DOI:
10.1039/D4RA05559D
(Paper)
RSC Adv., 2024,
14, 35697-35703
Synthesis of supramolecular polymers with calix[4]arene and β-cyclodextrin and their application in heavy metal ion absorption†
Received
31st July 2024
, Accepted 28th October 2024
First published on 8th November 2024
Abstract
Two categories of supramolecular polymer monomers were produced by introducing the ureidopyrimidone quadruple-hydrogen bonding assemblies on the calix[4]arene and the β-cyclodextrin host units. The adsorption capacity of these supramolecular polymers for different metal ions was investigated by static adsorption. The results showed that at pH = 6 and when the adsorption equilibrium was reached, the supramolecular polymer with calixarene and β-cyclodextrin as the main body adsorbed up to 99% of Pb2+ and Cd2+, respectively. Also, the supramolecular polymer connected with six carbon chains on β-cyclodextrin had better recognition of Cd2+ and Pb2+, and the highest adsorption rate reached 99%. Industrial adsorbent materials from such supramolecular polymers will provide more options for water pollution control, especially for heavy metal ions.
1. Introduction
Macrocyclic molecules, including calixarene,1–4 cyclodextrin,5–8 crown ether,9–12 pillar arene,13–16 cyclic peptides,17–20 etc. have been used in various fields, such as host/guest recognition,21–25 selective adsorption separation,26–30 water pollution control,31–34 optical sensing detection35–39 and heterogeneous catalysis,40–43 owing to their guest binding properties. Non-covalent bonds bind together the supramolecular polymers to form novel self-assembled structures.44–48 Among the non-covalent bonds are hydrogen, ligand, host-guest, and donor–acceptor interactions. They have many repeating unit structures compared to the conventional high molecular polymers. Therefore, if a supramolecular polymer is made into an adsorbent material, the polymeric material will be characterized by efficient and rapid adsorption of heavy metal ions.
Hydrogen bonding is one of the weak interaction forces in supramolecular chemistry and molecular assembly. Its formation is directional and selective, mainly when using multiple hydrogen bonds. We can obtain very stable structural systems, which will increase the degree of polymerization of supramolecular polymers. For example, Meijer's group49–53 discovered a quadruple hydrogen bonding system with a very high binding constant in 1997. After being introduced into the supramolecular system, the degree of polymerization of supramolecular polymers significantly increased.
Calixarene54–58 and cyclodextrin,59–63 as the host units of supramolecular polymers, have the advantages of high efficiency, high speed, and high selectivity in the adsorption of heavy metal ions, especially the adsorption of highly toxic heavy metal ions. However, traditional polymers can only efficiently adsorb a single specific heavy metal ion, which cannot be carried out according to the characteristics of heavy metals in water. The incorporation of urea-pyrimidinone (UPy) containing quadruple hydrogen bonds into the supramolecular structure can introduce the property of quadruple hydrogen bonding into the supramolecular polymer, and the prepared supramolecular polymer can be self-repairing. From the reaction synthesis process perspective, it is highly feasible to introduce UPy's quadruple hydrogen bonds into the calixarenes and cyclodextrin structures. Therefore, two types of supramolecular monomers have been designed in this paper. Firstly, urea-pyrimidinone linked by three carbon chain on p-tert-butylcalix[4]arene (BC4PUPy); urea-pyrimidinone linked by six carbon chains on p-tert-butylcalix[4]arene (BC4HUPy); urea-pyrimidinone linked by three carbon chains on calix[4]arenes (C4PUPy); urea-pyrimidinone linked by six carbon chains on calix[4]arenes (C4HUPy). Secondly, urea-pyrimidinone was with three carbon chains on β-cyclodextrin (β-CDPUPy); urea-pyrimidinone was with four carbon chains on β-cyclodextrin (β-CDBUPy); urea-pyrimidinone linked with six carbon chains on β-cyclodextrin (β-CDHUPy). The synthetic supramolecular polymer names and related parameters are shown in Table 1 as below.
Table 1 The related parameters of title supramolecular polymers
Target materials |
Host unit |
Alkyl chain |
BC4PUPy |
tert-Butylcalix[4]arene |
Propyl |
BC4HUPy |
tert-Butylcalix[4]arene |
Hexyl |
C4PUPy |
Calix[4]arene |
Propanyl |
C4HUPy |
Calix[4]arene |
Hexanyl |
β-CDPUPy |
β-Cyclodextrin |
Methyl |
β-CDBUPy |
β-Cyclodextrin |
Ethyl |
β-CDHUPy |
β-Cyclodextrin |
Butyl |
As shown in Fig. 1, two types of supramolecular monomer polymers have unique adsorption capabilities and can be used to absorb heavy metal ions.
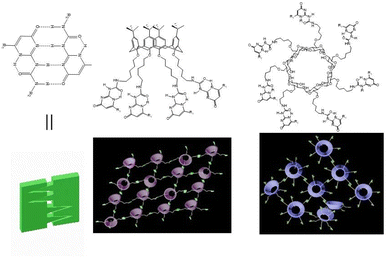 |
| Fig. 1 The chemical structure of the formation of the quadruple-hydrogen bonding assemblies in UPy and the supramolecular polymers dominated by β-cyclodextrin and calix[4]arene. | |
2. Results and discussion
2.1 Design and synthesis
Through the study of related literature, we have found that the derivatives with cyclodextrin and calixarene as host structures are similar to their unmodified host structures in the inclusion interaction of guest ions or molecules. So, the properties of urea-pyrimidinone linked by polycarbonate chain on calix[4]arenes are determined by the properties of calix[4]arenes. The properties of the β-cyclodextrin determine the properties of urea-pyrimidinone linked by polycarbon-chain on β-cyclodextrin. Therefore, by changing their substituents, cyclodextrin, and calixarene derivatives can regulate their selectivity to guest ions or molecules. The structure of cyclodextrin and calixarene derivatives can be regulated by changing the type of cyclodextrin and calixarene, the size of the central hole, and the type, position, and number of substituents among the supramolecular polymers assembled by hydrogen bonds. Two advantages can be obtained by introducing urea-pyrimidinone (UPy) into the supramolecular host. First, the supramolecular polymer with cyclodextrin and calixarene as the host units can be adjusted by the solvent method; second, by changing the position of UPy, the amount and the type of substituents are used to regulate the solubility of supramolecular polymers, which makes the supramolecular polymers have more abundant technological properties. At the same time, they also enhance the selectivity of different heavy metal ions and the adsorption effect of heavy metal ions.
