DOI:
10.1039/D4RA07179D
(Paper)
RSC Adv., 2024,
14, 35644-35649
Syntheses of optically active monapterin, 7,8-dihydromonapterin, and 5,6,7,8-tetrahydromonapterin from L-xylose†
Received
5th October 2024
, Accepted 22nd October 2024
First published on 8th November 2024
Abstract
We describe the syntheses of monapterin, dihydromonapterin and tetrahydromonapterin in optically active forms. The syntheses involved the condensation of L-xylose with phenylhydrazine, providing a hydrazone derivative. The reaction of the resulting hydrazone with triamino-pyrimidinone followed by oxidation of the resulting pteridinone with molecular oxygen furnished pterin containing a hydroxylated side chain. Hydrogenation of the pterin derivatives over RANEY® Ni catalyst afforded dihydromonapterin and tetrahydromonapterin in optically active forms. We also investigated an alternative route involving an Amadori rearrangement, followed by the Polonovski–Boon reaction as the key step to make these monapterin derivatives.
Introduction
Pterins are nitrogen-rich fused bicyclic biomolecules widely produced across all domains of life.1,2 They play critical roles in diverse biological processes, including cell signaling, modulation of the immune system, pigmentation and metabolism.3–5 Xanthopterin (1, Fig. 1) was first discovered in the late nineteenth century from the yellow and blue fluorescent pigments of butterfly wings. Later, xanthopterin was found in gastrointestinal endocrine cells.6,7 Pteridine rings are biosynthesized from guanosine triphosphate (GTP), and they are key building blocks for many biomolecules, including folic acid 2.8,9 Pterins with hydroxylated side chains exist in the fully oxidized form as well as partially reduced and fully reduced forms. In the human immune system, oxidized pterins have been demonstrated to modulate the oxidative burst of reactive oxygen and nitrogen species.10,11 Biopterin 3, a 6-(D-erythro-1,2-dihydroxypropyl) pterin, is a representative member of the family of biopterins. Biopterins exist in many different reduction states. They are endogenous enzyme cofactors for a family of aromatic amino acid hydroxylases involved in the synthesis of neurotransmitters, including dopamine, epinephrine, norepinephrine, and serotonin.12,13 Biopterin derivatives are also utilized as cofactors in the synthesis of nitric oxide.14 Monapterin, also known as neopterin 4, a 6-(D-erythro-1,2,3-trihydroxypropyl) pterin, is ubiquitous in insects, bacteria, and mammals. It is found in high levels, particularly in cerebrospinal fluid and plasma. Also, the level surges upon infection and during immune system activation.15,16 Neopterin is viewed as an early biomarker for diseases associated with the activation of cell-mediated immunity.17,18
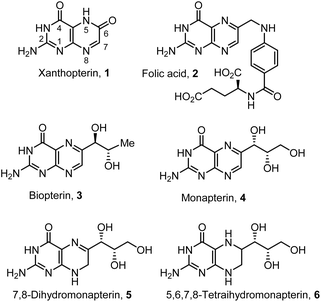 |
| Fig. 1 Structures of bioactive pterin derivatives 1–6. | |
Pterins serve as critical cofactors in eukaryotic redox enzymes and in many bacterial systems.1,19 In the model pathogen Agrobacterium tumefaciens, the pteridine reductase PruA catalyzes the nicotinamide adenine dinucleotide phosphate hydrogen (NADPH)-dependent reduction of 7,8-dihydromonapterin 5 to 5,6,7,8-tetrahydromonapterin 6.20,21 Recently, we reported mechanistic insights into a complex regulatory system discovered in A. tumefaciens that controls biofilm formation depending upon excreted pterins.22 This pathway is conserved among a wide range of Gram-negative bacteria, including pathogens of animals and plants. These studies may lead to effective treatment modalities for biofilms.23,24 As part of our studies on the mechanism for biofilm regulation in A. tumefaciens, we required both dihydromonapterin and tetrahydromonapterin for our studies. Commercial supplies of specific hydroxylated pterins are very limited. Also, the practical syntheses as well as purification protocols of these very polar biomolecules have not been reported.25–27 Herein, we report the syntheses of monapterin, 7,8-dihydromonapterin, and 5,6,7,8-tetrahydromonapterin in optically active forms using commercially available L-xylose.
