DOI:
10.1039/D4RA05619A
(Paper)
RSC Adv., 2024,
14, 33587-33591
Synthesis of β-enamino malonates through caesium carbonate-promoted reaction of nitro-substituted donor–acceptor cyclopropanes†
Received
2nd August 2024
, Accepted 16th October 2024
First published on 22nd October 2024
Abstract
A caesium carbonate-promoted reaction of nitro-substituted donor–acceptor cyclopropanes (DACs) with primary aromatic amines in water provides a convenient access to β-enamino malonates under mild reaction conditions. The transformation takes place through the formation of allene intermediates from the DACs followed by the conjugate addition of various primary aromatic amines to the intermediates. The reaction proceeds more efficiently in water as compared with organic solvents and the products were isolated in good yields by filtering through a silica gel column without any prior extraction procedure.
Introduction
Donor–Acceptor Cyclopropanes (DACs) are one of the types of readily available and easily manipulable building blocks that give access to different classes of organic compounds.1 Simple reagents are often enough to trigger their characteristic reactions such as ring-opening,2 annulation3 and rearrangement.4 DACs having an extra aroyl or nitro group (apart from the regular donor–acceptor groups) usually display distinct reactivity and afford products that are difficult to obtain by other methods.5 In this context, nitro-substituted DACs are renowned for their unique reactivity and they preferentially undergo ring-opening reactions rather than annulation reactions.6 When treated with Lewis acids, nitro-substituted DACs form aroylmethylidene malonates as products or intermediates, which serve as versatile building blocks for a handful of heterocyclic and acyclic compounds.6c,7 On the other hand, when treated with bases, they form arylethylidenes or allenes as intermediates, which too serve as potential precursors for various compounds.8
Recently, we reported that nitro-substituted DACs when reacted with salicylaldehydes in the presence of caesium carbonate and a surfactant in water furnished a series of chromane derivatives through allene intermediates.9 During the course of the study, we observed that nitro-substituted DAC 1a reacts with 2-iodophenol (2) under the same reaction conditions to give the respective Michael adduct 3 (Scheme 1, eqn (1)). The observation prompted us to investigate the reaction of nitro-substituted DACs 1 with amines 4 for the synthesis of β-enamino malonates 5 (or 6) (Scheme 1, eqn (2)).
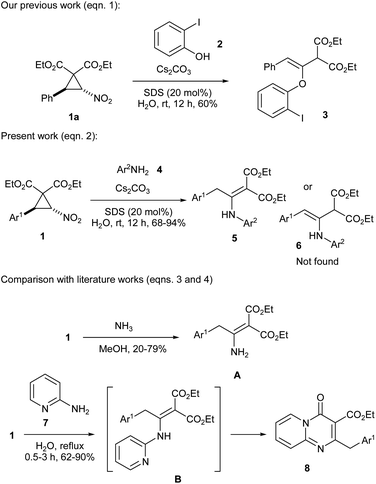 |
| Scheme 1 Comparison of the present work with literature works. | |
It may be noted that enamines and their derivatives are versatile synthetic intermediates and are usually synthesized by the condensation of amines with carbonyl compounds,10 hydroamination of alkynes11 or cross-coupling reaction between amines and vinyl halides or triflates.12 Although, a handful of methods are available for the synthesis of enamino esters such as β-enamino malonates,13 DACs have been rarely used as starting materials for their synthesis. Sopova and coworkers have used nitro-substituted DACs 1 for the access of enamino malonates A using ammonia in methanol (Scheme 1, eqn (3)).14 We record that our previous work involving synthesis of pyridopyrimidinones 7 from nitro-substituted DACs 1 and 2-aminopyridine (6) in water should have also taken place through the formation of the respective enamino malonate intermediates B (Scheme 1, eqn (4)).8b In this context, the present work represents a convenient green strategy for the access of β-enamino malonates from amines and nitro-substituted DACs using water as solvent. It is interesting to note that water acts as a better solvent than organic solvents for certain reactions of nitro-substituted DACs, likely due to the involvement of polar intermediates.
