DOI:
10.1039/D4RA05177G
(Paper)
RSC Adv., 2024,
14, 34668-34678
Application of magnetic deep eutectic solvents as an efficient catalyst in the synthesis of new 1,2,3-triazole-nicotinonitrile hybrids via a cooperative vinylogous anomeric-based oxidation†
Received
17th July 2024
, Accepted 13th October 2024
First published on 29th October 2024
Abstract
Magnetic deep eutectic solvents (MDESs) are adjuvants and an emerging subclass of heterogeneous catalysts in organic transformations. Herein, choline chloride (Ch/Cl) embedded on naphthalene bis-urea-supported magnetic nanoparticles, namely, Fe3O4@SiO2@DES1, was constructed by a special approach. This compound was scrutinized and characterized by instrumental techniques such as FTIR, thermogravimetry and derivative thermogravimetry (TGA/DTG), field emission scanning electron microscopy (FESEM), energy-dispersive X-ray spectroscopy (EDS), elemental mapping, vibrating sample magnetometer (VSM) and X-ray diffraction (XRD) analyses. Potential catalytic activity of Fe3O4@SiO2@DES1 was impressive, facilitating the synthesis of new 1,2,3-triazole-nicotinonitrile hybrids via a multicomponent method with 65–98% yields. Enhanced rates, high yields, mild reaction conditions, and recycling and reusability of Fe3O4@SiO2@DES1 are the distinct benefits of this catalytic organic synthetic methodology.
Introduction
Deep eutectic solvents (DESs), one of the progressive families of ionic liquids, are composed of binary or ternary mixtures of compounds that can associate mainly via hydrogen bond interactions.1–3 Due to the brilliant, useful, and efficient properties of DESs, these materials are excellent alternatives to conventional organic solvents and classic ionic liquids.4–6 DESs have easy synthetic routes, and they are biodegradable, cheap and non-flammable.7 The applications of these materials, including catalytic applications,8 separation processes,9 biotechnology,10 material sciences11 and environmental protection,12 have been significantly developed. In recent years, DESs have been widely used in many catalytic transformations.13–15 Their intrinsic catalytic activity, designability and excellent stability have a robust partnership in catalytic oxidations,16 CO2 fixation utilities,17 multicomponent reactions,18 desulfurization19 and coupling reactions.20 Urea derivatives, as an impactful component of DESs, act as hydrogen bond donors in these systems.21 Due to the inherent catalytic activity of urea-based compounds as organocatalysts, these moieties enhance the catalytic potential of DESs.22,23 Very recently, a new class of hybrid DESs, namely, eutectogels, was reported for the catalytic synthesis of pyridines and chromenes.24
Recently, the incorporation of magnetic nanoparticles (MNPs) with DESs to achieve unique heterogeneous catalytic systems has attracted great attention, which have suitable properties for various purposes.25 Since the recovery and reusability of DESs are problematic, when these compounds are decorated on the surface of MNPs, their defects get resolved.26 Considering the substantial research carried out on homogeneous DESs, designing and constructing these materials in the heterogeneous phase will endow them with new properties and advantages for their effective performance. Furthermore, heterogeneous DESs will not only retain many of the benefits of homogeneous DESs, but can also be easily separated from the reaction mixture. Considering these distinctions, magnetic DESs is a promising approach in various academic and industrial fields.27,28 The modification of MNPs by ionic liquids and DESs can enhance their catalytic utilities.27 Magnetic DESs provide more variations and efficiencies to most of the catalysts. On account of the environmentally friendly nature, high activity, easy recoverability and reusability as well as good stability of heterogeneous DESs, these materials can be robust and fruitful catalysts. In the last years, MNP-decorated DESs were used as heterogeneous catalysts in many organic reactions such as coupling reactions,28 reduction of aldehydes,29 and multicomponent reactions.30,31 Hence, the construction and applications of magnetic DESs have a developing trend and deserve more attention. Several catalytic applications of magnetic DESs in the multicomponent reactions are observed in Scheme 1.
 |
| Scheme 1 Several catalytic applications of magnetic DESs in multicomponent reactions. | |
According to the literature, pyridine-containing heterocycles have a brilliant background in many studies such as functional materials, biology, medicinal, agriculture, pharmaceutical molecules, and many miscellaneous areas.38–40 In an intensive approach, pyridines are widely used in supramolecular chemistry,41 polymers,42 coordination chemistry,43 chemosensors,44 surfactants,45 catalysts,46 organic dyes,47 etc. In addition, fabulous medicinal properties of pyridine cores such as anticancer,48 anti-inflammatory,48 anti-Parkinsonian,49 antidepressant,50 NADP−NADPH coenzymes51 and Alzheimer's diseases52 are interesting. Particularly, N-heterocyclic compounds with the nicotinonitrile moiety are one of the prominent families of pyridines.53,54 These substrates are present in many drugs. For example, anticancer, antimicrobial, antiproliferative and antihistaminic are only a few of the medicinal properties of these compounds.55–57 Very recently, the chemistry of the pyridine scaffold and its synthesis and applications as biologically active compound was comprehensively reviewed.58
Triazole scaffolds, as another type of N-heterocyclic compound with fundamental and superior applications in vast domains of chemistry such as metal complexes, energetic materials, chemo-sensors, pharmaceuticals, and dyes, have gained significant importance.59–61 Nevertheless, the most important use of triazoles is their pharmaceutical utilities such as antidepressant,62 anticancer,62 antibacterial63 and antiviral agents.64 Recently, hybrid heterocyclic materials, due to their extraordinary properties and synergistic effects in medicinal fields, have been increasingly explored.65 Due to the synergistic effect of pyridine-triazole hybrids to control the disease agents, much attention has been paid to these materials and several papers have reported on pyridine-triazole hybrids.66 Nonetheless, the 1,2,3-triazole-nicotinonitrile hybrid deserves more attention.