2.2 Determination of heavy metal ion adsorption properties
The 20 mg of the newly synthesized supramolecular polymer was added to the conical flask containing 25 mL of metal ion water solution, shaking in a water bath and shaker at a rate of 150 rpm. The temperature was 20 °C. At the end of time, the conical flask was removed. A centrifuge separated the solution at 10
000 rpm. An atomic absorption spectrophotometer then detected the supernatant. The concentration of heavy metal ions in the sample was measured. The PhSJ-3F pH meter produced by Shanghai Precision Scientific Instrument Co., Ltd is used for pH measurement. The concentration of heavy metal ions is measured using the Shimadzu AA6300 atomic absorption spectrophotometer. SHZ-03 high precision air water bath constant temperature shaker produced by Shanghai Kangxin Instrument Equipment Co., Ltd is used for shock treatment of supramolecular polymer absorption of heavy metal ions. Hunan Xingke Scientific Instrument Co., Ltd produced a TGL-16G table high-speed centrifuge for solid–liquid separation after the adsorption of clear liquid. Ultra-pure water is prepared by MO6-226 mole elemental ultra-pure water machine produced by Moler Biotechnology Co., Ltd The adsorption effect of the adsorbent on the heavy metal ion was obtained based on the calculation. The formula is as follows:64
E represents the adsorption percentage of heavy metal ions by the adsorbent. C0 represents the concentration of heavy metal ions in the solution, mg L−1. C denotes the concentration of heavy metals in the solution after adsorption treatment, mg L−1.
The main content of this paper is to investigate the inclusion adsorption of five heavy metal ions (Pb2+, Cd2+, Ni2+, Zn2+, Cu2+) by supramolecular polymers after self-assembly into supramolecular polymers. The selection of (Pb2+, Cd2+, Ni2+, Zn2+, and Cu2+) as the targets of our study was driven by their environmental significance and toxicity, with their ionic radii informing our understanding of their unique interactions and behaviors at the molecular level and their ionic radii as shown in Table 2.
Table 2 The ionic radii of chosen heavy metal ions
Ion |
Pb2+ |
Cd2+ |
Ni2+ |
Zn2+ |
Cu2+ |
Radii (pm) |
119 |
95 |
70 |
74 |
73 |
The adsorption equilibrium time of heavy metal ions by supramolecular polymers was studied at pH = 6. The results showed that the adsorption of Zn2+ by the supramolecular polymer formed by the self-assembly of β-CDBUPy reached equilibrium after 6 h. The adsorption of Ni2+ by supramolecular polymers formed by β-CDHUPy self-assembly reached equilibrium after 6 h. Other supramolecular polymers reached equilibrium in 2 h. Before reaching the adsorption equilibrium, the adsorption capacity of various supramolecular polymers for heavy metal ions increased with the adsorption time. When the adsorption equilibrium is reached, the adsorption rate remains almost unchanged.
At the same adsorption time (6 h), the adsorption effects of the supramolecular polymer on heavy metal ion solution were investigated at pH = 2, 4, and 6, respectively. The results showed that the adsorption capacity of heavy metal ions enhanced with the increase of pH from 2 to 6 (as shown in ESI Section II†). The highest adsorption rate and the best adsorption effect were observed at pH = 6.
The supramolecular polymers achieved an impressive maximum adsorption rate of 99% for Pb2+ and Cd2+, underscoring their remarkable selectivity. Notably, BC4HUPy, BC4HUPy, C4PUPy, C4HUPy were the standout performers in Pb2+ adsorption, while β-CDPUPy and β-CDBUPy demonstrated strong Cd2+ adsorption. β-CDHUPy exhibited good adsorption capacity for both ions. The highest adsorption rate is shown in Table 3.
Table 3 The highest adsorption rate of heavy metal ions
Polymer |
Pb2+ |
Cd2+ |
Ni2+ |
Zn2+ |
Cu2+ |
BC4PUPy |
99% |
4% |
19% |
21% |
5% |
BC4HUPy |
99% |
3% |
20% |
23% |
8% |
C4PUPy |
99% |
3% |
13% |
17% |
6% |
C4HUPy |
99% |
5% |
35% |
19% |
13% |
(-CDPUPy) |
14% |
99% |
2% |
7% |
4% |
(-CDBUPy) |
13% |
99% |
6% |
17% |
10% |
(-CDHUPy) |
99% |
99% |
25% |
22% |
20% |
The maximum adsorption rates of different metal ions by supramolecular polymers are shown in Fig. 2.
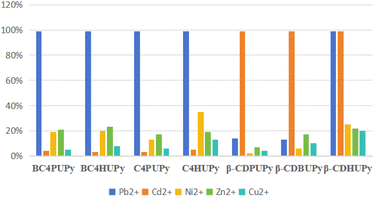 |
| Fig. 2 Maximum adsorption rate of 5 metal ions by title polymers. | |
By studying the pH value and adsorption time of heavy Metal solution, it can be found that the adsorption rate of calixarene-based supramolecular polymeric monomers on Pb2+ are 99% after their respective self-assembly and the adsorption of Cd2+, Ni2+, Zn2+ and Cu2+ is not apparent. This result may be related to the structure of supramolecular polymers. The cavity's size after the supramolecular polymer's formation is compatible with Pb2+, whose ionic radius is 0.119 nm, so it has a high rate of inclusion junction adsorption for lead ions. In contrast, the ionic radius of Cd2+, Ni2+, Zn2+, and Cu2+ is lower than the requirement of cavity adsorption, and desorption is evident. Therefore, the inclusion junction adsorption of Cd2+, Ni2+, Zn2+, and Cu2+ is not good.
