospheric nitrate in streams draining land of various uses by using triple oxygen isotopes as tracers, Biogeosciences, 2016, 13, 3441–3459 CrossRef CAS.
- M. F. Miller, Isotopic fractionation and the quantification of 17O anomalies in the oxygen three-isotope system: an appraisal and geochemical significance, Geochim. Cosmochim. Acta, 2002, 66, 1881–1889 CrossRef CAS.
- K. C. Weathers, S. M. Simkin, G. M. Lovett and S. E. Lindberg, Empirical modeling of atmospheric deposition in mountainous landscapes, Ecological Applications, 2006, 16, 1590–1607 CrossRef PubMed.
- K. C. Weathers, G. M. Lovett, G. E. Likens and R. Lathrop, The effect of landscape features on deposition to hunter mountain, Catskill Mountains, New York, Ecological Applications, 2000, 10, 528–540 CrossRef.
- K. J. Beven and M. J. Kirkby, A physically based, variable contributing area model of basin hydrology, Hydrol. Sci. Bull., 1979, 24, 43–69 CrossRef.
- A. Ogawa, H. Shibata, K. Suzuki, M. J. Mitchell and Y. Ikegami, Relationship of topography to surface water chemistry with particular focus on nitrogen and organic carbon solutes within a forested watershed in Hokkaido, Japan, Hydrol. Processes, 2006, 20, 251–265 CrossRef CAS.
- K. Shinozuka, M. Chiwa, I. Tayasu, C. Yoshimizu, K. Otsuki and A. Kume, Differences in stream water nitrate concentrations between a nitrogen-saturated upland forest and a downstream mixed land use River Basin, Hydrology, 2017, 4, 43 CrossRef.
- R. Sugimoto, T. Tsuboi and M. S. Fujita, Comprehensive and quantitative assessment of nitrate dynamics in two contrasting forested basins along the Sea of Japan using dual isotopes of nitrate, Sci. Total Environ., 2019, 687, 667–678 CrossRef CAS PubMed.
- S. Makino, N. Tokuchi, Y. Komai and T. Kunimatsu, Environmental factors regulating stream nitrate concentrations at baseflow condition in a large region encompassing a climatic gradient, Hydrol. Processes, 2021, 35, e14200 CrossRef.
- A. Hayakawa, Y. Funaki, T. Sudo, R. Asano, H. Murano, S. Watanabe, T. Ishida, Y. Ishikawa and S. Hidaka, Catchment topography and the distribution of electron donors for denitrification control the nitrate concentration in headwater streams of the Lake Hachiro watershed, Soil Sci. Plant Nutr., 2020, 66, 906–918 CrossRef CAS.
- Geospatial Information Authority of Japan, https://fgd.gsi.go.jp/download/menu.php, accessed October 2023.
- M. W. Raduła, T. H. Szymura and M. Szymura, Topographic wetness index explains soil moisture better than bioindication with Ellenberg's indicator values, Ecol. Indic., 2018, 85, 172–179 CrossRef.
- M. Maechler, P. Rousseeuw, A. Struyf, M. Hubert, K. Hornik, M. Studer, P. Roudier, J. González, K. Kozlowski, E. Schubert and K. Murphy, Package ‘cluster.’ Finding Groups in Data, 2022 Search PubMed.
- E. Joshi, M. R. Schwarzbach, B. Briggs, E. R. Coats and M. D. Coleman, Nutrient leaching potential along a time series of forest water reclamation facilities in northern Idaho, J. Environ. Manage., 2024, 366, 121729 CrossRef CAS PubMed.
- P. Wang, W. Ouyang, Z. Wu, X. Cui, W. Zhu, R. Jin and C. Lin, Diffuse nitrogen pollution in a forest-dominated watershed: source, transport and removal, J. Hydrol., 2020, 585, 124833 CrossRef CAS.
- M. Igawa, E. Hoka, T. Hosono, K. Iwase and T. Nagashima, Analysis and Scavenging Effect of Acid Fog, Nippon Kagaku Kaishi, 1991, 1991, 698–704 CrossRef.
- M. Igawa, Y. Tsutsumi, T. Mori and H. Okochi, Fogwater Chemistry at a Mountainside Forest and the Estimation of the Air Pollutant Deposition via Fog Droplets Based on the Atmospheric Quality at the Mountain Base, Environ. Sci. Technol., 1998, 32, 1566–1572 CrossRef CAS.
