DOI:
10.1039/D4RA05694A
(Review Article)
RSC Adv., 2024,
14, 33429-33448
The significance of chirality in contemporary drug discovery-a mini review
Received
6th August 2024
, Accepted 15th October 2024
First published on 22nd October 2024
Abstract
More than half of drugs are chiral compounds with their chirality determining their molecular interactions, ecofriendly environmental safety and efficacy. Overall nearly 90% of chiral compounds are marketed as racemates consisting of an equimolar mixture of two enantiomers. Despite having identical chemical structure and bonding, racemates function differently when exposed to chiral environments and demonstrate notable variances in biological properties such as pharmacology, toxicology, metabolism and pharmacokinetics, etc. Advancements in asymmetric synthesis in recent years have led to considerable interest in the development of single enantiomers of chiral drug molecules for medicinal chemistry settings. In this review, we want to compile examples of chiral medicines approved by the FDA in the years 2022 and 2023 with an emphasis on their synthesis along with information on chiral induction as well as enantiomeric excess.
1 Introduction
Chirality influences a drug's binding affinity and interactions with its target, hence defining its pharmacology. As a result, in 1992, the Food and Drug Administration (FDA) established a set of criteria for the pharmaceutical evolution of single enantiomers and racemates.1 Since then, the majority of medications on the market have been chiral, but the number of single-enantiomer and single-diastereomer pharmaceuticals has steadily increased.2,3 Fig. 1 depicts examples of chiral FDA-approved medications, as well as current clinical trial disclosures. Atropisomerism is an important system of chirality that results from a partial bond rotation, typically inside a sp2–sp2. The FDA-approved small-molecule medicines since 2011 signified that 30% of these molecules include at least one atropisomeric axis.4 Natural products contain a higher sp3 fraction (Fsp3) and more chiral centers than many drug-like small compounds that have been created so far. It has been proposed that improved drug-like qualities, such as greater solubility5 and fewer off-target hits (reduced promiscuity), are positively correlated with a higher degree of Fsp3, which is defined as the proportion of sp3-hybridized carbons.6,7 Fully sp2 compounds can occasionally have a high 3D character; a high Fsp3 number does not always correspond to an increased 3D shape.
 |
| Fig. 1 Few examples of FDA-approved chiral small-molecule drugs. | |
Single enantiomers have gained popularity in recent decades. In 2015, the FDA approved lesinurad as Zurampic to treat hyperuricaemia in gout patients. It was also proposed that (−)-lesinurad could be a more effective activity for hyperuricaemia than racemate. Additionally, the FDA authorized stiripentol in 2018. When used concurrently, it also inhibits the metabolism of other anticonvulsant medications. The R(+)-enantiomer of stiripentol (I) was discovered to be 2.4 times more potent than the S(−)-enantiomer of stiripentol (II), with the racemate's potency falling between the two enantiomers (Fig. 1). On the contrary, several FDA-approved racemic medicines outperform single enantiomers. The FDA approved amisulpride under the brand name Barhemsys in 2020 for the treatment of nausea and vomiting following surgery. The S(−)-enantiomer of amisulpride (I) is 40 times more efficient for the D2 receptor than the R(+)-enantiomer of amisulpride (II), but II is 50 times more potent for the 5-HT7 receptor. As a result, the racemic version of amisulpride has a poly-pharmaceutical potential benefit over its single enantiomers.8 Therefore, structure–activity relationship studies (SAR) along with chiral synthesis is of furthermost importance and anticipated to ease therapeutic activity of lead compounds. With the changes of chirality, structure of the compound will be changed. As a result of that the mode of action of particular compound will vary accordingly. Thus, chirality is paramount to determine the structure of the compounds.
Nature is handed. The complexity of life arises owing to the handedness of nature. Attempts to mimic the biologically active molecules in the lab are difficult, as living things are made up of sugars (mostly D-) and amino acids (mostly L-) that are chiral. Chiral chemistry was discovered in 1848 by French chemist Louis Pasteur.9 The pharmaceutical industry took almost a century to understand the importance of chiral molecules in human existence, agriculture, and the medicinal field. Chirality has a significant influence on the design and synthesis of medicines, as the database shows that about 50% of drugs launched so far contain stereochemical elements. The presence of chirality in receptors and enzymes demands stereoselective interaction of drug molecules. Employment of single enantiomers in medical treatment has brought asymmetric synthesis as a major theme in drug discovery.
One of the first utilized drug for the morning sickness of a pregnant women was Thalidomide. This drug was used for few years after that it was removed from the market due to its detrimental effect on fetal development. Here, the scenario was different, one of the enantiomers (R) of Thalidomide was actively potent for health, while other one (S-enantiomer) was less potent for that particular action. Recently, this drug has been introduced again and it works effectively to cure severe diseases, like cancer, specifically the multiple myeloma. It is so obvious that chirality act as a paramount role in the binding interaction and affinity between drug and its target. Since, then most of the marketed drugs are single enantiomer rather mixture of enantiomer.