Considering that the majority of our focus is on the design and synthesis of hybrid pyridines,67 herein, the catalytic synthesis of a new 1,2,3-triazole-nicotinonitrile hybrid using Fe3O4@DES1 as the magnetic DES was investigated (Scheme 2 and 3). Moreover, the role of a cooperative vinylogous anomeric-based oxidation (CVABO) as a stereoelectronic effect in the synthesis of pyridine rings was also studied.68
 |
| Scheme 2 Schematic of Fe3O4@SiO2@DES1 synthesis. | |
 |
| Scheme 3 Illustration of the synthesis of 1,2,3-triazole-nicotinonitrile derivatives. | |
Results and discussion
To the best of our knowledge, pyridine is one of the most famous scaffolds that is present as the structural core of various chemical, biological, and medicinal heterocycles and natural products. The intrinsic chemical and physical properties of pyridine make it a suitable core to prepare a wide range of suitable derivatives, including vitamins, dyes, agrochemicals, pesticides, adhesives, and biologically active compounds. Singh has published a book entitled “Recent Developments in the Synthesis and Application of Pyridines” with the collaboration of other authors such as us.48 In continuation of our pervious investigation on the synthesis of hybrid pyridines,60,64,65 we decided to design, synthesize and apply Fe3O4@SiO2@DES1 for the catalytic preparation of a new category of pyridine derivatives. At first, the characterization of the described catalyst will be discussed.
The synthesized Fe3O4@SiO2@DES1 was precisely characterized by several instrumental methodologies including FTIR, EDS, mapping, FESEM, VSM and XRD. FTIR spectroscopy were used to approve the chemical structure of Fe3O4@SiO2@DES1 and its related intermediate. In the FTIR spectrum of Fe3O4@SiO2 (Fig. 1), the characteristic peaks of Fe–O, Si
O and free hydroxy groups are obtained at 586, 1111 and 3378 cm−1, respectively. In the FTIR spectrum of Ligand 1, strong peaks are shown at 3306, 1626 and 1576 cm−1 arising from the NH, C
O and C
C stretching bands, respectively. The C
O and C
C stretching vibration for Fe3O4@SiO2@Ligand 1 appeared at 1634 and 1540 cm−1, respectively, and these confirm that the Ligand 1 is successfully supported at the Fe3O4@SiO2 surface. Similarly, in the FTIR spectrum of Fe3O4@SiO2@DES1, the characteristic peaks at 1635 and 1534 confirm the successful synthesis of the catalyst.
 |
| Fig. 1 FTIR spectra of Fe3O4@SiO2, Ligand 1, Fe3O4@SiO2@Ligand 1 and Fe3O4@SiO2@DES1. | |
EDS/mapping is a key analysis to show the successful construction of the DES system. The presence of Ch/Cl on the surface of Fe3O4@SiO2 (Cl as key part of DES) was confirmed by EDS/mapping analyses. Anyway, the presence and uniform distribution of other expected elements including Fe, Si, O, C and N related to previous steps of catalyst synthesis were well confirmed by the EDS signals and mapping images of Fe3O4@SiO2@DES1 (Fig. 2).
 |
| Fig. 2 EDS/mapping analyses of Fe3O4@SiO2@DES1 | |
FESEM images were used to investigate the morphology and size of the synthesized catalyst. These images revealed that Fe3O4@SiO2@DES1 has uniform spherical morphology, and these particles are in the size range of 26–44 nm. The magnetic nature of the catalyst leads to the creation of interwoven single spherical particles with sizes smaller than 40 nm (Fig. 3). In addition, the crystalline structure of Fe3O4@SiO2@DES1 was investigated by XRD analysis. The characteristic signals at 30, 35, 43, 53, 57 and 62° for the catalyst correspond to the cubic geometry for Fe3O4. The broad peak at 20–25° is related to the SiO2 groups Moreover, a decrease in the intensity of peaks for Fe3O4@SiO2@DES1 compared to Fe3O4 is because of the presence of the organic and inorganic layers on the surface of Fe3O4. Nonetheless, after the decoration of Fe3O4@SiO2 by organic layers, its geometry is preserved (Fig. 4).
 |
| Fig. 3 FESEM images of Fe3O4@SiO2@DES1 | |
 |
| Fig. 4 XRD patterns of (a) Fe3O4 and (b) Fe3O4@SiO2@DES1 | |
In another study, VSM analysis was achieved from Fe3O4, Fe3O4@SiO2, and Fe3O4@SiO2@DES1 for the determination of their magnetic properties. The magnetic value of the described catalyst is 7.2 emu g−1, which is significantly less than the magnetic value of Fe3O4 and Fe3O4@SiO2. Based on the achieved data, the organic layers are well supported on the surface of magnetic cores and Fe3O4@SiO2@DES1 has a considerable magnetic value (Fig. 5). TGA analysis indicates that Fe3O4@SiO2@DES1 can be stable up to 325 °C and retain 80% of its initial mass even at 600 °C under N2. The first weight loss in the range of 325–383 °C is related to the removal of Ch/Cl,69 and the second weight loss at 513 °C is because of the decomposition of organic layers, which is linked to surface of Fe3O4@SiO2 by covalent bonds (Fig. 6).
 |
| Fig. 5 VSM curves of Fe3O4 (a), Fe3O4@SiO2 (b) and Fe3O4@SiO2@DES1 (c). | |
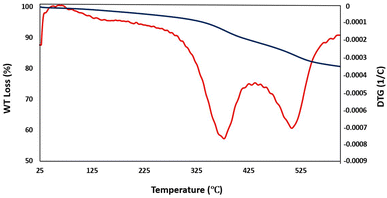 |
| Fig. 6 TGA/DTG curves of Fe3O4@SiO2@DES1. | |
After the characterization of Fe3O4@SiO2@DES1, it was used as a heterogeneous catalyst for the synthesis of new 1,2,3-triazole-nicotinonitrile hybrids. To improve the methodology of the synthesis of Fe3O4@SiO2@DES1, the effects of the temperature, solvent and amount of catalyst were explored. For this goal, the synthesis of 1f molecule was used as a model reaction (Table 1). With respect to the influence of the temperature, several temperatures were applied for the synthesis of 1f. According to the obtained results, 120 °C was selected as the optimum temperature. With respect to the influence of the solvent, the model reaction was done under solvent-free and reflux conditions. Several solvents such as CH3OH, CHCl3, EtOAc, acetone, CH3CN, and EtOH were used, but solvent-free conditions gave the best result. Finally, the amount of the catalyst was examined, wherein 10 mg of the catalyst was selected as the optimum value.
