DOI:
10.1039/D4RA05084C
(Paper)
RSC Adv., 2024,
14, 29168-29173
Vitamin B12-catalyzed coupling reaction of nitroalkanes and diazo compounds†
Received
14th July 2024
, Accepted 6th September 2024
First published on 13th September 2024
Abstract
Vitamin B12 is a natural and environmentally friendly catalyst. When exposed to light or heat, central Co(I) can react with electrophiles to obtain alkyl radicals, which can subsequently be used in complex processes. Herein, the vitamin B12-catalyzed coupling reaction of nitroalkanes and diazo compounds is reported leading to substituted tertiary nitroalkanes in moderate yields. The reaction conditions were optimized, and the scope and limitations of the reaction were also investigated.
Introduction
Vitamin B12 (cobalamin B12, 1a, Fig. 1) is the first known naturally occurring organometallic compound1 synthesized by bacteria,2 and plays a number of important roles in biological systems, participating in reactions such as enzymatic isomerization, methyl transfer and dehalogenation.3 Vitamin B12 is a highly functional and highly stable metal complex,4 which can be classified into four compounds, cyanocobalamin, methylcobalamin, aquacobalamin and adenosylcobalamin, depending on the substituent group to which the central cobalt atom is attached,5 which determines its solubility.6 Since the central cobalt atom is easily transformed between nucleophilic reagent Co(I), radical Co(II), and electrophilic reagent Co(III). In particular, the “super-nucleophilic” reagent Co(I) with a lone pair of electrons reacts readily with electrophilic reagents to give alkyl cobaltamins, which undergo homolysis of the C–Co bond in the presence of light or heat to give the alkyl radical and thus complete a series of complex reactions,7 and such natural compounds have proved to be useful catalysts for a number of organic reactions, such as alkene coupling and atom transfer radical polymerization reactions.8 In general, vitamin B12-catalysed reactions follow a radical mechanism,9 including organic halides,6 diazo compounds,10 epoxides,11 etc., all of which are suitable radical sources.7 Cobalamin has a wide appeal due to its remarkable stability, non-toxicity,12 eco-friendly and cost-effective as compared to precious metal complexes.13,14
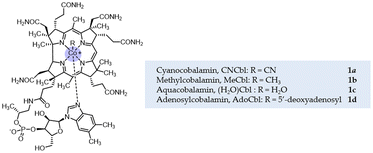 |
| Fig. 1 Structure of vitamin B12 derivatives. | |
C–H bonds are ubiquitous structural units of organic molecules. Developing a capable C–H bond functionalization system has been challenging due to its chemically inert nature, although significant progress has been made using some transition metal catalysts or metallaphotoredox catalysis, such as Pd, Ru, Cu, Ag, Co, and Fe complexes, etc.15–22 Diazo compounds are commonly used as building blocks in organic synthesis due to their broad and tunable reactivity, additionally, as photocatalysis has advanced, photocatalysts reduce diazo compounds through a single electron transfer process, leading to the discovery of different novel radical reactivities.23–25 In particular, α-carbonyl diazo compounds have a wide range of reactivities, they can be utilized in C–H functionalization, such as C(sp3)–H, aromatic C(sp2)–H, and C(sp)–H bonds insertion, in the presence of metal complexes.26 In 2014, Frank Glorius27 presented the first cobalt(III)-catalyzed directed C–H functionalization of heteroaromatic compounds with diazo compounds (Scheme 1A). In 2015, Dorota Gryko and co-worker28 took advantage of the high reactivity of α-carbonyl diazo compounds and used a natural non-toxic vitamin B12 derivative as a catalyst to catalyze a novel alkylation reaction of alkene C(sp2)–H bond (Scheme 1B). The range of applications of vitamin B12 was further broadened (Scheme 1).
