DOI:
10.1039/D4RA06342B
(Review Article)
RSC Adv., 2024,
14, 35727-35742
Revolutionizing applications: the impact of controlled surface chemistry on marble powder
Received
2nd September 2024
, Accepted 23rd October 2024
First published on 8th November 2024
Abstract
A large amount of marble powder is abundantly available as a byproduct and waste in the marble industry, and its reinforcement has been attempted in several applications through surface modification. This article examines the use of MP in the production of rubber, paper, foam stabilizers, asphalt, paint, textiles, and adhesives. This article aims to provide a foundation for the surface modification of MP to enhance its properties and broaden its range of applications. Data on modifiers such as organic acids, coupling agents, polymers, and surfactants have been gathered, along with comprehensive reaction details for various techniques, including wet modification, dry modification, in situ modification, ultrasonication, and sol–gel methods. A general overview of MP modification and its impact on composite properties is provided in this review paper, mainly focusing on the distinctive features of composites, contemporary field challenges, and the correlation between the properties and applications of MP.
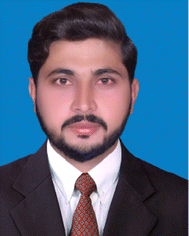 Ali Zia Noor | Ali Zia Noor recently completed his MS in Chemistry from the University of Education Lahore, Vehari Campus. His research focuses on modifying the surface of filler materials to enhance various properties. |
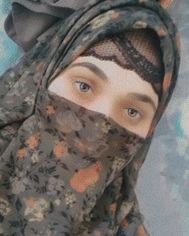 Sadia Bibi | Ms. Sadia Bibi completed her BSc from Women Postgraduate College Vehari, and her MSc in Chemistry from the University of Education Vehari Campus, Pakistan. Her research interests include photochemical surface modification protocols for inorganic filler particles. |
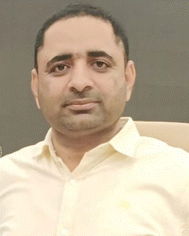 Muhammad Imran | Dr Muhammad Imran received his PhD from the International Center for Chemical and Biological Science, University of Karachi, Pakistan. He did his postdoctoral studies at the University of São Paulo, Brazil, and the University of Bristol, United Kingdom. Dr Imran has served Ghazi University Pakistan as Assistant and Associate Professor. Currently, he is working as Associate Professor of chemistry at King Khalid University, Abha, Saudi Arabia. |
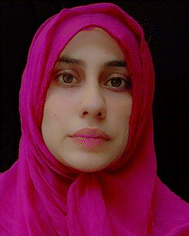 Sadia Afzal | Sadia Afzal recently completed her MS in Chemistry from the University of Education Lahore, Vehari Campus. Her academic and research pursuits focus on surface modification, particularly the practical applications of chemical principles to address real-world challenges. |
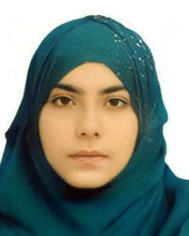 Sadiqa Abdal | Sadiqa Abdal completed her MSc in Chemistry from the University of Education Lahore, Vehari Campus. Her research interests include surface-modified particles. Her research topic is “Surface modification and its applications in magnetic particles derived from sesame husks”. |
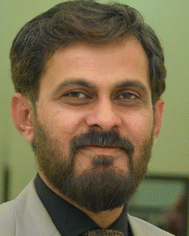 Muhammad Atif | Dr Muhammad Atif is Associate Professor (Chemistry) at the University of Education, Vehari Campus, Lahore, Pakistan. His PhD is on the surface modification of carbonaceous and other inorganic filler particles. |
1. Introduction
Marble is a metamorphic rock that is primarily made of recrystallized calcite (CaCO3) and additional minerals whose compositions might vary (Table 1). Marble is highly demanded in the construction business owing to its strength, adaptability, and aesthetic qualities. The largest centers for producing marble are in China, Italy, India, Turkey, Egypt, Iran, Brazil, and Sweden.5 A substantial amount of MP is produced while shaping, slicing, and polishing marble blocks. MP produced during marble processing constitutes greater than 60% of the total marble extract.6 Pakistan produced 350 million tons of MP in 2022.7 Every year, 140 billion tons of marble is manufactured worldwide.8 MP from building sites pollutes the environment on a global scale. Discarded MP can endanger people's health and agricultural soil.9 MP increases alkalinity, reducing soil fertility and exerting harmful environmental effects.10
Table 1 Chemical composition of MP
|
CaO |
SiO2 |
Al2O3 |
Fe2O3 |
MgO |
SO3 |
K2O |
Na2O |
Ref. |
Percentage (%) |
64.7 |
23.9 |
5.4 |
3.6 |
3.5 |
2.9 |
2.4 |
1.3 |
1 |
55.30 |
1.47 |
0.35 |
0.14 |
0.01 |
0.01 |
0.04 |
0.12 |
2 |
40.22 |
5.04 |
0.05 |
0.16 |
13.22 |
— |
0.03 |
0.01 |
3 |
54.08 |
0.77 |
0.24 |
0.05 |
0.94 |
— |
0.02 |
0.39 |
4 |
1.1 MP chemistry
MP is a finely ground powder obtained from marble. Its composition varies based on its origin and processing techniques. A comprehensive analysis of the standard composition of MP is outlined in Table 1. Generally, MP comprises approximately 80–95% calcium carbonate (CaCO3), 2–5% silica (SiO2), 0.5–2% aluminium oxide (Al2O3), 0.2–1% iron oxide (Fe2O3), 0.5–2% magnesium carbonate (MgCO3), 0.5–2% dolomite (CaMg(CO3)2), and 1–3% additional minerals (such as quartz, feldspar, and mica). The precise composition of MP may differ based on the particular marble origin, processing techniques, and any supplementary treatments or additives.
Calcium carbonate (CC), a significant constituent of MP, has widespread application as a reinforcing agent in a wide variety of manufacturing sectors, including asphalt,11 rubber,12,13 paper,14 foam stabilizer,15 textile,16 paint,17 cosmetic,18 and adhesive19 sectors. It serves as an optimal filler and reinforcement across several sectors owing to its low hardness, affordability, non-toxicity, and thermal and chemical stability.20 CC is a biogenic mineral obtained from marble and a wide variety of natural ores, including calcite, limestone, chalk, and precipitated CC, as well as eggshells, pearls, rocks, and microbial shells.21,22 Rather than relying on chemically synthesized or extracted CC, MP can be utilized with various morphologies, including spherical, cubic, rhombic, and polygonal shapes.23
2. Modification
MP surface modification refers to an alteration of its surface properties, either chemically or physically.24
2.1 Modification material
Various materials have been utilized for surface modification of MP, as reported in the literature (Table 2). Broad categories of materials include modifiers (the MP surface functionality enhancer) and additives (supporting modifiers).
Table 2 Commonly used modifiers and additives for MP
Modifier |
Additives |
Ref. |
Silane coupling agent |
Ammonium zirconium |
25 |
Stearic acid (SA) |
Isopropyl tristearyltitanate (ITT) |
26 |
Citric acid (CA) |
27 |
Titanate and aluminate |
28 |
Sodium stearate (SS) |
Starch |
29 |
Oleic acid (OA) |
n-Hexane |
30 |
2.1.1 Modifiers. MP modifier attaches to the MP surface through a range of modification processes. A modifier changes the material's quality and often makes it react as a reinforcing agent. Different kinds of modifiers, such as silicone-based molecules, organic acids, and esters, have been reported for the surface modification of MP (Table 2).
2.1.2 Additives. MP additives refer to metal-based or organic moiety compounds that facilitate the reactivity of modifiers to the surface of MP. These additives introduce structural changes in the modifier that enter into the MP surface, hence accelerating the modification process and altering reaction kinetics. Different additives have been reported for MP modification (Table 2).
2.2 MP morphology: surface, interior, and pores
The surface, interior, and pores of MP are essential factors in determining their modification and sorption characteristics. Surface properties, such as area, roughness, and functional groups, influence adsorption and reaction kinetics.31,32 MP interior affects diffusion and sorption rates. Pores control the accessibility and capacity of sorption. Modifications (functionalization, coating, or doping) can enhance or reduce sorption capabilities. Understanding the interplay between surface, interior, and pores is essential for tailoring MP's properties for specific applications.33
The section below presents an examination of the roles of the surface, interior, and pores of MP, together with their linkages to sorption and reactions.
The surface is the primary site for sorption and modification reactions. It influences wettability, dispersibility, and reactivity. The surface can be modified through functionalization, coating, or grafting.34
The interior represents bulk properties and affects overall performance. An interior structure can influence diffusion and sorption rates. Interior modification protocols involve doping, impregnation, or solid-state reactions.35
Pores relate to porosity and pore size distribution, impacting sorption capacity and rate. Depending on the application, pores can be modified to enhance or reduce sorption. Modification methods include pore expansion, contraction, or surface functionalization within pores.24
2.3 Types of modifications
MP utilization as filler and reinforcement requires surface functionalization to change the properties of the composite material. Depending on the purpose for which it is used, various properties can be achieved, such as reinforcement (by enhancing filler and matrix interactions)35 and compatibility (by better dispersion with minimal oligomerization).36 To improve the thermal stability of composite materials, enhanced adhesion and rheological properties are also influenced by surface modification.31,37,38 Surface modification is enhanced by employing various modifiers through a range of modification processes.
(1) Wet modification: it entails the use of liquid or solution for surface modification,39 e.g. chemical,24,39 surfactant,40 polymer,41 or sol–gel37,42 treatments.
(2) Dry modification: it modifies the material surface without using liquids or solutions,27 e.g. mechanical,24 thermal,43 plasma,44 radiation,45 and mechanochemical46 treatments.