Asymmetric synthesis has recently attracted significant interest across the global pharmaceutical market as it facilitates scalable and efficient routes to the synthesis of potent drug candidates (Fig. 2). Asymmetric synthesis can be approached in three ways: (1) chiral pool synthesis: synthesis from inexpensive and naturally available chiral substrates such as sugars and amino acids (2) chiral resolution: resolving the racemic mixture to produce a single enantiomer by adopting chiral chromatographic techniques (3) enantioselective induction: asymmetric induction achieved by employing chiral auxiliary, chiral catalyst, or chiral reagent (Fig. 3).10
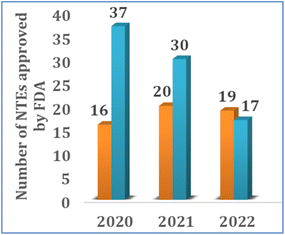 |
| Fig. 2 The total number of biologic (blue) and small molecule (orange) NTEs approved by the FDA between 2020 and 2022. | |
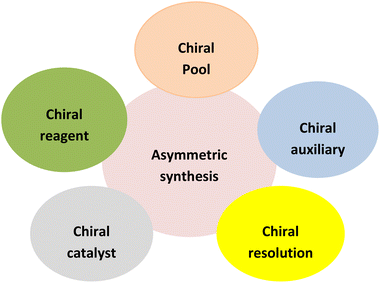 |
| Fig. 3 Methods of asymmetric synthesis. | |
2 Pharmaceutical approvals and chirality
During past decades, the total drugs were approved by FDA was mentioned in Table 1. FDA approved 37 new drugs in the year of 2022, 55 drugs in the year 2023 and almost 30 drugs in the year of 2024. The brand names, active ingredients, indications, and therapeutic area of some of the chiral drugs approved by the FDA in the years 2022, 2023 and 2024 (Fig. 4–6) were summarized and presented in Tables 2–4.12,13
Table 1 The total numbers of FDA approved achiral, single enantiomer, and racemic NMEs from 2002–2022
11
Year |
Achiral |
Single enantiomer |
Racemate |
Total no. |
No. |
% |
No. |
% |
No. |
% |
2003–2012 |
68 |
32 |
120 |
57 |
23 |
11 |
211 |
2013–2022 |
105 |
38 |
163 |
59 |
10 |
3.6 |
278 |
2002–2022 |
180 |
36 |
291 |
58 |
35 |
6.9 |
506 |
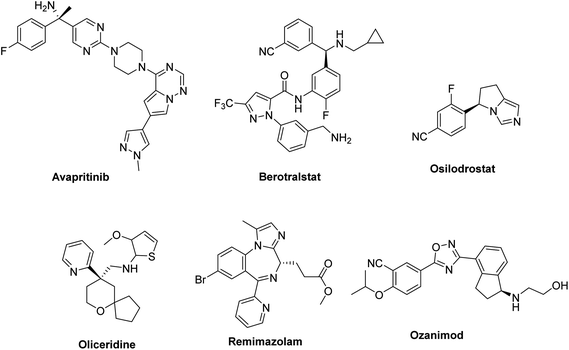 |
| Fig. 4 Few examples of structure of chiral drugs approved by FDA in 2000–2021. | |
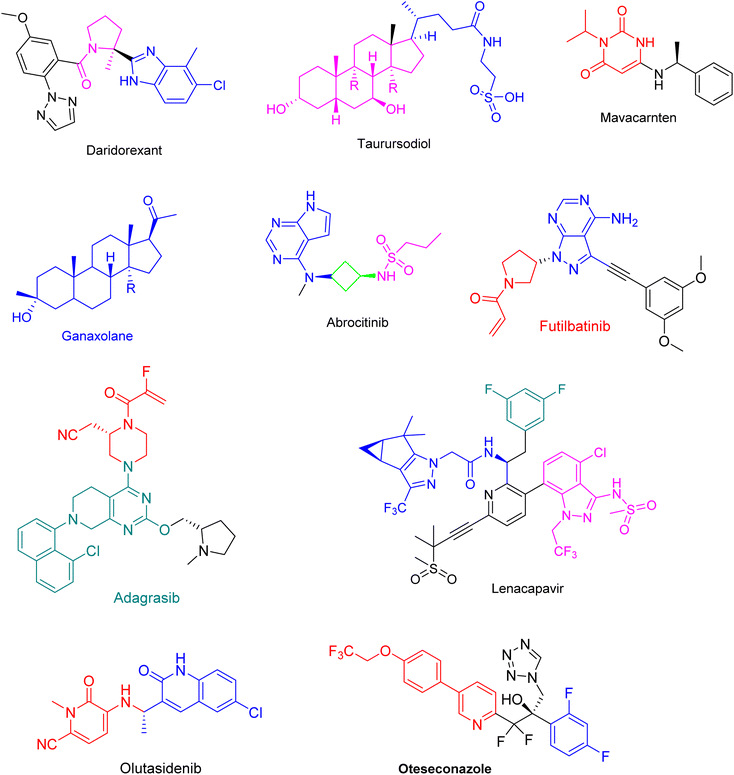 |
| Fig. 5 Structure of chiral drugs approved by FDA in 2022. | |
 |
| Fig. 6 Structure of chiral drugs approved by FDA in 2023. | |
Table 2 List of chiral drugs approved by FDA in 2022
Medicine name |
Active chemical |
Therapeutic area |
Indication/use |
Vivjoa |
Oteseconazole |
Infections |
To treat recurrent vulvovaginal candidiasis |
Quviviq |
Daridorexant |
Central nervous system |
To treat insomnia |
Cibinqo |
Abrocitinib |
Dermatology |
To treat atopic dermatitis |
Lytgobi |
Futibatinib |
Cancer |
To treat intrahepatic cholangiocarcinoma |
Krazati |
Adagrasib |
Cancer |
To treat non-small cell lung cancer |
Sunlenca |
Lenacapavir |
Infections |
To treat HIV-1 virus |
Rezlidhia |
Olutasidenib |
Haematology |
To treat relapsed or refractory acute myeloid leukemia |
Camzyos |
Mavacamten |
Cardiology |
To treat obstructive hypertrophic cardiomyopathy |
Ztalmy |
Ganaxolone |
Central nervous system |
To treat seizures in people with CDD |
Table 3 List of chiral drugs approved by FDA in 2023
Medicine name |
Active chemical |
Therapeutic area |
Indication/use |
Xacduro |
Sulbactam |
Infections |
To treat bacterial pneumonia |
Veozah |
Fezolinetant |
Central nervous system |
To treat vasomotor symptoms |
Daybue |
Trofinetide |
Central nervous system |
To treat rett syndrome |
Truqap |
Capivasertib |
Cancer |
To treat HR positive, HER2 negative breast cancer |
Joenja |
Leniolisib |
Immunology |
To treat APDS |
Litfulo |
Ritlecitinib |
Dermatology |
To treat alopecia areata |
Fabhalta |
Iptacopan |
Immunology |
To treat PNH |
Orserdu |
Elacestrant |
Cancer |
To treat breast cancer |
Ogsiveo |
Nirogacestat |
Dermatology |
To treat progressive systemic fibrosis |
Jaypirca |
Pirtobrutinib |
Central nervous sysem |
To aliment relapsed or refractory mantle cell |
Zavzpret |
Zavegepant |
Nuerology |
To treat migraines |
Velsipity |
Etrasimod |
Gastroenterology |
To aliment moderately to severely active ulcerative colitis in adults |
Augtyro |
Repotrectinib |
Cancer |
To treat ROS1-positive non-small cell lung cancer |
Table 4 List of chiral drugs approved by FDA in 2024
Medicine name |
Active chemical |
Therapeutic area |
Tryvio |
Aprocitentan |
To treat hypertension |
Rezdiffra |
Resmetirom |
To treat noncirrhotic, non-alcoholic steatohepatitis with moderate to severe liver scarring |
Tevimbra |
Tislelizumab-jsgr |
To aliment undetectable or metastatic esophageal squamous cell carcinoma |
Letybo |
letibotulinumtoxinA-wlbg |
To temporarily improve the appearance of moderate-to-severe glabellar lines |
Exblifep |
Cefepime, enmetazobactam |
To treat complicated urinary tract infections |
Zelsuvmi |
Berdazimer |
To treat molluscum contagiosum |
Lumisight |
Pegulicianine |
To treat optical imaging agent for the detection of cancerous tissue |
Zevtera |
Ceftobiprole medocaril sodium |
To treat definite bloodstream infections, bacterial skin and related to tissue infections, and community-acquired bacterial pneumonia |
Voydeya |
Danicopan |
To treat extravascular hemolysis with paroxysmal nocturnal hemoglobinuria |
Vafseo |
Vadadustat |
To treat anemia due to chronic kidney disease |
Winrevair |
Sotatercept-csrk |
To treat pulmonary arterial hypertension |
Duvyzat |
Givinostat |
To treat Duchenne muscular dystrophy in individuals aged 6 years and older |
Iqirvo |
Elafibranor |
To treat primary biliary cholangitis in combination with ursodeoxycholic acid |