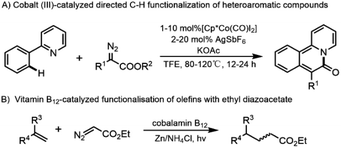 |
| Scheme 1 Co(III)-catalyzed C–H functionalization with diazo compounds. | |
In this process, ethyl diazoacetate (EDA) was reacted with cobalester in the presence of a reducing system (Zn/NH4Cl) to give an alkyl cobalamin intermediate. Subsequently, the C–Co bond of the cobalt alkyl esters is cleaved to obtain alkyl radical, which in turn inserts into the C(sp2)–H bonds of the alkene. This reaction mechanism gave us a hint whether vitamin B12 has the activity to catalyze the insertion of alkyl radicals into C(sp3)–H bonds under the stimulation of light or heat.
Nitroalkanes have been used as nucleophiles for example in Henry reaction. Even in the absence of base, these compounds readily tautomerize to a structure that is nucleophilic at carbon (Scheme 2A)29–31 and, as such, have been used as substrates for C–C bond-forming reactions catalyzed by electrophile-activating enzymes (Scheme 2B).31 In 2022, Todd Hyster has developed a highly chemo and stereoselective C-alkylation of nitroalkanes with alkyl halides catalyzed by an engineered flavin-dependent “ene”-reductase (ERED) (Scheme 2C).32 Based on those reports, we envisioned that the diazo compounds can be transformed to an alkyl radical that can add to an in situ-generated tautomerized nitronate to form a new C–C bond.
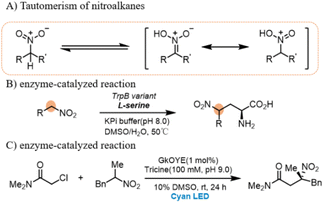 |
| Scheme 2 Reactivity of nitroalkanes. | |
Results
In our preliminary experiments, we found that under heating conditions, using cyanocobalamin as a catalyst and methanol as a solvent, nitrocyclohexanes and ethyl diazoacetate in the presence of the reducing agent Zn could achieve alkylation reaction of the C(sp3)–H bond to give tertiary nitroalkanes (Scheme 3).
 |
| Scheme 3 Model reaction of nitrocyclohexane (2) with EDA (3). | |
Using the reaction of nitrocyclohexane and EDA as a model reaction, we systematically performed optimization of the reaction conditions with regard to solvents, pH, reagent dosage, reaction time and temperature. When methanol or ethanol was used, nitrocyclohexane can coupled with EDA gave traces of tertiary nitroalkanes, and when the solvent was changed into DMSO, the yields were greatly improved (Table 1, entries 1–3), the presence of water would slightly increase the reaction yield to 38%, which can improve the solubility of vitamin B12. We next investigated the effects of mixed solvents with water, including isopropanol, 2-propanol, DMSO, THF, and 1,4-dioxane on the yield (Table 1, entries 4–12). When DMSO
:
H2O = 1
:
1 was used as the reaction solvent, the yield increased to 38%. Moreover, when the aqueous solution was replaced with potassium phosphate buffer solutions of different pH, a decrease in the yield was observed (see ESI† for details). The reaction temperature has a strong influence on the yield, 50 °C is the optimal reaction temperature (see ESI† for more details).