(3) Ultrasonication treatment refers to the use of high-frequency sound waves and ultrasonic waves, usually ranging from 20 kHz to 40 kHz, to modify the surface properties of materials. It enhances modifier dispersion by applying sound waves.47,48
(4) In situ modification means changing the surface characteristics of MP while it is being applied, rather than doing it in a separate processing step. It enhances surface functionalities during chemical reactions.49 In situ modification techniques for MP include in situ polymerization,50 in situ coating (depositing thin films or coatings directly onto MP),51 in situ functionalization (introducing functional groups on MP surface),52 and in situ dispersion53 (Table 3 and Fig. 1).
Table 3 Survey of application-based modification of MP
Application |
Modification |
Ref. |
Method |
Reagent |
Paper industry |
Wet modification |
Carboxymethyl cellulose (CMC)/poly aluminum chloride |
35 |
Rubber industry |
Sodium oleate (SO) |
54 |
Construction industry |
Glycidoxypropy trimethoxysilane (GPTMS) |
55 |
Microwavable packaging |
SA |
56 |
Reinforcement |
Dry modification |
SO |
57 |
Protective textile/hydrophobic material |
In situ modification |
SA |
49 and 58 |
Paint industry |
Ultrasonication |
Triethoxy vinyl silane (TEVS) |
47 |
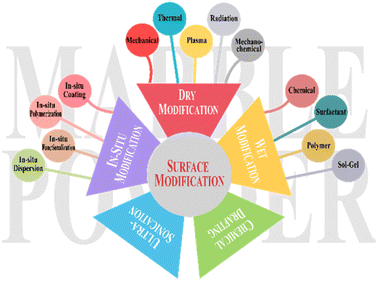 |
| Fig. 1 Types of MP surface modification. | |
Although several investigations have been reported regarding the reuse of MP for various applications, no specific report has been found that investigated the purity of MP before modification. MP throughout the world contains the same chemistry/composition. All the studies performed on MP have particularly focused on modification rather than purity. However, researchers have reported the purification of artificial MP (AMP) through thermos-chemical treatment under pressure. Purification was done using methanol at different temperature and pressure ranges.59 The literature has reported surface modification of MP waste for air quality improvement,60 polymeric composites,61 the mechanical strength of artificial stoneware,55,62 and super-hydrophobic coating,63 but none of these studies considered the impact of impurities on MP modification. A range of modifiers have been investigated for the surface modification of MP waste with TiO2,60 organic acids,61 and ethoxy silane,63 but any effect of impurity on MP modification has not been reported.61 Researchers have also reported typical sample preparation techniques, such as physical sieve and calcination (thermal process), to remove impurities.64 This might be attributed to the fact that inherent impurities in MP have a chemical nature similar to that of CC; therefore, no specific studies have been conducted to evaluate their impact on the modification protocols being applied to MP.
2.3.1 Wet modification. Wet modification involves a solid–liquid interaction between the modifier and particle, followed by stirring and dispersal at a predetermined temperature to produce the changed particles. Because the modifier and particle are in constant, close contact during wet modification, they have received more attention than dry modification. Silicone-based coupling agents, natural organic acids, organic and inorganic modifiers, and others were used to boost surface functionalities.
2.3.1.1 Silicone-based coupling agents. Silylether has been used as a modifier most frequently because it broadens the application range of filler particles. CC's surface modification has been reported with aminopropyl trimethoxysilane (APTS) to improve hydrophobicity.65 To improve APTS adhesion to the particles, CC particles were modified to increase the surface hydroxyl content; after that, these hydroxyl functionalities were used to attach multiple modifier molecules to the CC (Fig. 2). Contact angles were 99 and 110° when CC-APTS and CC–OH–APTS were compared, with CC–OH–APTS having the highest tensile strength (49.6 MPa). APTS modification has also been reported to improve the mechanical properties of CC.66 Modified CC demonstrated a maximum tensile strength of 497.28 ± 24.86 MPa and a maximum flexural strength of 19.91 ± 0.59 MPa at 3% loading. Wondu and coworkers67 used APTS as a coupling agent for CC surface modification. Thermal treatment of CC with silylether as a coupling agent produced 10 μm sized modified particles with a 58° contact angle. Modified particles alone disintegrated at 460 °C but upon mixing with the matrix demonstrated excellent thermal stability along with adhesion and dispersion.
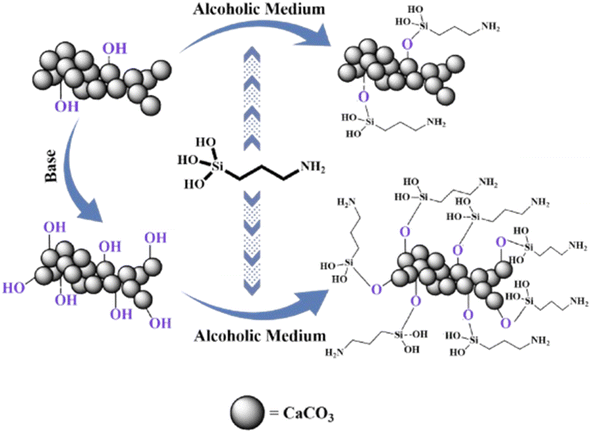 |
| Fig. 2 Surface modification of CC with ATPS. | |
MP modification with GPTMS has been reported55 for increased strength of artificial stoneware, with 2–5 μm particle size and 34.4 MPa flexural strength. The SA-supported GPTMS modification of CC has been reported62 for the improved mechanical strength of polyurethane (PU) composites. GPTMS modification reduces CC hydrophobicity, while SA modification augments it. With modified CC, an augmentation in PU composite elongation at break (196.4%) and tensile modulus (0.16 MPa) was reported. According to another study,68 GPTMS modification produced 45 nm-sized particles with enhanced surface functionalities and particle–matrix interaction. With 3 and 5 wt% of modified CC, the compression modulus, flexural, and tensile strength rose by 30/36/14% and 65/31/26%, respectively. Additives have also been reported with GPTMS to advance the modification process and properties of materials. Evidence suggests that adding ammonium zirconium to GPTMS-modified CC used as a paper filler improves both the strength and retention of the paper.25 Treating CC with 4% starch and 1% ammonium zirconium enhanced burst and tensile strength to 4.58 kPa m2 g−1 and 74.9 N m g−1, respectively.
A further investigation69 detailed the modification of CC using methyltrimethyoxysilane (MTMS) to improve foam stability, which was demonstrated by 15 wt% emulsions of MTMS-CC. This method produced particles of 78.40 nm size and increased the contact angle from 18° to 89.6°.
2.3.1.2 Natural organic acid. Numerous studies have documented the utilization of organic acids as modifiers for MP, aimed at altering their surface chemistry and expanding their range of applications. A brief description is presented in Table 4.
Table 4 List of organic acids used for surface modification
Natural organic acid |
Application |
Ref. |
CA |
Oil absorption |
27 |
SA |
Microwavable packaging |
56 |
Reinforcement |
70–72 |
Corrosion inhibitor |
33 |
Myristic acid (MA) |
Reinforcement |
73 |
OA |
Stabilizer |
74 |
Palmitic acid (PA) |
Microwaveable packaging |
75 |
Lauric acid (LA) |
Hydrophobic |
76 |
Tannic acid (TA) |
Biomedical |
77 |
Acrylic acid (AA) |
Composites |
78 |
Polylactic acid (PLA) |
Composites |
79 |
Amino hexanoic acid (AHA) |
Reinforcement |
80 |
Glutamic acid (GA) |
Reinforcement |
80 |
Antacid |
Biomedical |
77 |
Given its significant commercial relevance, SA finds application across various industries, prompting researchers to investigate it for CC surface modification. SA-modified CC has been reported70 for 70 nm-sized particles with improved mechanical strength and a water contact angle of 140°. Composite with 30% modified CC showed 19.21 MPa tensile strength, while adding more filler lowered the composite's mechanical characteristics. However, below the threshold, the incorporation of filler significantly improved the mechanical properties of the composite. Above the threshold concentration of CC, modification induced particle aggregation instead of improving the mechanical performance of the composites. Similarly, SA forbetter mechanical and thermal properties of polyvinyl chloride (PVC) composites has been reported,81 with two-phase compatibility. Thermal treatment (105 °C) of CC particles with the SA addition resulted in 65–135 nm-sized modified particles. Modified CC at 3.75 wt% exhibited a tensile strength of 577 kg m−2, whereas an increase in the modified CC percentage resulted in a decrease in the mechanical properties of the composite. The highest values for thermal stability, mechanical strength, and minimal elongation have been observed at 3.75% CC. SA modification has also been reported to affect CC interfacial structure and dispersion in thermoplastic composites.72 In dry treatment, 1.5 wt% SA and 98.5 wt% CC were mixed, while in wet treatment, SA was dissolved in NaOH at 75 °C, and CC was dispersed in distilled water; both solutions were mixed for 15 min at 75 °C. In the complex treatment, NaOH, SA, and CC were dissolved in water. The prepared particles were combined with high-density polypropylene (PP) to coat CC with a modifier. The maximum tensile strength for modified CC has been reported to be 33.81 MPa, while neat, dry, and complex treatment particles had 29.82 MPa, 28.52 MPa, and 30 MPa, respectively. Complex method particles were densely packed with a matrix. Complex and wet-treated CC composites have far better mechanical properties than plain and dry composites. The complex approach reduced void coalescence, which improved previous methods. SA modification of CC for enhanced hydrophobicity has been reported.33 The average diameters of the modified and unmodified particles were 4.09 μm and 8.30 μm, respectively, indicating the size reduction of the modified particles. The contact angles were 110° and 124° for modified and unmodified particles, respectively, indicating higher hydrophobicity and corrosion resistance. Modified particles resist pressure and heat well. Wet modification of SA-treated CC71 led to a reduction in particle size (0.08 μm), as well as a decrease in particle–particle and polymer–particle interactions, thereby enhancing particle dispersion within the polymer matrix.