Rytelo |
Imetelstat |
To treat low- to intermediate-1 risk myelodysplastic syndromes |
Imdelltra |
Tarlatamab-dlle |
To treat extensive stage small cell lung cancer |
Xolremdi |
Mavorixafor |
To treat WHIM syndrome (warts, hypogammaglobulinemia, infections and myelokathexis) |
Ojemda |
Tovorafenib |
To treat relapsed or refractory pediatric low-grade glioma |
Anktiva |
Nogapendekin alfa inbakicept-pmln |
To treat bladder cancer |
Nemluvio |
Nemolizumab-ilto |
To treat prurigo nodularis |
Yorvipath |
Palopegteriparatide |
To treat hypoparathyroidism |
Voranigo |
Vorasidenib |
To treat grade 2 astrocytoma or oligodendroglioma |
Leqselvi |
Deuruxolitinib |
To treat severe alopecia areata |
Kisunla |
Donanemab-azbt |
To treat Alzheimer's disease |
Ohtuvayre |
Ensifentrine |
To treat chronic obstructive pulmonary disease |
Piasky |
Crovalimab-akkz |
To treat paroxysmal nocturnal hemoglobinuria |
Sofdra |
Sofpironium |
To treat primary axillary hyperhidrosis |
Lazcluze |
Lazertinib |
To treat non-small cell lung cancer |
Niktimvo |
Axatilimab-csfr |
To treat chronic graft-versus-host disease (cGVHD) |
Livdelzi |
Seladelpar |
To treat primary biliary cholangitis (PBC) |
2.1 Chiral drugs and schematic route
Chiral drugs are very important in pharmaceutical drug delivery system. Here some of the drugs were developed by research groups and approved by FDA before 2022–2023. Feldman and co-workers reported that after treating A85 with the chiral substrate A86 in chloroform, the secondary product was produced. This product then underwent condensation aided by acetic acid and base-promoted Fmoc-deprotection, ultimately forming the cyclized intermediate Remimazolam A87 in three stages.14 Yamashita and co-workers mentioned, the decarboxylic reaction yields the chiral intermediate in the complete synthesis of oliceridine, which is then separated chirally by SFC.15 Chiral HPLC osilodrostat separation of the intermediate directly results in drug formation was reported by Zhang and co-workers.16 El-Kattan and co-workers explained about the synthesizing berotralstat in its pure enantiomeric form using supercritical fluid chromatography,17 using the same process as avapritinib, Ohsawa and co-workers, reported a method for the synthesis of enantiopure avapritinib is produced in a 68% yield using the chiral resolution process using supercritical fluid chromatographic separation of the intermediate.18
2.1.1 Oteseconazole (Vivjoa™). Mycovia oteseconazole (a fluorine-rich polyheterocyclic anti-fungal drug molecule) sold under the brand name Vivjoa is used for the treatment of recurrent vulvovaginal candidiasis (RVVC), an infection caused by vulvovaginal candidiasis in women with no reproductive potential in the years 2016 and 2022.19,20 Hoekstra William and co-workers reported a method for the synthesis of oteseconazole. The total synthesis of oteseconazole is carried out in 7 steps. Oteseconazole is obtained by chiral resolution. It is afforded in its pure enantiomeric form via resolution methodology by adding the racemic mixture of the fragment OTES-7 with di-p-toluoyl-L-tartaric acid (L-DPTTA) with the solvent mixture of isopropanol and acetonitrile. The yield of the overall product 91% and enantiomeric excess was 60–90% ee (Scheme 1).21
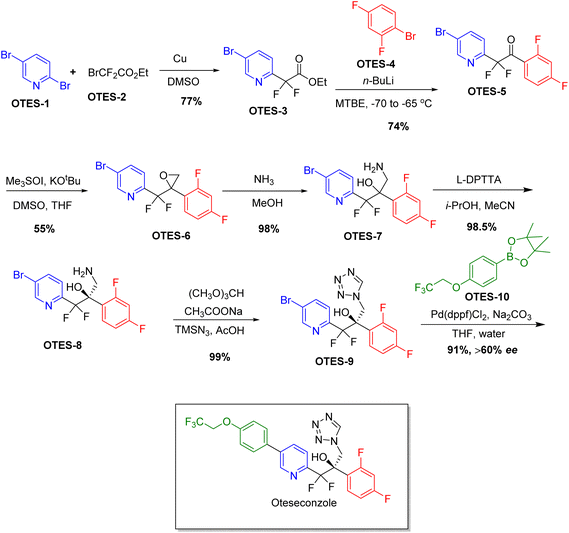 |
| Scheme 1 Synthesis of oteseconazole. | |
2.1.2 Daridorexant (Quviviq™). Daridorexant was developed by Idorsia Pharmaceuticals. It is an orexin receptor antagonist that is clinically used to treat insomnia in adult patients with difficulties of sleep concern.22,23 The total synthesis of daridorexant is performed in 5 steps, starting from the commercially available chiral substrate 2-methyl-L-proline hydrochloride DARI-1 (Scheme 2).24 The amino group in 2-methyl-L-proline was protected with Boc2O and condensed with 6-chloro-2,3-diaminotoluene DARI-3, which furnishes amide DARI-4. The amide cyclizes intramolecularly at 100 °C to provide DARI-5, which is deprotected and further condensed with 5-methoxy-2-(2H-1,2,3-triazol-2-yl) benzoic acid DARI-7 to give daridorexant (yield 63%) with enantiomeric excess of 90% ee. The chirality is installed in the molecule from the chiral proline derivative.
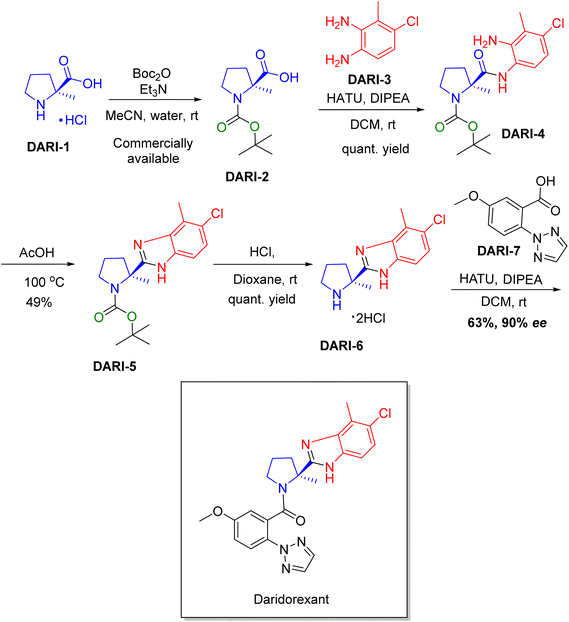 |
| Scheme 2 Synthesis of daridorexant. | |
2.1.3 Abrocitinib (Cibinqo™). Abrocitinib (containing sulfonamide moiety) is a Janus kinase inhibitor improved by Pfizer. It is used in the treatment of eczema (atopic dermatitis) in adult patients.25,26 Curtius rearrangement of 3-oxocyclobutane-1-carboxylic acid ABRO-1 with phenylmethanol ABRO-2 gives intermediate ABRO-3, which is condensed with methylamine in acetic acid to give imine. The imine is stereoselectively reduced using NaBH4 to provide the chiral fragment ABRO-4. Nucleophilic aromatic substitution of pyrimidine derivative ABRO-5 with ABRO-4 followed by dechlorination and hydrolysis gives ABRO-7, which is sulfonated using propane-1-sulfonyl chloride to give abrocitinib with yield of 74% (99% ee). The single stereogenic center in abrocitinib is induced during the reduction step (Scheme 3).27
 |
| Scheme 3 Synthesis of abrocitinib. | |