Results and discussion
We began the study by reacting nitro-substituted DAC 1a with various primary aromatic amines 4a–f (Table 1, entries 1–6). Owing to the greenness of the reaction conditions reported in the previous work [caesium carbonate, sodium dodecylsulphate (20 mol%), water, rt],9 we adapted the same reaction conditions for the present transformation. Accordingly, we reacted DAC 1a with aniline 4a under the above reaction conditions for 12 h. Then, the crude reaction mixture (without extraction) was made into slurry by mixing with a little silica gel and charged on a silica gel column. After eluting the column with 10% EtOAc in hexane (filtration), the expected enamino malonate 5a was obtained in 87% yield (Table 1, entry 1). Out of curiosity, we also tested the suitability of organic solvents such as MeOH, EtOH, DCM, 1,2-DCE, THF, acetone, MeCN, DMF, DMSO and toluene for the transformation. Though the reaction takes place in MeOH and EtOH (the yields of 5a were 28% and 23%, respectively, in these solvents), it did not take place in other organic solvents. Next, we investigated the reaction of 1a with anilines having different electron donating, halogen and electron withdrawing substituents. With p-toluidine, the respective product enamino malonate 5b was obtained in 68% yield by adapting the same procedure (entry 2). The presence of strong electron donating or withdrawing groups on aniline was not tolerated in the transformation. Thus, the reaction of 1a with 4-methoxyaniline (4c) or p-nitroaniline (4d) led to a complicated mixture of products (entries 3 and 4). The reaction proceeded smoothly with o-iodoaniline (4e) and 1-naphthylamine (4f) and the respective enamino malonates 5e and 5f were obtained in 73% and 76% yields, respectively (entries 5 and 6). Next, we investigated the scope of the transformation for nitro-substituted DACs 1b–j having different aryl groups with various primary aromatic amines (Table 1, entries 7–32). The presence of moderately electron donating methyl and isopropyl groups and also halogens on the aryl ring of DACs (1b, 1c and 1e–g) was well tolerated in the transformation and the respective enamino malonates were obtained in 71–94% yields (entries 7–14 and 17–25). At the same time, DACs having a good electron donating group such as methoxy on the aryl ring (1d) as well as an electron-rich aryl ring such as 2-thienyl (1j) were not compatible in the transformation and they formed complex mixtures of products, possibly due to the enhanced reactivity of the aryl rings (entries 15, 16, 31 and 32). Similarly, DAC 1h having a strong electron withdrawing nitro group also did not undergo the expected reaction owing to the destabilizing effect of the aryl ring on the 1,3-zwitterionic intermediate that would be formed from the cyclopropane during the course of the reaction (see the mechanism).6c,7a We also reacted DAC 1i having a 1-naphthyl group with different anilines and the corresponding enamino malonates 5ab–5ad were formed in 78–93% yields (entries 28–30).