Results and discussion
The synthesis of monapterin is shown in Scheme 1. First, L-xylose phenyl hydrazone was prepared by the condensation of phenyl hydrazine and L-xylose in ethanol at 20 °C in the presence of a catalytic amount of acetic acid to provide 8 in a 99% yield. Interestingly, the treatment of L-xylose with phenyl hydrazine hydrochloride salt in ethanol at 23 °C for 3 h furnished (phenylhydrazinyl)pentan-2-one 9 in a 69% yield. Pterins are generally synthesized using the Viscontini reaction with amino pyrimidinone and sugar hydrazone.1,28 Therefore, commercially available 2,5,6-triaminopyrimidine-4(1H)-one sulfate 10 was treated with L-xylose phenyl hydrazone 8 in MeOH in the presence of 5 N HCI solution and a catalytic amount of mercaptoethanol at 23 °C. The resulting reaction mixture was heated at reflux for 3 h to provide a mixture (1
:
1) of tetrahydropyrano pteridine 11 and tetrahydrofurano pteridine 12 derivatives.28,29 Similarly, the reaction of (phenylhydrazinyl)pentan-2-one 9 with 2,5,6-triaminopyrimidine-4(1H)-one sulfate 10 provided the pteridine derivatives 11 and 12. These pteridine derivatives 11 and 12 are quite unstable as they are prone to autoxidation. Therefore, these compounds were not separated, and the mixture was dissolved in a pH 10 buffer and the resulting solution was stirred at 23 °C under a balloon filled with oxygen for 20 h. Acidification followed by evaporation of the solvents provided a crude product, which was recrystallized using a mixture (1
:
10) of methanol and ether to furnish monapterin 4 in a 66% isolated yield.
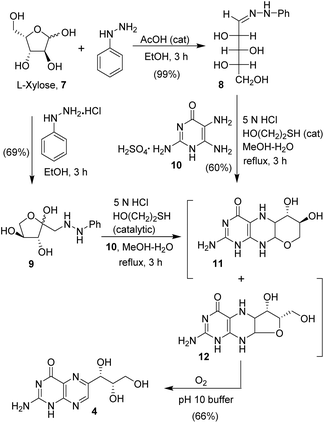 |
| Scheme 1 Synthesis of monapterin 4. | |
Monapterin 4 was converted to dihydromonapterin 5 and tetrahydromonapterin 6 as shown in Scheme 2. We investigated the selective reduction of monapterin to dihydromonapterin under a variety of hydrogenation conditions, as summarized in Table 1. This hydrogenation requires careful monitoring otherwise the overreduction product tetrahydromonapterin 6 becomes a major by-product. The catalytic hydrogenation of monapterin 4 with Pearlman's catalyst (100% by weight) in NaH2PO4 buffer (pH 5.7) under a hydrogen-filled balloon at 23 °C for 2 h resulted in a mixture (30
:
70 by HPLC analysis) of dihydromonapterin 5 and fully reduced tetrahydromonapterin 6 in a 56% yield (entry 1). The hydrogenation of 4 with 10% Pd–C (100% by weight) in pH 5.7 buffer for 4 h improved the selectivity and the reaction mixture (70
:
30) showed more of the desired dihydromonapterin 5 in a combined yield of 63% (entry 2). Reducing the time to 1.3 h led to improvements in the yield and selectivity, providing a 95
:
5 mixture of 5 and 6 in a 79% yield (entry 3). We then examined hydrogenation over the RANEY® Ni catalyst. The hydrogenation of 4 over RANEY® Ni (W2, 100% by weight) in pH 5.7 buffer under a hydrogen-filled balloon at 23 °C for 2 h furnished dihydromonapterin 5 in a highly selective manner (98
:
2 ratio) in an 88% yield. Only a trace amount of the fully reduced tetrahydromonapterin 6 was formed during this reaction (entry 4). This optimized procedure was utilized for the synthesis of dihydromonapterin for our biological studies.22 The hydrogenation of 4 in EtOH after 2 h resulted in only a trace amount of product, and mainly unreacted monapterin remained (entry 5). We also investigated hydrogenation using PtO2 and 5% Rh/Al2O3 as the catalysts. In both cases, there were hardly any hydrogenation products, mostly the starting monapterin remained after the work up (entries 6 and 7). For the synthesis of tetrahydromonapterin 6, monapterin 4 was hydrogenated over Pd on C (100% by weight) in NaH2PO4 buffer (pH 5.7) at 23 °C for 8 h to furnish tetrahydromonapterin 6 in a 75% yield.