Table 1 Optimization: the influence of solvent
Entrya |
Solvent |
Temp (°C) |
Time (h) |
Yield (%) |
Reaction conditions: nitrocyclohexane (0.25 mmol), EDA (2 equiv.), Zn (6 equiv.), vitamin B12 (2 mol%), solvent (2.5 mL), 24 h. |
1 |
CH3OH |
50 |
24 |
7 |
2 |
CH3CH2OH |
50 |
24 |
4 |
3 |
DMSO |
50 |
24 |
36 |
4 |
H2O : DMSO = 1 : 1 |
50 |
24 |
38 |
5 |
H2O : DMSO = 2 : 1 |
50 |
24 |
36 |
6 |
H2O : DMSO = 1 : 2 |
50 |
24 |
36 |
7 |
(CH3)3COH : H2O = 1 : 1 |
50 |
24 |
13 |
8 |
(CH3)2CHOH : H2O = 1 : 1 |
50 |
24 |
11 |
9 |
THF : H2O = 1 : 1 |
50 |
24 |
7 |
10 |
DMF : H2O = 1 : 1 |
50 |
24 |
14 |
11 |
1,4-Dioxane : H2O = 1 : 1 |
50 |
24 |
14 |
12 |
HFIP : H2O = 1 : 1 |
50 |
24 |
13 |
The product yield strongly depended on the amount of catalyst and EDA, using an equal amount of EDA with 2.0 mol% of catalyst, tertiary nitroalkanes can be isolated with a yield of 32%. The use of higher concentrations of EDA resulted in an increase in yield, but a decrease in yield was observed when the amount of EDA exceeded 2.0 eq. (Table 2, entries 1–3). And then the catalyst was increased from 2.0 mol% to 8.0 mol% in the presence of 2.0 eq. EDA, only a small increase in yield can be observed (Table 2, entries 3–6), but from an economic point of view, the amount of catalyst of 2.0 mol% is chosen in this reaction. Furthermore, we determined 6 equiv. of Zn as the optimal reducing system loading (see ESI† for more details).
Table 2 Optimization: the influence of vitamin B12 and EDA
Entrya |
Vitamin B12 (mol%) |
EDA (equiv.) |
Yield (%) |
Reaction conditions: nitrocyclohexane (0.25 mmol), EDA (2 equiv.), Zn (6 equiv.), vitamin B12 (2 mol%), solvent (2.5 mL), 24 h. |
1 |
2.0 |
1.0 |
32 |
2 |
2.0 |
2.0 |
38 |
3 |
2.0 |
3.0 |
29 |
4 |
4.0 |
2.0 |
40 |
5 |
6.0 |
2.0 |
40 |
6 |
8.0 |
2.0 |
42 |
7 |
10.0 |
2.0 |
38 |
The scope and limitation of the reaction were then investigated under the establishing the optimal reaction conditions above (Scheme 4). Among the series of diazo compounds (3a–3j), only diazoacetates 3b, 3c, 3d can give the corresponding products with relatively low yield. No product for 3a, 3e–3j was detected for other diazo compounds, probably because the higher steric effects prevented the progress of the reaction.
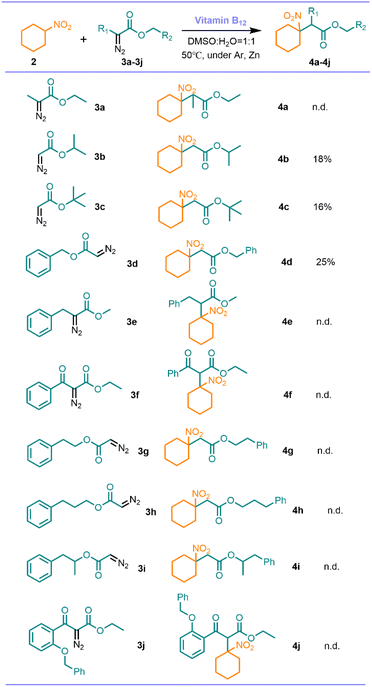 |
| Scheme 4 Scope and limitation. Reaction conditions: nitrocyclohexane (0.25 mmol), diazo compounds (2 equiv.), Zn (6 equiv.), vitamin B12 (2 mol%), solvent (2.5 mL), 24 h. | |
In addition, the reactions of ethyl diazoacetate and benzyl 2-diazoacetate with a series of nitro compounds were investigated (Scheme 5). 2-Nitropropane with 3 and 3d could produce the coupling product in 28% and 14% respectively. However, when phenyl nitromethanes were employed, no expected product was observed, presumably because the benzene is conjugated to the nitro group, making the formed carbanion not reactive enough to drive the reaction in progress.
