DOI:
10.1039/D4RA05772D
(Paper)
RSC Adv., 2024,
14, 32350-32357
Green chemistry approach to the synthesis of 2-aryl/heteroaryl substituted 2,3-dihydroquinazolin-4(1H)-ones using lemon juice under concentrated solar radiations as a renewable source†
Received
9th August 2024
, Accepted 24th September 2024
First published on 14th October 2024
Abstract
This study explores a novel and eco-friendly synthesis of 22 derivatives of 2-aryl/heteroaryl substituted 2,3-dihydroquinazolin-4(1H)-ones, compounds with significant medicinal potential, using concentrated solar radiation (CSR) and lemon juice as a natural catalyst. Traditional methods for synthesizing these compounds often involve complex, energy-intensive processes and toxic reagents. In contrast, the method presented here utilizes solar energy and a biodegradable, non-toxic catalyst, aligning with the principles of green chemistry. The reaction, involving 2-aminobenzamide and various aromatic and heteroaromatic aldehydes, was optimized by varying temperature, catalyst concentration, and solvent. Through optimization, a combination of 0.3 mL of lemon juice and CSR achieved a 97% product yield in 10 minutes. A wide range of aromatic and heteroaromatic aldehydes were tested, all of which produced excellent yields, confirming the method's broad applicability. The substrate scope was explored with different aldehydes, containing groups/structures like chloro, bromo, nitro, methyl, methoxy, fluoro, hydroxy, imidazole, thiazole, chromone, pyrrole, and 1,4-dioxane, yielding up to 97%. Comparative studies with other catalysts and solvents confirmed the superior efficiency of lemon juice. This study not only demonstrates a sustainable approach to synthesizing 2,3-dihydroquinazolin-4(1H)-ones but also highlights the potential of solar energy in organic synthesis, offering a viable alternative to conventional methods. This environmentally benign method offers an efficient and sustainable route for synthesizing 2,3-dihydroquinazolin-4(1H)-one derivatives.
1 Introduction
The twelve green chemistry principles were first disclosed by Paul T. Anastas and John Warner,1 and since then, innumerable scientific disclosures and discoveries have ascended through “Green Chemistry”. The twelve green chemistry principles have served as an excellent springboard for the advancement of new green chemistry research.2 Green chemistry has a significant impact on chemical synthesis.3–6 Green solvents,7,8 sustainable catalysts,9,10 and microwave and ultrasonic technologies have all changed the face of organic synthesis. In recent years, eco-friendly techniques for the synthesis of heterocyclic compounds of medicinal value have been considered in order to optimise reaction conditions.11–13
The synthesis of bioactive compounds is necessary for facilitating the development of new drugs, especially when looking for therapeutic treatments. 2,3-dihydroquinazolin-4-one (DHQ), a nitrogen-containing heterocyclic compound, is a major structural component of a number of biologically active compounds. Because of the prevalence of the quinazoline structure as a building block in a variety of natural products and synthetic compounds, researchers have focused their efforts on the synthesis of quinazolines due to their diverse biological and therapeutic activity. Antimicrobial,14,15 antineoplastic,16 anti-inflammatory,17 anti-cancer,18 and anticonvulsive19 characteristics are among the potential pharmacological profiles of DHQ-containing heterocyclic compounds. It has been demonstrated that adding 2-aryl/heteroaryl to these compounds improves their pharmacological characteristics, making them attractive targets for future therapeutic development.
Throughout the last few decades, several approaches for the synthesis of the DHQ framework, particularly the 2-aryl/heteroaryl substituted derivatives, have been established. Nevertheless, multistep synthesis, harsh reaction conditions, and the use of toxic chemicals and solvents have limited their promise as a flexible component. Green chemistry and alternative methodologies have lately been researched for the synthesis of several DHQ derivatives. There is a considerable amount of research on the synthesis of DHQ derivatives utilising various techniques. Some previous synthetic strategies include CoFe2O4@SiO2-CPTES-guanidine-Cu(II),20 Cp2TiCl2,21 CuCl2/Fe3O4-TEDETA,22 boehmite silica dopamine sulfamic acid,23 palladium S-methylisothiourea,24 SbCl3,25 L-proline@Fe3O4,26 succinimide-N-sulfonic acid,27 and many others.28–31
The various reported strategies include lengthy catalyst synthesis procedures, complex structures of the catalysts, operational complexity, long reaction run, use of non-renewable energy sources, etc. This demands the development of highly efficient, green and use of clean energy source for the synthesis of DHQ derivatives. The solar energy is largely available non-renewable clean energy source and lemon juice is one of the best natural biocatalyst. The increased use of lemon juice in organic synthesis is mostly due to its biodegradability, nontoxicity, water-solubility, clean reaction profile, ready availability, and low cost.