Table 1 Optimization of reaction conditions for the synthesis of 1fa

|
Entry |
Solvent |
Catalyst loading (mg) |
Temperature (°C) |
Time (min) |
Yieldb (%) |
Reaction conditions: 4-chlorobenzaldehyde (1 mmol, 0.14 g), 1-(5-methyl-1-(4-nitrophenyl)-1H-1,2,3-triazol-4-yl)ethan-1-one (1 mmol, 0.246 g), malononitrile (1 mmol, 0.066 g); ammonium acetate (1.5 mmol, 0.078 g). Isolated yield. |
1 |
— |
5 |
130 |
20 |
78 |
2 |
— |
10 |
120 |
20 |
94 |
3 |
— |
20 |
120 |
20 |
94 |
4 |
— |
— |
120 |
60 |
52 |
5 |
— |
10 |
120 |
30 |
54 |
6 |
— |
10 |
90 |
30 |
46 |
10 |
CH3OH |
10 |
Reflux |
60 |
88 |
11 |
CHCl3 |
10 |
Reflux |
60 |
64 |
12 |
EtOAc |
10 |
Reflux |
60 |
72 |
13 |
Acetone |
10 |
Reflux |
60 |
56 |
14 |
CH3CN |
10 |
Reflux |
60 |
50 |
15 |
EtOH |
10 |
Reflux |
60 |
78 |
In continuation, we are interested in developing the generality of catalytic performance of Fe3O4@SiO2@DES1 for the synthesis of a new library of 1,2,3-triazole-nicotinonitrile hybrids. Hence, several aromatic aldehydes bearing electron-withdrawing and electron-releasing substituents were used for this purpose. The achieved products were synthesized in the clean reaction and have high yield as well as short reaction times (Table 2).
Table 2 Synthesis of 1,2,3-triazole-nicotinonitrile hybrids in the presence of Fe3O4@SiO2@DES1 as a catalysta
Reaction conditions: aldehyde (1 mmol), 1-(5-methyl-1-(4-nitrophenyl)-1H-1,2,3-triazol-4-yl)ethan-1-one (1 mmol, 0.246 g), malononitrile (1 mmol, 0.066 g), ammonium acetate (1.5 mmol, 0.078 g), solvent-free, 120 °C, catalyst = 10 mg, reported yields refer to the isolated yields. |
 |
To explore the important role of the components of Fe3O4@SiO2@DES1, the 1f molecule was synthesized using Fe3O4, Fe3O4@SiO2, Ch/Cl and Ligand 1 was compared to Fe3O4@SiO2@DES1. According to the obtained results, each of these components can have a catalytic role to some extent. The free hydroxy groups on the surface of Fe3O4 and Fe3O4@SiO2 have significant catalytic activity, and the ionic structure of Ch/Cl enhances its catalytic performance. Moreover, urea segments in Ligand 1 can act as a hydrogen-bonding catalyst. Therefore, the synergistic effects of these parts can improve the catalytic potential of Fe3O4@SiO2@DES1 (Table 3).
Table 3 Investigation of the catalytic performance of Fe3O4@SiO2@DES1 compared to Fe3O4, Fe3O4@SiO2, Ligand 1 and Ch/Cl upon the preparation of 1fa
Entry |
Catalyst |
Load of catalyst |
Yield (%) |
Reaction conditions: 4-Cl-benzaldehyde (1 mmol, 0.14 g), 1-(5-methyl-1-(4-nitrophenyl)-1H-1,2,3-triazol-4-yl)ethan-1-one (1 mmol, 0.246 g), malononitrile (1 mmol, 0.066 g); ammonium acetate (1.5 mmol, 0.078 g). Solvent-free, 120 °C. |
1 |
Fe3O4@SiO2@DES1 |
10 mg |
94 |
2 |
Fe3O4 |
10 mg |
30 |
3 |
Fe3O4@SiO2 |
10 mg |
40 |
4 |
Ligand 1 |
10 mol% |
35 |
|
Ch/Cl |
10 mol% |
50 |
Scheme 4 exhibits the suggested mechanism for the synthesis of 1,2,3-triazole-nicotinonitrile hybrids using Fe3O4@SiO2@DES1 catalyst. The Knoevenagel reaction using activated benzaldehyde and malononitrile as the starting materials yields intermediate a. Besides, ammonia, derived from the in situ dissociation of ammonium acetate, reacts with 1-(5-methyl-1-(4-nitrophenyl)-1H-1,2,3-triazol-4-yl)ethan-1-one and intermediate b is produced. Hereafter, these intermediates thus formed undergo condensation reaction to yield intermediate c. Then, due to the intramolecular nucleophilic attack and tautomerization processes, intermediate f was performed. Then, the final product was achieved via the cooperative vinylogous anomeric-based oxidation (CVABO)66–68,70 of dihydropyridine intermediates and their conversion to the corresponding pyridines.
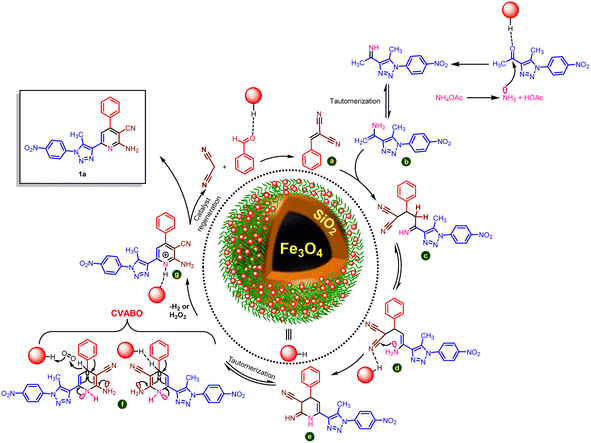 |
| Scheme 4 Suggested mechanistic route for the synthesis of 1,2,3-triazole-nicotinonitrile hybrids. | |
The recycling and reusability of Fe3O4@SiO2@DES1 was investigated using the model reaction. After running the model reaction each time, the reaction mixture was dissolved in DMF, and the catalyst was separated by an external magnet and washed with EtOH three times. The separated catalyst was dried and used the next time. Finally, it was found that the catalyst can be recovered and reused up to 5 times, and a slight drop in yield of the product is observed (Fig. 7). The recovered catalyst was characterized using several methods such as XRD, EDS/mapping and FESEM analyses (see in the ESI†).
 |
| Fig. 7 Recycling and reusability test of Fe3O4@SiO2@DES1 upon model reaction. | |
Experimental section
The required precursors were synthesized according to our recently reported educational synthetic organic theory.71
Synthesis of Ligand 1
Initially, naphthalene-1,5-diamine (1 mmol, 0.158 g) and triethoxy(3-isocyanatopropyl)silane (2 mmol, 0.495 g) were added to a 10 mL round-bottomed flask and stirred under solvent-free condition at 50 °C for 24 h. After that, the white solid that appeared was washed with a mixture of n-hexane and EtOAc (5/1, v/v) to give a pure product.