2.1.4 Futibatinib (Lytgobi™). Futibatinib (a fluorine and nitrogen aromatic heterocycle-rich drug molecule) developed by Taiho Pharmaceuticals is a permanent inhibitor of the fibroblast growth factor receptor (FGFR) used in the aid of intrahepatic cholangiocarcinoma.28 The synthesis of futibatinib can be done in 2 steps, starting from the chiral substrate FUTI-1, which undergoes Sonogashira coupling with L-ethynyl-3,5-dimethoxybenzene FUTI-2 to give the chiral fragment FUTI-3, which upon the treatment with 3-chloropropionyl chloride FUTI-4 furnishes futibatinib (75% yield, 96.24% ee). The single chiral carbon in futibatinib is obtained when the coupling reaction was conducted (Scheme 4).29
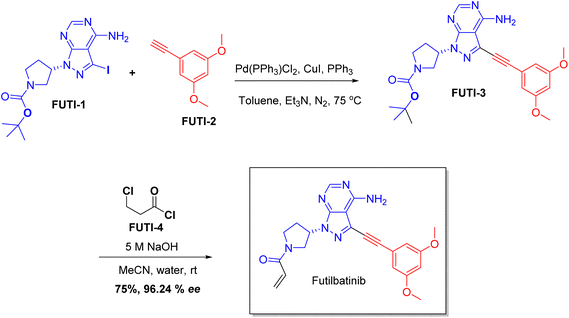 |
| Scheme 4 Synthesis of futibatinib. | |
2.1.5 Adagrasib (Krazati™). Adagrasib, developed by Mirati, is an inhibitor of KRAS that is applied for the treatment of metastatic or KRAS G12C-mutated locally modern non-small cell lung cancer in adults mostly one prior systemic.30,31 The total synthesis of adagrasib is carried out in 10 steps with a yield of 89%. There are two chiral centers in the adagrasib molecule. The first chiral center was introduced by Buchwald coupling (S)-(1-methylpyrrolidin-2-yl) methanol ADAG-4 (a proline-based chiral amino alcohol) with ADAG-3. The second chiral center was introduced by coupling the chiral fragment ADAG-11 with (S)-2-(piperazin-2-yl) acetonitrile ADAG-12 to produce an intermediate ADAG-13 with two chiral centers (Scheme 5).32,33
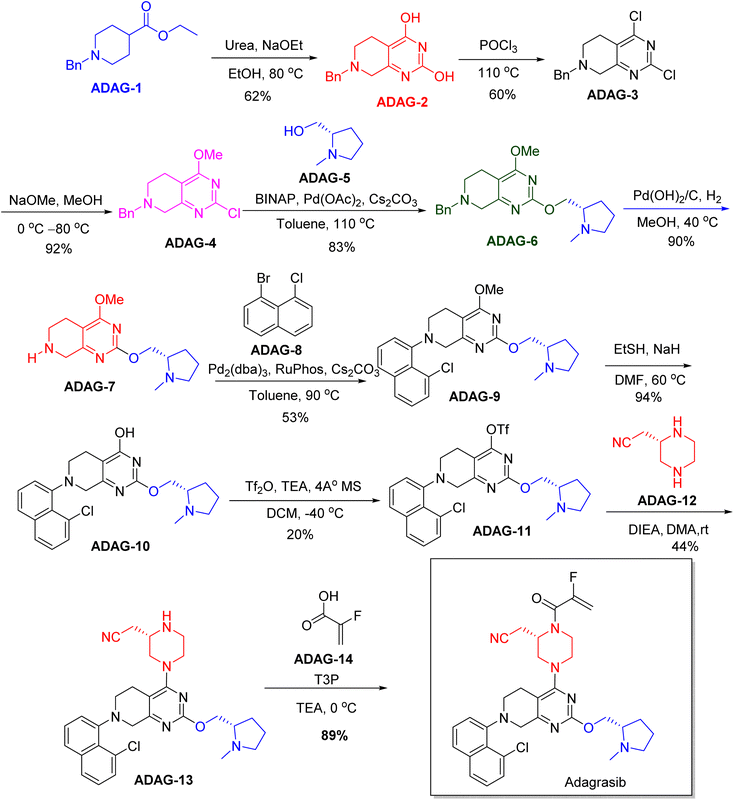 |
| Scheme 5 Synthesis of adagrasib. | |
2.1.6 Lenacapavir (Sunlenca™). Lenacapavir (a fluorine and nitrogen aromatic heterocycles-rich drug molecule) developed by Gilead Sciences is an antiretroviral for treating HIV It is a first-line drug that blocks HIV-1 capsid protein and interfering with replication of HIV-1 virus.34,35 The total synthesis of lenacapavir (yield 92%) is carried out in 12 steps chiral resolution of racemic LENA-1 intermediate with (R)-2-hydroxy-2-phenylacetic acid LENA-2 generates single enantiomer salt LENA-3. Sonogashira coupling of LENA-3 and 3-methyl-3-(methylsulfonyl) but-1-yne LENA-4 produces the chiral alkyne LENA-5, which on condensation with the carboxylicacid LENA-6 under basic conditions to provide the chiral amide intermediate LENA-7 with three stereocenter (Scheme 6).36
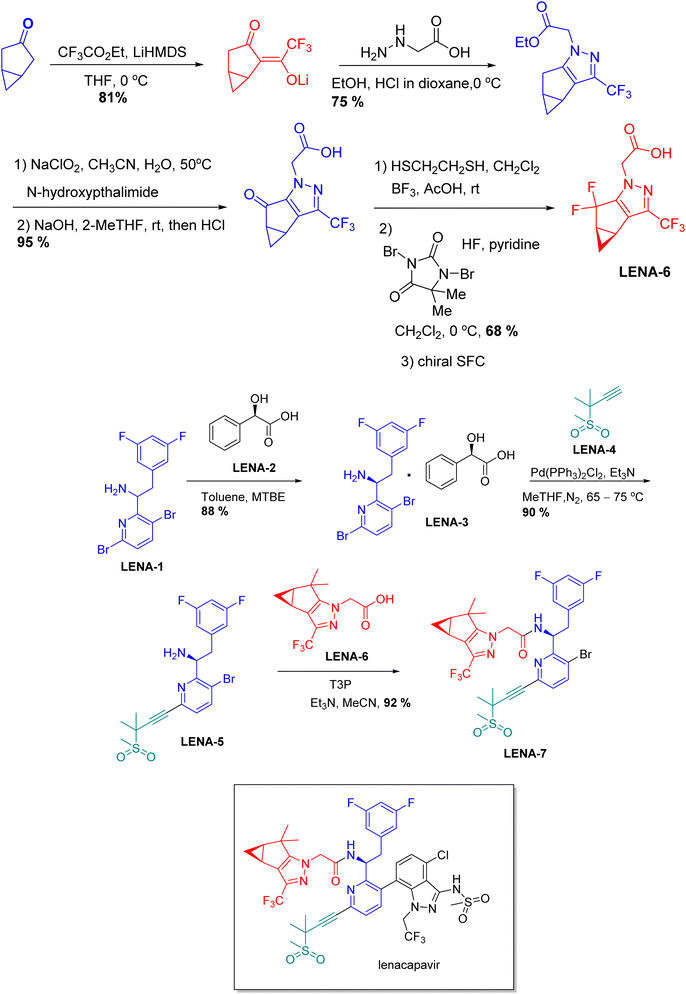 |
| Scheme 6 Synthesis of lenacapavir. | |
2.1.7 Olutasidenib (Rezlidhia™). Rigel pharmaceuticals and forma therapeutics developed olutasidenib (a fluorine and nitrogen aromatic heterocyclic drug molecule), is a potential inhibitor of isocitrate dehydrogenase-1 (IDH1). It is used in the treatment of relapsed or refractory acute myeloid leukemia (AML) in adult patients with susceptible IDH1 mutation.37,38 N-oxidation followed by acylation of 5-fluoropicolinonitrile OLUT-1 gives acetate OLUT-3, which is hydrolysed and tautomerized under basic conditions to provide pyridone OLUT-4. N-methylation with methyl iodide followed by condensation of OLUT-4 with chiral amine OLUT-6 affords olutasidenib with yield of 74% and 99% ee (Scheme 7). Chirality in olutasidenib is reproduced from the chiral fragment OLUT-6 (Scheme 7).39
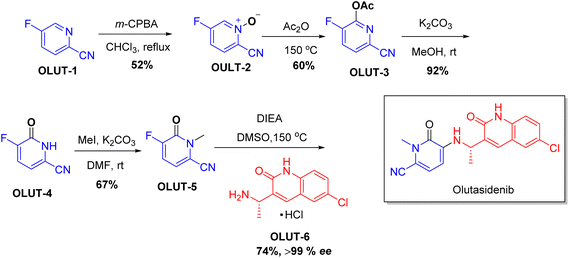 |
| Scheme 7 Synthesis of olutasidenib. | |
2.1.8 Mavacamten (Camzyos™). MyoKardia, Inc. developed mavacamten (a fluorine and nitrogen aromatic heterocycle-rich drug molecule), an effective cardiac myosin inhibitor and also used for the treatment of obstructive hypertrophic cardiomyopathy (OHCM) in adult patients.40,41 Condensation of isopropylamine MAVA-1 with trimethylcilyl isocyanate MAVA-2, followed by the cyclization with dimethyl malonate, gives MAVA-3, and MAVA-5. In the end, the coupling reaction of MAVA-5 with (S)-α-methylbenzylamine MAVA-6 in dioxane at 90 °C furnishes expected product mavacamten (yield 69%) with a stereocenter transferred from the chiral amine unit (Scheme 8).42
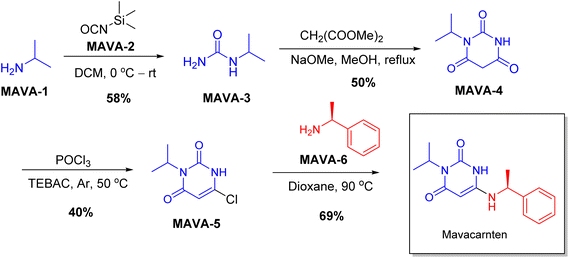 |
| Scheme 8 Synthesis of mavacamten. | |
2.1.9 Ganaxolone (Ztalmy™). Developed by Marinus, ganaxolone is a steroid molecule for the treatment of seizures in people with cyclin-dependent kinase-like 5 deficiency disorder (CDD).43,44 Pregnenolone GANA-1, a chiral starting material, on reduction followed by subsequent oxidation gives diketone GANA-3. Epoxidation of GANA-3 followed by NaI-promoted ring-opening produces ganaxolone in good yield of 90% (Scheme 9).45
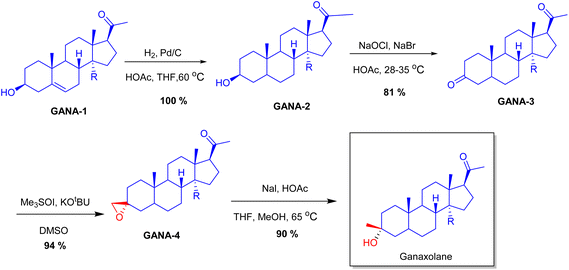 |
| Scheme 9 Synthesis of ganaxolone. | |
2.1.10 Sulbactam/durlobactam (Xacduro™). Xacduro was developed by Entasis Therapeutics, Inc. It is marketed for the treatment of bacterial pneumonia caused by the acinetobacter baumannii–calcoaceticus complex.46 Xacduro consists of sulbactam and durlobactam. Sulbactam eliminates acinetobacter baumannii, while durlobactam protects Sulbactam from the enzymes produced by acinetobacter baumannii.47 The total synthesis of sulbactam is conducted in 3 steps, starting from the commercially available chiral substrate 6-aminopenicilanic acid (6-APA) SULB-1. Sulbactam is obtained in 88% yield (Scheme 10).48
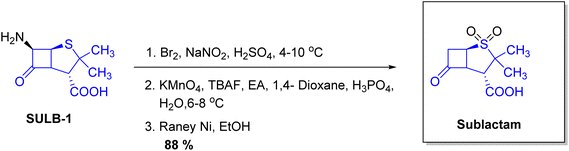 |
| Scheme 10 Synthesis of sulbactam. | |
2.1.11 Fezolinetant (Veozah™). Fezolinetant, developed by Euroscreen Srl/Ogeda SA, which is applied for the treatment of moderate to severe vasomotor symptoms (hot flashes and night sweats) caused by menopause in women.49 Fezolinetant was synthesized from chiral piperazinone FEZO-1, which is alkylated under buffered Meerwein conditions to give tetrahydropyrazine, which is further cyclized and followed by condensation with 4-fluorobenzoyl chloride FEZO-3 to give the desired molecule with yield of 97% (Scheme 11).50 The chiral center in the drug molecule was reflected from the chiral starting material.