There have been reports of using additives with acids to enhance the modification process, resulting in CC being an exceptional reinforcement agent. CC's surface modification, through adsorption, has been reported73 with chloroform and 2-propanol solutions of SA, MA, OA, PA, and LA. This modification enhanced CC's suitability for industrial applications, such as rubber, paint, and plastic. Modified CC's interaction with various fatty acids has been optimized, considering variables, such as reaction speed, modifier concentration, temperature, solvent solubility, hydrophobic characteristics, and dispersibility. SA had the highest activation degree and dispersion ratio of all the fatty acids, at almost 95% and 67%, respectively. Another group presented enhanced matrix-filler compatibility26 through SA and ITT reactive therapy (CC modification) and unreactive therapy (CC coating). Unmodified, ITT-modified, and SA-modified particles averaged 2.98 μm, 2.68 μm, and 2.73 μm, with tensile strengths of 15.8 ± 0.6, 34.7 ± 0.7, and 23.4 ± 1, respectively. The ITT- and SA-modified particles had contact angles of 136° ± 3° and 155° ± 5°, respectively. Both agents augmented the composite's dispersion and mechanical qualities but darkened CC. SA, titanate, and aluminate-modified CC in asphalt composite have been reported for superior mechanical characteristics and rutting resistance compared to unmodified28 while being used to improve road performance. SA impregnation on CC has also been reported to impact the alloy's mechanical and thermal properties.82 Modified particles increased composite tensile strength (42 ± 0.3 MPa) and elongation at break (19% ± 8%).
Along with SA, styrene acrylic polymer emulsion (SAPE) has been reported for CC modification to improve PP mechanical properties.41 Modification increased the particle size from 100 nm of unmodified CC to 115.2 nm of SAPE-modified CC. Composites with modified particles have high mechanical strength, i.e. 25% CC/SAPE composite showed a maximum strength of 37.5 ± 2 MPa. Base-mediated SA modification of CC has been reported to enhance the quality of microwave passive material.56 The mechanical strength of modified particles has been reported to be better than unmodified ones, i.e. maximum tensile strength (26.93 MPa) was achieved with 1% SA.
CC surface modification by OA has also been reported in the literature. Because of OA's distinct chemical properties, especially its hydrophobicity, which allows it to interact with the CC surface and serve as an important surface modifier for CC, many researchers have expressed interest in the material. Augmentation in composite strength has been reported with OA modification of CC.30 Through this modification, 48.6 nm scalenohedral particles were obtained. Mechanical strength was increased by 21.5% with a maximum 5% modifier. Composite disintegrated at 468.7 °C, with unaffected CC morphology. Another research group reported enhancing compressive and acoustic characteristics in CC modified with OA.15 Modification improved the matrix-filler interfacial contact. The results for stress relaxation (28.5%) and hysteresis loss (5.2%) were markedly improved with chemically treated fillers compared to the untreated cases. This enhancement was primarily attributed to the improved interfacial interactions and compatibility between the PU matrix and the calcium carbonate filler surfaces. Another group reported polyethylene (PE) mechanical properties by modifying CC with OA. Zapata et al.83 modified CC's surface with OA to increase PE's mechanical qualities. Young moduli for the reference and 3% and 5% composites were 202 MPa, 230 MPa and 250 MPa, respectively. Kim et al.74 found that phosphatidylcholine (PC) and OA increased CC's suspension stability. The modified particles had an average size of 0.098 μm. OA was linked to CC by ionic interaction, while PC was attached by hydrophobic interaction, which enhanced suspension stability. CC surface functionalities were increased by OA, which in turn produced a good matrix and modifier interaction to enhance mechanical properties.
Surface modification of CC has also been reported with SS through adsorption. Enhanced dispersibility and compatibility of CC in different media have been reported. CC was modified with a homogenous coating of SS84 for enhanced thermal stability. An increase of up to 5% in the modifier increased the contact angle from 24.7° to 118.9°, yet the value decreased as further increase was made, i.e. for 10% modifier, the contact angle was 97.5°. With and without thermal treatment, the modified CC's water contact angle reached 139.6° from 112.8°.
SS and additives have also been reported in the literature, prompting the utilization of CC as a reinforcing agent to improve the properties of diverse materials. Sodium hexametaphosphate, SS and starch-based modification of CC (100 μm) has been reported for enhanced mechanical properties of paper,85 with an optimized concentration of 1.5% at 60 °C resulting in a 25% increase in tensile strength. Modified CC enhanced paper whiteness due to better distribution. Another study29 proved the SS functionalization of CC for enhanced mechanical strength, brightness, and optical properties.
Additional types of organic acids have been reported to alter the surface of CC and transform it into a reinforcing agent. TA-modified CC has been reported77 for biological uses. TA, CaCl2 and Na2CO3 generated nanoparticles, which were agglomerated into macromolecules. Antacid has been reported to modify TA-treated spherical-shaped CC (3–6 μm) for anti-inflammatory and antioxidant effects.
AA-modified CC via chemical deposition has been reported86 for ultra-high molecular weight PE composite (UHMWPC) processability. Wax and AA were used to graft PE onto the modified particles. Increased modified particle content lowered composite torque, indicating decreased melt viscosity. With increasing modified CC content, composite thermal stability improved. Modified CC enhanced the crystallization temperature, enthalpy, and friction.78 Methacrylic acid (MAA) has also been reported31 as a modifier for CC to improve rheological and thermomechanical properties. The average particle size was greater than 3 μm. Composite with 2% modifier had 49.90 ± 0.8 MPa tensile strength. Modified particles showed higher dispersion in the composite, which improved the mechanical characteristics, perceived viscosity, and pseudoplastic melting behavior. Another modifier PA has also been reported75 to enhance the thermomechanical characteristics of CC composites. In the PP film, the modified particles showed reduced aggregation and improved dispersion. PA-modified CC at 5 wt% exhibited 281 MPa mechanical strength. Polymeric modifiers have also been reported87 for CC modification via impregnation with polyethyleneimine, PU, PLA, and polyaluminum chloride (PAC) to pause corrosion. 2–5 μm spherical particles with no agglomerates were reported. Modified particles disintegrated at 465–629 °C. The epoxy coating containing impregnated particles demonstrated superior corrosion inhibition compared to the reference sample.
Glycidyl methacrylate-modified CC has been studied79 for thermo-mechanical characteristics of the PU/PLA blend. NaOH treatment introduced hydroxyl groups on the CC surface, which reacted with 5% glycidyl methacrylate, resulting in a 1 μm change in particle size. The contact angles for plain and modified PLA were 90° and 36°, respectively, revealing its polar nature and miscibility with water. The unmodified and modified particles had 7.9 ± 0.2 MPa and 7.5 ± 0.2 MPa tensile strengths, respectively. The modified composite was more substantial than the neat sample, where tensile strength decreased due to the PU's poor strength.
PAC-treated CC has been reported35 to improve the mechanical properties of paper materials. CC's functionalization with 0.8% PAC and 4% CMC produced 6626 N m−2 of tensile strength because the inter-fiber bond formation was increased. CC-fiber compatibility was increased by a modification that boosted the mechanical properties of the paper by increasing the fiber–fiber bond. The mechanical properties of composite materials have also been improved with sodium dodecyl sulphate. CC modification with sodium dodecyl sulphate, ethylene diamine tetra acetic acid, and sodium carbonate has been reported to investigate thermomechanical properties.36 This modification increased the CC surface area, which in turn enhanced the mechanical properties of a polymer. The modified CC acted as a nucleating agent to improve the crystallization rate, which contributed to the improvement of the thermomechanical properties. The addition of 0.8% modified CC increased the tensile strength from 76.4 to 77.9 MPa. Silica-functionalized CC has also been reported to enhance mechanical properties.37 The average diameters of modified and unmodified particles were 4.0 and 6.3 μm with 25% silica, respectively. Modified particles increased the mechanical properties of paper compared to unmodified particles. Improved mechanical strength resulted from hydrogen bonding between the hydroxyl group of silica on the modified CC and cellulose fiber. Sol–gel modification effectively enhanced the filler–fiber interaction. The modified and unmodified particles have tensile indices of 43.7 N m g−1 and 38.7 N m g−1, respectively. TiO2-modified MP has been reported60 to improve air quality by decomposing 95% of harmful chemicals. SO-modified CC has been reported54 for the rubber industry, with an average particle size of 10.75 μm and contact angles of 108° and 36° for modified and unmodified particles, respectively. Modified rubber presented 21% and 15% greater tensile strength and elongation at break, respectively, compared to common rubber. The hydrophobic character of CC has been improved by the adsorption of anionic fluorinated surfactant.88 The results showed that a low surfactant content emerged as the most suitable candidate for foam stabilizer applicability. The hydrophobic properties of CC were enhanced at levels below the dosage of the modifier needed for the mono coating layer. At high modifier concentrations, multilayer formation on CC changed its hydrophobic properties to a hydrophilic one. Sodium lignosulfonates (SLS) modified CC microparticles (2–10 μm) with spherical shapes have been reported for corrosion inhibition properties.89 CC microparticles prepared by CaCl2 and Na2CO3 co-precipitation were modified with SLSs, and after modification, the original spherical morphology was maintained with prismatic calcite. The calcite mole fractions for unmodified and modified CC were 39.39% and 44.05%, respectively, where an increase in the calcite fraction indicates SLS presence. Ethylene glycol (EG)-modified CC has been reported for bone regeneration application.90 CC was suspended in dimethylformamide (DMFA). Then, 1,6 hexamethylene diisocyanate (HMDI) and Sn(Oct)2 catalysts were added under a nitrogen environment to obtain 20–30 μm particles. The composite of the modified particles, with a 27.4 MPa tensile strength and 64.6° contact angle, showed promising results in bone repairing and bone tissue engineering.