Under concentrated solar radiation (CSR), we present a feasible and efficient synthesis of 2-aryl/heteroaryl substituted 2,3-dihydroquinazolin-4(1H)-ones from 2-aminobenzamide and aromatic/heteroaromatic aldehydes via lemon juice. Some previously used catalytic systems32–36 are depicted in Scheme 1. The use of synthetic catalysts for the synthesis of 2,3-dihydroquinazolin-4(1H)-ones is not completely sustainable and renewable. Instead, providing renewable natural source could lead to the sustainable way. Here, we are delighted to report that CSR, an entirely free, safe, and natural energy as a potential source to carry out the synthesis of 2,3-dihydroquinazolin-4(1H)-ones. In this study, we report the synthesis of 2-aryl/heteroaryl substituted 2,3-dihydroquinazolin-4(1H)-one derivatives using concentrated solar radiation. The application of solar energy in this context not only offers a green alternative to conventional heating methods but also demonstrates the feasibility of using solar technology in organic synthesis.
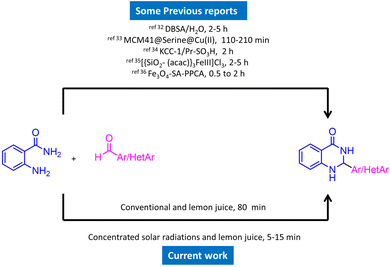 |
| Scheme 1 Greener approach for the synthesis of 2,3-dihydroquinazolin-4(1H)-ones. | |
2 Results and discussion
In this study, we used a combination of source of clean energy; CSR, and lemon juice to synthesize 2-aryl/heteroaryl substituted 2,3-dihydroquinazolin-4(1H)-ones from 2-aminobenzamide and aromatic/heteroaromatic aldehydes. Previously CSR was used for the synthesis of Biginelli adducts.37 Waste prevention, less hazardous route of synthesis, utilisation of renewable feedstock, catalysis, and energy efficiency are all advantages of this unique synthetic methodology. To investigate the optimum reaction parameters, the reaction between 2-aminobenzamide and 4-chlorobenzaldehyde was chosen as a model reaction. We investigated model reaction at room temperature, 60–70 °C and CSR in presence and absence of lemon juice catalyst (Table 1). First, we performed the reaction at room temperature with stirring; but we did not achieve product formation even after 2 hours. Following that, we opted to run the reaction at 60–70 °C. Notwithstanding, even this could provide the necessary output in a low yield. The lemon juice was then used as a natural catalyst. Two parallel reactions were carried out under stirring conditions in the presence of lemon juice (0.1 mL), one at room temperature and the other at 60–70 °C. The latter reaction resulted in an encouraging outcome, yielding 68% of the target product. This suggested that the reaction is being facilitated by lemon juice. Following that, we replicated the reaction under CSR using a Fresnel lens as a solar radiation concentrator. Unfortunately, it did not accomplish the intended result. Then we attempted the reaction by combining the CSR and lemon juice (0.1 mL), and to our credit, we obtained a 72% yield of the product in 20 minutes. We next varied the lemon juice concentration to observe the impact of lemon juice on the reaction time and product yield. The amount of lemon juice used ranged from 0.1 mL to 0.4 mL. We found that as the amount of lemon juice increased, so did the yield of the product, and this with a drop in reaction time. We acquired 97% yield in 10 minutes by adopting 0.3 mL lemon juice as the optimal quantity. There was no difference in time or yield when the lemon juice quantity was increased from 0.3 mL to 0.4 mL. We also investigated the two reactions, one at room temperature and one at 60–70 °C with an optimised amount of lemon juice. The first did not produce any product, while the latter could provide a 90% yield in 70 minutes. We also experimented with different solvents to identify the reaction favourable solvent. DMSO, DMF, THF, PEG-200, PEG-400, ethylene glycol, water, and ethanol were among the polar aprotic and protic solvents that were investigated (Table 2). Polar aprotic solvents DMSO, DMF, and THF yielded 15–30% product yield, whereas polar protic solvent yielded a greater yield (60–97%). The reaction failed to produce the product in the presence of water. We concluded that ethanol produces the highest yield and requires the less time to complete the reaction.
