DOI:
10.1039/D4RA06210H
(Paper)
RSC Adv., 2024,
14, 37788-37796
Ruthenium(II) catalyzed C-3 site selective alkenylation of indole derivatives via C–H activation†
Received
28th August 2024
, Accepted 13th November 2024
First published on 26th November 2024
Abstract
An efficient synthetic method has been developed for C-3 site-selective alkenylation of indole derivatives under ruthenium(II) catalysis with an ester as a directing group. Besides the presence of two potential C(sp2)–H sites available for functionalization in the substrates, exclusive C3 selectivity was achieved in a selective manner as only mono-functionalized products were formed. The high site selectivity is attributed to the formation of an uncommon six-membered metallacycle intermediate between the ruthenium catalyst and ester directing group, enabled by the selective alkenylation at the C3 position of indole derivatives. This protocol features high site selectivity, operational simplicity, broad substrate scope, and moderate to high yields.
Introduction
The indole ring system is one of the most prevalent heterocycles found in nature, playing a crucial role in medicinal chemistry.1 Numerous studies have demonstrated that its importance arises from the wide range of biological properties exhibited by compounds with various substituent patterns around the indole nucleus.2 Over the last decade, several studies have highlighted the diverse biological activities of molecules containing the indole moiety. These activities encompass a broad spectrum, including but not limited to anti-HIV,3a anticancer,3b anti-inflammatory,3c antiviral,3d and antipsychotic3e effects, among others (Fig. 1).
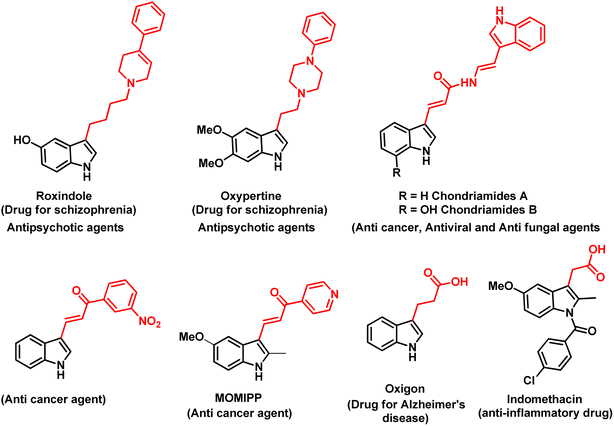 |
| Fig. 1 Pharmaceutically active compounds of 3-substituted indole derivatives. | |
Among various approaches, C–H activation has emerged as a pivotal strategy for the functionalization of a diverse array of organic molecules, serving as a common route for the formation of C–C bonds.4 Although a vast number of activation methods utilizing different metal catalysts, ligands, reaction conditions, and reagents have been discovered, achieving selective functionalization remains to be a challenging task.5 Moreover, even when directing groups are employed, achieving selective activation can be difficult, particularly when multiple C–H bonds are nearby, each with a similar likelihood of undergoing activation.6 The Ru(II) complexes have attracted a lot of interest in this context because of their great selectivity and affordability. They are extensively employed for the selective ortho C–H bond activation and oxidative Heck-type alkenylation of (hetero)aromatics with a wide range of alkenes.7,8
In 2005, the Gaunt group reported a palladium-catalyzed C2/C3 alkenylation of indoles, where they controlled the regioselectivity by employing different solvents9 (Scheme 1a). Additionally, independent studies conducted by Xu et al.,10a Carretero et al.,10b Choudhury et al.,10c Tanaka et al.,10d Zhu et al.10e and Walsh et al.10f have revealed that altering the reaction conditions can influence the reaction pathways. Furthermore, the generation of side products like over-olefination, annulation, and polymerization byproducts is a problem with metal-catalyzed C3-olefination of indoles, especially when palladium catalysts are used.11 Over the last two decades, Ru(II) catalysis has gained increasing attention for C–H functionalization due to its cost-effectiveness compared to metals such as Pd, Rh, and Ir.12 It has been reported that Ru catalysts have emerged as highly efficient and promising agents for the formation of C–C bonds using a directing group strategy.13 Although various strategies have been described for the regioselective insertion of functional groups at indole C-2, C-4, and C-7 positions.14 However, there have been no reports of selective alkenylation of indole derivatives at the C-3 position using a Ru catalyst and a directing group at the C-4 position, such as an ester. Therefore, our focus lies in achieving alkenylation at the C3 position using a Ru catalyst, a task that presents a significant challenge.