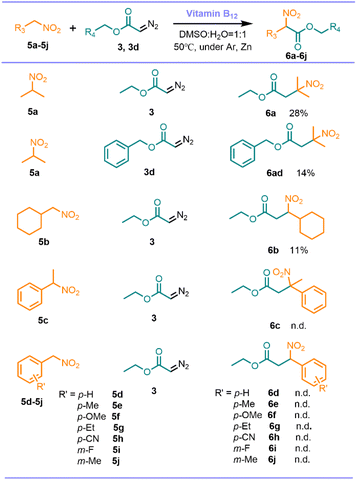 |
| Scheme 5 Scope and limitation. Reaction conditions: nitrocyclohexane (0.25 mmol), diazo compounds (2 equiv.), Zn (6 equiv.), vitamin B12 (2 mol%), solvent (2.5 mL), 24 h. | |
In order to gain insight into the mechanism of the reaction, a variety of experiments were conducted (Scheme 6). Firstly, TEMPO, a radical scavenger, was added to the reaction mixture, which halted the reaction, suggesting a radical mechanism. Furthermore, when vitamin B12 was completely reduced, only EDA was added to the reaction at the room temperature, the ESI MS spectra of the crude reaction mixture showed a peak at 1415.60, confirming the generation of alkyl cobalamin intermediate (8). In this reaction, an additional proton in the product originates from H2O, which was experimentally verified by H/D exchange experiments (see ESI† for detailed information).
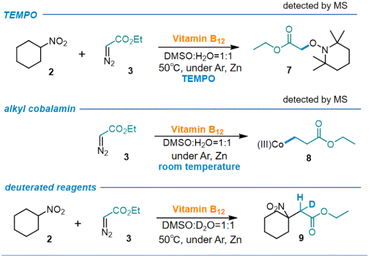 |
| Scheme 6 Mechanistic studies. | |
On the basis of the experiments described above and previous studies,28,32,33 we have proposed a mechanism for the formation of C–C bonds (Scheme 7). Zn acts as an additional reducing agent to reduce vitamin B12 to the catalytically active hypernucleophilic Co(I) (11), which reacted with EDA to produce an alkyl cobalt ester (8). The reaction is carried out under heating conditions at 50 °C, with sufficient energy to allow homolysis of the C–Co bond to give the alkyl radical (B). Subsequently, the alkyl radical can undergo an addition reaction with the in situ-generated nitronate (C) to give the nitro anion (E), while the construction of a new C–C bond is completed, leading to the tertiary nitroalkanes (F) by one-electron oxidation.
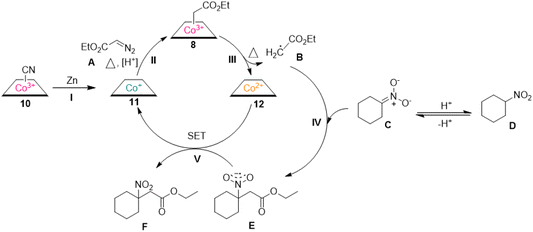 |
| Scheme 7 Plausible mechanism for the vitamin B12 catalyzed coupling reaction. | |
Nitro compounds are typical intermediates in organic chemistry that can be transformed directly into other functional groups. We use the product tertiary nitroalkane 4 as a precursor to be reduced to the corresponding tertiary amine 13 (Scheme 8), which is an important structural unit in the synthesis of many drugs and peptides. Thus, the coupling product can be used to create a broader range of compounds.
 |
| Scheme 8 Product derivatization. | |
Conclusions
We have demonstrated for the first time that vitamin B12, a natural and environmentally friendly catalyst, can effectively catalyze the C–H insert reaction of nitroalkanes with diazo compounds featuring a C(sp3)–C(sp3) bond-forming step to construct tetrasubstituted centers. Under the developed conditions, diazo compounds form nucleophilic radicals and then react with nitroalkanes leading to the C–C bond formation. This methodology expands the chemical toolbox of transformations for EDA and nitroalkanes.