After the self-assembly of supramolecular polymerized monomers, the adsorption rates of β-cyclodextrin-based supramolecular polymer adsorbs 99% of Cd2+ in all cases, and the adsorption rates on Zn2+, Ni2+, and Cu2+ are insignificant. After the formation of the supramolecular polymer, the size of the cavity is compatible with Cd2+ with a radius of 0.095 nm, so the adsorption rate of Cd2+ is higher, and the cavity of the supramolecular polymer is larger than the size of Ni2+, Zn2+ and Cu2+. The adsorption is poor as it is shed after adsorption. Conversely, the adsorption rate of supermolecular polymers formed by the self-assembly of β-CDPUPy and β-CDBUPy on Pb2+ is 15% or less. In contrast, the adsorption rate of supramolecular polymers formed by β-CDHUPy on Pb2+ is 99%.
We speculate that the possible mechanism is due to after the self-assembly of polymers with β-CDPUPy and β-CDBUPy, the cavity is enlarged, but it is still smaller than the size of Pb2+, so it is impossible to adsorb Pb2+. However, when β-CDHUPy forms a polymer, the cavity becomes more extensive and adapts to the size of Pb2+; at the same time, the polymer forms a simple network structure, so the inclusion effect is obviously enhanced, and Pb2+ can be adsorbed well. Under encapsulation, Pb2+ cannot easily fall off, so it has a superior adsorption effect on Pb2+ (Scheme 1).
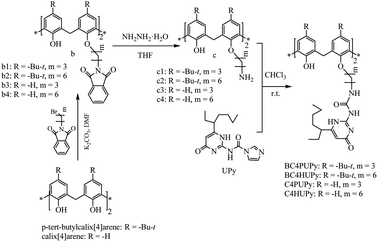 |
| Scheme 1 Synthetic routes of the polymer monomers with calixarenes as the host unit. | |
3. Experimental section
3.1 Apparatus and reagents
Commercially available reagents were used without further purification unless otherwise stated. The 1H and 13C nuclear magnetic resonance (NMR) spectra were recorded using a Bruker spectrometer at 500 MHz using TMS as an internal standard. The β-keto acid ester, urea pyrimidine ketone derivatives, and UPy were prepared by literature procedures.52
3.2 General synthesis of the polymer monomers with calixarenes as the host unit
3.2.1 Synthesis of intermediate b. With the protection of nitrogen, 1.0 mol calix[4]arene derivatives, 4.0 mmol N-bromoalkylphthalimide, 0.55 g K2CO3 (4.0 mmol) (baked in an oven at 130 °C for 6 h), and 20 mL anhydrous DMF are added to a 100 mL single-mouth flask, and the reaction is warmed to 65 °C for 2 days. The reaction is stopped when the reaction time is reached and cools to room temperature. The reaction solution is added to 100 mL of water, and a white turbid substance is immediately formed, extracted with 30 mL of chloroform three times. The organic phase is further treated with 30 mL of 5% NaOH solution and water (20 mL × 3). The solution is washed with saturated brine and dried with anhydrous magnesium sulfate. The solvent is evaporated to give intermediate b1 to b4.b1: (0.54 g, 54%), 1H NMR (CDCl3, ppm): δ = 7.76 (m, Ar–H, 4H), 7.62 (m, Ar–H, 4H), 7.07 (s, Ar–H, 8H), 6.82 (s, Ar–H, 8H), 4.35 (m, O–CH2–C, 4H), 4.15 (m, –CH2, 8H), 3.35 (m, N–CH2–C, 4H), 2.47 (m, –CH2, 4H), 1.30 (s, –C(CH3)3, 36H).
b2: (0.49 g, 45%).1H NMR (500 MHz, CDCl3): δ = 7.70 (m, Ar–H, 4H), 7.41 (m, Ar–H, 4H), 7.05 (s, Ar–H, H), 6.79 (s, Ar–H, 8H), 3.99–3.96 (m, O–CH2–C, 4H), 3.73–3.65 (m, N–CH2–C, 4H), 3.31 (dd, –CH2, 8H), 2.05–1.65 (m, O–C–CH2–C, 4H), 1.55–1.44 (m, N–C–CH2–C, 4H), 1.33 (s, –C (CH3)3, 36H), 0.88 (m, –CH2, 8H).
b3: (0.49 g, 51%), 1H NMR (500 MHz, CDCl3):δ = 7.80 (m, 4H), 7.70 (m, 4H), 7.22–6.26 (m, 12H), 4.02 (m, 4H), 3.9–3.4 (m, 8H), 3.04 (d, 4H), 2.22 (m, 4H).
b4: (0.49 g, 56%), 1H NMR (500 MHz, CDCl3): δ = 7.80 (m, 4H), 7.67 (m, 4H), 7.16–6.18 (m, 12H), 4.09 (dd, 8H), 3.74–3.49 (m, 8H), 1.56–0.89 (m, 16H).
3.2.2 Synthesis of intermediate c. 0.5 mmol b and THF (20 mL) are added to a 50 mL three-necked flask under a nitrogen atmosphere. The mixture is heated to reflux, and then 10 mL of hydrazine hydrate is added dropwise using a dropping funnel. The reaction mixture is refluxed for 8 h. Stop heating and cool to room temperature. Separate the lower layer with a separatory funnel and take the upper layer of liquid. Evaporate the solvent with a rotary evaporator and obtain a brownish oily body extracted with 60 mL of chloroform. The chloroform phase is washed with 40% sodium hydroxide solution and 50 mL of saturated brine and dried with anhydrous Na2SO4. The solvent is evaporated to give intermediate c1 to c4.c1: (0.28 g, 73.5%), 1H NMR (500 MHz, CDCl3): δ = 7.08 (s, 8H), 6.82 (s, 8H), 4.15 (m, 8H), 3.31 (d, 8H), 2.47 (m, 4H), 1.93 (m, 4H), 1.33 (s, 36H).
c2: (0.34 g, 80%), 1H NMR (500 MHz, CDCl3):δ = 7.28 (s, 8H), 6.91 (s, 8H), 4.0 (t, 4H), 3.41 (t, 4H), 3.31 (d, 8H), 1.91 (m, 4H), 1.45 (m, 16H), 1.29 (s, 36H).