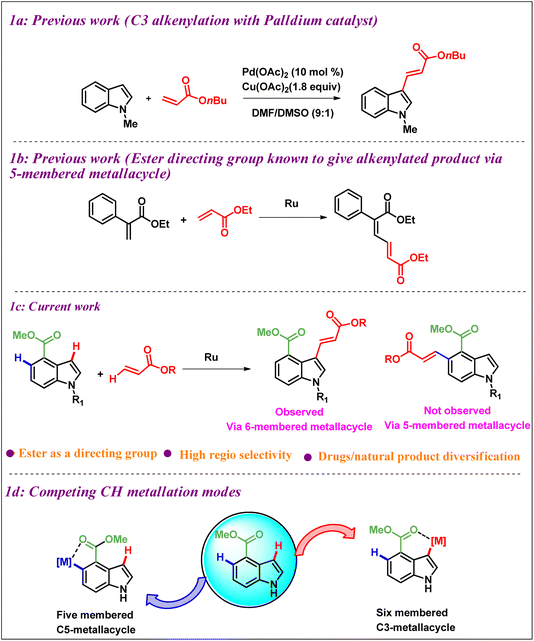 |
| Scheme 1 Comparative overview of reported catalytic alkenylation approaches for indoles, (1a). Palladium-catalyzed C2/C3 alkenylation of indoles, (1b). Ru-catalyzed C–H activation using an ester as a directing group, (1c). Ru(II)-catalyzed highly regioselective alkenylation of indole derivatives at the C-3 position, utilizing the ester functional group, (1d). Activation at C-3 must continue via a rare 6-membered metallacycle. | |
Previous studies have shown that Ru(II)-catalyzed C–H activation using an amide directing group proceeds through a rare 6-membered metallacycle, rather than the typical 5-membered metallacycle.15 Additionally, it has been reported that a stable 5-membered metallacycle, rather than a 6-membered one, can also promote Ru(II)-catalyzed C–H activation.7 In our research, we revealed that Ru(II)-catalyzed C–H activation can proceed through both 5- and 6-membered metallacycle intermediates, depending on the reaction conditions and the nature of the directing group used. As illustrated in Scheme 1b, the reaction of Ru-catalyzed C–H activation using an ester as a directing group with acrylates generally leads to the product through a five-membered metallacycle instead of six-membered metallacycle.16 Herein, we describe our recent finding (Scheme 1c) of a highly regioselective alkenylation of indole derivatives at the C-3 position, utilizing the ester functional group as a directing group at the C4 position and Ru(II) as a catalyst. Notably, unlike other known methods (Scheme 1c), our study reveals that the reaction proceeds via an uncommon six-membered metallacycle intermediate instead of a 5-membered metallacycle, resulting in the formation of selective C-3 alkenylated indole derivatives.
Selective functionalization of C-3 with a directing group at C-4 presents a challenging due to the presence of a substantially more reactive C-5 position. This is because activation at C-3 must proceed via a rare 6-membered metallacycle, whereas the activation at C-5 can proceed via a well-known stable 5-membered metallacycle, as shown in Scheme 1d.7,16 Hence, an appropriate C4-tethered directing group (DG) is in great demand to compete with C5-metalation and achieve C3-selectivity. In catalytic alkenylations, the presence of AgSbF6 and Cu(OAc)2 is crucial for ensuring the success of the reaction.17
Results and discussion
In this study, we initiated the optimization of the C-3 alkenylation of indole derivative 1a and methyl acrylate 2a as model substrates. Using methyl ester as a directing group, AgSbF6 as an additive, and Cu(OAc)2·H2O (1.2 equiv) as an oxidant in DMF solvent, the desired product, 3aa, was obtained with only a 17% yield (Table 1, entry 1). Our initial investigations revealed that substituting the ester group at the C-4 position of indole 1a with either an aldehyde or alcohol as the directing group resulted in no product formation.
Table 1 Optimization of Reaction Conditionsa
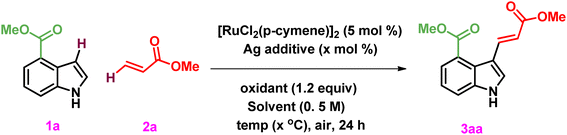
|
Entry |
Solvent |
Conditions |
Yield 3aab |
Reaction conditions: 1a (1 equiv, 0.2 mmol), 2b (3 equiv, 0.6 mmol), [RuCl2(p-cymene)]2 (5.0 mol%), additive (20 mol%), oxidant (1.2 equiv, 0.24 mmol), Solvent (0.5 M), temperature as mentioned, under air for 24 h. Isolated yield. Reaction was carried out at 50 °C. Reaction was carried out at room temperature. additive (40 mol%). Reaction without catalyst. RT = room temperature. n.d. = not detected. |
1 |
DMF |
AgSbF6/Cu(OAc)2·H2O/100 °C |
17% |
2 |
DCE |
AgSbF6/Cu(OAc)2·H2O/100 °C |
28% |
3 |
Dioxane |
AgSbF6/Cu(OAc)2·H2O/100 °C |
33% |
4 |
THF |
AgSbF6/Cu(OAc)2·H2O/100 °C |
52% |
5c |
DMF |
AgSbF6/Cu(OAc)2·H2O/50 °C |
24% |
6c |
DCE |
AgSbF6/Cu(OAc)2·H2O/50 °C |
34% |
7c |
Dioxane |
AgSbF6/Cu(OAc)2·H2O/50 °C |
73% |
8c |
THF |
AgSbF6/Cu(OAc)2·H2O/50 °C |
81% |
9d |
THF |
AgSbF6/Cu(OAc)2·H2O/RT |
16% |
10e |
THF |
AgSbF6/Cu(OAc)2·H2O/50 °C |
88% |
11e |
THF |
AgOTf/Cu(OAc)2·H2O/50 °C |
21% |
12e |
THF |
KPF6/Cu(OAc)2·H2O/50 °C |
63% |
13e |
THF |
AgBF4/Cu(OAc)2·H2O/50 °C |
56% |
14e |
THF |
AgSbF6/Ag2CO3/50 °C |
51% |
15e |
THF |
AgSbF6/Ag2O/50 °C |
22% |
16e |
THF |
AgSbF6/Na2S2O8/50 °C |
26% |
17e,f |
THF |
AgSbF6/Cu(OAc)2·H2O/50 °C |
n.d. |
18e |
THF |
AgSbF6/PhI(OAc)2/50 °C |
n.d. |
We proceeded to screen various reaction conditions to verify the effect of solvent polarity by using solvents such as DCE, 1,4-dioxane, and THF (refer to Table 1, entries 2–4). We observed a 52% yield using THF as the solvent (Table 1, entry 4). Furthermore, we observed that when the reaction temperature was lowered to 50 °C, the product yield was enhanced. To explore this, we attempted the same reaction by decreasing the temperature to 50 °C with a variety of solvents, resulting in the product yield being raised to 81% with THF as a solvent (Table 1, entries 5–8). It was also found that the yield of the product was disfavoured (16%, entry 9) when the reaction was performed at room temperature. By keeping other parameters fixed, when the equivalents of AgSbF6 were increased to 40 mol%, the yield of the product increased, reaching around 88% (Table 1, entry 10). We then examined different additives, such as AgOTf, KPF6, and AgBF4, and found that they all yielded less than AgSbF6 (Table 1, entries 11–13). Finally, to the effect of oxidants on product yield was explored by using a variety of oxidants: Ag2CO3, Ag2O, Na2S2O8, and PhI(OAc)2. These reactions displayed inferior results in all cases (Table 1, entries 14–17). The desired product did not form in the absence of the catalyst (Table 1, entries 18). The additive, oxidant, and catalyst were found to be essential in this process, as revealed by these control experiments. Thus, the optimal reaction condition for the C3 alkenylation of 1a involves the use of 5.0 mol% [RuCl2(p-cymene)]2, 40 mol% AgSbF6, and Cu(OAc)2·H2O (1.2 equiv.) in 0.5 M THF at 50 °C (Table 1, entry 10).