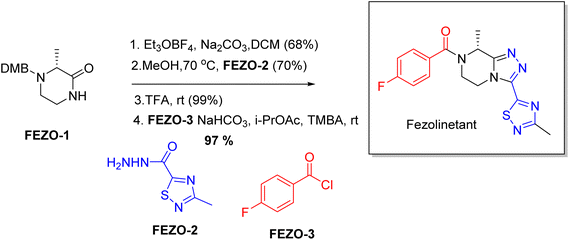 |
| Scheme 11 Synthesis of fezolinetant. | |
2.1.12 Trofinetide (Daybue™). Trofinetide, developed by the Walter Reed Army Institute of Research, a tripeptide also referred to as glycine-proline-glutamate (GPE), is used for the treatment of Rett syndrome, an X-linked neurodevelopmental method analysed by several cognitive, motor, and autonomic nervous system manifestations.51 The total synthesis of trofinetide starts with the simple, commercially available (S)-2-methylpyrrolidine-2-carboxylic acid TROF-1. TROF-1 was acylated and condensed with methanol to afford the corresponding methyl ester. The amino group in the proline was further reaction with (benzyloxy)carbonyl glycine TROF-2 and hydrolyzed under alkaline protocol afforded acid TROF-3. Condensation of TROF-3 with dibenzyl L-glutamate 4-methylbenzenesulfonate TROF-4 results in new peptide bond formation. Deprotection of benzyl group in the amide TROF-5 under palladium-carbon catalytic hydrogenation conditions gives trofinetide (yield 86%). Trofinetide has two chiral centers, one from the proline molecule and the other from the glutamate derivate (Scheme 12).52
 |
| Scheme 12 Synthesis of trofinetide. | |
2.1.13 Capivasertib (Truqap™). Astra Zeneca Pharmaceutical Co. Ltd developed capivasertib, which is used in combination with fulvestrant (Faslodex™) to treat hormone receptor (HR) positive, human epidermal growth factor receptor-2-negative (HER2) breast cancer with metastatic disease in adult patients.53 The protocol of synthesizing capivasertib is illustrated in Scheme 13.54 The chiral amino acid CAPI-1 was reduced to amino alcohol. Amino groups of the alcohol condense further with carboxylic acid CAPI-2 to obtain amide CAPI-3. Deprotection of CAPI-3 in acidic medium gives the corresponding amine. Nucleophilic aromatic substitution of 4-chloropyrimidine CAPI-4 with the chiral amine gives capivasertib with enantiomeric excess 100% ee. The stereocenter was introduced at the initial stage of synthesis by the chiral amino acid.
 |
| Scheme 13 Synthesis of capivasertib. | |
2.1.14 Leniolisib (Joenja™). Novartis Pharma AG developed leniolisib for the treatment of activated phosphoinositide 3-kinase delta syndrome (APDS), an immunodeficiency disorder that results from mutations in the gene responsible for encoding phosphotidylinsitol-3-kinase.55,56 Nucleophilic aromatic substitution of LENI-2 with chiral N-Boc-(S)-aminopyrrolidine LENI-1 and removal of benzyl protecting group under palladium hydroxide/carbon give. LENI-3, which is coupled with 5-bromo-2-methoxy-3-(trifluoromethyl) pyridine LENI-4 gives LENI-5. LENI-5 was deprotected under acidic conditions and acylated to give leniolisib (76% yield, 95% ee) (Scheme 14).57 The chirality is induced into the molecule from the chiral substrate.
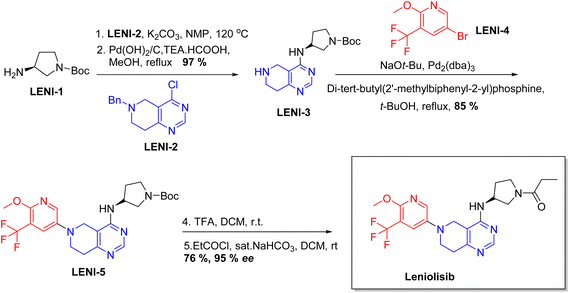 |
| Scheme 14 Synthesis of leniolisib. | |
2.1.15 Ritlecitinib (Litfulo™). Ritlecitinib, produced by Pfizer, in the treatment of alopecia areata (severe hair loss). It is a potent kinase inhibitor, acting on Janus kinase and tyrosine kinase. The following scheme shows one of the methods to synthesize ritlecitinib.58,59 Boc-protected 5-amino-2-methylpyridine RITL-2 underwent hydrogenation to produce piperidine RITL-3, whose nitrogen is shielded using CbzCl. Now the diastereoisomeric mixture of RITL-4 is subjected to Chiral SFC. Chiral SFC separates the mixture of diastereoisomers, to produce RITL-5 (rac-cis). The Boc protecting group in RITL-5 is removed under acidic conditions and further subjected to a nucleophilic aromatic substitution reaction with pyrimidine RITL-6 to produce RITL-7. The Cbz protecting group was removed from RITL-7 and reaction of amidation with acryloyl chloride. Chiral SFC is employed again to obtain the L-isomer of ritlecitinib with low yield of 17% and enantiomeric excess of 99.98% ee (Scheme 15).60
 |
| Scheme 15 Synthesis of ritlecitinib. | |
2.1.16 Iptacopan (Fabhalta™). Novartis AG of Switzerland developed iptacopan, a small-molecule complement factor B inhibitor, which is used for the treatment of paroxysmal nocturnal hemoglobinuria (PNH).61,62 The total synthesis of iptacopan is a 12-step process that begins with the reaction of 4-bromobenzonitrile IPTA-1 with 4-methoxypyridine IPTA-2. The key step in the asymmetric synthesis of iptacopan is the chiral resolution of intermediate IPTA-3 to produce chiral fragment IPTA-4, which was condensed with IPTA-5 to obtain IPTA-6. Ester hydrolysis followed by N-deprotection of IPTA-6 under alkaline conditions produces iptacopan (38% yield, 99% ee) (Scheme 16).63
 |
| Scheme 16 Synthesis of iptacopan. | |
2.1.17 Elacestrant (Orserdu™). Elacestrant created by Eisai Co., Ltd is a selective estrogen receptor degrader (SERD) that is approved for the treatment of breast cancer.64,65 The synthesis of elacestrant is a 15-step process started with demethylation of ester ELAC-1 (Scheme 17).66 Elacestrant is a drug molecule with a single chiral center with enantiomeric excess of 99% ee. The chiral center is introduced by resolution of the ester fragement ELAC-2 using a chiral chromatographic column.