Synthesis of Fe3O4@SiO2@DES1
First and foremost, Fe3O4@SiO2 was prepared according to the previously reported synthetic method.72 Then, 1 g of Fe3O4@SiO2, Ligand 1 (2 mmol, 0.652 g) and 90 mL of dry toluene were poured into a 100 mL round-bottomed flask and sonicated for 15 min. Then, it was refluxed for 48 h at 110 °C. The remaining solid was separated by an external magnet and washed with EtOH. The separated precipitate was dried to yield Fe3O4@SiO2@Ligand 1. In the next step, 1 g of Fe3O4@SiO2@Ligand 1, Ch/Cl (4 mmol, 0.56 g) and 50 mL toluene were mixed into a 100 mL round-bottomed flask and again refluxed for 12 h. Finally, Fe3O4@SiO2@DES1 was separated by an external magnet. The separated solid was washed with EtOH and dried at 80 °C.
Synthesis of 1,2,3-triazole-nicotinonitrile hybrids in the presence of Fe3O4@SiO2@DES1
In the first step, 1-(5-methyl-1-(4-nitrophenyl)-1H-1,2,3-triazol-4-yl)ethan-1-one was prepared according to the literature.73 Then, the aryl aldehyde derivatives (1 mmol), 1-(5-methyl-1-(4-nitrophenyl)-1H-1,2,3-triazol-4-yl)ethan-1-one (1 mmol, 0.246 g), malononitrile (1 mmol, 0.066 g) and ammonium acetate (1.5 mmol, 0.078 g) were mixed into a 10 mL round bottomed flask containing 10 mg Fe3O4@SiO2@DES1 as the catalyst and stirred vigorously under solvent-free conditions at 120 °C. At the end of the reaction, the catalyst was separated from the reaction mixture using DMF. Then, cold water was added to the DMF solution containing organic compounds, which led to the appearance of a bulk solid. Finally, the desired products were purified by recrystallization in 10 mL acetonitrile.
Spectral data
1,1′-(Naphthalene-1,5-diyl)bis(3-(3-(triethoxysilyl)propyl)urea) (Ligand 1). FTIR (KBr, ν, cm−1): 3317, 2928, 1633, 1581, 1497, 1103, 1079. 1H NMR (400 MHz, DMSOd6) δppm 8.43 (s, 2H), 8.00 (d, J = 8 Hz, 2H), 7.71 (d, J = 8 Hz, 2H), 7.44 (t, J = 8 Hz, 2H), 6.63 (t, J = 5.8 Hz, 2H), 3.77 (q, J = 7.0 Hz, 12H), 3.12 (q, J = 8 Hz, 4H), 1.56–1.47 (m, 4H), 1.16 (t, J = 8 Hz, 18H), 0.63–0.59 (m, 4H).
2-Amino-6-(5-methyl-1-(4-nitrophenyl)-1H-1,2,3-triazol-4-yl)-4-phenylnicotinonitrile (1a). M.p. = 233–235 °C, FTIR (KBr, ν, cm−1): 3495, 3371, 2213, 1625, 1584, 1348. 1H NMR (400 MHz, DMSOd6) δppm 8.50 (d, J = 8 Hz, 2H), 8.05–8.03 (m, 3H), 7.68–7.58 (m, 4H), 7.46 (s, 1H), 7.17 (s, 2H), 2.85 (s, 3H). 13C NMR (101 MHz, DMSOd6) δppm 160.9, 154.6, 153.4, 147.7, 141.8, 140.4, 136.8, 135.3, 129.7, 128.9, 128.1, 126.4, 125.1, 116.9, 108.9, 86.1, 10.5. MS (m/z) = calcd for C21H15N7O2: 397.40, found: 398.219.
2-Amino-6-(5-methyl-1-(4-nitrophenyl)-1H-1,2,3-triazol-4-yl)-4-(p-tolyl) nicotinonitrile (1b). M.p. = 215–217 °C, FTIR (KBr, ν, cm−1): 3505, 3381, 2209, 1728, 1614, 1590, 1350. 1H NMR (400 MHz, DMSOd6) δppm 8.50 (d, J = 8 Hz, 2H), 8.04 (d, J = 12 Hz, 2H), 7.57 (d, J = 12 Hz, 2H), 7.44 (s, 1H), 7.39 (d, J = 8 Hz, 2H), 7.13 (s, 2H), 2.83 (s, 3H), 2.42 (s, 3H). MS (m/z) = calcd for C22H17N7O2: 411.42, found: 412.242.
2-Amino-6-(5-methyl-1-(4-nitrophenyl)-1H-1,2,3-triazol-4-yl)-4-(4-methoxyphenyl) nicotinonitrile (1c). M.p. = 270–271 °C, FTIR (KBr, ν, cm−1): 3505, 3395, 2209, 1603, 1558, 1345. 1H NMR (400 MHz, DMSOd6) δppm 8.51 (broad peak, 2H), 8.10–7.96 (m, 2H), 7.65 (s, 2H), 7.45 (s, 1H), 7.25–6.99 (m, 4H), 3.87 (s, 3H), 2.85 (d, J = 8.2 Hz, 3H). 13C NMR (101 MHz, DMSOd6) δppm 161.0, 154.2, 153.2, 147.7, 141.8, 135.1, 131.6, 129.7, 128.9, 126.4, 126.3, 125.1, 117.2, 114.3, 108.7, 85.8, 55.3, 10.5. MS (m/z) = calcd for C22H17N7O3: 427.42, found: 427.300.