After obtaining the optimum reaction conditions, we explored the substrate scope and generality of the reaction for the C3 alkenylation of various indole derivatives, 1, with different acrylates, 2, resulting in good yields (Table 2). The reaction of methyl indole-4-carboxylate, 1a, with sterically distinct acrylates furnished products, 3aa, 3ab, 3ac, and 3ad, with yields ranging from 69 to 88%. However, no product was observed with acrylic acid. Similarly, methyl-1-methyl-1H-indole-4-carboxylate (3b) underwent alkenylation reactions with acrylates to afford products, 3ba–3bd, in 78–83% yield. Furthermore, the alkenylation reaction of methyl-1-ethyl-1H-indole-4-carboxylate with acrylates resulted in the corresponding products, 3ca–3cd, in 72–82% yields.
Table 2 Scope of indoles and acrylatesa,b
Reaction conditions: 1 (1 equiv, 0.2 mmol), 2 (3 equiv, 0.6 mmol), [RuCl2(p-cymene)]2 (5.0 mol%), AgSbF6 (20 mol%), Cu(OAc)2·H2O (1.2 equiv, 0.24 mmol), THF (0.5 M), 50 °C, 24 h. Isolated yield; (Me = methyl, Et = ethyl, Bn = benzyl, Ph = Phenyl, PMB = p-methoxybenzyl). |
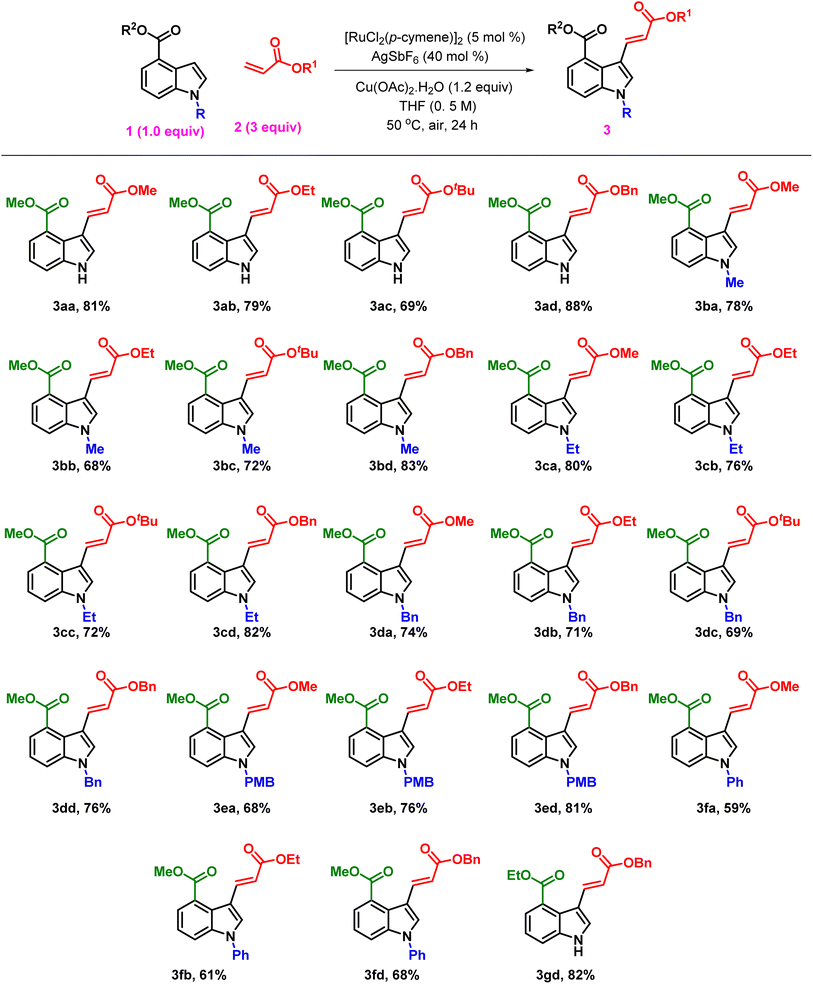 |
To investigate the effect of benzyl substituents on N–H indoles on alkenylation, we performed alkenylation on methyl-1-benzyl-1H-indole-4-carboxylate and methyl-1-(4-methoxybenzyl)-1H-indole-4-carboxylate, resulting in products, 3da–3dd, in moderate to good yields (69–76%). Additionally, we examined the reactivity of methyl-1-(4-methoxybenzyl)-1H-indole-4-carboxylate with different acrylates, which resulted in products, 3ea–3ec, in 68–81%. The effect of electron-withdrawing substituents present on indole toward alkenylation was studied by the alkenylation of methyl-1-phenyl-1H-indole-4-carboxylate, yielding products, 3fa–3fd, in moderate yields (59–68%). To investigate the influence of various esters at the C-4 position on the indole framework, we performed an alkenylation reaction with ethyl 1H-indole-4-carboxylate as the starting material. This process produced the intended product 3gd with an 82% yield. 1H NMR study indicated that only the trans-alkenylated product was formed. Additionally, as shown in Scheme 4c, a scale-up reaction was carried out on a 3 mmol scale to illustrate the feasibility of this approach, yielding 3aa in a 78% yield. Furthermore, we explored the reactivity of a useful olefin partner, such as methyl vinyl ketone (2e), with methyl 1H-indole-4-carboxylate (3a) under standard conditions (Scheme 2). Surprisingly, this resulted in the corresponding C-3 alkylated product, 3ae, with a yield of 76%. Similarly, indole derivatives, 3d and 3e, yielded the C3-alkylated products, 3de and 3ee, respectively with good yields of 66% and 69%.