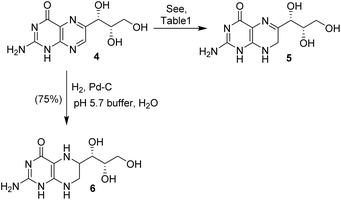 |
| Scheme 2 Syntheses of dihydromonapterin and tetrahydromonapterin. | |
Table 1 Hydrogenation of monapterin 4 under various conditionsa
Entry |
Catalysts (wt%) |
Solvent |
Time (h) |
Yieldb (%) |
Ratioc (5 : 6) |
Reactions were carried out in a 0.1 mmol scale at 23 °C. Combined yields after purification by HPLC. Ratios determined by HPLC and LCMS analysis of the crude products. |
1 |
20% Pd(OH)2 |
pH 5.7 buffer |
2 |
56 |
30 : 70 |
2 |
10% Pd/C |
pH 5.7 buffer |
4 |
63 |
70 : 30 |
3 |
10% Pd/C |
pH 5.7 buffer |
1.3 |
79 |
95 : 5 |
4 |
Ra–Ni (W2) (25%) |
pH 5.7 buffer |
2 |
88 |
98 : 2 |
5 |
Ra–Ni (W2) (125%) |
EtOH |
2 |
Trace |
— |
6 |
PtO2 (25%) |
THF |
6 |
Trace |
— |
7 |
5% Rh/Al2O3 |
EtOH |
9 |
Trace |
— |
8 |
10% Pd/C |
pH 5.7 buffer |
8 |
75 |
1 : 99 |
We also explored an alternative route to monapterin and dihydromonapterin due to issues of overreduction. Andrews and co-workers reported the synthesis of biopterin using L-arabinose as the starting sugar.30 In a modified synthesis, as shown in Scheme 3, L-xylose was reacted with dibenzylamine in ethanol in the presence of an equivalent amount of acetic acid at 70 °C for 6 h. This condition resulted in the Amadori rearrangement product,31 α-ketobenzylamine derivative 13 in a 70% yield. The catalytic hydrogenolysis of ketoamine 13 with 10% Pd–C under a hydrogen-filled balloon in a mixture (4
:
1) of ethanol and 1 N HCl at 23 °C for 48 h furnished the corresponding amine in an excellent yield. The resulting amino ketone was condensed with 2-amino-6-chloro-5-nitropyrimidinone 14 in ethanol at 50 °C for 30 min. Then, the addition of NaHCO3 followed by heating the resulting mixture in a (3
:
1) mixture of ethanol and water at 60 °C for 1 h resulted in the nitro derivative 15 in a 42% yield over 2 steps. Exposure of the nitro derivative 15 to RANEY® Ni under a hydrogen-filled balloon in water at 23 °C for 2 h furnished 7,8-dihydromonapterin 4 in an 80% yield. The oxidation of dihydromonapterin with MnO2 in water for 3 h afforded monapterin 5 in a 60% yield. Overall, the route is straight forward and provided convenient access to dihydromonapterin 5 and monapterin 4 in good yields.
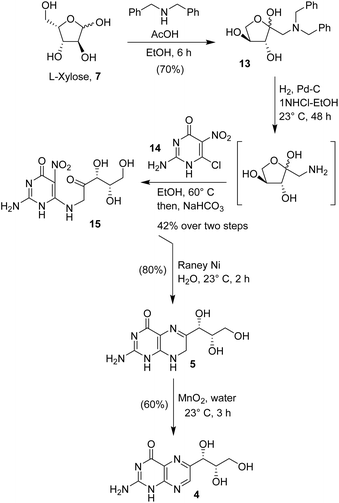 |
| Scheme 3 Syntheses of dihydromonapterin and monapterin. | |
Conclusions
In summary, we describe the practical syntheses of monapterin, 7,8-dihydromonapterin, and 5,6,7,8-tetrahydromonapterin in optically active forms using commercially available L-xylose as the starting material. The synthesis of dihydromonapterin involved the selective hydrogenation of monapterin. We investigated a number of catalytic systems and solvents to obtain optimum results. Catalytic hydrogenation with RANEY® Ni in pH 5.7 buffer under a hydrogen-filled balloon provided the best results. The product dihydromonapterin is highly sensitive to air and it underwent spontaneous autooxidation to provide monapterin along with a small amount of decomposition product. We investigated the stability of dihydromonapterin in D2O under atmospheric conditions. It turns out that dihydromonapterin completely converted to monapterin within 24 h at 23 °C in D2O. The current synthetic route provides access to both dihydro- and tetrahydro monapterin for biological studies. We also developed an alternate route to avoid overreduction, as shown in Scheme 3, in which L-xylose was converted to the α-ketobenzylamine derivative 13 by using Amadori rearrangement followed by Polonovski–Boon cyclization32 to afford the nitro derivative 15. RANEY® Ni reduction of the nitro compound afforded dihydromonapterin, and then further oxidation with MnO2 gave monapterin in a more efficient manner. Further work involving monapterin is ongoing in our laboratories.
Experimental section
All the reactions were carried out under an inert atmosphere, in either nitrogen or argon, using a magnetic stirrer and oven-dried glassware. All the solvents were anhydrous and distilled prior to use. Dichloromethane and triethylamine were distilled from calcium hydride. Tetrahydrofuran, diethyl ether, and benzene were distilled from sodium and benzophenone. All the other solvents were HPLC grade or better. Flash column chromatography was performed using EM Science 60–200 mesh silica gel. Thin-layer chromatography was performed using 60 F-254 E. Merck silica gel plates. 1H- and 13C-NMR were recorded using Bruker AV-500, Avance DRX-500, Varian Mercury-Vx-300, and Gemini-2300 spectrometers with Me4Si as an internal standard. Optical rotations were recorded on a PerkinElmer 341 polarimeter. A Thermo Finnigan LCQ Classic mass spectrometer was used for the MS analyses. The purity of test compounds was determined by HRMS and HPLC analyses. All the test compounds showed ≥95% purity.