2-Amino-6-(5-methyl-1-(4-nitrophenyl)-1H-1,2,3-triazol-4-yl)-4-(naphthalen-1-yl)nicotinonitrile (1d). M.p. = 266–270 °C, FTIR (KBr, ν, cm−1): 3501, 3399, 2209, 1610, 1565, 1523, 1338. 1H NMR (400 MHz, DMSOd6) δppm 8.50 (d, J = 8 Hz, 2H), 8.13–8.03 (m, 4H), 7.70–7.56 (m, 5H), 7.22 (s, 2H), 2.88 (s, 3H). 13C NMR (101 MHz, DMSOd6) δppm 160.42, 154.0, 153.1, 147.7, 141.8, 140.3, 135.4, 134.7, 133.1, 129.9, 129.4, 128.6, 127.1, 126.6, 126.5, 126.4, 125.5, 125.1, 124.5, 116.3, 112.4, 110.5, 88.6, 10.5. MS (m/z) = calcd for C25H17N7O2: 447.46, found: 447.000.
2-Amino-4-(1H-indol-3-yl)-6-(5-methyl-1-(4-nitrophenyl)-1H-1,2,3-triazol-4-yl)nicotinonitrile (1e). M.p. > 300 °C FTIR (KBr, ν, cm−1): 3353, 3205, 2210, 1662, 1590, 1422, 1344. 1H NMR (400 MHz, DMSOd6) δppm 11.88 (s, 1H), 8.53 (broad peak, 4H), 8.30 (s, 1H), 8.11 (broad peak, 4H), 7.57 (s, 1H), 7.28 (s, 2H), 2.88 (s, 3H). 13C NMR (101 MHz, DMSOd6) δppm 158.4, 151.1, 147.6, 145.1, 143.5, 140.6, 137.1, 133.3, 126.6, 126.3, 125.1, 124.6, 122.1, 120.7, 118.9, 116.3, 112.8, 112.6, 86.5, 10.6.
2-Amino-4-(4-chlorophenyl)-6-(5-methyl-1-(4-nitrophenyl)-1H-1,2,3-triazol-4-yl)nicotinonitrile (1f). M.p. = 294–297 °C, FTIR (KBr, ν, cm−1): 3490, 3358, 2220, 1636, 1590, 1526, 1346. 1H NMR (400 MHz, DMSOd6) δppm 8.50 (d, J = 8 Hz, 2H), 8.03 (d, J = 8 Hz, 2H), 7.72–7.65 (m, 4H), 7.45 (s, 1H), 7.21 (s, 2H), 2.84 (s, 3H). 13C NMR (101 MHz, DMSOd6) δppm 160.8, 153.5, 153.3, 147.7, 141.7, 140.4, 135.6, 135.3, 134.6, 130.1, 128.9, 126.4, 125.1, 116.7, 108.8, 85.9, 10.5. MS (m/z) = calcd for C21H14ClN7O2: 431.84, found: 432.200.
2-Amino-4-(4-bromophenyl)-6-(5-methyl-1-(4-nitrophenyl)-1H-1,2,3-triazol-4-yl)nicotinonitrile (1g). M.p. > 300 °C, FTIR (KBr, ν, cm−1): 3489, 3358, 2219, 1634, 1590, 1524, 1348. 1H NMR (400 MHz, DMSOd6) δppm 8.50 (d, J = 8 Hz, 2H), 8.04 (d, J = 8 Hz, 2H), 7.80 (d, J = 8 Hz, 2H), 7.64 (d, J = 8 Hz, 2H), 7.45 (s, 1H), 7.21 (s, 2H), 2.84 (s, 3H). 13C NMR (101 MHz, DMSOd6) δppm 160.8, 153.5, 153.4, 147.7, 141.7, 140.4, 1356.0, 135.3, 131.9, 130.3, 126.4, 125.1, 123.3, 116.7, 108.7, 85.8, 10.5. MS (m/z) = calcd for C21H14BrN7O2: 476.29, found: 477.700.
2-Amino-4-(3-bromophenyl)-6-(5-methyl-1-(4-nitrophenyl)-1H-1,2,3-triazol-4-yl)nicotinonitrile (1h). M.p. = 233–235 °C, FTIR (KBr, ν, cm−1): 3455, 3384, 2209, 1609, 1588, 1502, 1344. 1H NMR (400 MHz, DMSOd6) δppm 8.50 (d, J = 8 Hz, 2H), 8.04 (d, J = 8 Hz, 2H), 7.87 (s, 1H), 7.78 (d, J = 8 Hz, 1H), 7.68 (d, J = 8 Hz, 1H), 7.55 (t, J = 8 Hz, 1H), 7.45 (s, 1H), 7.23 (s, 2H), 2.84 (s, 3H). 13C NMR (101 MHz, DMSOd6) δppm 160.8, 153.5, 152.9, 147.7, 141.7, 140.4, 139.1, 135.3, 132.5, 131.0, 130.7, 127.4, 126.4, 125.1, 121.9, 116.6, 108.9, 86.0, 10.5. MS (m/z) = calcd for C21H14BrN7O2: 476.29, found: 477.100.
2-Amino-4-(4-chloro-3-nitrophenyl)-6-(5-methyl-1-(4-nitrophenyl)-1H-1,2,3-triazol-4-yl)nicotinonitrile (1i). M.p. > 300 °C, 1H NMR (400 MHz, DMSOd6) δppm 8.50 (d, J = 12 Hz, 2H), 8.41 (s, 1H), 8.05–8.01 (m, 4H), 7.53 (s, 1H), 7.31 (s, 2H), 2.84 (s, 3H). 13C NMR (101 MHz, DMSOd6) δppm 160.7, 153.8, 151.2, 147.8, 147.7, 141.6, 140.3, 136.9, 135.5, 133.5, 132.1, 126.4, 126.1, 126.0, 125.4, 125.1, 116.4, 108.8, 85.8, 40.0, 39.9, 39.6, 39.4, 39.2, 39.0, 38.8, 10.5. MS (m/z) = calcd for C21H13ClN8O4: 476.84, found: 477.400.
2-Amino-4-(4-florophenyl)-6-(5-methyl-1-(4-nitrophenyl)-1H-1,2,3-triazol-4-yl)nicotinonitrile (1j). M.p. = 251–255 °C, FTIR (KBr, ν, cm−1): 3496, 3377, 2213, 1632, 1588, 1561, 1347. 1H NMR (400 MHz, DMSOd6) δppm 8.50 (d, J = 8 Hz, 2H), 8.04 (d, J = 8 Hz, 2H), 7.76–7.73 (m, 2H), 7.45–7.41 (m, 3H), 7.19 (s, 2H), 2.84 (s, 3H). 13C NMR (101 MHz, DMSOd6) δppm 160.8, 153.6, 153.4, 147.7, 141.8, 140.4, 138.0, 135.3, 130.6, 130.5, 126.4, 125.1, 117.9, 116.0, 115.8, 108.9, 95.3, 10.4. MS (m/z) = calcd for C21H14FN7O2: 415.39, found: 415.900.