Table 1 Optimization of amount of lemon juice and CSRa
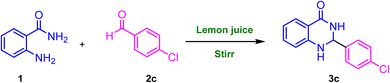
|
Entry |
Lemon juice (mL) |
Energy/temperature |
Reaction time (min) |
Yieldb (%) |
The reaction was performed on 10 mmol scale, ethanol-10 mL. Yield of isolated pure product. RT – room temperature, nr – no reaction, CSR – concentrated solar radiations. |
1 |
0 |
RT |
120 |
nr |
2 |
0 |
60–70 °C |
120 |
10 |
3 |
0.1 |
RT |
120 |
nr |
4 |
0.1 |
60–70 °C |
80 |
68 |
5 |
0 |
CSR |
30 |
nr |
6 |
0.1 |
CSR |
20 |
72 |
7 |
0.2 |
CSR |
15 |
85 |
8 |
0.3 |
CSR |
10 |
97 |
9 |
0.4 |
CSR |
10 |
97 |
10 |
0.3 |
RT |
120 |
nr |
11 |
0.3 |
60–70 °C |
80 |
90 |
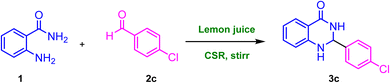
|
Entry |
Solvent |
Reaction time (min) |
Yieldb (%) |
The reaction was performed on 10 mmol scale under CSR, solvent-10 mL. Yield of isolated pure product. |
1 |
DMSO |
30 |
30 |
2 |
DMF |
30 |
30 |
3 |
THF |
30 |
15 |
4 |
PEG-200 |
25 |
65 |
5 |
PEG-400 |
25 |
60 |
6 |
Ethylene glycol |
20 |
75 |
7 |
Water |
20 |
nr |
8 |
Ethanol |
10 |
97 |
We also looked into our previously synthesised nanocatalysts as well as Brønsted acid catalysts and nanomaterials (Table 3). All these catalysts were used in the presence of CSR. Initially, we conducted the reaction in the presence of sulfamic acid (10 mg and 20 mg). The reaction yielded less than 80% in both cases after 20 minutes. The pTSA (10 mg) provided a product yield of 65% within 30 minutes, our previously synthesised catalysts, 5% Fe–ZnO, La2O3, and ZnO–CuO, produced 25–40% yield. Inorganic acid (aq. 1
:
1 HCl) yielded 45%, while acetic acid yielded 65% product. We obtained 15% yield in the presence of chitosan nanopowder. These findings revealed that lemon juice, as a natural catalyst, provides a higher yield in short period of time. To validate the synthetic protocol, we tested various aromatic and heteroaromatic aldehydes under optimized reaction conditions (Table 4). The aromatic aldehydes comprising chloro, bromo, nitro, methyl, methoxy, fluoro, and hydroxy groups were used. All these aromatic aldehydes with diverse substitution nature offered excellent yields. The heteroaromatic aldehydes containing imidazole, thiazole, chromone, pyrrole and 1,4-dioxane moieties were also gave yield up to 96%. The substrate scope validity affirms the practical viability of the novel route designed for the synthesis of diverse range of 2,3-dihydroquinazolin-4(1H)-one derivatives.
Table 3 Comparative study with other catalystsa
Table 4 Substrate scope for the synthesis of 2,3-dihydroquinazolin-4(1H)-one derivatives and physicochemical data
The probable reaction mechanism44 (Scheme 2) begins with the nucleophilic attack of the amine group from 2-aminobenzamide on the carbonyl carbon of a protonated aldehyde (I), facilitated by lemon juice acting as an acid catalyst to enhance the electrophilicity of the aldehyde. This forms an iminium ion intermediate (II), which then undergoes intramolecular cyclization via the amide nitrogen attacking the iminium carbon, resulting in a bicyclic intermediate (III). Deprotonation of this intermediate leads to the formation of the final 2,3-dihydroquinazolin-4(1H)-one product. Lemon juice promotes the reaction through acid catalysis, while CSR supplies energy required for efficient product formation in a short reaction time.