Experimental procedures
(2S,3S,4R,Z)-5-(2-Phenylhydrazineylidene)pentane-1,2,3,4-tetraol (8). To a stirred solution of phenylhydrazine (453 mg, 4.2 mmol) and L-xylose 7 (420 mg, 2.8 mmol) in ethanol (10 mL),two drops of acetic acid were added, and the mixture was stirred at 23 °C for 3 h. After this period, the reaction mixture was concentrated under reduced pressure. The residue was diluted with diethyl ether (20 mL) and the resulting precipitate was collected by filtration and the filter cake was washed with ether to provide the title compound 8 (668 mg, 99%) as a brown solid. [α]D20 = +4.5 (c 0.66, MeOH). 1H NMR (400 MHz, methanol-d4) δ 7.19–7.11 (m, 3H), 7.00–6.94 (m, 2H), 6.72 (dt, J = 7.3, 1.2 Hz, 1H), 4.37 (t, J = 5.7 Hz, 1H), 3.78 (ddd, J = 6.4, 5.3, 3.2 Hz, 1H), 3.71 (dd, J = 5.7, 3.2 Hz, 1H), 3.69–3.60 (m, 2H). 13C NMR (100 MHz, methanol-d4) δ 145.5, 138.4, 128.5, 118.6, 111.8, 72.4, 72.1, 71.6, 62.9. LRMS-ESI (m/z): 241.1 [M + H]+; HRMS-ESI (m/z): [M + H]+ calcd for C11H17N2O4, 241.1183; found 241.1184.
(3R,4S)-3,4,5-Trihydroxy-1-(2-phenylhydrazineyl)pentan-2-one (9). To a stirred solution of phenyl hydrazine hydrochloride (1.44 g, 10.0 mmol) and L-xylose 7 (1 g, 6.6 mmol) in ethanol (25 mL), five drops of acetic acid were added. The resulting mixture was stirred at 23 °C for 3 h. After this period, the reaction mixture was concentrated under reduced pressure. The residue was diluted with diethyl ether (50 mL). The resulting precipitate was collected by filtration and the filter cake was washed with ether to provide the title compound 9 as a mixture of anomers (1.1 g, 69%) as a grey solid. 1H NMR (400 MHz, MeOD) δ 7.33 (dd, J = 8.6, 7.4 Hz, 2H), 7.12–6.95 (m, 3H), 5.04 (d, J = 3.6 Hz, 1H), 3.76–3.60 (m, 1H), 3.54 (dd, J = 10.9, 5.3 Hz, 1H), 3.51–3.42 (m, 1H), 3.36 (dd, J = 9.1, 3.6 Hz, 1H), 3.30 (p, J = 1.6 Hz, 1H).13C NMR (100 MHz, methanol-d4) δ 144.8, 129.1, 122.5, 114.6, 97.5, 92.6, 76.5, 74.7, 73.4, 72.4, 70.1, 69.9, 65.6, 61.2. LRMS-ESI (m/z): 241.1 [M + H]+.
(6S,7S)-2-Amino-6,7-dihydroxy-1,5,5a,6,7,8,9a,10-octahydro-4H-pyrano[3,2-g] pteridin-4-one. To a stirred mixture of 2,5,6-triaminopyrimidin-4(1H)-one sulfate (540 mg, 2.26 mmol) and L-xylose phenylhydrazone 8 (595 mg, 2.48 mmol) in aqueous methanol (50%, 30 mL), 5 N HCl (1.4 mL) was added and mercaptoethanol (3 drops) under nitrogen. The resulting mixture was heated at 100 °C for 2–3 h. After this period, the reaction mixture was cooled to 23 °C. The reaction was concentrated under reduced pressure. The resulting gummy residue was dissolved in MeOH (2 mL). The reaction mixture was diluted with diethyl ether (30 mL). The resulting precipitate was collected by filtration and the filter cake was washed with ether to provide a mixture of compounds (11, 12) (400 mg, 69%). The compounds were used in the following reaction without further purification.