- M. Igawa and Y. Wang, Characteristics of Fog and Drizzle in Yokohama and in Mt. Oyama, Japan, Water, Air, Soil Pollut., 2022, 233, 533 CrossRef CAS.
- B. Mayer, E. W. Boyer, C. Goodale, N. A. Jaworski, N. Van Breemen, R. W. Howarth, S. Seitzinger, G. Billen, K. Lajtha, K. Nadelhoffer, D. Van Dam, L. J. Hetling, M. Nosal and K. Paustian, Sources of nitrate in rivers draining sixteen watersheds in the northeastern U.S.: isotopic constraints, in The Nitrogen Cycle at Regional to Global Scales, 2002, pp. 171–197 Search PubMed.
- D. H. Campbell, C. Kendall, C. C. Y. Chang, S. R. Silva and K. A. Tonnessen, Pathways for nitrate release from an alpine watershed: determination using δ15N and δ18O, Water Resour. Res., 2002, 38, 101–109 Search PubMed.
- D. A. Burns and C. Kendall, Analysis of δ15N and δ18O to differentiate NO3− sources in runoff at two watersheds in the Catskill Mountains of New York, Water Resour. Res., 2002, 38, 91–912 CrossRef.
- G. Michalski, T. Meixner, M. Fenn, L. Hernandez, A. Sirulnik, E. Allen and M. Thiemens, Tracing Atmospheric Nitrate Deposition in a Complex Semiarid Ecosystem Using Δ17O, Environ. Sci. Technol., 2004, 38, 2175–2181 CrossRef CAS PubMed.
- L. K. Lautz and R. M. Fanelli, Seasonal biogeochemical hotspots in the streambed around restoration structures, Biogeochemistry, 2008, 91, 85–104 CrossRef CAS.
- S. A. Comer-Warner, D. C. Gooddy, S. Ullah, L. Glover, N. Kettridge, S. K. Wexler, J. Kaiser and S. Krause, Seasonal variability of sediment controls of nitrogen cycling in an agricultural stream, Biogeochemistry, 2020, 148, 31–48 CrossRef CAS.
- T. Liu, F. Wang, G. Michalski, X. Xia and S. Liu, Using 15N, 17O, and 18O to determine nitrate sources in the Yellow River, China, Environ. Sci. Technol., 2013, 47, 13412–13421 CrossRef CAS PubMed.
- A. Amberger and H.-L. Schmidt, Natürliche Isotopengehalte von Nitrat als Indikatoren für dessen Herkunft, Geochim. Cosmochim. Acta, 1987, 51(10), 2699–2705, DOI:10.1016/0016-7037(87)90150-5.
- R. F. Spalding, A. J. Hirsh, M. E. Exner, N. A. Little and K. L. Kloppenborg, Applicability of the dual isotopes δ15N and δ18O to identify nitrate in groundwater beneath irrigated cropland, J. Contam. Hydrol., 2019, 220, 128–135, DOI:10.1016/j.jconhyd.2018.12.004.
- H. Takaharra and J. Matsumoto, Climatological Study of Precipitation Distribution in Yakushima Island, Southern Japan, J. Geogr., 2002, 111, 726–746 CrossRef PubMed.
- M. Yamanaka, M. Okumura and T. Nakano, Isotopic altitude effect and discharge characteristics of river water in Yakushima Island, southwestern Japan, Journal of Japanese Association of Hydrological Sciences, 2007, 37, 41–54 CrossRef.
- E. M. Lacroix, M. Aeppli, K. Boye, E. Brodie, S. Fendorf, M. Keiluweit, H. R. Naughton, V. Noël and D. Sihi, Consider the Anoxic Microsite: Acknowledging and Appreciating Spatiotemporal Redox Heterogeneity in Soils and Sediments, ACS Earth Space Chem., 2023, 7, 1592–1609 CrossRef CAS PubMed.
- K. Satake, T. Inoue, K. Kasasaku, O. Nagafuchi and T. Nakano, Monitoring of nitrogen compounds on Yakushima Island, a world natural heritage site, Environ. Pollut., 1998, 102, 107–113 CrossRef CAS.
- T. Nakano, S.-R. Jeon, J. Shindo, T. Fumoto, N. Okada and J. Shimada, Sr Isotopic Signature in Plant-Derived Ca in Rain, Water, Air, Soil Pollut., 2001, 130, 769–774 CrossRef.
|
This journal is © The Royal Society of Chemistry 2024 |
Click here to see how this site uses Cookies. View our privacy policy here.