2.3.1.3 Polybutylene adipate co-terephthalate (PBAT). Researchers have also worked on PBAT as a modifier to enhance the photocatalytic and mechanical responses of materials. Yang et al.91 utilized PBAT to improve the hydrolytic and photo-oxidative degradation of CC. This modification enhanced filler matrix interaction, resulting in improved CC shading, which subsequently postponed film deterioration, whereas the neat film exhibited faster degradation. A 2 wt% silane-coated CC film exhibited a maximum tensile strength of 210.51 MPa; however, its mechanical properties diminished when exposed to a water spray. Zhang et al.92 demonstrated that PBAT anchored to the CC surface enhanced the mechanical properties of the film while reducing its thermoplastic values. Tensile strength and break at elongation decreased with particle content but increased with particle size. Optimized conditions were 30% particle content, 5 μm particle size, and 2% silane modifier.
2.3.2 Dry modification. Dry modification involves the mechanical blending of modifiers with dry particles at predetermined temperatures to produce modified particles. Dry modification is an alternative to conventional ball milling due to some drawbacks associated with conventional methods. These drawbacks include low stirring, uneven mixing, and inadequate dispersion of materials and modified agents.CC modification with AHA has been reported to enhance thermomechanical characteristics. SA, GA, caprolactam, and L-arginine have been compared against AHA80 for CC activation through thermal treatment regarding the impact on its mechanical properties. Coated CC had superior tensile strength (66 MPa) and excellent elongation (610%), at just 2.5 mmol dose of 6-AHA/100 square feet CC. Additionally, CC dry modification through 6-AHA has been reported43 for the mechanical and thermal properties of polyamide 12. The average particle size of CC was 9 μm with an irregular shape. Researchers reported an increase of 510% in elongation at the break, with 2–3 mmol AHA for 100 m2 CC.
Another modifier, SA, has also been used commonly for CC. SA-based surface medication of CC through tumbling ball milling has been reported,93 resulting in an 18 μm particle size with a 34% oil absorption ratio. Additives have also been reported with SA to enhance the surface chemistry of CC. Anhydrous CA has been documented27 as an additive for SA-based CC modification, with modified CC exhibiting thermal decomposition at 350 °C. The oil absorption values for the unmodified and modified particles were 33.9 ml/100 g and 19 ml/100 g, respectively. Oil absorption value decreased due to the presence of SA on the surface of CC.
Another modifier reported is SO, which is used to improve the hydrophobic character of CC.57 This work was performed on calcite through ball milling. The average particle size of the CC was 7.32 μm. The contact angles for the unmodified and modified particles were 22.8° ± 3° and 109.6° ± 3°, respectively. The hydrophobicity of calcite was significantly increased after modification.
2.3.3 Chemical grafting method. Chemical grafting encompasses two primary methodologies: ‘grafting to’ and ‘grafting from.’ ‘Grafting to’ entails a reaction between a functionalized reagent and the functional groups present on the substrate. While ‘grafting from’ initiates a graft from the surface of the substrate, it somehow corresponds to in situ modification. The ‘grafting to’ technique along with the ion exchange method has been reported94 for surface modification. Modifiers included quaternary ammonium salt and sulfonate salt. The modification was carried out in two steps: the first involved grafting a hydroxyl group onto quaternary ammonium salt and the second involved an ionic reaction with sulfonate salt. The modified CC had an average particle size of 50 nm, and the presence of S, Si, Ca, and C indicated that the salts had been successfully grafted. Modified CC thermally disintegrated at 600 °C, indicating convenient preparation of asphalt binder at standard temperature. At low temperatures, the modified particles improved the asphalt binder's compatibility and crack resistance performance. However, at high temperatures, the modified particles had a detrimental impact.
2.3.4 In situ modification method. In situ treatment involves increased surface chemistry during the synthesis or processing of material. The significance of in situ modification surpasses other modification processes due to its ability to achieve high product quality and efficiency without the need for high-temperature conditions or organic solvents. In situ modification with calcium stearate (CS) has been reported,95 which was adsorbed on the CC particle surface. The average particle size after modification was 34 nm, with a hydrophobic nature and excellent dispersion. The contact angle and specific area were 107.8° and 30 m2 g−1, respectively.CC modification with SA has been reported49 through dip coating in situ surface modification for water-resistive textiles. The contact angles for 1 w/w% and 1.5 w/w% were 150.2° and 148.5°, respectively. This showed that a higher amount of SA has no effect on the contact angle but makes white spots on the fabric due to the multilayer assembly of SA. The optimized ratios for maximum hydrophobic properties were 1% SA and 6% CC. Dang et al.58 prepared hydrophobic vaterite particles using SA-based in situ surface modification. The average particle sizes of the CC and modified CC were 3.26 μm and 2.96 μm, respectively. Modified particles showed a 130.2° ± 1.2° contact angle, whereas unmodified showed 0° contact angle. The contact angle increase indicates a notable enhancement in particle hydrophobicity. This modification enhanced the purity and stability of vaterite particles, elevating their content to 97%.
2.3.5 Ultrasonication. Ultrasonication modifies the physical and chemical properties of the materials using high-frequency sound vibrations. Ultrasound-assisted surface modification of CC has been reported for the paint industry47 through precipitation using TEVS as a coupling agent. Ultrasound cavitation was used for better mixing and dispersion, where the ultrasonic probe was immersed in CC/acetone solution to produce sonic waves; then, TEVS was added dropwise into it. The average size of modified particles increased from 80 to 90 nm, with square crystal morphology, and 152° contact angle. Improved particle dispersion in the polymeric matrix was a result of sonication-based modification, which also reduced processing time and increased yield.
3. Comparison of MP modification methods
The surface modification process is a crucial step in tailoring material properties to meet a wide range of application requirements. Several techniques are used to modify surface characteristics, including wet and dry treatments, chemical grafting, and in situ modification. Wet modification involves the utilization of liquid or solution-based treatments that are both simple to execute and cost-effective. Dry modification necessitates the deposition of gas or vapor onto a surface that is suitable for high-temperature procedures. It is important to note that the deposition rate in dry treatment is generally slower than that in wet treatment and is limited to the modification of thin layers. Wet modification treatment can be preferred over dry modification for the following reasons: wet modification provides stronger mechanical properties to materials than dry modification by increasing the adsorption density of the modifier, which in turn enhances filler–matrix interaction, increasing mechanical properties. Another benefit of wet modification over dry modification is that wet modification provides less void content due to the good dispersion of particles, which makes the dense and closed internal structure of the composite and enhances adhesion impact.72,96 Dry modification provides numerous advantages in contrast to wet modification, especially when it comes to material processing. First, it significantly decreases the requirement for large amounts of water, which not only benefits the environment but also proves to be cost-effective by reducing expenses associated with water treatment and disposal. Additionally, it improves process efficiency by decreasing drying time and energy usage.72 The phenomenon of chemical grafting refers to the movement of atoms or molecules across a material surface, leading to changes in its composition. However, the treatment process mentioned above requires specialized equipment and expertise, and managing the diffusion profile may present a challenging task. The in situ surface modification process involves the alteration of surface properties during the synthesis or processing phases of a material. This approach allows for greater control over the final material properties and facilitates the surface modification of bulk materials. This specific treatment requires complex and specialized processing techniques, which in turn require a substantial investment of time. Numerous surface modification techniques, including wet, dry, chemical grafting, and in situ modifications, offer a diverse array of options for tailoring material surfaces to meet specific requirements. The choice of a suitable technique depends on the desired modifications, substrate properties, and intended application. The data for the modification methods, modifiers, properties, and their applications are summarized in Table 5.