c3: (0.11 g, 41.5%), 1H NMR (500 MHz, CDCl3): δ = 7.80 (m, 4H), 7.70 (m, 4H), 7.22–6.26 (m, 12H), 4.02 (m, 4H), 3.9–3.4 (m, 4H), 3.04 (d, 8H), 2.22 (m, 4H).
c4: (0.095 g, 31%), 1H NMR (500 MHz, CDCl3): δ = 11.45 (s, 2H), 9.71 (s, 2H), 8.55 (s, 2H), 7.23–7.06 (m, 8H), 5.73 (s, 2H), 4.20–4.14 (m, 8H), 3.66–3.43 (m, 8H), 3.32 (m, 4H), 2.21 (s, 2H), 2.08 (m, 8H), 1.5 (m, 8H), 0.88–0.72 (m, 12H) ppm.
3.2.3 Synthesis of the polymer monomers with calixarenes as the host unit. Under nitrogen protection, 0.5 mmol intermediate c and UPy (0.6 g, 2 mmol) is added to a 50 mL three-necked flask, and 20 mL of CHCl3 (already dehydrated and dried) is added at 25 °C. Stir and avoid light for 12 h. The reaction is stopped when the reaction time is reached and cools to room temperature. CHCl3 treated (50 mL) is added to the reaction solution, and the reaction solution is washed sequentially with 2 N HCl (20 mL), saturated NaHCO3 (30 mL), and saturated brine (30 mL). The organic phase is dried with anhydrous Na2SO4, and the solvent is evaporated. The crude product is separated by column chromatography and then precipitated in methanol to obtain the target materials as a white solid (Scheme 2).
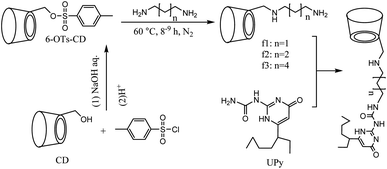 |
| Scheme 2 Synthetic routes of the polymer monomers with β-cyclodextrin as the host unit. | |
BC4PUPy: (0.21 g, 35%), the rate of UPy substitution is 2.3 estimated from the 1H NMR data. 1H NMR (500 MHz, CDCl3): δ = 13.54 (s, 2H), 11.77 (s, 2H), 10.18 (s, 2H), 7.99 (s, 2H), 6.99 (s, 4H), 6.83 (s, 4H), 5.47 (s, 2H), 4.21 (d, 8H), 3.9 (m, 8H), 3.2 (m, 4H), 2.23 (s, 2H), 2.20 (m, 8H), 1.6 (m, 8H), 1.21 (s, 36H), 0.90–0.70 (m, 12H) ppm; 13C NMR (125 MHz, CDCl3): δ = 205.79, 171.77, 155.46, 154.64, 154.11, 150.40, 148.76, 145.91, 139.97, 131.88, 126.19, 124.56, 123.99, 104.25, 73.87, 44.10, 38.38, 33.00, 32.78, 31.38, 30.94, 30.05, 29.86, 29.33, 28.21, 25.09, 21.65, 12.96, 10.67.
BC4HUPy: (0.21 g, 33%),the rate of UPy substitution is 2.5 estimated from the 1H NMR data. 1H NMR (500 MHz, CDCl3): δ = 13.18 (s, 2H), 11.83 (s, 2H), 10.16 (s, 2H), 7.62 (s, 2H), 6.96 (s, 4H), 6.75 (s, 4H), 5.73 (s, 2H), 4.20 (d, 8H), 3.89 (m, 8H), 2.2–2.0 (m, 16H), 1.59–1.44 (m, 16H), 1.20 (s, 36H), 0.89–0.70 (m, 12H) ppm; 13C NMR (125 MHz, CDCl3): δ = 205.77, 172.08, 171.96, 155.73, 154.15, 153.94, 149.83, 149.78, 149.14, 145.63, 140.19, 131.80, 126.83, 124.50, 124.43, 124.01, 105.23, 105.04, 44.32, 44.19, 39.14, 39.07, 32.92, 32.78, 31.87, 31.68, 30.86, 30.69, 30.05, 29.83, 29.33, 28.99, 28.29, 26.00, 25.59, 25.40, 24.76, 24.57, 21.76, 21.53, 21.47, 12.87, 10.68.
C4PUPy: (0.37 g, 75%),the rate of UPy substitution is 2.2 estimated from the 1H NMR data. 1H NMR (500 MHz, CDCl3): δ = 11.45 (s, 2H), 9.71 (s, 2H), 8.55 (s, 2H), 7.23–7.06 (m, 8H), 5.73 (s, 2H), 4.20–4.14 (m, 8H), 3.66–3.43 (m, 8H), 3.32 (m, 4H), 2.21 (s, 2H), 2.08 (m, 8H), 1.5 (m, 8H), 0.88–0.72 (m, 12H) ppm; 13C NMR (125 MHz, CDCl3): δ = 206.93, 172.91, 156.63, 155.81, 155.25, 151.61, 149.89, 147.08, 141.11, 133.02, 127.57, 125.71, 125.13, 105.39, 75.05, 45.24, 39.52, 34.15, 33.92, 31.86, 29.36, 26.24, 22.80, 14.11, 11.82.
C4HUPy: (0.32 g, 60%), the rate of UPy substitution is 2.3 estimated from the 1H NMR data. 1H NMR (500 MHz, CDCl3): δ = 11.45 (s, 2H), 9.72 (s, 2H), 8.39 (s, 2H), 7.23–7.05 (m, 8H), 5.73 (s, 2H), 4.21–4.0 (m, 8H), 3.66–3.43 (m, 8H), 3.33 (m, 4H), 2.22–2.08 (m, 10H), 1.20–1.17 (m, 8H), 0.81–0.72 (m, 12H) ppm. 13C NMR (125 MHz, CDCl3): δ = 206.90, 173.22, 173.09, 156.85, 155.06, 150.96, 150.91, 150.27, 146.76, 141.32, 132.93, 127.95, 125.63, 125.56, 125.14, 106.17, 76.77, 76.61, 45.44, 45.32, 40.27, 40.20, 34.05, 33.90, 31.99, 31.82, 30.46, 30.11, 29.42, 27.13, 26.72, 26.53, 25.89, 25.70, 22.66, 22.60, 14.00, 11.81.