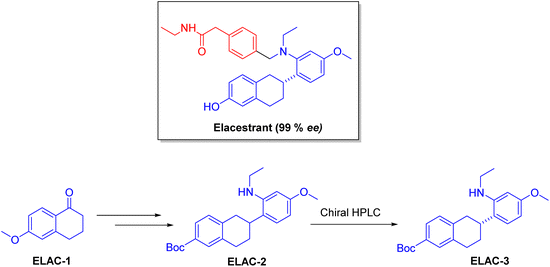 |
| Scheme 17 Synthesis of elacestrant. | |
2.1.18 Nirogacestat (Ogsiveo™). Nirogacestat (which contains a difluorophenyl moiety) was developed by Pfizer Inc. and is a selective, noncompetitive inhibitor of γ-secretase which is exploited under adult patients with progressive systemic fibrosis.67 The synthesis of nirogacestat begins with the reduction of the ester part of NIRO-1 to give aldehyde, which is condensed further with 2,2-dimethylpropan-1-amine NIRO-2 to afford amine NIRO-3. The reduction of the nitro group of NIRO-3 gave amine NIRO-4, which was subjected to condensation with chiral carboxylic acid NIRO-5 to give amide nirogacestat (Scheme 18).68 The single chiral center is introduced during the amide coupling reaction.
 |
| Scheme 18 Synthesis of nirogacestat. | |
2.1.19 Pirtobrutinib (Jaypirca™). Pirtobrutinib (which contains the CF3 group and F-phenyl moiety) developed by Eli Lilly is Bruton's tyrosine kinase (BTK) inhibitor to treat relapsed or refractory mantle cell lymphoma (MCL) in adult patients.69 In the asymmetric synthesis of pirtobrutinib, the chirality is achieved by cyclization of PIRT-1 with chiral fragment PIRT-2 in the presence of TEA to furnish pyrazole PIRT-3, which was later hydrolyzed in the presence of methanesulfonic acid (MsOH) to give pirtobrutinib in good yield 84% (Scheme 19).70
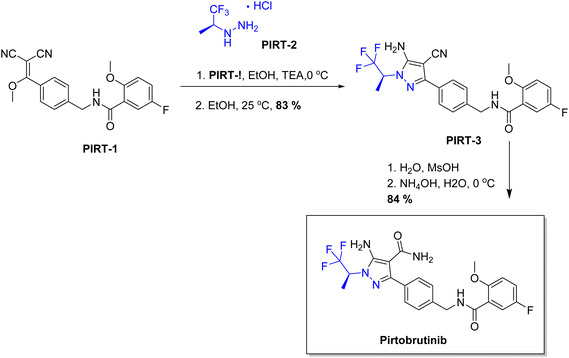 |
| Scheme 19 Synthesis of pirtobrutinib. | |
2.1.20 Lotilaner (Xdemvy™). Tarsus Pharmaceuticals, Inc. developed lotilaner in the treatment of Demodex blepharitis (inflammation of the eyelid) caused by tiny parasitic mites living in the hair follicles.71 Chiral chromatographic separation of racemic intermediate LOTI-1 gives the chiral lotilaner in a three-step process (yield 74% and 95% ee) (Scheme 20).72
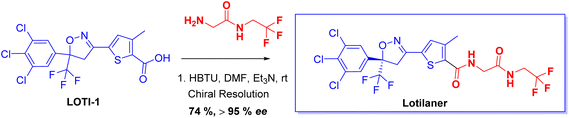 |
| Scheme 20 Synthesis of lotilaner. | |
2.1.21 Zavegepant (Zavzpret™). Bristol Myers Squibb Coworkers developed zavegepant, which is a calcitonin gene-related peptide receptor antagonist in the treatment of acute treatment of migraines.73,74 A convenient method for the synthesises of zavegepant was disclosed in 2013 which is an 8-step process. Chirality is induced during the coupling reaction of ZAVE-1 and ZAVE-2 with N,N′-succinimidyl carbonate (DSC), where ZAVE-2 is transformed into the chiral urea derivative. Zavegepant (yield 78% and 99.9% ee) is synthesized in consecutive path from the intermediate derivative, ZAVE-3 (Scheme 21)75
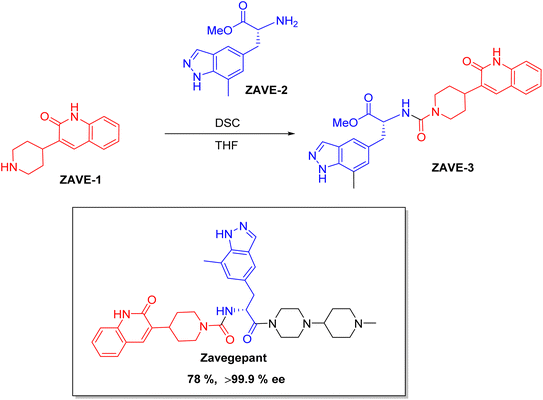 |
| Scheme 21 Synthesis of zavegepant. | |
3 Conclusion
The fundamental pillar of drug discovery and development is chirality evidenced by the fact that the majority of clinically employed drugs are chiral. It has been clear that the individual enantiomers are clinically more potent and can have considerable advantages in safety and efficacy compared to racemates. Since single enantiomer outperform racemates, this leads to the development of single isomer as drugs. The past ten years (2013–2023) have not seen a complete decline in the approval of racemic drugs. But some of the novel racemates that the FDA and/or EMA approved during this period were either analogues of well-known medications or had been marketed for several decades abroad. The undefined stereocenter that is involved in therapeutic activity is absent from all four of the remaining cases. There are no longer any novel medications on the market with unknown stereocenters that are therapeutically significant. This research highlights how crucial stereoselective synthetic procedures and characterization methods are in the context of modern pharmaceutical manufacture. However, it's important to consider the prospect of selling innovative drugs as racemates.
Asymmetric synthesis serves a way to induce chirality in the drug molecule. The technological advancement in asymmetric synthesis and analytical separation techniques encourage the synthesis of chiral drugs in a single enantiomeric form. Over the last ten years, the traditional chiral swap method has vanished. In general, this is regarded as an excellent progression because there is no proof that the patient has benefited from it. Combining chiral swapping with drug repurposing has become popular.76,77 A limitation/challenges of these FDA approve molecules: (1) chiral medicines serve a significant role in modern medicine, however getting pure enantiomers from racemic combinations might offer difficulties. (2) This significant search approach recognizes that axial chirality caused by atropisomerism has the potential to be missed, particularly, Class II atropisomers. The important Class III atropisomers are anticipated to be easily detected, whereas Class I compounds are not considered chiral. In addition to offering various benefits, this combination strategy circumvents the drawbacks of the traditional chiral switch approach. As of right now, this method has only been used to sell two pharmaceuticals. By using this strategy more extensively, it may be possible to create medicinally useful drugs more quickly.
Abbreviation
AML | Acute myeloid leukemia |
6-APA | 6-Aminopenicilanic acid |
APDS | Activated phosphoinositide 3-kinase δ syndrome |
BINAP | 2-Diphenylphosphinosnaphthyl |
Boc | t-Butyloxy carbonyl |
BTK | Bruton's tyrosine kinase |
Cbz | Benzyloxycarbony |
CDD | Cyclin-dependent kinase-like 5 deficiency disorder |
DCM | Dichloro methane |
DIBAL-H | Diisobutylaluminium hydride |
DIPEA | N,N-diisopropylethylamine |
DMA | Dimethylacetamide |
DMF | N,N-dimethyl formamide |
DPPA | Diphenylphosphoryl azide |
DSC | N,N′-succinimidyl carbonate |
FDA | U.S. Food and Drug Administration |
FGFR | Fibroblast growth factor receptor |
GPE | Glycine–proline–glutamate |
HATU | Hexafluorophosphate azabenotriazole tetramethyl uranium |
HER2 | Human epidermal growth factor receptor 2 |
HIV | Human immunodeficiency virus |
HR | Hormone receptor |
IDH1 | Isocitrate dehydrogenase-1 |
MCL | Mantle cell lymphoma |
MsOH | Methanesulfonic acid |
MTBE | Methyl t-butyl ether |
OHCM | Obstructive hypertrophic cardiomyopathy |
PI3Kδ | Phosphotidylinsitol-3-kinase δ |
TBAF | Tetrabutylammonium fluoride |
TBTU | O-(benzotriazol-1-yl)-N,N,N′,N′-tetramethyluronium tetrafluoroborate |
TEA | Triethylamine |
TEMPO | 2,2,6,6-Tetramethylperidinooxyl |
TFA | Trifluoroacetic acid |
THF | Tetrahydrofuran |
SERD | Selective estrogen receptor degrader |
Data availability
The data used to support the findings of the study are included within the article.
Author contributions
Narmatha Senkuttuvan: writing – review & editing for synthesis. Boopathi Komarasamy: writing – review & editing for synthesis. Rajavenkatesh Krishnamoorthy: writing – review for literature analysis. Shuvajyoti Sarkar: writing – review & editing for synthesis. Sivasankar Dhanasekaran: writing – review for literature analysis. A. Parthiban: supervision – conceptualization.