Table 5 Surface modification of CC
Method |
Modifier |
Matrix |
Reaction condition |
Particle size (μm) |
Tensile strength (MPa) |
Contact angle |
Application |
Ref. |
Wet modification |
APTS |
Epoxy resin |
95% ethanol, 10 m ultrasonication, 8 h reflux (80 °C), pH 4–5 |
N/R |
497.28 ± 24.86 |
N/R |
Industrial |
66 |
PVC |
2 h stirring, 1.5% mass ratio of CC : APTS, 125 °C drying (8 h) |
N/R |
49.60 |
110.0° |
Reinforcement |
65 |
PLA |
Ethanol, 80 °C (6 h) |
10 |
N/R |
58.0° |
Water soluble PU composite |
67 |
SLS |
Epoxy resin |
Soaked in 15 wt% SLS, 50 °C |
2–10 |
N/R |
N/R |
Corrosion inhibitor |
89 |
Unmodified |
PP |
Coupling agent (1 wt% of CC), 2000 rpm/5 m |
2.98 |
15.80 ± 0.60 |
|
Reinforcement |
26 |
SA |
2.73 |
23.40 ± 1.00 |
155 ± 5.0° |
ITT |
2.68 |
34.70 ± 0.70 |
136 ± 3.0° |
TA |
N/R |
CaCl2/TA, Na2CO3, 5 m stirring, 1200 rpm |
3–6 |
N/R |
N/R |
Biomedical |
77 |
SS |
N/R |
SS, 80 °C, 1 h stirring |
2.39 |
N/R |
118.9° |
Hydrophobic materials |
84 |
SA |
PVC |
CC preheating 105 °C; SA |
0.06–0.14 |
56.58 |
N/R |
PVC composite reinforcement |
81 |
Polycaprolactone |
N/R |
0.07 |
19.21 |
140.0° |
Reinforcement |
70 |
PP |
1.5 h stirring, 5 h (100 °C) |
0.08 |
N/R |
N/R |
71 |
High-density PE |
SA, NaOH, 75 °C |
N/R |
33.81 |
78.8° |
72 |
Epoxy resin |
N/R |
0.90 |
54.00 |
N/R |
97 |
Polylactide |
SA in ethanol by ultrasonication (10 m), 300 rpm for 6 h |
5.00 |
N/R |
110.0° |
Corrosion inhibitor |
33 |
Isotactic PP |
CC preheated at 60 °C. SA in aqueous NaOH |
N/R |
26.93 |
N/R |
Microwavable packaging |
56 |
PAC & CMC |
N/R |
Stirred CC/water (15 m), water/CMC heated at 60 °C, mixed and then added 10% PAC, and dried 4 days in the oven |
N/R |
6.63 × 10−3 |
N/R |
Paper industry |
35 |
SAPE and SA |
PP |
SAPE sprayed on CC, ultrasonicated (10 m), then stirrer at 300 rpm for 2 h (80 °C) |
0.12 |
37.50 ± 2 |
N/R |
Polymer composite reinforcement |
41 |
Silane coupling agent |
PBAT |
CC/modifier stirrer at high speed for 3–5 m (100 °C) |
5.00 |
21.00 ± 0.51 |
N/R |
Biodegradable mulching film |
91 |
CC/modified mixed with high-speed mixer at 100 °C for 3–5 m |
5.00–12.00 |
21.00 |
N/R |
92 |
Polyimide |
N/R |
0.02–0.03 |
N/R |
75.6° |
Reinforcement |
98 |
Epoxy resin |
CC/95% ethanolic solution/modifier, stirrer for 12 h at 80 °C |
0.04 |
11 150.00 ± 15 |
N/R |
68 |
GPTMS |
N/R |
Methanol/distilled water/silane mixed, and then added MP |
2.00–5.00 |
34.40 |
N/R |
Construction industry |
55 |
MTMS |
N/R |
CC/aqueous solution containing silane stirrer 24 h |
7.80 × 10−2 |
N/R |
89.6° |
Emulsion and foam stabilizer |
69 |
SO |
Organic |
CC/SO/water mixed constantly, filtered and dried |
10.75 |
N/R |
108.0° |
Rubber industry |
54 |
Oleate |
N/R |
N/R |
0.01–2.50 |
N/R |
N/R |
Paper industry |
99 |
Starch, SS, SHMP |
Polyacrylamide |
15% starch/CC dispersed in cool water at 200 rpm for 10 m, heated at 95 °C/50 m, and added SS/SHMP for 30 m at 200 rpm |
2.00 |
N/R |
N/R |
29 and 85 |
N/R |
15% starch/CC added in water for 10 m at 200 rpm, heated at 95 °C/50 m, then added SS temp. decrease to 60 °C, SHMP for 30 m/200 rpm |
N/R |
N/R |
115.7° |
100 |
Anionic fluorinated surfactant |
N/R |
CC/aqueous solution of surfactant stirrer for 25 m, heated at 25 °C for a day |
7.80 × 10−2 |
N/R |
115.1° |
Foam stabilizer |
88 |
EG & PLL |
Polyester |
CC was added in DMFA and HMDI for 8 h at 50 °C, added EG at 60 °C/12; PLL dissolved in xylene (60 °C) and then CC was added for 24 h at 120 °C (under N2 protection) |
20.00–30.00 |
27.40 |
64.6° |
Bone regeneration |
90 |
Lanthanum |
PP |
Lanthanum dissolved in water, then added in CC suspension at rt, followed by alkali solution, washed, and dried 70–80 °C |
0.04 |
36.00 ± 1.00 |
N/R |
Reinforcement |
101 |
Titanate coupling agent & SA |
PU |
CC/isopropyl alcohol stirrer for 10 m, added coupling agent at stirrer for 30 m, and oven dried at 100 °C for 24 h |
3.50 |
0.16 ± 0.10 |
N/R |
62 |
Sodium dodecyl sulfonate |
N/R |
CC/sodium dodecyl sulfonate mixed at 220 rpm, heated for 4 h at 80 °C, filtered, washed and dried |
2.70 |
N/R |
N/R |
Hydraulic oil additive |
102 |
PA |
PP |
CC preheated at 60 °C for 24 h, PA dispersed in NaOH, then added CC for 30 m, and dried for 24 h at 100 °C |
N/R |
28.38 |
N/R |
Microwaveable packaging |
75 |
Sol gel |
Silica |
Acrylonitrile butadiene |
Ethanol/water/NH3 mixed together, CC/silica then added to the above solution and stirred at 200 rpm for 24 h |
0.05–0.09 |
3.60 ± 0.20 |
N/R |
Rubber industry |
103 |
Dry modification |
MAA |
PP |
CC/methacrylate/poly-propylene mixed in twin-screw extruder |
3.00 |
40.09 ± 0.80 |
N/R |
Reinforcement |
31 |
SO |
CC/SO/grinding media mixed at 1000 rpm, and then filtered |
7.32 |
N/R |
109.6 ± 3.0° |
57 |
SA |
High density PE |
CC/SA mixed through screw extruder (16 mm diameter) |
N/R |
28.52 |
75.3° |
72 |
Ultrasonication |
TEVS |
Polymer |
CC dispersed in acetone under ultrasound, modifier dissolved in acetone, ultrasound probe added in CC suspension, and added modifier solution in it |
0.05–0.08 |
N/R |
152.0° |
Paint industry |
47 |
In situ modification |
CS |
N/R |
CC/CS heated |
3.40 × 10−2 |
N/R |
107.8° |
Industrial application |
95 |
SA |
Polyester |
SA mixed with isopropyl alcohol, then added CC with stirring (10 m), washed with water, and dried |
1.00 |
N/R |
150.2° |
Protective textile |
49 |
Polymer |
SA dissolved in ethanol; CC mixed with the solution through a homogenizer at 20 000 rpm for 1 m |
3.26 |
N/R |
130.2 ± 1.2° |
Hydrophobic material |
58 |
Regardless of the modification process, the size of the modified CC particles ranges from nanometers to micrometers (Table 5). Evidence clearly demonstrates that the modification technique does not impact particle size. Instead, the modifier is responsible for individual particle sizes. The thickness of the modifier coating is an important factor that affects the size of the modified particle although not very promising.104,105 A mechanistic explanation is required to discuss the significant disparity in particle size after modification. This mechanistic explanation can be provided by examining the distinctions among particle, aggregate, and agglomerate sizes.106 Particulate size represents the size of individual crystallites, while aggregates refer to several particles linked together by modifier crosslinking. Agglomerates, however, reflect the connection of aggregates through covalent bonding, physical contact, or adsorption.
4. Applications
A comparison of modification techniques based on the applications of the materials revealed certain facts. An increase in the tensile strength of a material typically results in more brittleness and less potential for deformation, while a decrease in tensile strength correlates with enhanced plasticity, more prone to deformation, and greater ductility.107 A contact angle exceeding 90° suggests that the material surface exhibits hydrophilic characteristics, which is attributed to the presence of polar surface groups and higher surface energy.108,109 Conversely, a contact angle below 90° signifies a hydrophobic nature linked to non-polar surface groups and lower surface energy.63,110 The sizes of the particles significantly affect the material's surface area and the quantity of pores present on that surface. A smaller particle size suggests an increased surface area, whereas particles exhibiting a larger surface area demonstrate a reduced surface size.26,54 The surface modification of CC for industrial applications is illustrated in Fig. 3.
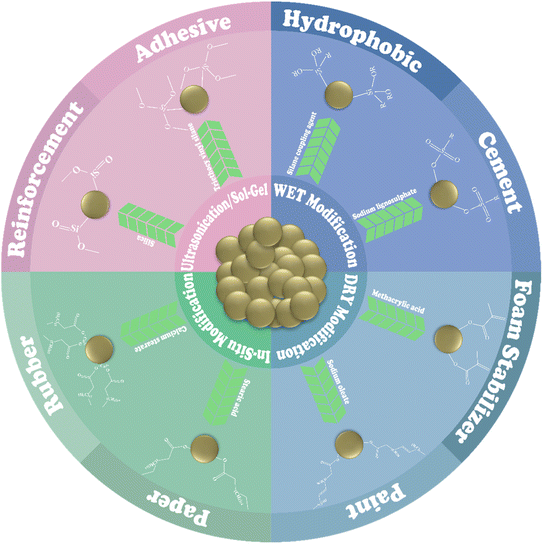 |
| Fig. 3 Industrial application of modified CC. | |
4.1 Plastic industry
CC is frequently used as a filler for plastics, such as PLA, PVC, PP, and PE, to create polymer-based composite materials. This practice may lower costs while simultaneously enhancing the strength and toughness of plastics and extending the range of applications. Piekarska et al.97 produced PLA films containing CC modified with SA. The results showed that using 5 wt% of modified CC as filler increased the elongation at break and tensile toughness by about two times (Fig. 4). Composite films in food packaging and biomedical applications are gaining popularity because PLA is a biodegradable polymer.
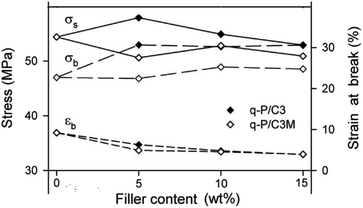 |
| Fig. 4 Tensile strength and strain at break at different wt% values for filler content, adopted from ref. 97 with license 5513130080154. | |
Due to its many advantageous qualities, such as low cost, ease of production, high strength and toughness, and resistance to environmental stress cracking, PP is one of the three most commonly used plastics. The mechanical strength (about 65%) and hardness (about 5%) of a PP-based composite filled with SA-modified CC have recently been shown to be significantly improved by Buasri et al.111 Additionally, it was found that adding less than 10 wt% of modified CC to both high- and low-density PE when creating composite materials could significantly increase their tensile and impact strengths.112,113 The mechanical properties of PVC-based composites with CC as a filler improved similarly. The toughness of CC/PVC composite materials can be increased using ultrafine CC with excellent dispersibility as a filler because previous studies suggest that the debonding of particles and matrix can absorb stress.114 As modifier particle size decreases, the impact strength of composites containing CC increases.115 Elastic modulus, tensile yield strength, and elongation at break of CC/PVC composite material were found to be best at 5 wt% CC. Due to the modified CC's high dispersion and ability to scatter UV light, PVC-based composites have improved their antiaging capabilities.116 Recently, there has been much interest in superhydrophobic materials due to their distinct characteristics and exceptional performance. This implies that a superhydrophobic-modified CC coating has a wide range of potential applications. With the aid of epoxy resins containing epoxidized fatty acid-modified CC,117 a superhydrophobic coating that makes steel waterproof and self-cleaning has been created. This coating has a sliding angle of 1.3° and a water contact angle of 160.5°.