3.3 General synthesis of the polymer monomers with β-cyclodextrin as the host unit
3.3.1 Synthesis of intermediate 6-OTs-CD. Add 10 g of β-cyclodextrin (8.8 mmol) to a 500 mL single-necked flask with 100 mL of double distilled water and stir magnetically at room temperature for 2 h. The solution is emulsified by adding 20 mL of 50% NaOH solution dropwise with a dropping funnel and stirring for 30 min. Add 20 mL of 50% NaOH solution drop by drop with a dropping funnel, and continue stirring for 30 min. 1.5 g of p-toluenesulfonyl chloride (TsCl) is weighed and dissolved in 30 mL of acetonitrile and then ultrasonicated in an ultrasonic apparatus to dissolve p-toluenesulfonyl chloride completely. The solution is added to the reaction dropwise with a constant pressure burette funnel and stirred at 10 °C for three h. The unreacted p-toluenesulfonyl chloride is filtered out by filtration. The filtrate is adjusted to a pH value of about 7 with 2 mol L−1 hydrochloric acid and stored at a low temperature below 5 °C for 12 h. A large amount of white precipitate is produced, and a white solid is obtained by filtration under reduced pressure. The product is recrystallized twice with 100 mL of double distilled water and dried in a vacuum oven at 60 °C for 12 h. The final product 6-OTs-CD is obtained with a yield of 20.0%. 1H NMR (500 MHz, DMSO): δ = 8.75 (d, 2H, Ar–H), 7.75 (d, 2H, Ar–H), 5.75 (s, 14H, 2- and 3-OH), 4.70 (s, 7H, H-1 of CD), 4.5–4.1 (m, 1H, 6-OH), 3.75–3.25 (m, 42H, H-2, 3, 4, 5, 6 of CD), 2.40 (s, 3H, –CH3).
3.3.2 Synthesis of intermediate f. Under nitrogen protection, 2.0–2.5 g of 6-OTs-β-CD and 15 mL of 1,3-alkyl diamine is added in a 100 mL single-mouth flask. The reaction is warmed to 70 °C, and reacts for 8 h. The reaction is stopped when the reaction time is reached and cools to room temperature. A vacuum distillation apparatus is set up, and excess unreacted 1,3-propane diamine is distilled off to obtain a yellow impurity. Add a small amount of hot water to dissolve the yellow debris. A large amount of anhydrous ethanol is added to produce a sizeable white precipitate. Stir for 20 min and let stand. A white solid can be obtained by filtration, the target crude product. Purification method: Sephadex C-25 ion column was used for impurity removal by eluting with 0.05, 0.15, and 0.3 mol L−1 sodium chloride solution. The sample is collected and purified. The purified sample is washed with a Sephadex G-25 gel column to offer f1–f3.f1: yield 70%, 1H NMR (500 MHz, DMSO-d6): δ = 4.80–4.75 (m, 7H, H-1 of CD), 3.70–3.50 (m, 28H, H-3,5,6 of CD), 3.45–3.40 (m, 14H, H-2,4 of CD), 3.40–3.30 (t, 1H, H-6 of CD), 2.90–2.80 (t, 2H, C–CH2–N), 2.70–2.55 (t, 2H, C–CH2–N), 1.55–1.45 (m, 2H, C–CH2–C).
f2: yield 55%, 1H NMR (500 MHz, DMSO-d6): δ = 5.03–4.98 (m, 7H, H-1 of CD), 4.02–3.98 (t, 1H, H-6′ of CD), 3.92–3.71 (m, 28H, H-3,5,6 of CD), 3.60–3.49 (m, 14H, H-2, 4 of CD), 3.45–3.39 (t, 1H, H-6′of CD), 2.96–2.93 (t, 2H, C–CH2–N), 2.89–2.84 (t, 2H, C–CH2–N), 1.68–1.61 (m, 4H, C–CH2–C).
f3: yield 65%, 1H NMR (500 MHz, DMSO-d6): δ = 4.85–4.75 (m, 7H, H-1 of CD), 4.60–4.25 (s, 1H, H-6′ of CD), 3.75–3.50 (m, 28H, H-3,5,6 of CD), 3.45–3.20 (m, 14H, H-2,4 of CD), 2.90–2.80 (t, 2H, C–CH2–N), 2.75–2.55 (t, 2H, C–CH2–N), 1.45–1.0 (m, 8H, C–CH2–C).
3.3.3 Synthesis of the polymer monomers with β-cyclodextrin as the host unit. Try to avoid light reactions. Under nitrogen protection, add intermediate f1 (0.6 g, 0.5 mmol), UPy (0.6 g, 2 mmol) and 20 mL DMF into a 50 mL three-necked flask. The reaction is stirred at 35 °C overnight and cooled to room temperature. Add 150 mL of acetone into a 250 mL beaker and stir vigorously. The cooled reaction solution is added dropwise to acetone, and the acetone solution gradually becomes turbid. A white solid can be obtained by filtration. Wash sequentially with 1 N HCl (20 mL), saturated NaHCO3 (20 mL), and saturated brine (20 mL). The target materials can be obtained by vacuum drying as white solids.β-CDPUPy: yield, 0.21 g, 30%, the rate of UPy substitution is 2.2 estimated from the 1H NMR data. 1H NMR (500 MHz, DMSO-d6): δ = 7.95 (s, –NH, 3H), 5.73–5.68 (s, –CH, 1H), 4.83–4.81 (m, 7H, H-1 of CD), 4.46 (s, 1H, H-6′ of CD), 3.65–3.55 (m, 28H, H-3,6,5 of CD), 3.37–3.35 (m, 15H, H-2, 4, 6 of CD), 2.89 (m, –CH, 1H), 2.73 (m, N–CH2–C, 2H), 1.65–1.49 (m, –CH2, 4H), 1.23–1.13 (m, –CH2, 8H), 0.85–0.73 (m, –CH3, 6H). 13C NMR (125 MHz, DMSO-d6): δ = 206.68, 182.21, 168.00, 154.72, 102.09, 93.10, 73.23, 72.66, 72.62, 72.17, 68.28, 60.08, 47.50, 44.84, 43.23, 32.98, 30.81, 29.13, 26.58, 22.22, 13.96, 11.82.