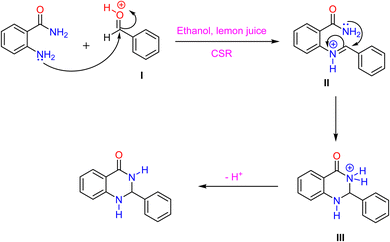 |
| Scheme 2 Plausible reaction mechanism for the synthesis of 2,3-dihydroquinazolin-4(1H)-ones using lemon juice under concentrated solar radiations as a renewable source. | |
The green chemistry metrics45,46 provided in Fig. 1 demonstrated that the formation of the compound 3c along with all other compounds is highly efficient and environmentally friendly. The high yield (97%) and atom economy (93.49%) suggest that the majority of the starting materials are utilized in the final product with minimal waste. This is further supported by the low E-factor (0.1), indicating very little waste generation. The reaction mass efficiency (89.26%) confirms that the process uses reactants effectively, and the high Ecoscale score (85.5) underscores the overall greenness of the process. Together, these metrics align with the principles of green chemistry, promoting sustainability, waste reduction, and efficient use of resources.
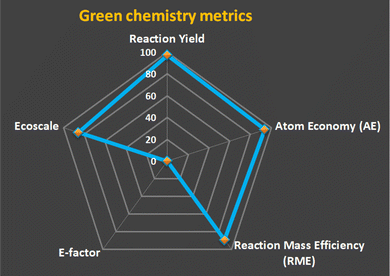 |
| Fig. 1 Green chemistry metrics for the synthesis of compound 3c. | |
3 Experimental
3.1. General remarks
All chemical reagents in high purity were purchased (Make: Sigma-Aldrich, Merck, SD fine chemicals and Avra synthesis) from local vendor and used without any further purification. The FT-IR, 1H NMR and 13C NMR spectral analyses were performed on a Bruker NMR spectrophotometer using CDCl3 and DMSO-d6 as solvents. The FT-IR spectroscopic analysis was recorded on Bruker FT-IR spectrophotometer with KBr pellets. Melting points were determined in open capillaries and uncorrected. All the glass apparatus were dried prior to use.
3.2. Preparation of catalyst
We took fresh lemons, washed them thoroughly with water, and cut them using a knife. The pieces were then pressed manually to extract the juice. The juice was filtered to remove any solid material, resulting in clear juice, which was used as a catalyst.
3.3. Synthesis of 2-aryl/heteroaryl substituted 2,3-dihydroquinazolin-4(1H)-ones
A mixture of anthranilamide (1, 10 mmol) and aryl/heteroaryl aldehyde (2a–2v, 10 mmol) and lemon juice (0.3 mL) were mixed in a 50 mL conical flask (fitted with condenser) containing ethanol as a solvent. This mixture was kept on magnetic stirrer and was exposed CSR for an appropriate time (monitored by TLC). The mixture is then cooled to room temperature and to this ethanol was added. The reaction mixture was then filtered to get pure products (3a–3v) (Scheme 3). The structures of representative products were confirmed by using FT-IR, 1H NMR and 13C NMR spectral data.
 |
| Scheme 3 Synthesis of 2-aryl/heteroaryl substituted quinozolin-4(3H)-ones. | |
3.4. Representative spectral results
3.4.1. 2-Phenyl-2,3-dihydroquinazolin-4(1H)-one. FT-IR (cm−1): 3298.88, 3168.51, 3058.15, 1652.02, 1608.01, 1505.01, 1477.18, 1440.25, 1383.18, 1325.59, 1299.44, 1245.78, 1155.34, 1026.57, 914.83, 857.45, 806.22, 743.81, 693.15, 662.45, 597.67, 564.45; 1H NMR (500 MHz, DMSO-d6) δ: 8.28 (t, J = 1.7 Hz, 1H), 7.61 (dd, J = 7.7, 1.6 Hz, 1H), 7.52–7.46 (m, 2H), 7.41–7.33 (m, 3H), 7.27–7.20 (m, 1H), 7.10 (s, 1H), 6.75 (dd, J = 8.2, 1.0 Hz, 1H), 6.71–6.64 (m, 1H), 5.75 (t, J = 1.7 Hz, 1H); 13C NMR (126 MHz, DMSO-d6) δ: 164.06, 148.34, 142.10, 133.78, 128.92, 128.80, 127.82, 127.33, 117.58, 115.42, 114.87, 67.03.