4.2 Paper industry
In modern paper manufacturing, the filler's significance has grown over time. Because of its high surface area, porosity, and whiteness, among other advantages, CC is frequently used to replace fiber in the production of paper. Studies have been conducted on the modification of CC particles with oleate and cetrimonium bromide (CTAB) for use in wet-end papermaking. Because adding more fillers did not affect the paper's tensile strength, the cost was reduced. Additionally, apparent density, opacity, and mechanical properties are factors that the oleate modifier affects more strongly than CTAB.118 Fan et al. also conducted several studies on using CC-modified composites in the papermaking process.29,100 Initially, researchers investigated the impact of starch/SS composite-modified CC on the strength attributes of paper. Particle size ranging from 35.2 to 54.0 μm was shown to be excellent for paper strength, and paper filled with modified CC outperformed unmodified CC in terms of tensile index, tear index, and burst index. Additional research on CC modified with starch, SS, and sodium hexametaphosphate revealed that adding CC modified at a lower temperature of 60 °C, 1.5 wt% of sodium hexametaphosphate, and 4.0 wt% of SS significantly improved paper strength performance.29,100 In addition to its application as a colored coating on dry-formed paper, CC may also be used as a filler in papermaking processing. Consequently, the paper acquires preferable properties, such as specific weight, air permeability, surface smoothness, opacity, and ink absorption rate. An anionic surfactant (i.e., SO) modified CC was shown to increase color dispersion and coating stability by Barhoum et al.99 The results showed 23.0%, 1.3%, 2.8%, and 2.3% improvements in the paper's smoothness, brightness, whiteness, and opacity, respectively. The paper's air permeability decreased by 26.0% when covered with the modified CC-pigmented coating. The enhanced CC-colored coating can help improve the paper's resistance to water. Surface coating and impregnation with fatty acids can give super hydrophobic paper (Fig. 5), resulting in the production of inexpensive paper with a water contact angle greater than 150°.119
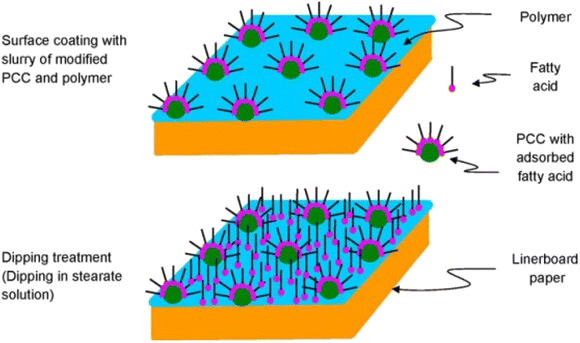 |
| Fig. 5 Superhydrophobic modification of paper, adopted from ref. 119 with license 5523500888812. | |
4.3 Rubber industry
Elastomers, such as butadiene rubber, silicone rubber, ethylene–propylene–diene mischpolymer, fluoro-rubber, and styrene–butadiene rubber, have advantageous properties for use in tires, gaskets, gloves, and assorted other items. Rubber can be reinforced and filled with CC and other inorganic fillers to increase volume, decrease costs, and improve processing performance without significantly affecting product efficacy. Jin et al.120 discovered that adding CC to a composite material significantly improved its tensile strength (Fig. 6), ductility, and thermal stability. Additionally, the improved interfacial compatibility of the CC particles with the rubber matrix significantly positively impacted the thermal stability and mechanical qualities of the composite material, particularly when CC was modified before being incorporated into the matrix. For instance, silane coupling agents may be used to treat CC particles to increase their hydrophobicity, dispersibility, and ability to reduce viscosity.121
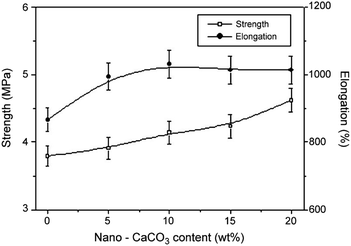 |
| Fig. 6 Elongation and strength at different wt% values for modified CC content, adopted from ref. 120 with license 5514630500751. | |
4.4 Environmental protection
There will always be new sources of pollution, but as society and humans face more severe problems over time, environmental protection becomes more and more critical for the planet's long-term progress. For example, waste marble from various processing techniques can have disastrous effects on people and the environment when working with marble resources. Lu et al.55 enhanced the properties of waste marble-derived CC powder using a silane coupling agent, producing durable synthetic ceramics suitable for construction and cement.122 Marine oil spills, a pressing global issue, have caused devastating consequences for the aquatic environment, atmosphere, plant life, and even human beings. It may be beneficial to use oil- and water-resistant materials when cleaning up oil spills. The maximum water contact angle of LA-modified CC was found to be 140°, and the oil absorption capacity improved with the enhancement of lipophilicity. LA was used to modify CC in situ to create hydrophobic and lipophilic materials. As a result of the substance's effective oil absorption in oil–water solutions, it can be used as a practical oil absorbent in various oil spill situations.76 The widespread presence of heavy metal contamination in our air, water, and soil poses a significant risk to ecosystems and human health. Mallakpour et al.123 examined the creation of composite materials using modified polyvinyl alcohol/CC (poly(vinyl alcohol)-APTS, or PVA). The ATS-CC/PVA composite material was shown to have an adsorption capacity for Cu(II) of 45.45 mg g−1 under the conditions of pH = 6.5 and room temperature. Additionally, the composite material's mechanical and thermal stability improved.
5. Challenges and future perspective
MP is employed as a filler in a matrix to add volume and, to some extent, affect the properties of the composite. The concentration of MP as a filler increases beyond a specific limit and has a detrimental effect on composites, i.e., it reduces the mechanical strength of composites.124,125 Given this restriction, surface functionalization using various modifiers to boost mechanical impact and associated ceramic composite qualities should be investigated. Additionally, the ideal combination to maximize the ceramic composite's characteristics and the use of MP to safeguard the environment from adverse consequences must be addressed. Modified MP with a silane coupling agent can also be used in other fields, such as heterogeneous catalysts, which have broad applications.126
6. Conclusion
This study outlined the MP modification process and its applications in several industries, including rubber, paper, paint, textile, and adhesives. The mechanical properties of the modified materials were enhanced, leading to increased efficacy as reinforcement, which was attributed to advancements in the surface functionalities of MP. Natural organic acids, coupling agents, anionic and cationic surfactants, and inorganic oxides are the primary materials used to attach to and coat the MP surface. This study examined the best modifier and matrix pairings that produced the most significant improvements in composite qualities.
Data availability
No primary research results, software or code has been included and no new data were generated or analysed as part of this review.
Conflicts of interest
There are no conflicts to declare.
Acknowledgements
The authors express their appreciation to the Deanship of Scientific Research at King Khalid University, Saudi Arabia, for this work through the research group program under grant number RGP-2/232/45.