Table 1 The scope of the transformationa
Isolated yield. Complicated mixture of products. No reaction. |
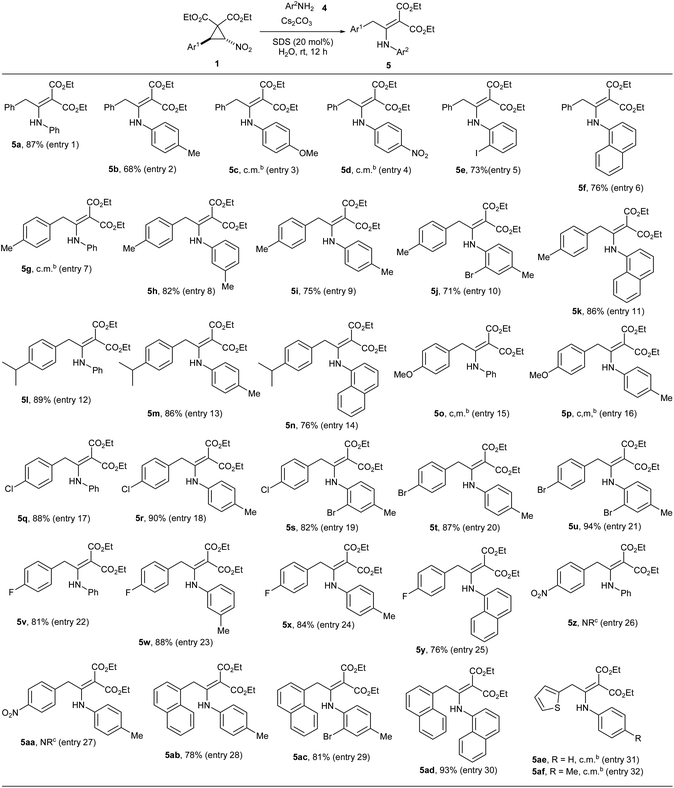 |
Next, we focused our attention on the reaction of DAC 1b with aromatic secondary amines such as N-methylaniline and indoline and aliphatic amines such as benzylamine, diisopropylamine, pyrrolidine and morpholine. Unfortunately, none of them gave the expected enamino malonates. During the investigation of the scope of the transformation with different amines, surprisingly, we observed that when DACs 1b and 1c were reacted with benzotriazole (9), they afforded the isomeric enamines 10a and 10b in 79 and 85% yields, respectively (Scheme 2). However, the transformation did not work with benzimidazole.
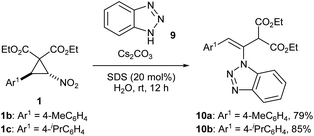 |
| Scheme 2 Reaction of DACs 1b and 1c with benzotriazole (9). | |
We propose a mechanism outlined in Scheme 3 for the formation of β-enamino malonates from DACs 1 and aromatic amines. Caesium carbonate removes the acidic proton at C-3 of the DAC, which opens the cyclopropane ring to produce intermediate P. The intermediate P may loose the nitro group to form the allene intermediate Q.8c,9 The aza-Michael addition of amine 4 to Q followed by migration of double bond to a conjugate position may afford enamino malonate 5. In case of benzotriazole, the final double bond migration is not taking place.
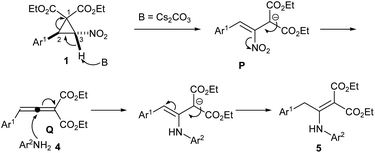 |
| Scheme 3 Plausible mechanism of the transformation. | |
We have also performed a gram scale synthesis of β-enamino malonate 5a as a model substrate in order to evaluate various green chemistry metrics for the transformation (Table 2). Pleasingly, the transformation has a low E-factor (0.3648 kg waste per kg product) and high atom economy (88%), atom efficiency (73%), carbon efficiency (100%) and reaction mass efficiency (73%), indicating the greenness of the methodology.