2-Amino-6-((1S,2S)-1,2,3-trihydroxypropyl) pteridin-4(1H)-one (4). A suspension of the above mixture of compounds (100 mg) in pH 10 buffer (10 mL) was covered with aluminium foil. To this suspension, a stream of oxygen was bubbled through for 5 h. After this period, the reaction mixture was stirred under an oxygen atmosphere until the starting material completely disappeared. The mixture was neutralized with acetic acid (adjusted to pH 6). The mixture was concentrated to dryness. The oily residue was diluted with MeOH. The resulting solid compound was diluted with (1
:
1) MeOH and diluted with diethyl ether (15 mL). The resulting precipitate was collected by filtration and the filter cake was washed with diethyl ether to provide a brown solid. Further purification of the compound was carried out using Pre HPLC-Agilent 1260 infinity (YMC PAK ODS-A C18, 2% ACN/H2O, 10 mL min−1, tr: 6.175, conc. 10 mg/1 mL of H2O) to provide the desired compound monapterin (10 mg, 60%). [α]D20 = +125.8 (c 0.5, DMSO). 1H NMR (500 MHz, DMSO-d6) δ 11.4 (br, 1H), 8.73 (s, 1H), 6.99–6.65 (br, 2H), 5.40 (s, 1H), 4.72 (s, 1H), 4.65–4.53 (m, 2H), 3.64 (m, 1H), 3.55–3.46 (m, 2H). 13C NMR (125 MHz, DMSO-d6) δ 164.8, 157.1, 157.0, 151.8, 148.5, 127.7, 74.6, 72.6, 62.9. LRMS ESI (m/z): 254.0 [M + H]+; HRMS-ESI (m/z): [M + H]+ calcd for C9H12N5O4Na, 276.07032; found 276.07062.
2-Amino-6-((1S,2S)-1,2,3-trihydroxypropyl)-7,8-dihydropteridin-4(1H)-one (5). To a solution of compound 4 (20 mg, 0.08) dissolved in aqueous NaH2PO4 (2 mL, prepared from 96 mg of NaH2PO4·H2O dissolved in 100 mL water), RANEY® Ni (20 mg) was added. The reaction mixture was stirred under a hydrogen-filled balloon for 2 h. After this period, the catalyst was filtered off and the filter cake containing the catalyst was washed with water. The water solution was concentrated under reduced pressure and in an argon environment to provide the title compound 5 as a white solid (15 mg, 75%). [α]D20 = +2.78 (c 0.55, H2O). 1H NMR (500 MHz, D2O) δ 4.19 (s, 1H), 4.14–4.06 (s, 2H), 3.86 (s, 1H), 3.64 (t, J = 7.2 Hz, 1H), 3.59 (d, J = 8.2 Hz, 1H), 1.91 (s, 1H). 13C NMR (126 MHz, D2O) δ 162.6, 156.2, 156.0, 153.3, 101.7, 73.3, 71.7, 62.5, 41.4. LRMS-ESI (m/z): 256.1 [M + H]+; HRMS-ESI (m/z): [M + H]+ calcd for C9H14N5O4, 256.1040; found 256.1041.
2-Amino-6-((1S,2S)-1,2,3-trihydroxypropyl)-5,6,7,8-tetrahydropteridin-4(3H)-one (6). Monapterin compound 4 (6 mg, 0.02 mmol) was dissolved in a mixture of solvents comprising water (1 mL) and 7 mM NaH2PO4·H2O (0.5 mL). The pH of the reaction mixture was set to 5.7 using 1 N HCl. After complete degassing of the reaction mixture using an argon balloon, 10% Pd/C (3.0 mg) was added to the reaction mixture. The resulting mixture was stirred under a hydrogen-filled balloon at 23 °C. The progress of hydrogenation was monitored continuously by LCMS. After completion of the reaction (8 h), the catalyst was filtered off and the filter cake with the catalyst was washed with water. Water solution was evaporated under reduced pressure to afford the tetrahydropteridin-4(3H)-one 6 as a (56
:
44) mixture of diastereomeric compounds based up on integration of the data from 13C NMR (4.5 mg, 75%). 1H NMR (800 MHz, D2O) δ 4.0 (m, 1H), 3.81 (m, 1H), 3.75 (m, 1H), 3.73–3.69 (m, 3H), 3.60–3.56 (m, 7H), 3.49–3.43 (m, 2H). 13C NMR (200 MHz, D2O) δ 165.6, 158.6, 158.3, 154.4, 153.8, 153.6, 85.7, 84.8, 71.2, 71.1, 67.5, 66.4, 61.9, 61.8, 54.3, 53.3, 38.9, 37.8. LRMS-ESI (m/z): 258 [M + H]+; HRMS-ESI (m/z): [M + H]+ calcd for C9H15N5O4H, 258.1202; found 258.1204.