3.4.2. 2-(2-Butyl-5-chloro-1H-imidazol-4-yl)-2,3-dihydroquinazolin-4(1H)-one. FT-IR (cm−1): 3275.43, 3182.52, 3059.37, 2932.11, 1648.85, 1610.32, 1514.65, 1482.01, 1433.48, 1375.76, 1326.46, 1295.80, 1231.87, 1156.67, 1129.78, 1088.26, 1028.88, 898.67, 858.89, 802.36, 747.37, 700.38, 657.66, 601.79, 576.71; 1H NMR (500 MHz, DMSO-d6) δ: 12.54 (s, 1H), 8.14 (d, J = 1.7 Hz, 1H), 7.65 (dd, J = 7.7, 1.6 Hz, 1H), 7.28 (ddd, J = 8.1, 7.2, 1.6 Hz, 1H), 6.95 (d, J = 1.6 Hz, 1H), 6.78–6.70 (m, 2H), 5.83 (s, 1H), 2.57 (t, J = 7.5 Hz, 2H), 1.60 (quin, J = 7.5 Hz, 2H), 1.31 (sext, J = 7.5 Hz, 2H), 0.89 (t, J = 7.5 Hz, 3H); 13C NMR (126 MHz, DMSO-d6) δ: 164.34, 148.70, 148.46, 133.74, 127.94, 126.19, 122.32, 118.21, 115.50, 114.98, 59.49, 30.45, 27.99, 22.07, 14.13.
3.4.3. 2-(1-Methyl-1H-pyrrol-2-yl)-2,3-dihydroquinazolin-4(1H)-one. FT-IR (cm−1): 3388.61, 3173.70, 3124.59, 3002.97, 2907.24, 2108.31, 1637.14, 1607.09, 1488.31, 1372.03, 1295.18, 1240.26, 1156.89, 1093.31, 1022.02, 984.98, 948.40, 858.10, 814.11, 767.84, 728.14, 603.62, 542.33; 1H NMR (500 MHz, DMSO-d6) δ: 8.14 (t, J = 1.7 Hz, 1H), 7.63 (dd, J = 7.8, 1.6 Hz, 1H), 7.24 (ddd, J = 8.1, 7.2, 1.6 Hz, 1H), 6.92 (d, J = 1.8 Hz, 1H), 6.77 (dd, J = 8.2, 1.1 Hz, 1H), 6.74 (dd, J = 2.7, 1.9 Hz, 1H), 6.70 (ddd, J = 8.0, 7.2, 1.1 Hz, 1H), 6.06 (dd, J = 3.6, 1.9 Hz, 1H), 5.91 (dd, J = 3.6, 2.7 Hz, 1H), 5.87 (t, J = 1.5 Hz, 1H), 3.70 (s, 3H); 13C NMR (126 MHz, DMSO-d6) δ: 164.43, 148.67, 133.57, 130.64, 127.84, 124.37, 117.75, 115.70, 115.08, 109.31, 106.46, 61.22, 34.80.
3.4.4. 2-(2,3-Dihydrobenzo[b][1,4]dioxin-6-yl)-2,3-dihydroquinazolin-4(1H)-one. FT-IR (cm−1): 3291.13, 3183.76, 3023.70, 2925.35, 1644.47, 1611.19, 1500.94, 1458.36, 1397.38, 1285.95, 1256.95, 1154.57, 1124.00, 1065.59, 913.70, 876.03, 761.55, 679.57, 645.64, 594.75, 555.71, 525.16; 1H NMR (500 MHz, DMSO-d6) δ 8.30–8.19 (m, 1H), 7.59 (dd, J = 7.8, 1.6 Hz, 1H), 7.23 (ddd, J = 8.1, 7.2, 1.7 Hz, 1H), 7.04 (d, J = 1.8 Hz, 1H), 6.98–6.90 (m, 2H), 6.85 (d, J = 8.3 Hz, 1H), 6.73 (dd, J = 8.2, 1.1 Hz, 1H), 6.69–6.63 (m, 1H), 5.63 (t, J = 2.0 Hz, 1H), 4.21 (s, 4H); 13C NMR (126 MHz, DMSO-d6) δ 164.03, 148.27, 143.90, 143.47, 135.32, 133.74, 127.79, 120.07, 117.52, 117.26, 116.05, 115.43, 114.87, 66.33, 64.55.