Table 2 Evaluation of green chemistry metrics for the gram-scale synthesis of 5a
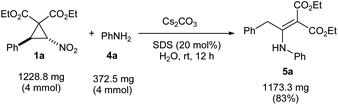
|
Entry |
Chemicals |
Molecular weight (g mol−1) |
Weight (mg) |
Substrate 1a |
Diethyl 2-nitro-3-phenylcyclopropane-1,1-dicarboxylate |
307.3020 (C15H17NO6) |
1228.8 |
Substrate 4a |
Aniline |
93.13 (C6H7N) |
372.52 |
Product 5a |
Diethyl 2-[2-phenyl-1-(phenylamino)ethylidene]malonate |
353.4116 (C21H23NO4) |
1173.3 |
Base |
Caesium carbonate |
325.82 (Cs2CO3) |
1433.61 |
Surfactant |
Sodium dodecyl sulphate |
288.38 (C12H25NaO4S) |
230.70 |
Solvent |
Water |
Product yield = 83% |
E-factor = [(1228.8 + 372.52) − 1173.3] ÷ 1173.3 = 0.3648 kg waste per kg product |
Atom economy = 353.4116 ÷ (307.3020 + 93.13) × 100 = 88% |
Atom efficiency = (83 × 88) ÷ 100 = 73% |
Carbon efficiency = 21 ÷ 21 = 100% |
Reaction mass efficiency = [1173.3 ÷ (1228.8 + 372.2)] × 100 = 73% |
In order to prove the synthetic utility of the products made in the present study, we subjected enamino malonate 5a to oxidation using SeO2 in 1,4-dioxane under reflux conditions. Pleasingly, the reaction afforded the aroylmethylidene malonate derivative 11 in 81% yield (Scheme 4).
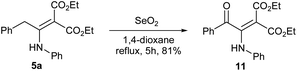 |
| Scheme 4 Synthetic transformation of 5a into aroylmethylidene malonate 11. | |
Conclusions
In summary, we have developed a green procedure for the synthesis of β-enamino malonates from nitro-substituted DACs and primary aromatic amines. Water was found to be an efficient medium for the transformation and the products were isolated in good yields simply by passing the crude reaction mixture through a silica gel column by bypassing the extraction step. The reaction proceeds through caesium carbonate promoted ring-opening of the cyclopropane to form allene intermediates followed by the addition of amines. The operational simplicity, the use of water as medium, mild reaction conditions, simple purification procedure and reasonably wide substrate scope are some of the green aspects of the methodology.
Experimental section
General remarks
Melting points were determined by the open capillary tube method and are uncorrected. The 1H and 13C NMR spectra were recorded on a 400 MHz NMR spectrometer. High-resolution mass spectra (ESI) were recorded on a Q-TOF mass spectrometer. X-ray crystallographic data were collected on a CCD diffractometer using graphite-monochromated Mo Kα radiation. Thin layer chromatography (TLC) was performed on precoated alumina sheets and detected under UV light. Silica gel (200–400 mesh) was used for column chromatography. Cyclopropanes 1a–j were prepared as per our reported procedure6c and all are known compounds.6c,8a,15
General procedure for the synthesis of β-enamino malonates 5a–af and 10a–b
To a suspension of nitrocyclopropane dicarboxylates 1 (1.0 mmol) in water (2 mL) were added aromatic primary amine 4 (1.0 mmol), sodium dodecyl sulfate (58 mg, 0.2 mmol), and caesium carbonate (358 mg, 1.1 mmol) and stirred at room temperature for 12 h. Next, the reaction mixture was mixed with silica gel to make slurry, charged on a silica gel column and eluted using ethyl acetate/hexane (1
:
9 v/v) to obtain the pure product.
Data availability
The data supporting this article have been included as part of the ESI.†
Conflicts of interest
There are no conflicts to declare.
Acknowledgements
The authors thank Science and Engineering Research Board (SERB), Council of Scientific and Industrial Research (CSIR) and MoE-RUSA 2.0, India for financial support and DST-FIST for instrumentation facilities at School of Chemistry, Bharathidasan University.