References
- J. Ahmad, O. Zaid, M. S. Siddique, F. Aslam, H. Alabduljabbar and K. M. Khedher, Mater. Res. Express, 2021, 8(7), 075505 CrossRef CAS
. - M. Belouadah, Z. E. Rahmouni, N. Tebbal and M. E. H. Hicham, Ann. Chim.: Sci. Mater., 2021, 45(5), 361–368 CrossRef
. - F. E. Santos, C. H. Borgert, L. R. Neto, J. R. De Oliveira, J. O. Alves, J. P. Machado, F. F. Grillo, V. B. Telles and E. Junca, Metall. Mater. Trans. B, 2021, 52(3), 1664–1680 CrossRef CAS
. - Z. Khan, A. Gul, S. A. A. Shah, S. Qazi, N. Wahab, E. Badshah and K. Shahzada, Civ. Eng. J., 2021, 7(09), 1488–1500 CrossRef
. - Y. Y. Lim, T. M. Pham and J. Kumar, Constr. Build. Mater., 2021, 283, 122795 CrossRef
. - O. Y. Bayraktar, G. Kaplan, O. Gencel, A. Benli and M. Sutcu, Constr. Build. Mater., 2021, 288, 123128 CrossRef
. - K. Khan, W. Ahmad, M. N. Amin, A. Ahmad, S. Nazar, A. A. Alabdullah and A. M. A. Arab, Mater, 2022, 15(12), 4108 CrossRef CAS PubMed
. - E. Kuoribo and H. Mahmoud, J. Cleaner Prod., 2022, 133872 CrossRef
. - A. S. SD, A. Dinesh and S. Babu, Mater. Today, 2021, 44, 4223–4226 Search PubMed
. - M. Sufian, S. Ullah, K. A. Ostrowski, A. Ahmad, A. Zia, K. Śliwa-Wieczorek, M. Siddiq and A. A. Awan, Mater, 2021, 14(14), 3829 CrossRef CAS PubMed
. - A. M. Yarahmadi, G. Shafabakhsh and A. Asakereh, Constr. Build. Mater., 2022, 317, 126127 CrossRef CAS
. - A. Krishna, S. Sabarinath, P. Abhishek, G. U. Chandran, S. Hema, and S. Sambhudevan, Macromolecular Symposia, Wiley Online Library, 2022 Search PubMed
. - C. Cui, H. Ding, L. Cao and D. Chen, Pol. J. Chem. Technol., 2015, 17(4), 128–133 CrossRef CAS
. - H. Zhou, R. Xun, Z. Zhou, Q. Liu, P. Wu and K. Wu, Fibers Polym., 2014, 15(3), 519–524 CrossRef CAS
. - H. Choe, J. H. Lee and J. H. Kim, Compos. Sci. Technol., 2020, 194, 108153 CrossRef CAS
. - Y. Liu, H. Zhang, Y. Zhang, C. Liang and Q. An, Composites, Part B, 2022, 109988 CrossRef CAS
. - O. Ersoy, D. Güler and M. Rençberoğlu, Coat., 2021, 12(1), 44 CrossRef
. - V. Lauth, M. Maas and K. Rezwan, Mater. Sci. Eng., C, 2017, 78, 305–314 CrossRef CAS PubMed
. - A. Rudawska and M. Frigione, Mater, 2021, 14(6), 1324 CrossRef CAS PubMed
. - Y. Boyjoo, V. K. Pareek and J. Liu, J. Mater. Chem. A, 2014, 2(35), 14270–14288 RSC
. - N. J. Vickers, Curr. Biol., 2017, 27(14), R713–R715 CrossRef CAS PubMed
. - B. Cantaert, D. Kuo, S. Matsumura, T. Nishimura, T. Sakamoto and T. Kato, ChemPlusChem, 2017, 82(1), 107–120 CrossRef CAS PubMed
. - C.-q. Li, C. Liang, Z.-m. Chen, Y.-h. Di, S.-l. Zheng, S. Wei and Z.-m. Sun, J. Cent. South Univ., 2021, 28(9), 2589–2611 CrossRef CAS
. - J. Qiu, J. W. Lyu, J. L. Yang, K. B. Cui, H. Z. Liu, G. F. Wang and X. Liu, Part. Syst. Charact., 2024, 2400097 CrossRef
. - Y. Wu, C. Zhao, Y. Jiang, and W. Han, 2016 4th International Conference on Machinery, Materials and Computing Technology, Atlantis Press, 2016 Search PubMed
. - O. Ersoy and H. Köse, Polym. Compos., 2020, 41(9), 3483–3490 CrossRef CAS
. - M. Li, X. Yang, and J. Chen, IOP Conference Series: Materials Science and Engineering, IOP Publishing, 2020 Search PubMed
. - J. Li, S. Yang, Y. Liu, Y. Muhammad, Z. Su and J. Yang, Constr. Build. Mater., 2019, 218, 413–423 CrossRef CAS
. - H. Fan, X. Wang, J. Liu and B. Xu, BioResources, 2015, 10(4), 6861–6871 CAS
. - A. H. Ritonga, N. Jamarun, S. Arief, H. Aziz, D. A. Tanjung, B. Isfa, V. Sisca and H. Faisal, J. Mater. Res. Technol., 2022, 17, 2326–2332 CrossRef CAS
. - H. U. Zaman and M. Beg, J. Thermoplast. Compos. Mater., 2016, 29(2), 189–203 CrossRef CAS
. - A. Irshad, M. Atif, A. Ghani, B. Ali, S. A. Ahmad and M. Alex, Sci. Rep., 2023, 13(1), 7356 CrossRef CAS PubMed
. - Y. He, Y. Fan, P. Luo and Q. Yang, Russ. J. Appl. Chem., 2015, 88(6), 962–969 CrossRef CAS
. - M. Atif, H. Z. Haider, R. Bongiovanni, M. Fayyaz, T. Razzaq and S. Gul, Surf. Interfaces, 2022, 31, 102080 CrossRef CAS
. - H. Mousavipazhouh, M. Azadfallah and I. R. Jouybari, Maderas: Cienc. Tecnol., 2018, 20(4), 703–714 CAS
. - Y. H. Cai, Y. H. Zhang and L. S. Zhao, Polimery, 2015, 60(2), 95–99 CrossRef
. - A. F. Lourenço, J. A. Gamelas, J. Sequeira, P. J. Ferreira and J. L. Velho, BioResources, 2015, 10(4), 8312–8324 CrossRef
. - M. Atif, R. A. Mehmood, A. Z. Noor, S. Shaukat, S. Mahmood and I. Ali, Diamond Relat. Mater., 2023, 140, 110394 CrossRef CAS
. - M. Uçurum and A. Özdemir, Powder Technol., 2024, 119970 CrossRef
. - Z. Liu, H. Onay, F. Guo, J. Chen, L. Poltorak, P. Hedayati and E. J. Sudholter, Energy Fuel, 2021, 35(2), 1358–1370 CrossRef CAS
. - N. Hu, E. Tang, D. Chang, S. Liu, X. Chu, X. Xing, R. Wang and X. Liu, Powder Technol., 2021, 394, 83–91 CrossRef CAS
. - C.-Y. Chen, Microscale Transport Phenomena and Interfacial Reactions in Diverse Applications, Tulane University, 2021 Search PubMed
. - F. Ippolito, G. Hübner, T. Claypole and P. Gane, Processes, 2021, 9(6), 937 CrossRef CAS
. - Y.-S. Hsieh, C.-Y. Chen, H.-Y. Lo, C.-Y. Lin and Y.-C. Cheng, IEEE Trans. Plasma Sci., 2022, 51(2), 303–310 Search PubMed
. - A. A. Yassene, E. H. Awad and A. A. Hegazy, Radiochim. Acta, 2024, 112(5), 339–350 CrossRef CAS
. - A. F. Ehsan and Ö. Toraman, Ömer Halisdemir Üniv. Mühendis. Bilim., 2024, 13(3), 760–765 Search PubMed
. - N. Shimpi, A. Mali, D. Hansora and S. Mishra, Nanosci. Nanoeng., 2015, 3(1), 8–12 CAS
. - P. Cheng, L. Yang, Y. Xie and Y. Liu, Mater, 2023, 16(10), 3788 CrossRef CAS PubMed
. - M. Abeywardena, M. Yashomala, R. Elkaduwe, D. Karunaratne, H. Pitawala, R. Rajapakse, A. Manipura and M. Mantilaka, Colloids Surf., A, 2021, 629, 127397 CrossRef CAS
. - Z. Zeng, Y. Li, C. Wang and P. Zhao, J. Wuhan Univ. Technol., Mater. Sci. Ed., 2020, 35(6), 1169–1176 CrossRef CAS
. - T. Feng, Y. Li, Y. Pang, L. Fang and Z. Chen, J. Thermoplast. Compos. Mater., 2023, 36(6), 2623–2642 CrossRef CAS
. - A. Karydas, H. Brecoulaki, B. Bourgeois, P. Jockey, and C. Zarkadas, Non-destructive examination of Cultural Objects—Recent Advances in X-ray Analysis, Proceedings of the 28th International Symposium on the Conservation and Restoration of Cultural Property, Tokyo, Japan, 2004 Search PubMed
. - C. Parlangeau, A. Dimanov and S. Hallais, Geosci., 2022, 12(6), 233 CrossRef CAS
. - Y. Liang, S. Sun, H. Ding and X. Hou, Surf. Rev. Lett., 2020, 27(10), 1950224 CrossRef CAS
. - J. Lu, X. Cong, Y. Li, Y. Hao and C. Wang, J. Cleaner Prod., 2018, 180, 728–734 CrossRef CAS
. - C. Pechyen and S. Ummartyotin, Polym. Bull., 2017, 74(2), 431–444 CrossRef CAS
. - I. Acar and O. Acisli, Appl. Surf. Sci., 2018, 457, 208–213 CrossRef CAS
. - H. Dang, Z. Xu, Z. Chen, W. Wu, J. Feng, Y. Sun, F. Jin, J. Li and F. Ge, Cryst. Res. Technol., 2019, 54(5), 1800243 CrossRef
. - G. Hong, K. Song and Y.-W. Lee, J. Supercrit. Fluids, 2021, 169, 105102 CrossRef CAS
. - Y. Ji, X. Rong, H. Zhong, Y. Wang, S. Wang and L. Lu, J. Nanomater, 2017, 1, 6501793–6501799 Search PubMed
. - A. Evcin, S. Akpinar, B. Ersoy, M. Özgül, Z. Ö. Yazici and M. Uçar, Int. J. Chem. Technol., 2019, 26, 270–273 CAS
. - G. K. Latinwo, O. R. Ogunleye, S. E. Agarry, E. O. Dada and I. A. Tijani, Int. J. Compos. Mater., 2018, 8(4), 91–96 CAS