3.4.5. 2-(4-Methylthiazol-5-yl)-2,3-dihydroquinazolin-4(1H)-one. FT-IR (cm−1): 3291.98, 3181.92, 1652.76, 1611.68, 1558.01, 1508.26, 1482.35, 1439.42, 1407.17, 1374.01, 1330.96, 1287.06, 1160.93, 1128.89, 1028.63, 995.66, 944.22, 855.56, 808.94, 746.88, 689.10, 597.71, 534.07;1H NMR (500 MHz, DMSO-d6) δ 8.91 (s, 1H), 8.33 (s, 1H), 7.68–7.62 (m, 1H), 7.32–7.25 (m, 1H), 7.13 (s, 1H), 6.79–6.72 (m, 2H), 6.18 (s, 1H), 2.49 (s, 3H); 13C NMR (126 MHz, DMSO-d6) δ 163.73, 152.88, 150.38, 147.98, 133.93, 132.93, 127.83, 118.36, 115.55, 115.17, 61.06, 15.66.
3.4.6. 2-(4-Oxo-4H-chromen-3-yl)-2,3-dihydroquinazolin-4(1H)-one. FT-IR (cm−1): 3189.26, 3129.54, 3066.13, 1674.41, 1592.86, 1558.79, 1501.99, 1471.87, 1364.67, 1333.19, 1284.57, 1230.46, 1131.54, 1074.29, 1025.38, 943.83, 899.09, 872.38, 822.90, 765.80, 737.70, 701.46, 647.86, 612.34, 563.46; 1H NMR (500 MHz, DMSO-d6) δ 8.40 (d, J = 0.6 Hz, 1H), 8.13–8.06 (m, 2H), 7.84 (ddd, J = 8.7, 7.2, 1.7 Hz, 1H), 7.67 (td, J = 8.2, 1.0 Hz, 2H), 7.54 (ddd, J = 8.1, 7.2, 1.0 Hz, 1H), 7.26 (ddd, J = 8.2, 7.2, 1.6 Hz, 1H), 6.87 (s, 1H), 6.81–6.78 (m, 1H), 6.74–6.68 (m, 1H), 5.96 (s, 1H); 13C NMR (126 MHz, DMSO-d6) δ 175.94, 164.10, 156.18, 155.78, 148.14, 135.10, 133.84, 127.92, 126.33, 125.54, 123.72, 123.43, 119.02, 118.09, 115.34, 115.29, 60.01.
4 Conclusion
The research presents a successful and sustainable approach to synthesizing 2-aryl/heteroaryl substituted 2,3-dihydroquinazolin-4(1H)-ones, utilizing CSR and lemon juice as a natural catalyst. This method offers a significant improvement over traditional synthetic routes, which often rely on harsh conditions, toxic reagents, and non-renewable energy sources. By harnessing solar energy and a biodegradable, readily available catalyst, the study aligns with the principles of green chemistry, emphasizing waste prevention, energy efficiency, and the use of renewable resources. The optimized reaction conditions—0.3 mL of lemon juice under CSR—achieved 97% yield in 10 minutes, showcasing the efficiency and effectiveness of this method. The study also demonstrated that lemon juice outperformed conventional Brønsted acids and nanocatalysts in terms of efficiency and yield, while ethanol proved to be the most favorable solvent. The broad substrate scope, confirmed through the successful synthesis of various aromatic and heteroaromatic aldehydes, further underscores the practicality of this approach. This green, eco-friendly methodology provides a practical, scalable, and sustainable route for the synthesis of 2,3-dihydroquinazolin-4(1H)-one derivatives with diverse substitution patterns, advancing the principles of green chemistry.
Data availability
Data are available in the research paper and ESI.†
Author contributions
Rehan A. Khan: methodology, writing – original draft, writing – review & editing. Vishnu A. Adole: conceptualization, supervision, methodology, software, data curation, writing – original draft, writing – review & editing. Thansing B. Pawar: conceptualization, supervision, writing – original draft, writing – review & editing. Bapu S. Jagdale: resources and supervision.