Methods
General information
All commercial reagents and solvents are to be used as received unless otherwise indicated. Reaction process monitoring using thin-layer chromatography (TLC) on Yantai Chemical Industry Research Institute silica gel (GF254, 0.23 mm thickness). Observations were made using UV light (254 nm) or potassium permanganate for color development. The crude product was separated and purified by column chromatography using Qingdao Ocean Chemical silica gel (200–300 mesh). 1H and 13C NMR spectra were recorded at ambient temperature on a Bruker 400 MHz instrument with TMS as an internal standard. Liquid chromatograph mass spectrums (LC-MS) data were obtained on an Water Xevo G2 QTof Liquid Chromatograph Mass Spectrometer and a SilGreen 100 C18 column.
General experimental procedure for the synthesis of the coupling products
The reactions were carried out under an argon atmosphere unless otherwise indicated. Vitamin B12 (2 mol%, 7 mg), Zn (196 mg, 6.0 mmol) were accurately weighed in a 10 mL two-necked flask using an electroanalytical balance. Degassed dimethyl sulfoxide and water (DMSO
:
H2O = 1
:
1, 2.5 mL) were added to the reaction flask, and the reaction mixture was stirred vigorously under argon gas at 50 °C until the vitamin B12 was completely reduced (colour changed from red to green). Nitroalkanes (0.25 mmol, 1.0 equiv.) and diazo compounds (0.50 mmol, 2.0 equiv.) dissolved in dimethyl sulfoxide were added to the reaction system with a syringe and the reaction was carried out for 24 h.
Zn needs to be activated before use. The activation process was as follows: (1) grinding in 10% HCl, (2) washing with water, methanol and water, (3) drying in vacuum. Activation of Zn has a great influence on the reaction yield.
2-(1-Nitrocyclohexyl) ethyl acetate (4). 1H NMR (400 MHz, chloroform-d) δ 4.13 (q, 2H), 2.91 (s, 2H), 2.35–2.29 (m, 2H), 1.88–1.83 (m, 2H), 1.62–1.38 (m, 6H), 1.22 (t, 3H). 13C NMR (101 MHz, chloroform-d) δ 168.62, 88.50, 61.02, 42.83, 34.27, 24.60, 22.29, 14.02. HRMS (ESI): [M + H]+ (C10H18NO4) calculated: 216.1230, found: 216.1217.
Isopropyl 2-(1-nitrocyclohexyl) acetate (4b). 1H NMR (400 MHz, chloroform-d) δ 5.04–4.94 (m, 1H), 2.89 (s, 2H), 2.37–2.31 (m, 2H), 1.90–1.84 (m, 2H), 1.61–1.41 (m, 6H), 1.22 (d, J = 6.3 Hz, 6H). 13C NMR (101 MHz, chloroform-d) δ 168.13, 88.55, 68.75, 43.23, 34.30, 24.64, 22.30, 21.65. HRMS (ESI): [M + H]+ (C11H20NO4) calculated: 230.1387, found: 230.1385.
Tert-butyl 2-(1-nitrocyclohexyl) acetate (4c). 1H NMR (400 MHz, chloroform-d) δ 2.83 (s, 2H), 2.35–2.30 (m, 2H), 1.88–1.82 (m, 2H), 1.63–1.49 (m, 6H), 1.42 (s. 9H). 13C NMR (101 MHz, chloroform-d) δ 167.82, 88.58, 81.88, 44.31, 34.32, 27.90, 24.67, 22.30. HRMS (ESI): [M + H]+ (C12H22NO4) calculated: 244.1543, found: 244.1548.
Benzyl 2-(1-nitrocyclohexyl) acetate (4d). 1HNMR (400 MHz, chloroform-d) δ 7.36–7.32 (m, 5H), 5.11 (s, 2H), 2.99 (s, 2H), 2.37–2.30 (m, 2H), 1.91–1.84 (m, 2H), 1.60–1.41 (m, 6H). 13C NMR (101 MHz, chloroform-d) δ 168.54, 135.26, 128.62, 128.46, 128.38, 88.52, 66.91, 42.65, 34.28, 24.60, 22.31. HRMS (ESI): [M + H]+ (C15H20NO4) calculated: 278.1387, found: 278.1386.