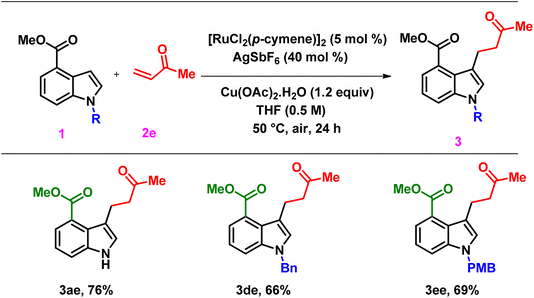 |
| Scheme 2 C3 alkylation with vinyl methyl ketone.a,b aReaction conditions: 1 (1 equiv, 0.2 mmol), 2 (3 equiv, 0.6 mmol), [RuCl2(p-cymene)]2 (5.0 mol%), AgSbF6 (20 mol%), Cu(OAc)2·H2O (1.2 equiv, 0.24 mmol), THF (0.5 M), 50 °C, 24 h. bIsolated yield. | |
3-alkenylated and alkylated indole derivatives represent attractive synthetic targets due to their pharmacological activity and their role precursors for many drugs, such as Roxindole, Oxypertine and Oxigon. To demonstrate the utility of C3-functionalized indoles, we attempted the conversion of the C3 alkenyl indoles into further modifications (Scheme 3).
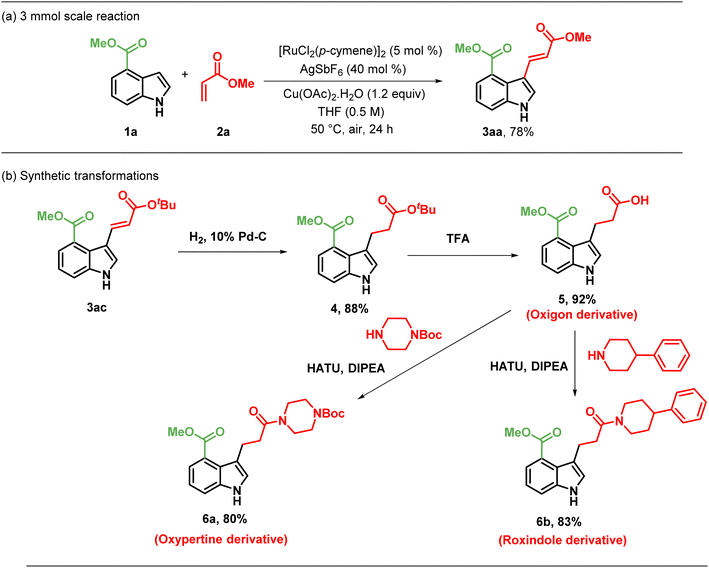 |
| Scheme 3 Synthetic Transformations of C3 Alkenyl Indoles. | |
The C-3 alkenylated indole derivatives were further modified into analogues of drug molecules to evaluate the feasibility of their synthesis (Scheme 3b). The C-3 alkenyl indole derivative, 3ac, was selectively reduced to the C-3 alkylated indole derivative 4 with an 88% yield, using hydrogen gas under 1 atm pressure in the presence of 10% Pd/C as a catalyst in dioxane. Further hydrolysis of 4 resulted in the generation of compound 5, a derivative of Oxigon (drug for Alzheimer's) and Tryptophan (natural amino acid). Consequent amide formation of 5 yielded 6a, a derivative of Oxypertine (antipsychotic agent), and 6b, a derivative of Roxindole (antipsychotic agent), with yields of 80% and 83% respectively.
To explain the origin of the site-selectivity of the ruthenium-catalysed C–H functionalization, deuterium incorporation experiments were conducted under standard reaction conditions (Scheme 4a). The analysis of the product revealed that methyl-1H-indole-4-carboxylate 1a in the absence of alkene resulted in indole, 1a-(D), with 50% deuterium incorporation at the C3 position, strongly supported by the observed regioselectivity (Scheme 4a). To ascertain whether the reaction proceeds through a radical pathway, the reaction was performed in the presence of 1 equiv. of TEMPO ((2,2,6,6-Tetramethylpiperidin-1-yl)oxyl) and BHT (2,6-di-tert-butyl-4-methylphenol). In both the cases, we obtained a good yield of the product 3ab (68% and 63%, Scheme 4b). These findings suggest that the reaction proceeds through a non-radical pathway.