Notes and references
-
(a) H. U. Reissig and R. Zimmer, Chem. Rev., 2003, 103, 1151–1196 CrossRef CAS PubMed;
(b) M. Yu and B. L Pagenkopf, Tetrahedron, 2005, 61, 321–347 CrossRef CAS;
(c) M. Y. Melnikov, E. M. Budynina, O. A. Ivanova and I. V. Trushkov, Mendeleev Commun., 2011, 21, 293–301 CrossRef CAS;
(d) T. F. Schneider, J. Kaschel and D. B. Werz, Angew. Chem., Int. Ed., 2014, 53, 5504–5523 CrossRef CAS PubMed;
(e) H. K. Grover, M. R. Emmett and M. A. Kerr, Org. Biomol. Chem., 2015, 13, 655–671 RSC;
(f) P. Singh, R. K. Varshnaya, R. Dey and P. Banerjee, Adv. Synth. Catal., 2020, 362, 1447–1484 CrossRef CAS;
(g) Y. Xia, X. Liu and X. Feng, Angew. Chem., Int. Ed., 2021, 60, 9192–9204 CrossRef CAS PubMed;
(h) Donor-Acceptor Cyclopropanes in Organic Synthesis, ed. P. Banerjee and A. T. Biju, Wiley-VCH, Weinheim, 2024 Search PubMed.
-
(a) E. M. Budynina, K. L. Ivanov, I. D. Sorokin and M. Y. Melnikov, Synthesis, 2017, 49, 3035–3068 CrossRef CAS;
(b) V. Pirenne, B. Muriel and J. Waser, Chem. Rev., 2021, 121, 227–263 CrossRef CAS PubMed;
(c) E. Reyes, U. Uria, L. Prieto, L. Carrillo and J. L. Vicario, Chem. Commun., 2024, 60, 7288–7298 RSC.
-
(a) V. K. Yadav and V. Sriramurthy, Angew. Chem., Int. Ed., 2004, 43, 2669–2671 CrossRef CAS PubMed;
(b) A. T. Parsons and J. S. Johnson, J. Am. Chem. Soc., 2009, 131, 3122–3123 CrossRef CAS PubMed;
(c) G. Sathishkannan and K. Srinivasan, Org. Lett., 2011, 13, 6003–6005 CrossRef;
(d) S. Chakrabarty, I. Chatterjee, B. Wibbeling, C. G. Daniliuc and A. Studer, Angew. Chem., Int. Ed., 2014, 53, 5964–5968 CrossRef CAS PubMed;
(e) M. A. Zotova, R. A. Novikov, E. V. Shulishov and Y. V. Tomilov, J. Org. Chem., 2018, 83, 8193–8207 CrossRef CAS PubMed;
(f) S. Nicolai and J. Waser, Angew. Chem., Int. Ed., 2022, 61, e202209006 CrossRef CAS PubMed;
(g) L. Yang, H. Wang, M. Lang and S. Peng, Synthesis, 2023, 56, 389–398 Search PubMed.
-
(a) J. Kaschel, T. F. Schneider, P. Schirmer, C. Maab, D. Stalke and D. B. Werz, Eur. J. Org. Chem., 2013, 4539–4551 CrossRef CAS;
(b) H. Chen, J. Zhang and D. Z. Wang, Org. Lett., 2015, 17, 2098–2101 CrossRef CAS PubMed;
(c) S. Thangamalar, M. Thangamani and K. Srinivasan, Org. Biomol. Chem., 2022, 20, 3145–3153 RSC;
(d) S. Jiru, Y. Fu and S. R. Wang, Org. Lett., 2023, 25, 555–559 CrossRef PubMed;
(e) F. L. Daniel and K. Srinivasan, J. Org. Chem., 2024, 89, 5304–5313 CrossRef CAS PubMed.
- T. Selvi and K. Srinivasan, Isr. J. Chem., 2016, 56, 454–462 CrossRef CAS.
-
(a) O. Litchits, D. Alberico, I. Zhakharian and A. B. Charette, J. Org. Chem., 2008, 73, 6838–6840 CrossRef PubMed;
(b) S. S. So, T. J. Auvil, V. J. Garza and A. E. Mattson, Org. Lett., 2012, 14, 444–447 CrossRef CAS PubMed;
(c) T. Selvi and K. Srinivasan, J. Org. Chem., 2014, 79, 3653–3658 CrossRef CAS PubMed.