β-CDBUPy: yield 0.18 g, 25%, the rate of UPy substitution is 2.0 estimated from the 1H NMR data. 1H NMR (500 MHz, DMSO-d6): δ = 7.95 (s, –NH, 3H), 5.81 (s, 1H), 4.83–4.80 (m, 7H, H-1 of CD), 4.51 (s, 1H, H-6 of CD), 3.65–3.56 (m, 28H, H-3,6,5 of CD), 3.40–3.29 (m, 15H, H-2, 4, 6 of CD), 2.88 (m, –CH, 1H), 2.72 (m, N–CH2–C, 2H), 1.51–1.48 (m, –CH2, 6H), 1.21–1.23 (m, –CH2, 8H), 0.85–0.73 (m, –CH3, 6H). 13C NMR (125 MHz, DMSO-d6): δ = 206.29, 171.75, 157.73, 154.55, 102.09, 96.25, 81.53, 73.06, 72.95, 72.55, 72.45, 72.00, 70.02, 68.13, 62.76, 59.91, 55.04, 49.45, 47.34, 47.31, 30.64, 28.96, 28.68, 26.41, 22.06, 21.97, 13.79, 11.65.
β-CDHUPy: yield 0.22 g, 30%, the rate of UPy substitution is 2.1 estimated from the 1H NMR data. 1H NMR (500 MHz, DMSO-d6):δ = 7.91 (s, –NH, 3H), 5.79 (s, –CH, 1H), 4.88–4.74 (m, 7H, H-1 of CD), 4.60–3.90 (d, 1H, H-6 of CD), 3.90–3.50 (m, 28H, H-3, 6,5 of CD), 3.39–3.32 (m, 15H, H-2,4,6 of CD), 2.90 (m, –CH, 1H), 2.75 (m, N–CH2–C, 2H), 1.50–1.26 (m, –CH2, 10H), 1.22–1.20 (m, –CH2, 8H), 0.85–0.72 (m, –CH3, 6H). 13C NMR (125 MHz, DMSO-d6): δ = 205.96, 171.42, 167.45, 154.22, 101.76, 92.60, 81.16, 72.72, 72.62, 72.12, 71.67, 59.58, 54.00, 46.98, 35.3, 33.58, 32.48, 30.31, 28.62, 28.34, 26.26, 26.08, 21.72, 21.64, 13.46, 11.32.
4. Conclusions
This proposed research designed and prepared seven supramolecular polymer monomers (BC4PUPy, BC4HUPy, C4PUPy, C4HUPy, β-CDPUPy, β-CDBUPy, β-CDHUPy). 1H NMR and 13C NMR were employed to characterize them. their adsorption effects on heavy metal ions (Pd2+, Gd2+, Ni2+, Zn2+ and Cu2+) were investigated by atomic absorption method. The adsorption rate of Pb2+ was 99% after self-assembly of supramolecular polymeric monomers based on calix[4]arene, and the adsorption rate of Cd2+ was 99% after self-assembly of supramolecular polymeric monomers based on β-cyclodextrin. In addition, the adsorption rate of the supramolecular polymer formed by the self-assembly of β-CDHUPy on Pb2+ was 99%, which can be used for the adsorption of Pb2+ under special circumstances. This research will provide more excellent adsorption materials to control heavy metal pollution.
Data availability
All relevant data are within the paper.
Conflicts of interest
There are no conflicts to declare.
Acknowledgements
We gratefully acknowledge the financial support of NSFC (No. 21461030).
Notes and references
- K. Mamleev, J. Cejka, V. Eigner, M. Krupicka, H. Dvoraková and P. Lhoták, RSC Adv., 2024, 14, 13463–13473 RSC.
- Y. S. Jiao, S. Sanz, N. V. Izarova, J. van Leusen, S. Sarwar, S. J. Dalgarno, E. K. Brechin and P. Kögerler, Dalton Trans., 2022, 51, 5409–5413 RSC.
- Z. Z. Zhang, Y. X. Yue, Q. S. Li, Y. Wang, X. Y. Wu, X. Li, H. B. Li, L. Q. Shi, D. S. Guo and Y. Liu, Adv. Funct. Mater., 2023, 33, 2213967 CrossRef CAS.
- M. R. Dworzak, C. M. Montone, N. I. Halaszynski, G. P. A. Yap, C. J. Kloxin and E. D. Bloch, Chem. Commun., 2023, 59, 8977–8980 RSC.
- D. A. Blaj, M. Balan-Porcarasu, V. H. C. Peptu and C. Peptu, Carbohydr. Polym., 2024, 334, 122032 CrossRef CAS PubMed.
- V. Patamia, E. Saccullo, C. Zagni, R. Tomarchio, G. Quattrocchi, G. Floresta and A. Rescifina, Chem.–Eur. J., 2024, 30, e202303984 CrossRef CAS PubMed.
- F. Bahavarnia, M. Hasanzadeh, P. Bahavarnia and N. Shadjou, RSC Adv., 2024, 14, 13384–13412 RSC.
- Y. Zhang, Y. Chen, J. Q. Li, S. E. Liu and Y. Liu, Adv. Sci., 2024, 11, 2307777 CrossRef CAS PubMed.
- L. Li, K. Kang, T. S. Chee, Z. J. Tian, Q. Sun and C. L. Xiao, Adv. Sci., 2024, 11, 2308663 CrossRef CAS PubMed.
- M. Prakashni and S. Dasgupta, Org. Biomol. Chem., 2024, 22, 1871–1884 RSC.
- R. R. Golwankar, A. C. Ervin, M. Z. Makos, E. R. Mikeska, V. A. Glezakou and J. D. Blakemore, J. Am. Chem. Soc., 2024, 146, 9597–9604 CrossRef CAS PubMed.