(3R,4S)-2-((Dibenzylamino)methyl)tetrahydrofuran-2,3,4-triol (13). To a solution of dibenzyl amine (650 mg, 3.33 mmole) and L-xylose 7 (500 mg, 3.33 mmole) in EtOH (10 mL), acetic acid (0.2 mL, 3.33 mmole) was added. The reaction mixture was refluxed at 70 °C for 5 h. After completion of the reaction, the solvent was evaporated under reduced pressure to afford a crude residue. Purification of the residue by silica gel chromatography (4% MeOH/CH2Cl2) afforded the title compound 13 as a light-yellow-coloured oil, which existed as a mixture of anomers (770 mg, 70%), Rf = 0.5 (4% MeOH/CH2Cl2); [α]D20 = +6.2 (c 0.9, CH3OH): 1H NMR (500 MHz, CDCl3) δ 7.38–7.24 (m, 10H), 4.36–4.30 (m, 1H), 4.17–4.12 (m, 1H), 4.01–3.88 (m, 1H), 3.86–3.81 (m, 1H), 3.78–3.72 (m, 1H), 3.69 (dd, J = 9.3, 3.6 Hz, 1H), 3.60–3.54 (m, 1H), 3.51–3.40 (m, 1H), 2.88–2.71 (m, 1H).; 13C NMR (125 MHz, CDCl3) δ 209.7, 137.6, 137.4, 129.7, 129.5, 129.4, 128.9, 128.8, 128.7, 128.6, 128.6, 127.7, 127.6, 127.6, 104.1, 102.6, 81.6, 80.0, 77.5, 77.4, 77.3, 77.2, 77.0, 76.9, 76.0, 74.9, 71.5, 71.4, 64.1, 60.3, 59.1, 58.9, 58.9, 57.4, 56.4; LRMS-ESI (m/z): 330 [M + H]+; HRMS-ESI (m/z): [M + H]+ calcd for C19H24NO4, 330.1705; found 330.1699.
2-Amino-5-nitro-6-(((3S,4R)-3,4,5-trihydroxy-2-oxopentyl)amino)pyrimidin-4(3H)-one (15). Compound 13 (500 mg, 1.5 mmol) was dissolved in a mixture of solvents comprising 6.0 mL of EtOH and 1.5 mL of 1 N HCl. After complete degassing of the reaction mixture by using an argon balloon, 10% Pd/C (150 mg) was added to the reaction mixture. The catalytic hydrogenation was performed under vigorous stirring under a hydrogen-filled balloon at rt for 48 h. The reaction was monitored continuously by mass spectrometry. After complete consumption of the starting material, the catalyst was filtered off and washed with EtOH to afford the amine solution. The amine solution was used directly for the next step.The above 2-amino-6-chloro-5-nitropyrimidin-4(3H)-one (100 mg, 0.5 mmol) was dissolved in a mixture of solvents comprising 2.0 mL of EtOH and 0.5 mL of H2O and the reaction mixture was heated at 50 °C for the period of 15 min. After 15 min, the above amine solution was added to the reaction mixture and the reaction mixture was further stirred for 5 min. The reaction mixture was slowly basified by the dropwise addition of aqueous NaHCO3 (300 mg in 3 mL of water) for a period of 45 min and the reaction mixture was further stirred for a period of 1.0 h at 60 °C. The reaction mixture was filtered in a hot condition, and then the filtrate was evaporated off under reduced pressure to afford the crude reside. The crude residues were crystallized in an EtOH
:
H2O (2
:
1) mixture of solvents to afford a light-yellow brown solid compound (200 mg, crude solid). Further purification of the compound was carried out using a Pre HPLC-Agilent 1260 infinity system (YMC PAK ODS-A C18, 2% ACN/H2O, 10 mL min−1, tr: 19.411, conc. 15 mg/1 mL of H2O) to provide compound 15 (60 mg, 42%, over two steps) as a white amorphous solid, which existed as a mixture of anomers. [α]D20 = +19.3 (c 0.3, H2O): 1H NMR (800 MHz, DMSO) δ 9.80 (s, 1H), 9.71 (s, 1H), 9.59 (s, 1H), 8.55 (s, 1H), 8.00 (s, 1H), 5.96 (d, J = 16.6 Hz, 1H), 5.63 (d, J = 4.8 Hz, 1H), 5.42 (m, 1H), 5.27–5.14 (m, 2H), 4.87 (d, J = 26.0 Hz, 1H), 4.73–4.62 (m, 2H), 4.48 (dd, J = 20.7, 4.5 Hz, 1H), 4.18 (s, 1H), 4.10 (d, J = 5.4 Hz, 1H), 3.98–3.88 (m, 2H), 3.82–3.73 (m, 2H), 3.69 (dd, J = 13.6, 5.5 Hz, 1H), 3.61 (dd, J = 8.5, 5.5 Hz, 1H), 3.59 (d, J = 4.4 Hz, 1H), 3.56–3.49 (m, 2H), 3.45 (t, J = 9.4 Hz, 2H), 3.40 (dd, J = 8.9, 4.7 Hz, 1H); 13C NMR (201 MHz, DMSO) δ 209.9, 161.6, 159.7, 159.7, 159.5, 159.2, 111.3, 111.1, 110.9, 105.3, 102.2, 82.4, 78.7, 76.7, 76.4, 75.4, 73.2, 71.2, 70.7, 61.9, 50.4, 47.4, 45.8; LRMS-ESI (m/z): 304 [M + H]+; HRMS-ESI (m/z): [M + H]+ calcd for C9H13N5O7, 304.0893; found 304.0884.