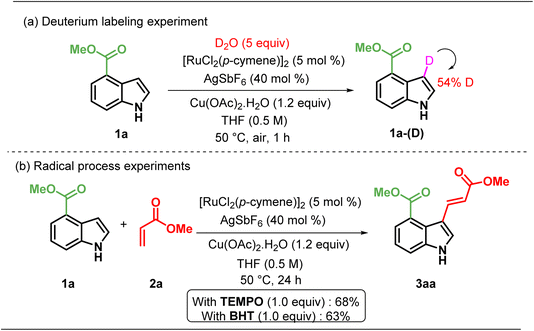 |
| Scheme 4 Mechanistic studies of site-selectivity of the ruthenium-catalysed C–H functionalization. | |
Scheme 5 illustrates a workable multistep catalytic cycle that has been proposed based on previous literature studies.18 In the presence of Cu(OAc)2·H2O and AgSbF6, an active catalyst, labelled as I, is formed. The first step involves the coordination of methyl-1H-indole-4-carboxylate, 1a, with an active ruthenium catalyst, followed by C–H metalation and releave of AcOH, leading to the formation of a six-membered ruthenium complex II. Subsequent coordination followed by insertion of acrylate 2 with intermediate II gave rise to the intermediate III. Finally, β-hydride elimination followed by release of AczH, led to the formation of the desired product 3, and the active catalyst was regenerated by reoxidation using Cu(OAc)2·H2O and air. Cu(OAc)2 act as a source of AcOH and air act as a terminal oxidant.16o
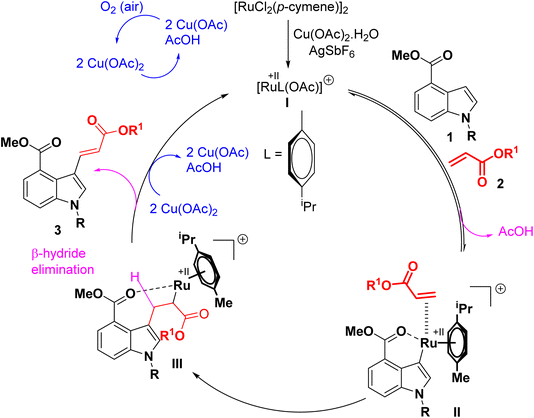 |
| Scheme 5 Mechanism of ruthenium-catalyzed site-selective C-3 alkenylation of indole derivatives. | |
Conclusions
In summary, we have developed a ruthenium-catalyzed site-selective C-3 alkenylation of indole derivatives by utilizing a directing group strategy via the formation of a 6-membered metallacycle. This method holds significance as it facilitates the formation of valuable 3-substituted indoles, a crucial class of molecules in medicinal chemistry, chemical biology, drug discovery, and related heterocyclic compounds. This method not only ensures high site selectivity but also offers mild reaction conditions, and broad substrate scope, making it a valuable approach for the functionalization of indole derivatives.
Data availability
Experimental procedures and the characterization data for reaction products, copies of NMR and additional information underlying this study are available in the published article and its ESI.† The authors confirm that the data supporting the findings of this study are available within the article [and/or] its ESI materials.†
Conflicts of interest
There are no conflicts to declare.
Acknowledgements
The authors are thankful to Dr Chandrasekhar Korapala, Dr Sathya Shanker and Dr Tonykurissery Anthappan of Syngene Intl. Ltd for their help and support during this research work. IS gratefully acknowledges the Department of Science and Technology (DST) and SERB for research grants (GITA/DST/TWN/P-103/2022) and (CRG/2021/003355) New Delhi, India.
References
-
(a) Y. Ban, Y. Murakami, Y. Iwasawa, M. Tsuchiya and N. Takano, Org. Biomol. Chem., 1988, 8, 231 CAS;
(b) U. Pindur and T. Lemster, Curr. Med. Chem., 2001, 8, 1681 CrossRef CAS PubMed;
(c) A. Ramirez and S. Garcia-Rubio, Curr. Med. Chem., 2003, 10, 1891 CrossRef CAS PubMed;
(d) A. J. Kochanowska-Karamyan and M. T. Hamann, Chem. Rev., 2010, 110, 4489 CrossRef CAS PubMed.
-
(a) Y. Meng, B. Yu, H. Huang, Y. Peng, E. Li, Y. Yao, C. Song, W. Yu, K. Zhu, K. Wang, D. Yi, J. Du and J. Chang, J. Med. Chem., 2021, 64, 925 CrossRef CAS PubMed;
(b) G. M. Ziarani, R. Moradi, T. Ahmadi and N. Lashgari, RSC Adv., 2018, 8, 12069 RSC;
(c) V. Sharma, P. Kumar and D. Pathak, J. Heterocycl. Chem., 2010, 47, 491 CrossRef CAS;
(d) H. Xu and M. Lv, Curr. Pharm. Des., 2009, 15, 2120–2148 CrossRef CAS PubMed;
(e) V. Sharma, P. Kumar and D. Pathak, J. Heterocycl. Chem., 2010, 47, 491 CrossRef CAS;
(f) S. Lal and T. J. Snape, Curr. Med. Chem., 2012, 19, 4828 CrossRef CAS PubMed.
-
(a) Q. Chen, C. Wu, J. Zhu, E. Li and Z. Xu, Med. Chem., 2022, 22, 993 CAS;
(b) Y. Wang, Y. Li, C. Yu, Y. Mi and Z. Tian, Eur. J. Med. Chem., 2019, 183, 111691 CrossRef PubMed;
(c) K. Hemalatha, G. Madhumitha and S. M. Roopan, Chem. Sci. Rev. Lett., 2013, 2, 287 Search PubMed;
(d) M. Z. Zhang, Q. Chen and G. F. Yang, Eur. J. Med. Chem., 2015, 89, 421–441 CrossRef CAS PubMed;
(e) N. K. Kaushik, N. Kaushik, P. Attri, N. Kumar, C. H. Kim, A. K. Verma and E. H. Choi, Molecules, 2013, 18, 6620 CrossRef CAS PubMed.