- H. Ju, Z. L. Yin, Z. Demchuk, V. Bocharova, C. Gainaru, J. A. Laub, K. Vogiatzis, R. Advincula, J. Y. Chen and P. F. Cao, Adv. Funct. Mater., 2024, 2402165, DOI:10.1002/adfm.202402165.
- K. Diao, C. Ruan, R. Wang, S. Li, J. Jiang and L. Wang, Tetrahedron Lett., 2024, 137, 154941 CrossRef CAS.
- C. Ruan, Z. Li, W. Lin, R. N. Wang, W. Xie, H. Li, Y. Lu, R. Wang, S. Li and L. Wang, Org. Lett., 2024, 4122–4126, DOI:10.1021/acs.orglett.4c01243.
- H. Zheng, L. Fu, R. Wang, J. Jiao, Y. Song, C. Shi, Y. Chen, J. Jiang, C. Lin, J. Ma and L. Wang, Nat. Commun., 2024, 14, 590 CrossRef PubMed.
- Y. Chen, B. Sun, R. Wang, C. Shi, M. Cheng, J. Jiang, C. Lin and L. Wang, Org. Lett., 2024, 23, 7423–7427 CrossRef PubMed.
- A. Bruce, V. Adebomi, P. Czabala, J. Palmer, W. M. McFadden, Z. C. Lorson, R. L. Slack, G. Bhardwaj, S. G. Sarafianos and M. Raj, Angew. Chem., Int. Ed., 2024, 63, e202320045 CrossRef CAS PubMed.
- G. J. Saunders, S. A. Spring, E. Jayawant, I. Wilkening, S. Roesner, G. J. Clarkson, A. M. Dixon, R. Notman and M. Shipman, Chem.–Eur. J., 2024, 30, e202400308 CrossRef CAS PubMed.
- S. Huh, N. Batistatou, J. Wang, G. J. Saunders, J. A. Kritzer and A. K. Yudin, RSC Chem. Biol., 2024, 5, 328–334 RSC.
- Y. T. Li, K. Takamatsu, T. Aota and H. Konno, Org. Lett., 2024, 3375–3379, DOI:10.1021/acs.orglett.4c00808.
- A. Cowart, M. L. Brük, N. Zoglo, H. Roithmeyer, M. Uudsemaa, A. Trummal, K. Selke, R. Aav, E. Kalenius and J. Adamson, RSC Adv., 2023, 13, 1041–1048 RSC.
- W. T. Hu, B. L. Ye, G. C. Yu, H. Yang, H. Wu, Y. Ding, F. H. Huang, W. L. Wang and Z. W. Mao, Adv. Sci., 2024, 11, 2305382, DOI:10.1002/advs.202305382.
- W. W. Han, H. L. Tian, T. T. Qiang, H. Wang and P. Wang, Chem.–Eur. J., 2024, 30, 1002–1003, DOI:10.1002/chem.202303569.
- B. Lu, J. C. Xia, Y. Y. Huang and Y. Yao, Chem. Commun., 2023, 59, 12091–12099 RSC.
- Q. Song, J. Yang, J. Y. Rho and S. Perrier, Chem. Commun., 2019, 55, 5291–5294 RSC.
- J. H. Ren, M. Chang, W. J. Zeng, Y. H. Xia, D. H. Liu, G. Maurin and Q. Y. Yang, Chem. Mater., 2021, 33(13), 5108–5114 CrossRef CAS.
- J. Jia, Y. L. Liu, D. Q. Wu, J. Y. Yu, T. T. Gao and F. X. Li, Chem. Eng. J., 2023, 457, 141331, DOI:10.1016/j.cej.2023.141331.
- X. H. Ruan, C. Zhang, Y. Z. Zhu, F. L. Cai, Y. J. Yang, J. H. Feng, X. J. Ma, Y. Zheng, H. H. Li, Y. Yuan and G. S. Zhu, Angew. Chem., Int. Ed., 2023, 62(7), e202216549, DOI:10.1002/anie.202216549.
- B. Moosa, L. O. Alimi, W. B. Lin, A. Fakim, P. M. Bhatt, M. Eddaoudi and N. M. Khashab, Angew. Chem., Int. Ed., 2023, 62(46), e202311555, DOI:10.1002/anie.202311555.
- Q. Chen, J. Zhou, Q. Han, Y. H. Wang and Y. Z. Fu, Biochem. Eng. J., 2012, 69, 155–158 CrossRef CAS.
- S. Y. Zhou, L. J. Jin, P. Y. Gu, L. C. Tian, N. J. Li, D. Y. Chen, A. Marcomini, Q. F. Xu and J. M. Lu, Chem. Eng. J., 2022, 433, 134442, DOI:10.1016/j.cej.2021.134442.
- H. Y. Li, L. Zhang, W. J. Yu, W. W. Bie, M. J. Wei, Z. X. Wang, F. Y. Kong and W. Wang, Carbohydr. Polym., 2023, 312, 120832, DOI:10.1016/j.carbpol.2023.120832.
- G. M. Nisola, K. J. Parohinog, M. K. Cho, F. K. B. Burnea, J.
Y. Lee, J. G. Seo, S. P. Lee and W. J. Chung, Chem. Eng. J., 2020, 389, 123421, DOI:10.1016/j.cej.2019.123421.
- S. Y. Li, D. Wang, Y. L. L. Wang and L. P. Yang, Curr. Opin. Green Sustainable Chem., 2023, 40, 100755, DOI:10.1016/j.cogsc.2023.100755.
- C. Schäfer, P. N. Perera, F. Laible, D. L. Olynick, A. M. Schwartzberg, A. Weber-Bargioni, S. Cabrini, P. J. Schuck, D. P. Kern and M. Fleischer, Nanoscale, 2020, 12, 19170–19177 RSC.
- X. Q. Zhang, R. Cao, Z. H. Chen, L. L. Zhou, P. P. Li, H. Zhang and J. Wu, J. Electrochem. Soc., 2024, 171, 027515, DOI:10.1149/1945-7111/ad2646.