Conflicts of interest
The authors declare that they have no known competing financial interests or personal relationships that could have appeared to influence the work reported in this paper.
Acknowledgements
The present research work was supported by Loknete Vyankatrao Hiray Arts, Science and Commerce College Panchavati, Nashik. Authors gratefully acknowledge CIF, SPPU, Pune for NMR and FT-IR characterization of samples.
References
- P. T. Anastas and J. C. Warner, Green Chemistry: Theory and Practice, Oxford University Press, 1998 Search PubMed.
- P. T. Anastas and N. Eghbali, Chem. Soc. Rev., 2010, 39, 301–312 RSC.
- K. J. Ardila-Fierro and J. G. Hernández, ChemSusChem, 2021, 14, 2145–2162 CrossRef CAS.
- A. Kurowska-Susdorf, M. Zwierżdżyński, A. M. Bevanda, S. Talić, A. Ivanković and J. Płotka-Wasylka, Trends Anal. Chem., 2019, 111, 185–196 CrossRef CAS.
- C. Michelin and N. Hoffmann, Curr. Opin. Green Sustainable Chem., 2018, 10, 40–45 CrossRef.
- J. Becker, C. Manske and S. Randl, Curr. Opin. Green Sustainable Chem., 2022, 33, 1–9 Search PubMed.
- A. Santana-Mayor, R. Rodriguez-Ramos, A. V. Herrera, B. Socas-Rodriguez and M. Á. Rodríguez-Delgado, Trends Anal. Chem., 2021, 134, 1–19 Search PubMed.
- K. G. Liu, Z. Sharifzadeh, F. Rouhani, M. Ghorbanloo and A. Morsali, Coord. Chem. Rev., 2021, 436, 1–44 CrossRef.
- B. Borah, K. D. Dwivedi, B. Kumar and L. R. Chowhan, Arabian J. Chem., 2021, 15, 1–38 Search PubMed.
- G. Chatel and R. S. Varma, Green Chem., 2019, 21, 6043–6050 Search PubMed.
- A. Naikwade, M. Jagadale, D. Kale, S. Gajare and G. Rashinkar, Catal. Lett., 2018, 148, 3178–3192 CrossRef CAS.
- S. Gajare, A. Patil, S. Hangirgekar, S. Dhanmane and G. Rashinkar, Res. Chem. Intermed., 2020, 46, 2417–2436 CrossRef CAS.
- M. Waheed, M. A. Alsharif, M. I. Alahmdi, S. Mukhtar and H. Parveen, Tetrahedron Lett., 2023, 119, 1–5 CrossRef.
- P. Selvam, K. Girija, G. Nagarajan and E. De Clercq, Indian J. Pharm. Sci., 2005, 67, 484–487 CAS.
- P. P. Kung, M. D. Casper, K. L. Cook, L. Wilson-Lingardo, L. M. Risen, T. A. Vickers, R. Ranken, L. B. Blyn, J. R. Wyatt, P. D. Cook and D. J. Ecker, J. Med. Chem., 1999, 42, 4705–4713 CrossRef CAS.
- V. Murugan, M. Kulkarni, R. M. Anand, E. P. Kumar, B. Suresh and V. M. Reddy, Asian J. Chem., 2006, 18, 900–906 CAS.
- V. Alagarsamy, V. R. Solomon and K. Dhanabal, Bioorg. Med. Chem., 2007, 15, 235–241 CrossRef CAS PubMed.
- Z. Rachid, F. Brahimi, Q. Qiu, C. Williams, J. M. Hartley, J. A. Hartley and B. J. Jean-Claude, J. Med. Chem., 2007, 50, 2605–2608 Search PubMed.
- M. Hori, R. Iemura, H. Hara, A. Ozaki, T. Sukamoto and H. Ohtaka, Chem. Pharm. Bull., 1990, 38, 681–687 CrossRef CAS.
- L. Heidari and L. Shiri, Appl. Organomet. Chem., 2019, 33, 1–11 Search PubMed.
- Y. Luo, Y. Wu, Y. Wang, H. Sun, Z. Xie, W. Zhang and Z. Gao, RSC Adv., 2016, 6, 66074–66077 RSC.
- A. Ghorbani-Choghamarani and M. Norouzi, J. Mol. Catal. A: Chem., 2014, 395, 172–179 Search PubMed.