-
(a) P. B. Arockiam, C. Bruneau and P. H. Dixneuf, Chem. Rev., 2012, 112, 5879 CrossRef CAS PubMed;
(b) L. Ackermann, Chem. Rev., 2011, 111, 1315 CrossRef CAS PubMed;
(c) K. M. Engle, T.-S. Mei, M. Wasa and J. Q. Yu, Acc. Chem. Res., 2012, 45, 788 CrossRef CAS PubMed;
(d) X. Chen, K. M. Engle, D. H. Wang and J. Q. Yu, Angew. Chem., Int. Ed., 2009, 48, 5094 CrossRef CAS PubMed;
(e) V. S. Thirunavukkarasu, S. I. Kozhushkov and L. Ackermann, Chem. Commun., 2014, 50, 29 RSC;
(f) S. R. Neufeldt and M. S. Sanford, Acc. Chem. Res., 2012, 45, 936–946 CrossRef CAS PubMed;
(g) J. F. Hartwig, Acc. Chem. Res., 2017, 50, 549–555 CrossRef CAS PubMed;
(h) T. Brandhofer and O. García Mancheño, Eur. J. Org Chem., 2018, 2018(44), 6050–6067 CrossRef CAS;
(i) V. K. Tiwari and M. Kapur, Org. Biomol. Chem., 2019, 17(5), 1007–1026 RSC;
(j) R. Das, N. P. Khot, A. S. Deshpande and M. Kapur, Chem.–Eur. J., 2019, 26(4), 927–938 CrossRef PubMed;
(k) T. Iwai and M. Sawamura, ACS Catal., 2015, 5(9), 5031–5040 CrossRef CAS;
(l) K. Hirano and M. Miura, Chem. Sci., 2018, 9(1), 22–32 RSC;
(m) W. Wang, M. M. Lorion, J. Shah, A. R. Kapdi and L. Ackermann, Angew. Chem., 2018, 57(45), 14700–14717 CrossRef CAS PubMed;
(n) W. Ma, P. Gandeepan, J. Li and L. Ackermann, Org. Chem. Front., 2017, 4(7), 1435–1467 RSC.
-
(a) J. Wencel-Delord, T. Dröge, F. Liu and F. Glorius, Chem. Soc. Rev., 2011, 40, 4740 RSC;
(b) L. Ackermann, Chem. Rev., 2011, 111, 1315 CrossRef CAS PubMed;
(c) V. Ritleng, C. Sirlin and M. Pfeffer, Chem. Rev., 2002, 102, 1731 CrossRef CAS PubMed;
(d) K. Padala and M. Jeganmohan, Org. Lett., 2011, 13, 6144 CrossRef CAS PubMed;
(e) D. Colby, A. Tsai, R. Bergman and J. Ellman, Acc. Chem. Res., 2012, 45, 814 CrossRef CAS PubMed.
-
(a) T. W. Lyons and M. S. Sanford, Chem. Rev., 2010, 110, 1147 CrossRef CAS PubMed;
(b) K. M. Engle, T.-S. Mei, M. Wasa and J. Q. Yu, Acc. Chem. Res., 2012, 45, 788 CrossRef CAS PubMed;
(c) S. R. Neufeldt and M. S. Sanford, Acc. Chem. Res., 2012, 45, 936 CrossRef CAS PubMed.
-
(a) L. Ackermann and J. Pospech, Org. Lett., 2011, 13, 4153 CrossRef CAS PubMed;
(b) K. Padala and M. Jeganmohan, Org. Lett., 2011, 13, 6144 CrossRef CAS PubMed;
(c) K. Padala and M. Jeganmohan, Org. Lett., 2012, 14, 1134 CrossRef CAS PubMed;
(d) X. Lu, C. Shen, K. Meng, L. Zhao, T. Li, Y. Sun, J. Zhang and G. Zhong, Chem. Commun., 2019, 55, 826 RSC;
(e) R. Manikandan, P. Madasamy and M. Jeganmohan, ACS Catal., 2016, 6, 230 CrossRef CAS;
(f) G. D. Dias, T. A. Nascimento, K. A. Andresa, D. Almeida, A. C. S. Bombaça, F. S. Rubem, M. Barreto, C. Jacob, S. Warratz, N. Eufrânio, D. S. Júnior and L. Ackermann, Eur. J. Org Chem., 2019, 13, 2344 CrossRef;
(g) P. Zhao, R. Niu, F. Wang, K. Han and X. Li, Org. Lett., 2012, 14, 4166 CrossRef CAS PubMed;
(h) M. Bakthadoss and P. V. Kumara, Adv. Synth. Catal., 2018, 360, 1 CrossRef.
-
(a) H. Liang and J. Wang, Chem.–Eur. J., 2022, 29, 7 Search PubMed;
(b) H. E. Ketcham, M. T. Bennett, C. W. Reid and T. B. Gunnoe, Adv. Organomet. Chem., 2023, 93, 93–176 CrossRef;
(c) P. Singh, K. K. Chouhan and A. Mukherjee, Chem.–Asian J., 2021, 16, 2392–2412 CrossRef CAS PubMed;
(d) G.-F. Zha, H.-L. Qin and E. A. B. Kantchev, RSC Adv., 2016, 6, 30875–30885 RSC;
(e) C. Shan, L. Zhu, L.-B. Qu, R. Bai and Y. Lan, Chem. Soc. Rev., 2018, 47, 7552–7576 RSC;
(f) S. I. Kozhushkov and L. Ackermann, Chem. Sci., 2013, 4, 886–896 RSC.