2-Amino-6-((1S,2S)-1,2,3-trihydroxypropyl)-7,8-dihydropteridin-4(3H)-one (5). Compound 15 (15.0 mg, 0.05 mmol) was dissolved in a mixture of solvents comprising 1.0 mL of water and 0.5 mL of 7 mM NaH2PO4·H2O. After complete degassing of the reaction mixture using an argon balloon, RANEY® Ni slurry in water (0.1 mL) was added to the reaction mixture. The catalytic hydrogenation was performed under vigorous stirring under a hydrogen-filled balloon at rt. The reaction was monitored continuously by mass spectrometry. After complete consumption of the starting material after 2 h, the catalyst was filtered off and washed with deoxygenated water. The water was then evaporated off under reduced pressure under an argon environment to afford dihydromonapterin 5 as an amorphous solid (10 mg, 80%). 1H NMR (500 MHz, D2O) δ 4.19 (s, 1H), 4.14–4.06 (s, 2H), 3.86 (s, 1H), 3.64 (t, J = 7.2 Hz, 1H), 3.59 (d, J = 8.2 Hz, 1H), 1.91 (s, 1H). 13C NMR (126 MHz, D2O) δ 162.6, 156.2, 156.0, 153.3, 101.7, 73.3, 71.7, 62.5, 41.4. LRMS-ESI (m/z): 256.1 [M + H]+; HRMS-ESI (m/z): [M + H]+ calcd for C9H14N5O4, 256.1040; found 256.1041.
2-Amino-6-((1S,2S)-1,2,3-trihydroxypropyl)pteridin-4(3H)-one (4). To a stirred solution of dihydromonapterin (20 mg, 0.08 mmol) in H2O (1.5 mL) at room temperature, MnO2 (68 mg, 0.8 mmol) was added, and the reaction mixture was stirred for 3 h at room temperature. The reaction was monitored continuously by mass spectrometry. After complete consumption of the starting material, the catalyst was filtered off and washed with water (5 mL) and 0.1 molar NaOH (1.0 mL). The solvent was then evaporated off under reduced pressure to afford the crude residue. Further purification of the compound was carried out using Pre HPLC-Agilent 1260 infinity (YMC PAK ODS-A C18, 2% ACN/H2O, 10 mL min−1, tr: 6.175, conc. 10 mg/1 mL of H2O) to provide monapterin as a white solid (10 mg, 60%). Further purification of the compound was carried out using Pre HPLC-Agilent 1260 infinity system (YMC PAK ODS-A C18, 2% ACN/H2O, 10 mL min−1, tr: 6.175, conc. 10 mg/1 mL of H2O) to provide the desired compound monapterin (10 mg, 60%). [α]D20 = +125.8 (c 0.5, DMSO). 1H NMR (500 MHz, DMSO-d6) δ 11.4 (br, 1H), 8.73 (s, 1H), 6.99–6.65 (br, 2H), 5.40 (s, 1H), 4.72 (s, 1H), 4.65–4.53 (m, 2H), 3.64 (m, 1H), 3.55–3.46 (m, 2H). 13C NMR (125 MHz, DMSO-d6) δ 164.8, 157.1, 157.0, 151.8, 148.5, 127.7, 74.6, 72.6, 62.9. LRMS ESI (m/z): 254.0 [M + H]+; HRMS-ESI (m/z): [M + H]+ calcd for C9H12N5O4Na, 276.0703; found 276.0706.
Data availability
The authors confirm that the data supporting these studies are available within the article and its ESI.†
Author contributions
A. K. Ghosh (writing original draft, review, editing, supervision, resources, methodology, funding acquisition, formal analysis); A. Sharma (methodology, investigation, data curation); S. Nagam (methodology, investigation, data curation); C. Fuqua (review, editing, formal analysis).
Conflicts of interest
There are no conflicts to declare.
Acknowledgements
This research was supported by the National Institutes of Health (Grants AI150466 and GM120337). The authors would like to thank the Purdue University Center for Cancer Research, which supports the shared NMR and mass spectrometry facilities.
Notes and references
- N. Feirer and C. Fuqua, Pteridine, 2017, 28, 23–36 CrossRef CAS.
- T. Hanaya and H. Yamamoto, IUBMB Life, 2013, 65, 300–309 CrossRef CAS PubMed.
- P. Galland and H. Senger, Photochem. Photobiol., 1988, 48, 811–820 CrossRef CAS.
- C. A. Nichol, G. K. Smith and D. S. Duch, Annu. Rev. Biochem., 1985, 54, 729–764 CrossRef CAS PubMed.
- P. Andrade and M. Carneir, Biol. Lett., 2021, 17, 20210221 CrossRef CAS PubMed.
- J. Ferré, Insects, 2024, 15, 370 CrossRef PubMed.