Ethyl 3-methyl-3-nitrobutanoate (6a). 1H NMR (400 MHz, chloroform-d) δ 4.15 (q, J = 7.1 Hz, 2H), 2.97 (s, 2H), 1.69 (s, 6H), 1.25 (t, J = 7.1 Hz, 3H). 13C NMR (101 MHz, chloroform-d) δ 168.83, 84.95, 61.05, 44.00, 26.31, 14.05.
3-Methyl-3-nitrobutyric acid benzyl ester (6ad). 1H NMR (400 MHz, chloroform-d) δ 7.40–7.32 (m, 5H), 5.13 (s, 2H), 3.03 (s, 2H), 1.69 (s, 6H). 13C NMR (101 MHz, chloroform-d) δ 168.70, 135.26, 128.64, 128.49, 128.36, 84.94, 66.86, 43.89, 26.33. HRMS (ESI): [M + H]+ (C12H16NO4) calculated: 238.1074, found: 238.1088.
Ethyl-3-cyclohexyl-3-nitropropanoate (6b). 1H NMR (400 MHz, chloroform-d) δ 4.73 (ddd, 1H), 4.13 (q, 2H), 3.10 (dd, 1H), 2.66 (dd, 1H), 1.91–1.76 (m, 1H), 1.69–1.63 (m, 5H), 1.26–1.02 (m, 8H). 13C NMR (101 MHz, chloroform-d) δ 169.75, 88.16, 61.24, 41.33, 34.37, 29.17, 28.53, 25.73, 14.00. HRMS (ESI): [M + H]+ (C11H20NO4) calculated: 230.1387, found: 230.1382.
Data availability
All data in this study are included in this article and its ESI.†
Author contributions
Z. Z. performed the study, and manuscript writing. M. C. conceived the project, performed the study and revised the manuscript. G. Z. supervised the project, approved and submitted the final manuscript. All authors have read and agreed to the published version of the manuscript.
Conflicts of interest
The authors declare no conflict of interest.
Acknowledgements
This work was supported by the National Key R&D Program of China No. 2021YFC2102900, No. 2021YFC2101503 and Beijing Natural Science Foundation No. L212001.
Notes and references
- Y. Chen and X. P. Zhang, J. Org. Chem., 2004, 69, 2431–2435 CrossRef CAS PubMed.
- F. Watanabe, Exp. Biol. Med., 2007, 232, 1266–1274 CrossRef CAS PubMed.
- P. H. Degnan, M. E. Taga and A. L. Goodman, Cell Metab., 2014, 20, 769–778 CrossRef CAS PubMed.
- T. Wdowik and D. Gryko, ACS Catal., 2022, 12, 6517–6531 CrossRef CAS.
- M. Giedyk, K. Goliszewska and D. Gryko, Chem. Soc. Rev., 2015, 44, 3391–3404 RSC.
- S. Smolen, A. Wincenciuk, O. Drapala and D. Gryko, Synthesis, 2021, 53, 1645–1653 CrossRef CAS.
- K. R. Dworakowski, S. Pisarek, S. Hassan and D. Gryko, Org. Lett., 2021, 23, 9068–9072 CrossRef CAS PubMed.
- J. Demarteau, A. Debuigne and C. Detrembleur, Chem. Rev., 2019, 119, 6906–6955 CrossRef CAS PubMed.
- K. ó. Proinsias, A. Jackowska, K. Radzewicz, M. Giedyk and D. Gryko, Org. Lett., 2018, 20, 296–299 CrossRef CAS PubMed.
- M. Karczewski, M. Ociepa, K. Pluta, K. ó. Proinsias and D. Gryko, Chem.–Eur. J., 2017, 23, 7024–7030 CrossRef CAS PubMed.
- A. Potrząsaj, M. Musiejuk, W. Chaładaj, M. Giedyk and D. Gryko, J. Am. Chem. Soc., 2021, 143, 9368–9376 CrossRef PubMed.
- H. Sharghi, M. Aali Hosseini, J. Aboonajmi and M. Aberi, ACS Sustainable Chem. Eng., 2021, 9, 11163–11170 CrossRef CAS.