- N. P. Grimster, C. Gauntlett, C. R. A. Godfrey and M. J. Gaunt, Angew. Chem., Int. Ed., 2005, 20, 3125 CrossRef PubMed.
-
(a) X. Zhang, Y. Li, H. Shi, L. Zhang, S. Zhang, X. Xu and Q. Liu, Chem. Commun., 2014, 50, 7306 RSC;
(b) A. M. Martinez, J. Echavarren, I. Alonso, N. Rodriguez, R. G. Arrayas and J. C. Carretero, Chem. Sci., 2015, 6, 5802 RSC;
(c) D. Ghorai, C. Dutta and J. Choudhury, ACS Catal., 2016, 6, 709 CrossRef CAS;
(d) T. Yamada, Y. Shibata and K. Tanaka, Chem.–Eur. J., 2019, 25, 16022 CrossRef CAS PubMed;
(e) S. Wu, Z. Wang, D. Ma, C. Chen and B. Zhu, Org. Chem. Front., 2020, 7, 1158 RSC;
(f) X. Xu, C. Luo, H. Zhao, Y. Pan, L. Zhang, J. Li, L. Xu, M. Lei and P. J. Walsh, Chem.–Eur. J., 2021, 27, 8811 CrossRef CAS PubMed.
-
(a) J. Sim, B. Ryou, M. Choi, C. Lee and C.-M. Park, Org. Lett., 2022, 24(23), 4264–4269 CrossRef CAS PubMed;
(b) C. Tan, H. Jiang, M. Zeng, K. Li, Z. Chen and G. Yin, Org. Biomol. Chem., 2022, 20(7), 1425–1435 RSC;
(c) C.-J. Wu, W.-X. Cao, B. Chen, C.-H. Tung and L.-Z. Wu, Org. Lett., 2021, 23(6), 2135–2139 CrossRef CAS PubMed;
(d) X.-R. Wu, H.-L. Peng, L.-Q. Wei, L.-P. Li, S.-Y. Yao and B.-H. Ye, Catal. Commun., 2019, 124, 12–18 CrossRef CAS;
(e) F. G. Cirujano, P. Leo, J. Vercammen, S. Smolders, G. Orcajo and D. E. De Vos, Adv. Synth. Catal., 2018, 360, 3872–3876 CrossRef CAS;
(f) A. K. Verma, A. K. Danodia, R. K. Saunthwal, M. Patel and D. Choudhary, Org. Lett., 2015, 17, 3658–3661 CrossRef CAS PubMed;
(g) R. K. Saunthwal, M. Patel, S. Kumar, A. K. Danodia and A. K. Verma, Chem.–Eur. J., 2015, 21, 18601–18605 CrossRef CAS PubMed.
-
(a) C. Bruneau and R. Gramage-Doria, Coord. Chem. Rev., 2021, 428, 213602 CrossRef;
(b) P. B. Arockiam, C. Bruneau and P. H. Dixneuf, Chem. Rev., 2012, 112, 5879 CrossRef CAS PubMed.
- X. Cui, X. Xu, S. J. McClain, B. Donnadieu, Z. Zhu, X. Qian, H. H. C. Lakmal and Z. U. Li, J. Am. Chem. Soc., 2019, 141, 15730 CrossRef PubMed.
-
(a) Y. Zhang, J. Zheng and S. Cui, J. Org. Chem., 2014, 79, 6490 CrossRef CAS PubMed;
(b) H. Ikemoto, T. Yoshino, K. Sakata, S. Matsunaga and M. Kanai, J. Am. Chem. Soc., 2014, 136, 5424 CrossRef CAS PubMed;
(c) Z. Q. Song, R. Samanta and A. P. Antonchick, Org. Lett., 2013, 15, 5662 CrossRef CAS PubMed;
(d) B. Li, J. Ma, W. Xie, H. Song, S. Xu and B. Wang, J. Org. Chem., 2013, 78, 9345 CrossRef CAS PubMed;
(e) X. F. Yang, X. H. Hu, C. Feng and T. P. Loh, Chem. Commun., 2015, 51, 2532 RSC;
(f) J. Shi, Y. Yan, Q. Li, H. E. Xu and W. Yi, Chem. Commun., 2014, 50, 6483 RSC;
(g) V. Lanke and K. R. Prabhu, Org. Lett., 2013, 15, 6262 CrossRef CAS PubMed;
(h) Q. Liu, Q. Li, Y. Ma and Y. Jia, Org. Lett., 2013, 15, 4528 CrossRef CAS PubMed.