2-Amino-4-(2-florophenyl)-6-(5-methyl-1-(4-nitrophenyl)-1H-1,2,3-triazol-4-yl)nicotinonitrile (1k). M.p. = 206–209 °C, FTIR (KBr, ν, cm−1): 3494, 3358, 2222, 1628, 1584, 1520, 1257. 1H NMR (400 MHz, DMSOd6) δppm 8.50 (d, J = 8 Hz, 2H), 8.04 (d, J = 8 Hz, 2H), 7.65–7.58 (m, 2H), 7.48–7.40 (m, 3H), 7.21 (s, 2H), 2.84 (s, 3H). 13C NMR (101 MHz, DMSOd6) δppm 160.3, 159.7, 157.3, 153.5, 149.3, 147.7, 141.6, 140.3, 135.4, 132.0, 131.9, 130.7, 126.4, 125.1, 124.6, 124.4, 116.2, 116.0, 109.7, 87.6, 10.4. MS (m/z) = calcd for C21H14FN7O2: 415.39, found: 416.031.
2-Amino-6-(5-methyl-1-(4-nitrophenyl)-1H-1,2,3-triazol-4-yl)-4-(4-nitrophenyl)nicotinonitrile (1l). M.p. = 266–268 °C, FTIR (KBr, ν, cm−1): 3482, 3367, 2208, 1613, 1562, 1527, 1347. 1H NMR (400 MHz, DMSOd6) δppm 8.52–8.41 (m, 4H), 8.16–7.90 (m, 4H), 7.54 (s, 1H), 7.30 (s, 2H), 2.85 (s, 3H). 13C NMR (101 MHz, DMSOd6) δppm 160.8, 153.8, 152.3, 147.9, 147.7, 140.3, 138.2, 135.4, 134.9, 130.6, 126.4, 125.1, 123.0, 116.5, 108.9, 85.9, 10.5. MS (m/z) = calcd for C21H14N8O4: 442.40, found: 442.000.
2-Amino-4-(4-cyanophenyl)-6-(5-methyl-1-(4-nitrophenyl)-1H-1,2,3-triazol-4-yl)nicotinonitrile (1m). M.p. > 300 °C, FTIR (KBr, ν, cm−1): 3482, 3368, 2229, 2219, 1636, 1589, 1527, 1503, 1406, 1311. 1H NMR (400 MHz, DMSOd6) δppm 8.53 (broad peak, 2H), 8.08–7.92 (m, 6H), 7.49–7.12 (m, 3H), 2.87 (s, 3H). 13C NMR (101 MHz, DMSOd6) δppm 160.8, 153.9, 152.9, 147.7, 141.3, 140.3, 135.4, 132.8, 129.7, 129.3, 126.4, 126.0, 125.1, 118.4, 112.3, 108.8, 85.8, 10.5.
Conclusion
In conclusion, we were able to construct Fe3O4@SiO2@DES1 using a straightforward methodology. Instrumental characterization confirmed the successful synthesis and determination of the physicochemical properties of the presented catalyst. The proposed catalyst was used for the synthesis of 1,2,3-triazole-nicotinonitrile hybrids. The free hydroxy groups on the surface of Fe3O4@SiO2 have catalytic activity, and the ionic structure of Ch/Cl can act like an ionic liquid catalyst. Moreover, urea segments act as hydrogen bonding catalysts. These moieties activate the starting materials and intermediates through hydrogen bonding interactions and facilitate the pyridine synthesis reaction. Therefore, the synergistic effects of these parts significantly improve the catalytic potential of Fe3O4@SiO2@DES1, and this hybrid and versatile systems can lead to the activation of different starting materials and intermediates during pyridine synthesis. Besides, due to the heterogeneous nature of Fe3O4@SiO2@DES1, it can be easily separated from the reaction mixture. Thus, desired products were obtained under mild conditions and with high yields. In future studies, this approach can be extended to other catalytic transformations and can be inspiring in chemical studies.
Data availability
The data supporting this article have been included as part of the ESI.†
Conflicts of interest
There are no conflicts to declare.
Acknowledgements
We thank the Bu-Ali Sina University for financial support to our research group.
References
- E. L. Smith, A. P. Abbott and K. S. Ryder, Chem. Rev., 2014, 114, 11060 CrossRef CAS PubMed.
- B. B. Hansen, S. Spittle, B. Chen, D. Poe, Y. Zhang, J. M. Klein and J. R. Sangoro, Chem. Rev., 2020, 121, 1232 CrossRef PubMed.
- P. Janicka, M. Kaykhaii, J. Płotka-Wasylka and J. Gębicki, Green Chem., 2022, 24, 5035 RSC.
- B. Nian and X. Li, Int. J. Biol. Macromol., 2022, 217, 255 CrossRef CAS PubMed.
- J. Afonso, A. Mezzetta, I. M. Marrucho and L. Guazzelli, Green Chem., 2023, 25, 59 RSC.
- A. Sanati, M. R. Malayeri, O. Busse and J. J. Weigand, J. Mol. Liq., 2022, 361, 119641 CrossRef CAS.
- L. Lomba, M. P. Ribate, E. Sangüesa, J. Concha, M. P. Garralaga, D. Errazquin, C. B. Garcia and B. Giner, Appl. Sci., 2021, 11, 10061 CrossRef CAS.
- A. E. Ünlü, A. Arıkaya and S. Takaç, Green Process. Synth., 2019, 8, 355 Search PubMed.
- G. García, S. Aparicio, R. Ullah and M. Atilhan, Energy Fuels, 2015, 29, 2616 CrossRef.
- Y. P. Mbous, M. Hayyan, A. Hayyan, W. F. Wong, M. A. Hashim and C. Y. Looi, Biotechnol. Adv., 2017, 35, 105 CrossRef CAS PubMed.
- Y. Nahar and S. C. Thickett, Polymers, 2021, 13, 447 CrossRef CAS PubMed.