- B. Wijnen, H. L. Leertouwer, D. G. Stavenga and J. Insect, Physiol, 2007, 53, 1206–1217 CAS.
- A. Kirschning, Angew. Chem., Int. Ed., 2021, 60, 6242–6269 CrossRef CAS PubMed.
- T. D. Ames, D. A. Rodionov, Z. Weinberg and R. R. Breaker, Chem. Biol., 2010, 17, 681–685 CrossRef CAS PubMed.
- C. Murr, B. Widner, B. Wirleitner and D. Fuchs, Curr. Drug Metab., 2002, 3, 175–187 CrossRef CAS PubMed.
- G. Weiss, D. Fuchs, A. Hausen, G. Reibnegger, E. R. Werner, G. Werner-Felmayer, E. Semenitz, M. P. Dierich and H. Wachter, FEBS Lett., 1993, 321, 89–92 CrossRef CAS PubMed.
- H. Fanet, L. Capuron, N. Castanon, F. Calon and S. Vancassel, Curr. Neuropharmacol., 2021, 19, 591–609 CAS.
- E. R. Werner, N. Blau and B. Thöny, Biochem. J., 2011, 438, 397–414 CrossRef CAS PubMed.
- N. S. Kwon, C. F. Nathan and D. J. Stuehr, J. Biol. Chem., 1989, 264, 20496–20501 CrossRef CAS PubMed.
- H. Wachter, D. Fuchs, A. Hausen, G. Reibnegger and E. R. Werner, Adv. Clin. Chem., 1989, 27, 81–141 CAS.
- A. Lindsay, G. Baxter-Parker and S. P. Gieseg, J. Clin. Med., 2019, 8, 1383 CrossRef CAS PubMed.
- D. Fuchs, P. Avanzas, R. Arroyo-Espliguero, M. Jenny, L. Consuegra-Sanchez and J. C. Kaski, Curr. Med. Chem., 2019, 16, 4644–4653 CrossRef PubMed.
- S. K. Pingle, R. G. Tumane and A. A. Jawade, Indian J. Occup. Environ. Med., 2008, 12, 107–111 CrossRef PubMed.
- A. Pribat, I. K. Blaby, A. Lara-Núnez, J. F. Gregory III, V. de Crécy-Lagard and A. D. Hanson, J. Bacteriol., 2010, 192, 475–482 CrossRef CAS PubMed.
- M. Labine, L. DePledge, N. Feirer, J. Greenwich, C. Fuqua and K. D. Allen, J. Bacteriol., 2020, 202, e00098 CrossRef CAS PubMed.
- K. L. Kavanagh, H. Jörnvall, B. Persson and U. Oppermann, Cell. Mol. Life Sci., 2008, 65, 3895–3906 CrossRef CAS PubMed.
- L. Greenwich, J. L. Eagan, N. Feirer, K. Boswinkle, G. Minasov, L. Shuvalova, N. L. Inniss, J. Raghavaiah, A. K. Ghosh, K. J. F. Satchell, K. D. Allen and C. Fuqua, Proc. Natl. Acad. Sci. U. S. A., 2024, 121, e2319903121 CrossRef PubMed.
- O. Ciofu, C. Moser, P. Ø. Jensenand and N. Høiby, Nat. Rev. Microbiol., 2022, 20, 621–635 CrossRef CAS PubMed.
- L. Hall-Stoodley, J. W. Costerton and P. Stoodley, Nat. Rev. Microbiol., 2004, 2, 95–108 CrossRef CAS PubMed.
- K. J. Colston and P. Basu, Molecules, 2022, 27, 3324 CrossRef CAS PubMed.
- Biochemical and Clinical Aspects of Pteridines. Cancer Immunology Metabolic Diseases, ed. R. Soyka and W. Pfleiderer, Walter de Gruyter, Berlin, 1985, vol. 4, pp. 33–43 Search PubMed.
- W. Pfleiderer, in Folates and Pterins, ed. R. L. Blakley and S. J. Benkovic, Wiley, New York, 1985, vol. 2, pp. 43–114 Search PubMed.
- M. Viscontini, R. Provenzale, S. Ohlgart and J. Mallevialle, Helv. Chim. Acta, 1970, 53, 1202–1207 CrossRef CAS.
- R. Soyka, W. Pfleiderer and R. Prewo, Helv. Chim. Acta, 1990, 73, 808–826 CrossRef CAS.
- K. J. Andrews, W. E. Barber and B. P. Tong, J. Chem. Soc. C, 1969, 6, 928–930 RSC.
- J. E. Hodge, Adv. Carbohydr. Chem., 1955, 10, 169–205 CAS.
- W. R. Boon, W. G. M. Jones and G. R. Ramage, J. Chem. Soc., 1951, 21, 96–102 RSC.
|
This journal is © The Royal Society of Chemistry 2024 |
Click here to see how this site uses Cookies. View our privacy policy here.