- F. Zelder, Chem. Commun., 2015, 51, 14004–14017 RSC.
- T. Koide, T. Ono, H. Shimakoshi and Y. Hisaeda, Coord. Chem. Rev., 2022, 470, 214690 CrossRef CAS.
- C. K. Prier, R. K. Zhang, A. R. Buller, S. Brinkmann-Chen and F. H. Arnold, Nat. Chem., 2017, 9, 629–634 CrossRef CAS.
- Q.-F. Wu, P.-X. Shen, J. He, X.-B. Wang, F. Zhang, Q. Shao, R.-Y. Zhu, C. Mapelli, J. X. Qiao, M. A. Poss and J.-Q. Yu, Science, 2017, 355, 499–503 CrossRef CAS PubMed.
- G. Chen, W. Gong, Z. Zhuang, M. S. Andrä, Y.-Q. Chen, X. Hong, Y.-F. Yang, T. Liu, K. N. Houk and J.-Q. Yu, Science, 2016, 353, 1023–1027 CrossRef CAS.
- F.-L. Zhang, K. Hong, T.-J. Li, H. Park and J.-Q. Yu, Science, 2016, 351, 252–256 CrossRef CAS PubMed.
- G. Zhang, Y. Zhang and R. Wang, Angew. Chem., Int. Ed., 2011, 50, 10429–10432 CrossRef CAS PubMed.
- S. Erfan Masaeli, M. Teimouri, B. Adhikari, M. Attarroshan, J. W. Akin, S. Raju, S. L. Stokes and J. P. Emerson, Tetrahedron Lett., 2023, 122, 154520 CrossRef CAS PubMed.
- M. Teimouri, S. Raju, E. Acheampong, A. N. Schmittou, B. Donnadieu, D. O. Wipf, B. S. Pierce, S. L. Stokes and J. P. Emerson, Molecules, 2024, 29, 730 CrossRef CAS PubMed.
- S. Dongbang, Organometallics, 2024, 43(16), 1662–1681 CrossRef CAS.
- F. Mo, D. Qiu, L. Zhang and J. Wang, Chem. Rev., 2021, 121, 5741–5829 CrossRef CAS PubMed.
- K. A. Mix, M. R. Aronoff and R. T. Raines, ACS Chem. Biol., 2016, 11, 3233–3244 CrossRef CAS.
- Z. Zhang and V. Gevorgyan, Chem. Rev., 2024, 124, 7214–7261 CrossRef CAS PubMed.
- Z. Zhang and J. Wang, Tetrahedron, 2008, 64, 6577–6605 CrossRef CAS.
- D. Zhao, J. H. Kim, L. Stegemann, C. A. Strassert and F. Glorius, Angew. Chem., Int. Ed., 2015, 54, 4508–4511 CrossRef CAS PubMed.
- M. Giedyk, K. Goliszewska, K. O. Proinsias and D. Gryko, Chem. Commun., 2016, 52, 1389–1392 RSC.
- P. G. Gildner, A. A. S. Gietter, D. Cui and D. A. Watson, J. Am. Chem. Soc., 2012, 134, 9942–9945 CrossRef CAS PubMed.
- S. E. Milner, T. S. Moody and A. R. Maguire, Eur. J. Org Chem., 2012, 2012, 3059–3067 CrossRef CAS.
- D. K. Romney, N. S. Sarai and F. H. Arnold, ACS Catal., 2019, 9, 8726–8730 CrossRef CAS PubMed.
- H. Fu, T. Qiao, J. M. Carceller, S. N. MacMillan and T. K. Hyster, J. Am. Chem. Soc., 2023, 145, 787–793 CrossRef CAS.
- H. Fu, J. Cao, T. Qiao, Y. Qi, S. J. Charnock, S. Garfinkle and T. K. Hyster, Nature, 2022, 610, 302–307 CrossRef CAS PubMed.
|
This journal is © The Royal Society of Chemistry 2024 |
Click here to see how this site uses Cookies. View our privacy policy here.