-
(a) T. Selvi and K. Srinivasan, Chem. Commun., 2014, 50, 10845–10848 RSC;
(b) T. Selvi and K. Srinivasan, Adv. Synth. Catal., 2015, 357, 2111–2118 CrossRef CAS;
(c) T. Selvi, G. Vanmathi and K. Srinivasan, RSC Adv., 2015, 5, 49326–49329 RSC;
(d) M. Meenakshi and K. Srinivasan, Org. Biomol. Chem., 2022, 20, 8741–8746 RSC.
-
(a) G. Yang, W. Liu, Z. He and Zh. He, Org. Lett., 2016, 18, 4936–4939 CrossRef PubMed;
(b) S. Selvi and K. Srinivasan, Eur. J. Org. Chem., 2017, 5644–5648 CrossRef CAS;
(c) Z.-Y. Zhou, Z.-Y. Xu, Q.-Y. Shen, L.-S. Huang, X. Zhang, A.-B. Xia, D.-Q. Zu and Z.-Y. Xu, Chem. Commun., 2021, 57, 6424–6427 RSC.
- S. R. Jeny and K. Srinivasan, J. Org. Chem., 2023, 88, 9395–9400 CrossRef CAS PubMed.
-
(a) D. Sanchez, D. Bastida, J. Bures, C. Isart, O. Pineda and J. Vilarrasa, Org. Lett., 2012, 14, 536–539 CrossRef CAS PubMed;
(b) D. Xin and K. Burgess, Org. Lett., 2014, 16, 2108–2110 CrossRef CAS PubMed.
-
(a) S. Kramer, K. Dooleweerdt, A. T. Lindhardt, M. Rottlander and T. Skrydstrup, Org. Lett., 2009, 11, 4208–4211 CrossRef CAS PubMed;
(b) J. Bahri, N. Tanbouza, T. Ollevier, M. Taillefer and F. Monnier, Synthesis, 2019, 51, 2086–2090 Search PubMed.
-
(a) J. R. Dehli, J. Legros and C. Bolm, Chem. Commun., 2005, 973–986 RSC;
(b) X. Ji, H. Huang, W. Wu, X. Li and H. Jiang, J. Org. Chem., 2013, 78, 11155–11162 CrossRef CAS PubMed.
-
(a) N. D. Koduri, H. Scott, B. Hileman, J. D. Cox, M. Coffin, L. Glicksberg and S. R. Hussaini, Org. Lett., 2012, 14, 440–443 CrossRef CAS;
(b) N. D. Koduri, B. Hileman, J. D. Cox, H. Scott, P. Houng, A. Robbins, K. Bowers, L. Tsebaot, K. Miao, M. Castaneda, M. Coffin, G. Wei, T. D. W. Claridge, K. P. Roberts and S. R. Hussaini, RSC Adv., 2013, 3, 181–188 RSC;
(c) N. D. Koduri, Z. Wang, G. Cannell, K. Cooley, T. M. Lemma, K. Miao, M. Nguyen, B. Frohock, M. Castaneda, H. Scott, D. Albinescu and S. R. Hussaini, J. Org. Chem., 2014, 79, 7405–7414 CrossRef CAS PubMed;
(d) P.-Q. Huang, W. Ou and J.-L. Ye, Org. Chem. Front., 2015, 2, 1094–1106 RSC;
(e) P.-Q. Huang, W. Ou and J.-L. Ye, Eur. J. Org. Chem., 2017, 582–592 CrossRef CAS.
- A. S. Sopova, O. S. Bakova, K. L. Metelkina and V. V. Perekalin, Zh. Org. Khim., 1975, 11, 68–71 (Chem. Abstr., 1975, 83, 9406h) CAS.
- S. Thangamalar and K. Srinivasan, Eur. J. Org. Chem., 2023, 26, e202201084 CrossRef CAS.
|
This journal is © The Royal Society of Chemistry 2024 |
Click here to see how this site uses Cookies. View our privacy policy here.