. - Y. Wong, L. Tong, Y. Hu and P. Wu, Mater. Trans., 2016, 57(12), 2127–2131 CrossRef CAS
. - A. Nawar, H. Ghaedi, M. Ali, M. Zhao, N. Iqbal and R. Khan, Process Saf. Environ. Prot., 2019, 132, 214–225 CrossRef CAS
. - Z. Jiang, J. Wang, R. Ge and C. Wu, Polym. Bull., 2018, 75(3), 1123–1139 CrossRef CAS
. - A. Abdi, R. Eslami-Farsani and H. Khosravi, Fibers Polym., 2018, 19(3), 635–640 CrossRef CAS
. - E. Wondu, Z. C. Lule and J. Kim, Polym., 2020, 12(4), 747 CAS
. - S. Shahbakhsh, E. Tohidlou and H. Khosravi, J. Text. Inst., 2020, 111(4), 550–554 CrossRef CAS
. - J. Lee, S. H. Jo and J. Lim, J. Ind. Eng. Chem., 2019, 74, 63–70 CrossRef CAS
. - J. H. Kim, J. H. Ahn, J. S. Hong and K. H. Ahn, Korea Aust. Rheol. J., 2020, 32(1), 29–39 CrossRef
. - M. Rahmani, F. A. Ghasemi and G. Payganeh, Mech. Ind., 2014, 15(1), 63–67 CrossRef CAS
. - Z. Cao, M. Daly, L. Clémence, L. M. Geever, I. Major, C. L. Higginbotham and D. M. Devine, Appl. Surf. Sci., 2016, 378, 320–329 CrossRef CAS
. - C. W. Jeon, S. Park, J.-H. Bang, S. Chae, K. Song and S.-W. Lee, Coat., 2018, 8(1), 43 CrossRef
. - J. Kim, S. K. Bea, Y. H. Kim, D.-W. Kim, K.-Y. Lee and C.-M. Lee, Biotechnol. Bioprocess Eng., 2015, 20(4), 794–799 CrossRef CAS
. - A. Pradittham, N. Charitngam, S. Puttajan, D. Atong and C. Pechyen, Energy Procedia, 2014, 56, 264–273 CrossRef CAS
. - A. Sarkar, A. K. Ghosh and S. Mahapatra, J. Mater. Chem., 2012, 22(22), 11113–11120 RSC
. - S.-Y. Jung, H. Hwang, H.-S. Jo, S. Choi, H.-J. Kim, S.-E. Kim and K. Park, Int. J. Mol. Sci., 2021, 22(9), 4614 CrossRef CAS PubMed
. - Z. Wu, Z. Zhang and K. Mai, J. Therm. Anal. Calorim., 2020, 139(2), 1111–1120 CrossRef CAS
. - E. Wondu, Z. C. Lule and J. Kim, Mater. Chem. Phys., 2021, 267, 124624 CrossRef CAS
. - F. Ippolito, G. Hübner, T. Claypole and P. Gane, Polym., 2020, 12(6), 1295 CAS
. - A. E. M. A. Abou elfettouh, A. A. A. Haroun, A.-G. M. Rabie, G. A. M. Ali and M. Y. M. Abdelrahim, Turk. J. Chem., 2019, 43(3), 750–759 CrossRef
. - S. Bahlouli, A. Makhlouf and N. Haddaoui, Int. J. Polym. Anal. Charact., 2021, 26(5), 440–457 CrossRef CAS
. - P. A. Zapata, H. Palza, B. Díaz, A. Armijo, F. Sepúlveda, J. A. Ortiz, M. P. Ramírez and C. Oyarzún, Mol., 2018, 24(1), 126 CrossRef PubMed
. - Y. Liang, K. Yu, Q. Zheng, J. Xie and T.-J. Wang, Appl. Surf. Sci., 2018, 436, 832–838 CrossRef CAS
. - H. Fan, P. Liu, J. Liu and B. Xu, BioResources, 2015, 10(3), 5655–5665 CrossRef CAS
. - Z. Wu, Z. Zhang and K. Mai, J. Thermoplast. Compos. Mater., 2020, 33(4), 464–476 CrossRef CAS
. - R. Raj, Y. Morozov, L. Calado, M. Taryba, R. Kahraman, R. Shakoor and M. Montemor, Corros. Sci., 2020, 167, 108548 CrossRef CAS
. - D. Kim, J. Lee, S. Lee and J. Lim, Colloids Surf., A, 2018, 536, 213–223 CrossRef CAS
. - W. Liu, J. Li, X. Huang and J. Bi, Mater, 2021, 14(8), 1982 CrossRef CAS PubMed
. - J. Wang, Z. Fan, S. Li, X. Liu, X. Shen and F. Su, Polym. Adv. Technol., 2017, 28(12), 1892–1901 CrossRef CAS
. - Y. Yang, C. Zhang and Y. Weng, Polym. Test., 2021, 102, 107334 CrossRef CAS
. - T. Zhang, C. Zhang, Y. Yang, F. Yang, M. Zhao and Y. Weng, J. Appl. Polym. Sci., 2021, 138(38), 50970 CrossRef CAS
. - Ö. Y. Toraman, O. Ersoy and H. Köse, in Proceedings from: 6th International Conference on Materials Science and Nanotechnology for Next Generation, 2019, pp. 241–244 Search PubMed
. - C. Shen, R. Li, J. Pei, J. Cai, T. Liu and Y. Li, Appl. Sci., 2019, 10(1), 91 CrossRef
. - C. Han, Y. Hu, K. Wang and G. Luo, Powder Technol., 2019, 356, 414–422 CrossRef CAS
. - S. K. Nemani, R. K. Annavarapu, B. Mohammadian, A. Raiyan, J. Heil, M. A. Haque, A. Abdelaal and H. Sojoudi, Adv. Mater. Interfaces, 2018, 5(24), 1801247 CrossRef
. - K. Piekarska, E. Piorkowska and J. Bojda, Polym. Test., 2017, 62, 203–209 CrossRef CAS
. - K.-H. Nam, J. Seo, K. Seo, W. Jang and H. Han, Macromol. Res., 2014, 22(6), 669–677 CrossRef CAS
. - A. Barhoum, H. Rahier, R. E. Abou-Zaied, M. Rehan, T. Dufour, G. Hill and A. Dufresne, ACS Appl. Mater. Interfaces, 2014, 6(4), 2734–2744 CrossRef CAS PubMed
. - H. Fan, X. Wang, J. Liu and B. Xu, BioResources, 2016, 11(1), 957–964 CAS
. - T. Guo, L. Wang, A. Zhang and T. Cai, J. Appl. Polym. Sci., 2005, 97(3), 1154–1160 CrossRef CAS
. - Y.-s. Ding, H. Baoquan, X. Zhai and F. Lu, Funct. Mater., 2018, 25(3), 564–567 CrossRef CAS
. - K. Roy, M. Alam, S. K. Mandal and S. C. Debnath, J. Sol-Gel Sci. Technol., 2015, 73(2), 306–313 CrossRef CAS
. - M. Atif, S. Asrar Ahmad, A. Ghani, A. Mahmood and R. Bongiovanni, Appl. Surf. Sci., 2020, 508, 145281 CrossRef CAS
. - M. Atif, M. R. Abdul Karim, I. Ali and R. Bongiovanni, Compos. Interfaces, 2020, 27(6), 617–630 CrossRef CAS
. - M. Atif, R. Bongiovanni, M. Giorcelli, E. Celasco and A. Tagliaferro, Appl. Surf. Sci., 2013, 286, 142–148 CrossRef CAS
. - A. Ergün, Constr. Build. Mater., 2011, 25(2), 806–812 CrossRef
. - J. Jin, Z. Huang, Y. Xie, Z. Shen, B. Liu and H. Chen, Commun. Mater., 2024, 5(1), 64 CrossRef CAS
. - A. J. López, A. Ramil, J. S. Pozo-Antonio, T. Rivas and D. Pereira, Sustainability, 2019, 11(15), 4079 CrossRef
. - T. Li, S. Zeng, Y. Ji, B. Shen, Z. Wang, H. Zhong and S. Wang, Appl. Sci., 2019, 9(13), 2619–2629 CrossRef CAS
. - A. Buasri, N. Chaiyut, K. Borvornchettanuwat, N. Chantanachai and K. Thonglor, J. Mater. Metall. Eng., 2012, 6(8), 695–698 Search PubMed
. - H. U. Zaman, M. A. Khan, R. A. Khan and M. D. H. Beg, J. Thermoplast. Compos. Mater., 2014, 27(12), 1701–1710 CrossRef
. - A. Khan, R. Patidar and A. Pappu, Constr. Build. Mater., 2021, 269, 121229 CrossRef CAS
. - I. Kemal, A. Whittle, R. Burford, T. Vodenitcharova and M. Hoffman, Polymer, 2009, 50(16), 4066–4079 CrossRef CAS
. - N. Fernando and N. L. Thomas, J. Vinyl Addit. Technol., 2007, 13(2), 98–102 CrossRef CAS
. - H. Liu, L. Dong, H. Xie, L. Wan, Z. Liu and C. Xiong, J. Appl. Polym. Sci., 2013, 127(4), 2749–2756 CrossRef CAS
. - A. M. Atta, H. A. Al-Lohedan, A. O. Ezzat and S. A. Al-Hussain, Prog. Org. Coat., 2016, 101, 577–586 CrossRef CAS
. - S. El-Sherbiny, S. M. El-Sheikh and A. Barhoum, Powder Technol., 2015, 279, 290–300 CrossRef CAS
. - Z. Hu, X. Zen, J. Gong and Y. Deng, Colloids Surf., A, 2009, 351(1–3), 65–70 CrossRef CAS
. - F.-L. Jin and S.-J. Park, Mater. Sci. Eng., A, 2008, 478(1–2), 406–408 CrossRef
. - Z. Yang, Y. Tang and J. Zhang, Chalcogenide Lett., 2013, 10(4), 131 CAS
. - A. A. Aliabdo, A. E. M. Abd Elmoaty and E. M. Auda, Constr. Build. Mater., 2014, 50, 28–41 CrossRef
. - S. Mallakpour and E. Khadem, Ultrason. Sonochem., 2017, 39, 430–438 CrossRef CAS PubMed
. - M. A. El-Mandouh, J.-W. Hu, A. S. Mohamed and A. S. Abd El-Maula, Mater, 2022, 15(20), 7125 CrossRef CAS PubMed
. - A. Amani, A. Babazadeh, A. Sabohanian and A. Khalilianpoor, Int. J. Pavement Eng., 2021, 22(12), 1531–1540 CrossRef CAS
. - C. Lizandara-Pueyo, X. Fan, C. Ayats and M. A. Pericàs, J. Catal., 2021, 393, 107–115 CrossRef CAS
.
|
This journal is © The Royal Society of Chemistry 2024 |
Click here to see how this site uses Cookies. View our privacy policy here.