- R. Rodríguez-Ramos, Á. Santana-Mayor, B. Socas-Rodríguez and M. Á. Rodríguez-Delgado, Appl. Sci., 2021, 11, 4779 CrossRef.
- A. Monem, D. Habibi and H. Goudarzi, Sci. Rep., 2023, 13, 18009 CrossRef CAS.
- P. Liu, J. W. Hao, L. P. Mo and Z. H. Zhang, RSC Adv., 2015, 5, 4867 Search PubMed.
- J. P. Bittner, N. Zhang, L. Huang, P. D. de María, S. Jakobtorweihen and S. Kara, Green Chem., 2022, 24, 1120 RSC.
- I. M. Pateli, A. P. Abbott, G. R. Jenkin and J. M. Hartley, Green Chem., 2022, 22, 8360 RSC.
- X. Yang, Q. Zou, T. Zhao, P. Chen, Z. Liu, F. Liu and Q. Lin, ACS Sustain. Chem. Eng., 2021, 9, 10437 CrossRef CAS.
- M. N. T. Tran, X. T. T. Nguyen, H. T. Nguyen, D. K. N. Chau and P. H. Tran, Tetrahedron Lett., 2020, 61, 151481 CrossRef CAS.
- W. Jiang, K. Zhu, H. Li, L. Zhu, M. Hua, J. Xiao, C. Wang, Z. Yang, G. Chen, W. Zho, H. Li and S. Dai, Chem. Eng. J., 2020, 394, 124831 CrossRef CAS.
- S. E. Hooshmand, R. Afshari, D. J. Ramón and R. S. Varma, Green Chem., 2020, 22, 3668 RSC.
- A. Triolo, M. E. Di Pietro, A. Mele, F. Lo Celso, M. Brehm, V. Di Lisio and O. Russina, J. Chem. Phys., 2021, 154, 244501 CrossRef CAS PubMed.
- B. Atashkar, M. A. Zolfigol and S. Mallakpour, Mol. Catal., 2018, 452, 192 CrossRef CAS.
- M. Torabi, M. Yarie and M. A. Zolfigol, Appl. Organomet. Chem., 2019, 33, e4933 CrossRef.
- P. N. Nguyen, L. H. T. Nguyen, T. L. H. Doan, P. H. Tran and H. T. Nguyen, RSC Adv., 2024, 14, 7006 RSC.
- P. Makoś-Chełstowska, M. Kaykhaii, J. Płotka-Wasylka and M. de la Guardia, J. Mol. Liq., 2022, 365, 120158 CrossRef.
- M. Á. Aguirre and A. Canals, Trends Anal. Chem., 2022, 146, 11650 CrossRef.
-
(a) M. Bakhtiarian and M. M. Khodaei, Colloids Surf., A, 2022, 641, 128569 CrossRef CAS;
(b) M. Zhang, Y. H. Liu, Z. R. Shang, H. C. Hu and Z. H. Zhang, Catal. Commun., 2017, 88, 39–44 CrossRef CAS.
-
(a) M. Niakan, M. Masteri-Farahani, H. Shekaari and S. Karimi, Carbohydr. Polym., 2021, 251, 117109 CrossRef CAS PubMed;
(b) M. H. Zainal-Abidin, M. Hayyan, J. Matmin, A. M. Al-Fakih, N. Jamaluddin, W. M. A. W. Mahmood, R. A. Vahab and F. Abdullah, J. Ind. Eng. Chem., 2023, 124, 1–16 CrossRef CAS;
(c) P. Makoś-Chełstowska, M. Kaykhaii, J. Płotka-Wasylka and M. de la Guardia, J. Mol. Liq., 2022, 365, 120158 CrossRef.
- N. Azizi, Z. Rahimi and M. Alipour, RSC Adv., 2015, 5, 61191 RSC.
- P. H. Tran, RSC Adv., 2020, 10, 9663 RSC.
- S. Alavinia and R. Ghorbani-Vaghei, J. Mol. Struct., 2022, 1270, 133860 CrossRef CAS.
- R. Manujyothi, T. Aneeja and G. Anilkumar, RSC Adv., 2021, 11, 19433 RSC.
- N. Zarei, M. A. Zolfigol, M. Torabi and M. Yarie, Sci. Rep., 2023, 13, 9486 CrossRef CAS PubMed.
- B. Saavedra, J. M. Pérez, M. J. Rodríguez-Álvarez, J. García-Álvarez and D. J. Ramón, Green Chem., 2018, 20, 2151 RSC.
- N. Aziizi, Z. Manochehri, A. Nahayi and S. Torkashvand, J. Mol. Liq., 2014, 196, 153 CrossRef CAS.
- M. R. Anizadeh, M. A. Zolfigol, M. Torabi, M. Yarie and B. Notash, J. Mol. Liq., 2022, 364, 120016 CrossRef CAS.
- J. Lu, X. T. Li, E. Q. Ma, L. P. Mo and Z. H. Zhang, ChemCatChem, 2014, 6, 2854 CrossRef CAS.
- J. A. Bull, J. J. Mousseau, G. Pelletier and A. B. Charette, Chem. Rev., 2012, 112, 2642 CrossRef CAS.
- C. Allais, J. M. Grassot, J. Rodriguez and T. Constantieux, Chem. Rev., 2014, 114, 10829 CrossRef CAS.
- P. Patil, S. P. Sethy, T. Sameena and K. Shailaja, Asian J. Res. Chem., 2013, 6, 888 Search PubMed.
- C. Wei, Y. He, X. Shi and Z. Song, Coord. Chem. Rev., 2019, 385, 1 CrossRef CAS PubMed.
- M. Torabi, M. A. Zolfigol and M. Yarie, Arabian J. Chem., 2023, 16, 105090 CrossRef CAS.
- G. de Ruiter, M. Lahav and M. E. van der Boom, Acc. Chem. Res., 2014, 47, 3407 CrossRef CAS.
- G. Mohammad Abu-Taweel, M. M. Ibrahim, S. Khan, H. M. Al-Saidi, M. Alshamrani, F. A. Alhumaydhi and S. S. Alharthi, Crit. Rev. Anal. Chem., 2022, 54, 599 CrossRef PubMed.
- R. El-Sayed, J. Oleo Sci., 2015, 64, 761 CrossRef CAS.