Conflicts of interest
The authors declare that there are no conflicts of interests.
Acknowledgements
We thank Prof. USN Murty, Director of the National Institute of Pharmaceutical Education and Research Guwahati (NIPER-G) for excellent support. We thank Dr Gayathri K, Postdoctoral fellow University of Iowa for valuable discussions.
References
- Food and Drug Administration, Development of New Stereoisomeric Drugs, Rockville, MD, 1992 Search PubMed.
- A. Calcaterra and I. D'Acquarica, J. Pharm. Biomed. Anal., 2018, 147, 323–340 CAS.
- I. Agranat, S. R. Wainschtein and E. Z. Zusman, Nat. Rev. Drug Discovery, 2012, 11, 972–973 CrossRef CAS PubMed.
- S. T. Toenjes and J. L. Gustafson, Future Med. Chem., 2018, 10, 409–422 CrossRef CAS PubMed.
- M. Ishikawa and Y. Hashimoto, J. Med. Chem., 2011, 54, 1539–1554 CrossRef CAS PubMed.
- F. Lovering, MedChemComm, 2013, 4, 515–519 RSC.
- I. P. Silvestri and P. J. J. Colbon, ACS Med. Chem. Lett., 2021, 12(8), 1220–1229 CrossRef CAS PubMed.
- R. U. McVicker and N. M. O'Boyle, J. Med. Chem., 2024, 67, 2305–2320 CrossRef CAS PubMed.
- L. A. Nguyen, H. He and C. Pham-Huy, Int. J. Biomed. Sci., 2006, 2, 85–100 CrossRef CAS PubMed.
- R. Tamatam and D. Shin, Pharmaceuticals, 2023, 16, 339 CrossRef CAS PubMed.
- New Drugs at FDA: CDER's New Molecular Entities and New Therapeutic Biological Products, U. S. Food and Drug Administration (FDA), 2023, available online: https://www.fda.gov/drugs/development-approval-processdrugs/new-drugs-fda-cders-new-molecular-entities-and-new-therapeutic-bi Search PubMed.
- J. Y. Zhang, Y. T. Wang, L. Sun, S. Q. Wang and Z. Y. Chen, Mol. Biomed., 2023, 4, 26 CrossRef PubMed.
- Y. T. Wang, P. C. Yang, Y. F. Zhang and J. F. Sun, Eur. J. Med. Chem., 2024, 265, 116124 CrossRef CAS PubMed.
- P. L. Feldman, D. K. Jung, I. Kaldor, G. J. Pacofsky, J. A. Stafford and J. H. Tidwell, WO2000069836, 2000.
- D. Yamashita, D. Gotchev, P. Pitis, X. T. Chen, G. Liu and C. C. K. Yuan, WO2012129495, 2012.
- C. Zhang and J. Chakma, WO2016109361, 2016.
- Y. El-Kattan and Y. S. Babu, US20200140389, 2020.
- I. Ohsawa, D. Honda, Y. Suzuki, T. Fukuda, K. Kohga, E. Morita, S. Moriwaki, O. Ishikawa, Y. Sasaki and M. Tago, Allergy, 2021, 76, 1789–1799 CrossRef CAS PubMed.
- S. M. Hoy, Drugs, 2022, 82, 1017–1023 CrossRef CAS PubMed.
- J. D. Sobel, Am. J. Obstet. Gynecol., 2016, 214, 15–21 CrossRef PubMed.
- W. J. Hoekstra, C. M. Yates, M. Behnke, A. Alimardanov, S. A. David, and D. F. Fry, WO2015143172A1, 2015.
- A. Markham, Drugs, 2022, 82, 601–607 CrossRef CAS PubMed.
- A. K. Morin, C. I. Jarvis and A. M. Lynch, Pharmacotherapy, 2007, 27, 89–110 CrossRef CAS PubMed.
- C. Boss, C. Brotschi, M. Gude, B. Heidmann, T. Sifferlen, and M. Von Raumer, WO2015083071, 2015.
- E. Niculet, C. Bobeica, I. A. Stefanopol, A. M. Pelin, A. Nechifor and C. Onisor, Ther. Clin. Risk Manage., 2022, 18, 399–407 CrossRef CAS PubMed.
- D. Y. Leung, M. Boguniewicz, M. D. Howell, I. Nomura and Q. A. Hamid, J. Clin. Invest., 2004, 113, 651–657 CrossRef CAS PubMed.
- X. Lu, Z. Zhong and X. Zhang, WO2021218948A1, 2021.
- Y. Y. Syed, Drugs, 2022, 82, 1737–1743 CrossRef CAS PubMed.
- M. Kondo, WO2020096042A1, 2020.
- S. Dhillon, Drugs, 2023, 83, 275–285 CrossRef CAS PubMed.
- E. O'Sullivan, A. Keogh, B. Henderson, S. P. Finn, S. G. Gray and K. Gately, Cancers, 2023, 15, 1635 CrossRef PubMed.
- J. B. Fell, J. P. Fischer, B. R. Baer, J. F. Blake, K. Bouhana and D. M. Briere, J. Med. Chem., 2020, 63, 6679–6693 CrossRef CAS PubMed.
- N. A. Margot, V. Naik, L. VanderVeen, O. Anoshchenko, R. Singh R and H. Dvory-Sobol, J. Infect. Dis., 2022, 226, 1985–1991 CrossRef CAS PubMed.
- T. Vishwanatha, N. R. Panguluri and V. V. Sureshbabu, Synthesis, 2013, 45, 1569–1601 CrossRef.
- H. Dvory-Sobol, N. Shaik, C. Callebaut and M. S. Rhee, Curr. Opin. HIV AIDS, 2022, 17, 15–21 CrossRef CAS PubMed.
- K. M. Allan, A. L. Batten, G. Brizgys, S. Dhar, I. J. Doxsee and A. Goldberg, WO2019035973A1, 2019.
- L. F. Newell and R. J. Cook, Br. Med. J., 2021, 375, n2026 CrossRef PubMed.
- Olutasidenib (Rezlidhia) for acute myeloid leukemia, Med. Lett. Drugs Ther., 2023, 65, e58–e59 Search PubMed.
- J. A. Caravella, J. Lin, R. B Diebold, A. M. Campbell, A. Ericsson and G. Gustafson, J. Med. Chem., 2020, 63, 1612–1623 CrossRef CAS PubMed.
- S. J. Keam, Drugs, 2022, 82, 1127–1135 CrossRef CAS PubMed.
- N. Lakdawala, S. Saberi, S. Day, J. Ingles, C. Semsarian, I. Olivotto, C. Ho, J. Fine, Y. Xu, M. Sutton, J. Xie and Y. Wang, J. Card. Failure, 2022, 28, S37–S38 CrossRef.
- J. Oslob, R. Anderson, D. Aubele, M. Evanchik, J. C. Fox and B. Kane, WO2014205223A1, 2014.
- V. Nohria and E. Giller, Ganaxolone, Neurotherapeutics, 2007, 4, 102–105 CrossRef CAS PubMed.
- H. E. Olson, S. T. Demarest, E. M. Pestana-Knight, L. C. Swanson, S. Iqbal and D. Lal, Pediatr. Neurol., 2019, 97, 18–25 CrossRef PubMed.
- D. S. Reddy, WO2019209850A1, 2019.
- S. J. Keam, Drugs, 2023, 83, 1245–1252 CrossRef CAS PubMed.
- A. El-Ghali, A. J. Kunz Coyne, K. Caniff, C. Bleick and M. J. Rybak, Pharmacotherapy, 2023, 43, 502–513 CrossRef CAS PubMed.
- Z. M. Song, W. Liu, J. Yang and Y. Sun, Chin. J. Med. Chem., 2004, 14, 180–181 CAS.
- A. Lee, Drugs, 2023, 83, 1137–1141 CrossRef CAS PubMed.
- H. R. Hoveyda, G. L. Fraser, G. Dutheuil, M. El Bousmaqui, J. Korac, F. Lenoir, A. Lapin and S. Noel, ACS Med. Chem. Lett., 2015, 6, 736–740 CrossRef CAS PubMed.
- S. J. Keam, Drugs, 2023, 83, 819–824 CrossRef PubMed.