- M. Hajjami, A. Ghorbani-Choghamarani, R. Ghafouri-Nejad and B. Tahmasbi, New J. Chem., 2016, 40, 3066–3074 RSC.
- N. Noori, M. Nikoorazm and A. Ghorbani-Choghamarani, Catal. Lett., 2017, 147, 204–214 Search PubMed.
- S. A. Pourmousavi, A. Kanaan, H. R. Fatahi, F. Ghorbani and D. Ajloo, J. Phys. Chem. Solids, 2017, 106, 82–93 Search PubMed.
- G. K. Kharmawlong, R. Nongrum, B. Chhetri, J. W. S. Rani, N. Rahman, A. K. Yadav and R. Nongkhlaw, Synth. Commun., 2019, 49, 2683–2695 Search PubMed.
- M. Ghashang, S. S. Mansoor and K. Aswin, Res. Chem. Intermed., 2015, 41, 3447–3460 CrossRef CAS.
- H. Kiyani, M. Tazari and F. Ghorbani, Lett. Org. Chem., 2018, 15, 523–529 Search PubMed.
- H. Ghafuri, N. Goodarzi, A. Rashidizadeh and M. A. Douzandegi Fard, Res. Chem. Intermed., 2019, 45, 5027–5043 CrossRef CAS.
- J. Chen, D. Wu, F. He, M. Liu, H. Wu, J. Ding and W. Su, Tetrahedron Lett., 2008, 49, 3814–3818 Search PubMed.
- M. Riyahinia, Z. S. Nazifi, H. Aghaei and A. R. Massah, Res. Chem. Intermed., 2023, 49, 979–995 Search PubMed.
- B. H. Chen, J. T. Li and G. F. Chen, Ultrason. Sonochem., 2015, 23, 59–65 CrossRef CAS PubMed.
- T. Tamoradi, M. Ghadermazi and A. Ghorbani-Choghamarani, Catal. Lett., 2018, 148, 857–872 CrossRef CAS.
- S. Azizi, J. Soleymani and M. Hasanzadeh, Nanocomposites, 2020, 6, 31–40 CrossRef CAS.
- A. Dutta, P. Trivedi, A. Kulshrestha, A. Kumar, V. Chaturvedi and D. Sarma, Appl. Organomet. Chem., 2021, 35, 1–14 Search PubMed.
- A. Ghorbani-Choghamarani and G. Azadi, RSC Adv., 2015, 5, 9752–9758 RSC.
- Y. U. Gadkari, N. T. Hatvate, B. S. Takale and V. N. Telvekar, New J. Chem., 2020, 44, 8167–8170 RSC.
- M. Norouzi, A. Ghorbani-Choghamarani and M. Nikoorazm, RSC Adv., 2016, 6, 92387–92401 RSC.
- L. Shiri, L. Heidari and M. Kazemi, Appl. Organomet. Chem., 2018, 32, 1–11 Search PubMed.
- L. Shiri, A. Ghorbani-Choghamarani and M. Kazemi, Appl. Organomet. Chem., 2017, 31, e3634 Search PubMed.
- A. Dutta, K. Damarla, A. Bordoloi, A. Kumar and D. Sarma, Tetrahedron Lett., 2019, 60, 1614–1619 Search PubMed.
- S. J. Wu, Z. Q. Zhao, J. S. Gao, B. H. Chen and G. F. Chen, Res. Chem. Intermed., 2019, 45, 2327–2339 CrossRef CAS.
- M. Mohammadi and A. Ghorbani-Choghamarani, RSC Adv., 2022, 12, 2770–2787 Search PubMed.
- S. Zhaleh, N. Hazeri and M. T. Maghsoodlou, Res. Chem. Intermed., 2016, 42, 6381–6390 CrossRef CAS.
- S. Yadav, S. Singh and C. Gupta, Curr. Res. Green Sustainable Chem., 2022, 5, 100260 CrossRef CAS.
- G. Yashwantrao, V. P. Jejurkar, R. Kshatriya and S. Saha, ACS Sustain. Chem. Eng., 2019, 7, 13551–13558 CrossRef CAS.
|
This journal is © The Royal Society of Chemistry 2024 |
Click here to see how this site uses Cookies. View our privacy policy here.