- H. D. Li, L. L. Li and B. Z. Yin, Inorg. Chem. Commun., 2014, 42, 1–4 CrossRef CAS.
- I. Pisagatti, G. Gattuso, A. Notti, M. F. Parisi, G. Brancatelli, S. Geremia, F. Greco, S. Millesi, A. Pappalardo, L. Spitaleri and A. Gulino, RSC Adv., 2018, 8, 33269–33275 RSC.
- T. T. Feng, S. Z. Yan, Z. Wang and X. H. Fan, J. Lumin., 2022, 37, 922–929 CrossRef CAS PubMed.
- J. M. Notestein, E. Iglesia and A. Katz, J. Am. Chem. Soc., 2004, 126, 16478–16486 CrossRef CAS PubMed.
- T. F. Machado, G. Utzeri, A. J. M. Valente, M. E. S. Serra and D. Murtinh, Carbohydr. Polym., 2024, 326, 121612, DOI:10.1016/j.carbpol.2023.121612.
- Y. T. Wu, Q. Li, J. J. Cao, Y. Liu, Z. J. Wang, L. Q. Shangguan and H. T. Z. Zhu, ACS Appl. Nano Mater., 2021, 4, 11126–11133 CrossRef CAS.
- R. X. Yao, Y. J. Li, J. H. Wang, J. Z. Chen and Y. S. Xu, J. Catal., 2023, 418, 78–89 CrossRef CAS.
- C. Lin, Y. Shen, X. J. Guo, W. G. Duan, Y. Huang, G. B. Huang and L. Z. Liu, RSC Adv., 2024, 14, 16278–16283 RSC.
- H. L. Fu, J. Y. Huang, J. J. B. van der Tol, L. Su, Y. Y. Wang, S. Dey, P. Zijlstra, G. Fytas, G. Vantomme, P. Y. W. Dankers and E. W. Meijer, Nature, 2024, 626, 1011–1018, DOI:10.1038/s41586-024-07034-7.
- C. J. Thrasher, F. Jia, D. W. Yee, J. M. Kubiak, Y. P. Wang, M. S. Lee, M. Onoda, A. J. Hart and R. J. Macfarlane, J. Am. Chem. Soc., 2024, 146, 11532–11541 CAS.
- J. P. Ouyang, Z. F. Zhang, J. Li and C. Z. Wu, Angew. Chem., Int. Ed., 2024, 63, e202400105, DOI:10.1002/anie.202400105.
- H. Liu, Z. Q. Hu and X. F. Ji, Chem.–Eur. J., 2024, 30, e202400099 CrossRef CAS PubMed.
- R. P. Sijbesma, F. H. Beijer, L. Brunsveld, B. J. Folmer, J. H. Hirschberg, R. F. Lange, J. K. Lowe and E. W. Meijer, Science, 1997, 278, 1601–1604 CrossRef CAS PubMed.
- W. P. J. Appel, M. M. L. Nieuwenhuizen, M. Lutz, B. F. M. de Waal and A. R. A. E. W. Meijer, Chem. Sci., 2014, 5, 3735–3745 RSC.
- G. B. W. L. Ligthart, H. Ohkawa, R. P. Sijbesma and E. W. Meijer, J. Am. Chem. Soc., 2005, 127, 810–811 CrossRef CAS PubMed.
- R. P. Sijbesma and E. W. Meijer, Chem. Commun., 2003, 5–16 RSC.
- A. El-ghayoury, E. Peeters, A. P. H. J. Schenning and E. W. Meijer, Chem. Commun., 2000, 1969–1970 RSC.
- F. Glasneck, Q. I. Roode-Gutzmer, T. Stumpf and B. Kersting, Chem.–Eur. J., 2022, 28, e202104301, DOI:10.1002/chem.202104301.
- S. Cataldo, P. Lo Meo, P. Conte, A. Di Vincenzo, D. Milea and A. Pettignano, Carbohydr. Polym., 2021, 267, 118151, DOI:10.1016/j.carbpol.2021.118151.
- L. Liu and K. Zhang, Environ. Sci. Technol., 2018, 52, 5884–5891 CrossRef CAS PubMed.
- G. U. Akkus, E. Al and S. E. Korcan, Supramol. Chem., 2015, 27, 522–526 CrossRef.
- S. Memon, E. Akceylan, B. Sap, M. Tabakci, D. M. Roundhill and M. Yilmaz, J. Polym. Environ., 2003, 11, 67–74 CrossRef CAS.
- W. W. Bie, S. Z. Zhang, L. Zhang, H. Y. Li, X. Y. Sun, T. P. Cai, Z. X. Wang, F. Y. Kong and W. Wang, Carbohydr. Polym., 2024, 324, 121509, DOI:10.1016/j.carbpol.2023.121509.
- N. Goyal, A. Amar, S. Gulati and R. S. Varma, ACS Appl. Nano Mater., 2023, 6, 13766–13791 CrossRef CAS.
- J. Chen, C. Y. Bao, M. S. Chen, Y. Wang, X. L. Xu, T. Yang, C. Y. Shang and Q. Zhang, Chem. Eng. J., 2023, 464, 142526, DOI:10.1016/j.cej.2023.142526.
- B. F. Zhao, L. Y. Jiang and Q. Jia, Chin. Chem. Lett., 2022, 33, 11–21 CrossRef CAS.
- G. Yang, D. F. Fang, L. M. Yang, Z. M. Wei, Y. Y. Tu, P. H. Shao, Z. Hua, Z. K. Wang and X. B. Luo, J. Cleaner Prod., 2022, 330, 129882, DOI:10.1016/j.jclepro.2021.129882.
- L. Sellaoui, D. I. Mendoza-Castillo, H. E. Reynel-Ávila, B. A. Ávila-Camacho, L. L. Díaz-Muñoz, H. Ghalla, A. Bonilla-Petriciolet and A. B. Lamine, Chem. Eng. J., 2019, 365, 305–316 CrossRef CAS.
|
This journal is © The Royal Society of Chemistry 2024 |
Click here to see how this site uses Cookies. View our privacy policy here.