- G. Tseberlidis, D. Intrieri and A. Caselli, Eur. J. Inorg. Chem., 2017, 2017, 3589 CrossRef CAS.
- Y. Wang, Y. Wu, H. Cong, S. Wang, Y. Shen and B. Yu, J. Polym. Environ., 2022, 30, 385 CrossRef CAS.
- M. Torabi, M. Yarie, S. Baghery and M. A. Zolfigol, Recent advances in catalytic synthesis of pyridine derivatives, Elsevier Inc., 2023, p. 503 Search PubMed.
- Y. Ling, Z. Y. Hao, D. Liang, C. L. Zhang, Y. F. Liu and Y. Wang, Drug Des., Dev. Ther., 2021, 15, 4289 CrossRef CAS.
- K. A. Kasabov, N. V. Kudryashov, A. V. Volkova, A. A. Shimshirt, T. S. Kalinina, L. A. Zhmurenko and T. A. Voronina, Bull. Exp. Biol. Med., 2020, 168, 449 CrossRef CAS PubMed.
- V. K. Sharma, J. M. Hutchison and A. M. Allgeier, ChemSusChem, 2022, 15, e202200888 CrossRef CAS PubMed.
- R. Zafar, H. Naureen, M. Zubair, K. Shahid, M. Saeed Jan, S. Akhtar and A. Sadiq, Drug Des., Dev. Ther., 2021, 15, 2679 CrossRef.
- A. E. Mekky and S. M. Sanad, Synth. Commun., 2022, 52, 2276 CrossRef CAS.
- R. M. Keshk, G. E. A. Elgawad, E. R. Sallam, M. S. Alsubaie and H. A. Fetouh, ChemistrySelect, 2022, 7, e202202678 CrossRef CAS.
- S. M. Sanad and A. E. Mekky, Synth. Commun., 2020, 50, 1468 CrossRef CAS.
- A. A. El-Sayed, E. A. Elsayed and A. E. G. E. Amr, ACS Omega, 2021, 6, 7147 CrossRef CAS PubMed.
- S. Jalali-Mola, M. Torabi, M. Yarie and M. A. Zolfigol, RSC Adv., 2022, 12, 34730 RSC.
- M. B. Islam, M. I. Islam, N. Nath, T. B. Emran, M. R. Rahman, R. Sharma and M. M. Matin, BioMed Res. Int., 2023, 2023, 9967591 CrossRef.
- J. R. Johansson, T. Beke-Somfai, A. Said Stålsmeden and N. Kann, Chem. Rev., 2016, 116, 14726 CrossRef CAS PubMed.
- Z. Xu, S. J. Zhao and Y. Liu, Eur. J. Med. Chem., 2019, 183, 111700 CrossRef CAS PubMed.
- J. P. Wan, S. Cao and Y. Liu, J. Org. Chem., 2015, 80, 9028 CrossRef CAS.
- M. Torabi, M. Yarie, M. A. Zolfigol, S. Azizian and Y. Gu, RSC Adv., 2022, 12, 8804 RSC.
- F. Gao, T. Wang, J. Xiao and G. Huang, Eur. J. Med. Chem., 2019, 173, 274 CrossRef CAS PubMed.
- R. Kharb, M. Shahar Yar and P. Chander Sharma, Mini-Rev. Med. Chem., 2011, 11, 84 CrossRef CAS PubMed.
- K. Haider, M. Shafeeque, S. Yahya and M. S. Yar, Eur. J. Med. Chem. Rep., 2022, 5, 100042 CAS.
- A. Tavassoli, M. Yarie, M. Torabi and M. A. Zolfigol, J. Phys. Chem. Solids, 2024, 186, 111786 CrossRef CAS.
-
(a) F. Karimi, M. Torabi, M. Yarie, M. A. Zolfigol and Y. Gu, J. Iran. Chem. Soc., 2023, 20, 2189 CrossRef CAS;
(b) N. Zarei, M. Torabi, M. Yarie and M. A. Zolfigol, Polycyclic Aromat. Compd., 2023, 43, 3072 CrossRef CAS;
(c) S. Kalhor, M. Yarie, M. Torabi, M. A. Zolfigol, M. Rezaeivala and Y. Gu, Polycyclic Aromat. Compd., 2022, 42, 4270 CrossRef CAS;
(d) M. Torabi, M. A. Zolfigol, M. Yarie and Y. Gu, Mol. Catal., 2021, 516, 111959 CrossRef CAS;
(e) M. Torabi, M. Yarie, M. A. Zolfigol, S. Rouhani, S. Azizi, T. O. Olomola, M. Maaza and T. A. M. Msagati, RSC Adv., 2021, 11, 3143 RSC;
(f) H. Sepehrmansourie, M. Mohammadi Rasooll, M. Zarei, M. A. Zolfigol and Y. Gu, Inorg. Chem., 2023, 62, 9217 CrossRef CAS.
-
(a) I. V. Alabugin, L. Kuhn, M. G. Medvedev, N. V. Krivoshchapov, V. A. Vil, I. A. Yaremenko, P. Mehaffy, M. Yarie, A. O. Terent’ev and M. A. Zolfigol, Chem. Soc. Rev., 2021, 50, 10253 RSC;
(b) I. V. Alabugin, L. Kuhn, N. V. Krivoshchapov, P. Mehaffy and M. G. Medvedev, Chem. Soc. Rev., 2021, 50, 10212 RSC.
- Y. Bide, M. A. Fashapoyeh and S. Shokrollahzadeh, Sci. Rep., 2021, 11, 17068 CrossRef CAS.
-
(a) M. Yarie, Iran. J. Catal., 2017, 7, 85–88 CAS;
(b) M. Yarie, Iran. J. Catal., 2020, 10, 79 CAS.
- M. A. Zolfigol, S. Azizian, M. Torabi, M. Yarie and B. Notash, J. Chem. Educ., 2024, 101, 877 CrossRef CAS.
- M. Torabi, M. A. Zolfigol, M. Yarie, B. Notash, S. Azizian and M. M. Azandaryani, Sci. Rep., 2021, 11, 16846 CrossRef CAS.
- M. R. Anizadeh, M. Torabi, M. A. Zolfigol and M. Yarie, J. Mol. Struct., 2023, 1277, 134885 CrossRef CAS.
|
This journal is © The Royal Society of Chemistry 2024 |
Click here to see how this site uses Cookies. View our privacy policy here.