-
(a) Y.-H. Sun, T.-Y. Sun, Y.-D. Wu, X. Zhang and Y. Rao, Chem. Sci., 2016, 7, 2229–2238 RSC;
(b) Y. C. Yuan, C. Bruneau, V. Dorcet, T. Roisnel and R. Gramage-Doria, J. Org. Chem., 2019, 84, 1898–1907 CrossRef CAS PubMed;
(c) Y. C. Yuan, C. Bruneau, T. Roisnel and R. Gramage-Doria, Org. Biomol. Chem., 2019, 17, 7517–7525 RSC;
(d) Y. C. Yuan, C. Bruneau, T. Roisnel and R. Gramage-Doria, Catal. Sci. Technol., 2019, 9, 4711–4717 RSC;
(e) Y. C. Yuan, C. Bruneau, T. Roisnel and R. Gramage-Doria, J. Org. Chem., 2019, 84, 12893–12903 CrossRef CAS PubMed;
(f) Y. Hashimoto, K. Hirano, T. Satoh, F. Kakiuchi and M. Miura, Org. Lett., 2012, 14, 2058–2061 CrossRef CAS PubMed;
(g) Y. Hashimoto, K. Hirano, T. Satoh, F. Kakiuchi and M. Miura, J. Org. Chem., 2013, 78, 638–646 CrossRef CAS PubMed;
(h) K. D. Collins, F. Lied and F. Glorius, Chem. Commun., 2014, 50, 4459–4461 RSC;
(i) W. Liu and L. Ackermann, Chem. Commun., 2014, 50, 1878–1881 RSC;
(j) Y. C. Yuan, Q. L. Lu, X. T. Zhu, S. Posada-Pérez, M. Solà, A. Poater and R. Gramage-Doria, Org. Chem. Front., 2023, 10, 42–53 RSC;
(k) J. Park, J. Lee and S. Chang, Angew. Chem., 2017, 129, 4320–4324 CrossRef;
(l) P. G. Chirila, L. Skibinski, K. Miller, A. Hamilton and C. J. Whiteoak, Adv. Synth. Catal., 2018, 360, 2324–2332 CrossRef CAS;
(m) R. Gramage-Doria, Chem.–Eur. J., 2020, 26, 9688–9709 CrossRef CAS PubMed;
(n) Y. C. Yuan, M. Goujon, C. Bruneau, T. Roisnel and R. Gramage-Doria, J. Org. Chem., 2019, 84, 16183–16191 CrossRef CAS PubMed.
-
(a) X. H. Hu, J. Zhang, X. F. Yang, Y. H. Xu and T. P. Loh, J. Am. Chem. Soc., 2015, 137, 3169 CrossRef CAS PubMed;
(b) K. Padala, S. Pimparkar, P. Madasamy and M. Jeganmohan, Chem. Commun., 2012, 48, 7140 RSC;
(c) Y. Hatamoto, S. Sakaguchi and Y. Ishii, Org. Lett., 2004, 6, 4623–4625 CrossRef CAS PubMed;
(d) Y. H. Xu, W. J. Wang, Z. K. Wen, J. J. Hartley and T. P. Loh, Tetrahedron Lett., 2010, 51, 3504–3507 CrossRef CAS;
(e) J. Zhang and T. P. Loh, Chem. Commun., 2012, 48, 11232–11234 RSC;
(f) X. H. Hu, J. Zhang, X. F. Yang, Y. H. Xu and T. P. Loh, J. Am. Chem. Soc., 2015, 137, 3169–3172 CrossRef CAS PubMed;
(g) T. Zhu, Z. Li, F. Xiao and W. L. Duan, Tetrahedron Lett., 2018, 59, 3238–3241 CrossRef CAS;
(h) C. Yu, F. Li, J. Zhang and G. Zhong, Chem. Commun., 2017, 53, 533–536 RSC;
(i) F. Li, C. Yu, J. Zhang and G. Zhong, Org. Lett., 2016, 18, 4582–4585 CrossRef CAS PubMed;
(j) R. Lekkala, R. Lekkala, B. Moku and H. L. Qin, Org. Chem. Front., 2019, 6, 796–800 RSC;
(k) M. Bakthadoss, T. T. Reddy, V. Agarwal and D. S. Sharada, Chem. Commun., 2022, 58, 1406–1409 RSC;
(l) H. Sindhe, A. Kamble, M. M. Reddy, A. Singh and S. Sharma, Org. Biomol. Chem., 2024, 22, 1162–1166 RSC;
(m) A. Aloia, M. Casiello, A. Nacci and A. Monopoli, Eur. J. Org Chem., 2024, 27, e202400111 CrossRef CAS;
(n) P. Sand and B. Schmidt, Eur. J. Org Chem., 2021, 2021(40), 5497–5506 CrossRef CAS;
(o) K. Graczyk, W. Ma and L. Ackermann, Org. Lett., 2012, 14, 4110–4113 CrossRef CAS PubMed.
-
(a) R. Giri, A. T. Brusoe and B. M. Trost, Org. Biomol. Chem., 2011, 9, 6496–6500 RSC;
(b) D. C. Powers and T. Ritter, Acc. Chem. Res., 2012, 45, 840–850 CrossRef CAS PubMed;
(c) J. Q. Yu and Z. Shi, Chem. Rev., 2016, 116, 4648–4682 CrossRef PubMed;
(d) T. W. Lyons and M. S. Sanford, Chem. Rev., 2010, 110, 1147–1169 CrossRef CAS PubMed;
(e) L. Ackermann, R. Vicente and A. R. Kapdi, Angew. Chem., Int. Ed., 2009, 48, 9792–9826 CrossRef CAS PubMed.
-
(a) S. R. Mohanty, N. Prusty, T. Nanda, S. K. Banjare and P. C. Ravikumar, J. Org. Chem., 2022, 87, 6189 CrossRef CAS PubMed;
(b) P. P. Jadhav, N. M. Kahar and S. G. Dawande, Org. Lett., 2021, 23, 8673 CrossRef CAS PubMed;
(c) J. Smith, A. Johnson, B. Lee and C. Wong, Catal. Sci. Technol., 2021, 11, 5772 RSC;
(d) K. Graczyk, W. Ma and L. Ackermann, Org. Lett., 2012, 14, 4110–4113 CrossRef CAS PubMed;
(e) J. A. Leitch, P. B. Wilson, C. L. McMullin, M. F. Mahon, Y. Bhonoah, I. H. Williams and C. G. Frost, ACS Catal., 2016, 6, 5520–5529 CrossRef CAS.
|
This journal is © The Royal Society of Chemistry 2024 |
Click here to see how this site uses Cookies. View our privacy policy here.