- D. Gluckman, G. B. Thomas, J. Guan, M. Dragunow, A. K. Anand, N. K. De Rosbo, F. Sieg and M. A. Brimble, US20070298009, 2007.
- B. R. Davies, H. Greenwood, P. Dudley, C. Crafter, D. H. Yu, J. Zhang, J. Li, B. Gao, Q. Ji, J. Maynard, S. A. Ricketts, D. Cross, S. Cosulich, C. C. Chresta, K. Page, J. Yates, C. Lane, R. Watson, R. Luke, D. Ogilvie and M. Pass, Mol. Cancer Ther., 2012, 11, 873–887 CrossRef CAS PubMed.
- P. D. Gluckman, G. B. Thomas, J. Guan, M. Dragunow, A. K. Anand, N. K. De Rosbo, F. Sieg and M. A. Brimble, US20070298009, 2007.
- A. Parthiban and P. Makam, RSC Adv., 2022, 12, 29253–29290 RSC.
- S. J. Woodhead, M. Frederickson, C. Hamlett, A. J. Woodhead, M. L. Verdonk, H. F. Sore, D. W. Walker, P. Blurton, I. Collins, K. M. Cheung, J. Caldwell, T. F. Da Fonseca McHardy, R. W. A. Luke, Z. S. Matusiak, A. Leach and J. J. Morris, US20100093748A1, 2010.
- S. Duggan and Z. T. Al-Salama, Drugs, 2023, 83, 943–948 CrossRef CAS PubMed.
- V. K. Rao, S. Webster, V. Dalm, A. Šediva, P. M. van Hagen, S. Holland, S. D. Rosenzweig, A. D. Christ, B. Sloth, M. Cabanski, A. D. Joshi, S. de Buck, J. Doucet, D. Guerini, C. Kalis, I. Pylvaenaeinen, N. Soldermann, A. Kashyap, G. Uzel, M. J. Lenardo, D. D. Patel, C. L. Lucas and C. Burkhart, Blood, 2017, 130, 2307–2316 CrossRef CAS PubMed.
- K. Hoegenauer, N. Soldermann, F. Zecri, R. S. Strang, N. Graveleau, R. M. Wolf, N. G. Cooke, A. B. Smith, G. J. Hollingworth, J. Blanz, S. Gutmann, G. Rummel, A. Littlewood-Evans and C. Burkhart, ACS Med. Chem. Lett., 2017, 8, 975–980 CrossRef CAS PubMed.
- H. A. Blair, Drugs, 2023, 83, 1315–1321 CrossRef PubMed.
- J. B. Telliez, M. Dowty, L. Wang, J. Jussif, T. Lin, L. Li, E. Moy, P. Balbo, W. Li, Y. Zhao, K. Crouse, C. Dickinson, P. Symanowicz, M. Hegen, M. E. Banker, F. Vincent, R. Unwalla, S. Liang, A. M. Gilbert, M. F. Brown, M. Hayward, J. Montgomery, X. Yang, J. Bauman, J. I. Trujillo, A. Casimiro-Garcia, F. F. Vajdos, L. Leung, K. F. Geoghegan, A. Quazi, D. Xuan, L. Jones, E. Hett, K. Wright, J. D. Clark and A. Thorarensen, ACS Chem. Biol., 2016, 11, 3442–3451 CrossRef CAS PubMed.
- A. Thorarensen, M. E. Dowty, M. E. Banker, B. Juba, J. Jussif, T. Lin, F. Vincent, R. M. Czerwinski, A. Casimiro-Garcia, R. Unwalla, J. I. Trujillo, S. Liang, P. Balbo, Y. Che, A. M. Gilbert, M. F. Brown, M. Hayward, J. Montgomery, L. Leung, X. Yang, S. Soucy, M. Hegen, J. Coe, J. Langille, F. Vajdos, J. Chrencik and J. B. Telliez, J. Med. Chem., 2017, 60, 1971–1993 CrossRef CAS PubMed.
- A. D. James, K. Kulmatycki, B. Poller, A. A. Romeo, J. J. Van Lier, K. Klein and D. Pearson, Drug Metab. Dispos., 2023, 51, 873–883 CrossRef CAS PubMed.
- D. V. Rizk, B. H. Rovin, H. Zhang, N. Kashihara, B. Maes, H. Trimarchi, V. Perkovic, M. Meier, D. Kollins, O. Papachristofi, A. Charney and J. Barratt, Kidney Int. Rep., 2023, 8, 968–979 CrossRef PubMed.
- N. Mainolfi, T. Ehara, R. G. Karki, K. Anderson, A. Mac Sweeney, S. M. Liao, U. A. Argikar, K. Jendza, C. Zhang, J. Powers, D. W. Klosowski, M. Crowley, T. Kawanami, J. Ding, M. April, C. Forster, M. Serrano-Wu, M. Capparelli, R. Ramqaj, C. Solovay, F. Cumin, T. M. Smith, L. Ferrara, W. Lee, D. Long, M. Prentiss, A. De Erkenez, L. Yang, F. Liu, H. Sellner, F. Sirockin, E. Valeur, P. Erbel, D. Ostermeier, P. Ramage, B. Gerhartz, A. Schubart, S. Flohr, N. Gradoux, R. Feifel, B. Vogg, C. Wiesmann, J. Maibaum, J. Eder, R. Sedrani, R. A. Harrison, M. Mogi, B. D. Jaffee and C. M. Adams, J. Med. Chem., 2020, 63, 5697–5722 CrossRef CAS PubMed.
- S. M. Hoy, Drugs, 2023, 83, 555–561 CrossRef CAS PubMed.
- S. Hamaoka, N. Kitazawa, K. Nara, A. Sasaki, A. Kamada and T. Okabe, US20120004315A1, 2012.
- P. Wei, M. Walls, M. Qiu, R. Ding, R. H. Denlinger, A. Wong, K. Tsaparikos, J. P. Jani, N. Hosea, M. Sands, S. Randolph and T. Smeal, Mol. Cancer Ther., 2010, 9, 1618–1628 CrossRef CAS PubMed.
- M. A. Brodney, D. D. Auperin, S. L. Becker, B. S. Bronk, T. M. Brown, K. J. Coffman, J. E. Finley, C. D. Hicks, M. J. Karmilowicz, T. A. Lanz, D. Liston, X. Liu, B. A. Martin, R. B. Nelson, C. E. Nolan, C. E. Oborski, C. P. Parker, K. E. Richter, N. Pozdnyakov, B. G. Sahagan, J. B. Schachter, S. A. Sokolowski, B. Tate, D. E. Wood, K. M. Wood, J. W. Van Deusen and L. Zhang, Bioorg. Med. Chem. Lett., 2011, 21, 2637–2640 CrossRef CAS PubMed.
- E. B. Gomez, M. S. Rosendahal, S. M. Rothenberg, S. W. Andrews and B. J. Brandhuber, Blood, 2019, 134, 4644 CrossRef.
- J. L. Jensen, A. R. Mato, C. Pena, L. E. Roeker and C. C. Coombs, Ther. Adv. Hematol., 2022, 13, 20406207221101697 CrossRef CAS PubMed.
- A. D. A. Jose, E. Charles, F. Jared, F. Scott and M. Nicholas, WO2022056100A1, 2022.
- S. Dhillon, Drugs, 2023, 83, 825–831 CrossRef PubMed.
- D. Moreno-Ajona, A. Perez-Rodríguez and P. J. Goadsby, Gepants, Curr. Opin. Neurol., 2020, 33, 309–315 CrossRef CAS PubMed.
- P. V. Chaturvedula, S. E. Mercer, S. S. Pin, G. Thalody, C. Xu, C. M. Conway, D. Keavy, L. Signor, G. H. Cantor, N. Mathias, P. Moench, R. Denton, R. Macci, R. Schartman, V. Whiterock, C. Davis, J. E. Macor and G. M. Dubowchik, Bioorg. Med. Chem. Lett., 2013, 23, 3157–3161 CrossRef CAS PubMed.
- I. D'Acquarica and I. Agranat, ACS Pharmacol. Transl. Sci., 2023, 6, 201–219 CrossRef PubMed.
- S. Ali and J. Zhou, Eur. J. Med. Chem., 2023, 256, 115476 CrossRef CAS PubMed.
Footnote |
† Equal contributions. |
|
This journal is © The Royal Society of Chemistry 2024 |
Click here to see how this site uses Cookies. View our privacy policy here.