DOI:
10.1039/D3SC06409C
(Edge Article)
Chem. Sci., 2024,
15, 1318-1323
A four-component reaction to access 3,3-disubstituted indolines via the palladium–norbornene-catalyzed ortho amination/ipso conjunctive coupling†
Received
29th November 2023
, Accepted 14th December 2023
First published on 14th December 2023
Abstract
As an important class of multicomponent reactions, the palladium/norbornene (Pd/NBE) cooperative catalysis has been mainly restricted to the coupling of an aryl halide, an electrophile and a nucleophile. Here, we report the development of a Pd/NBE-catalyzed four-component reaction, which involves ortho C–H amination/ipso conjunctive coupling using an alkene and an external nucleophile. The use of alkene-tethered nitrogen electrophiles provides a rapid and modular synthesis of 3,3-disubstituted indolines from readily available aryl iodides. The reaction exhibits broad functional group tolerance, and its utility is exemplified in a streamlined formal synthesis of a rhodamine dye. Preliminary results of the asymmetric version of this reaction have also been obtained.
Introduction
Multicomponent reactions—the reactions that assemble multiple reactive moieties in a sequential manner to form a single product—offer a rapid and modular approach to access complex structures from readily available reactants.1 These reactions have found broad utilities in synthesizing analogues and constructing compound libraries. As a unique class of multicomponent reactions, the palladium/norbornene (Pd/NBE) cooperative catalysis,2 also known as the Catellani-type reaction, has become an increasingly useful tool for arene functionalization. It allows simultaneous functionalization of the ortho C–H bond of aryl halide substrates through reacting with an electrophile and the ipso position via coupling with a nucleophile (including alkenes) (Scheme 1a). While significant progress has been made in this field, the Pd/NBE catalysis has been primarily restricted to the coupling of three components: aryl (or alkenyl) substrates, electrophiles, and nucleophiles. To the best of our knowledge, the only exception to date is the seminal work by Luan (Scheme 1b).3 This reaction shows a four-component coupling involving an aryl iodide, two kinds of electrophile (an anhydride and an alkyl halide),4 and a nucleophile, in which the two ortho C–H bonds of the arene substrate are functionalized by the different electrophiles. Then, an intriguing question arose: is it possible to involve two different kinds of nucleophile in a Catellani-type reaction? Here, we report the development of a unique four-component Pd/NBE catalysis, which employs two kinds of nucleophile, i.e., an alkene and an external nucleophile, to functionalize the arene ipso position through conjunctive coupling (Scheme 1c).5 As a specific application, this reaction allows for rapid construction of 3,3-disubstituted indolines,6 which are key structural motifs found in numerous FDA-approved drugs7 (e.g., vincristine8) and various other bioactive compounds9 (Fig. 1). We hypothesized that, by tethering an amine electrophile10 with a 1,1-disubstituted alkene,11 the normal ipso Heck reaction should be interrupted, and the resulting alkyl Pd(II) species can be trapped by an external nucleophile to realize the conjunctive coupling and furnish a quaternary carbon centre.12,13
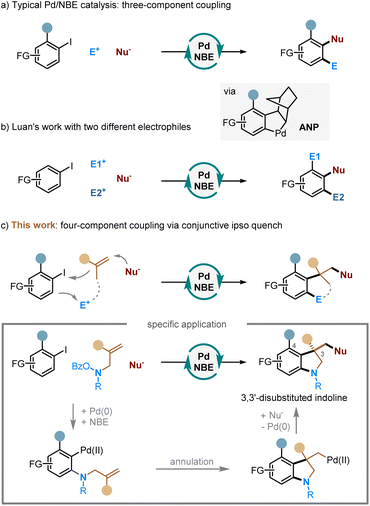 |
| Scheme 1 The Pd/NBE cooperative catalysis. | |
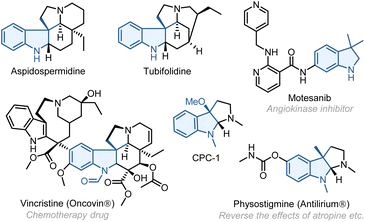 |
| Fig. 1 Representative bioactive compounds bearing 3,3′-disubstituted indoline moieties. | |
Results and discussion
To explore the proposed synthesis of 3,3-disubstituted indolines via ortho amination/ipso conjunctive coupling, we started with a model reaction system employing 2-iodoanisole (1a) as the arene substrate, N-benzoyloxy 2-methylallylamine (2a) as the alkene-tethered electrophile, and isopropyl alcohol as the hydride (external nucleophile) source.14 After systematically evaluating various reaction parameters, the desired indoline product 4a was obtained with a satisfying 83% yield (Table 1, entry 1). The major side-product was the benzocyclobutene 4a′ (10% yield), resulted from direct reductive elimination from the ANP intermediate.2h Control experiments were conducted to understand the role of each reactant. First, PPh3 was found to be the optimal ligand, and its absence led to no product formation (entry 2). Lowering the ligand loading decreased the yield to some extent (entry 3). In addition, replacement of PPh3 with other monodentate phosphine ligands gave somewhat lower yield (entry 4). Both NBE and palladium are essential to this reaction (entries 5). The use of 50 mol% NBE still afforded a comparable yield with reduced side-product formation (entry 6). For comparison, no obvious advantage was observed using structurally modified NBEs (smNBEs)2j such as N1,15N2 (ref. 16) and N3 (ref. 17 and 11c) for this substrate (entry 7). Furthermore, isopropanol was identified as the optimal hydride source, as lower efficiency was observed with bulkier alcohols, such as 3b or borneol (3c) (entry 8). Changing the leaving group on the nitrogen electrophile from simple benzoyl to a more electron-rich para-methoxy-substituted one or a more electron-deficient para-trifluoromethyl-substituted one gave no improvement of the yield (entry 9). The use of other Pd pre-catalysts, such as Pd2dba3 and Pd(OAc)2, gave comparable results (entries 10 and 11). Toluene proved to be a more suitable solvent; in contrast, the more polar 1,4-dioxane gave much lower yield and more side-product formation (entry 12), likely because the amine electrophile tends to decompose in more polar solvents.
Table 1 Control experimentsa
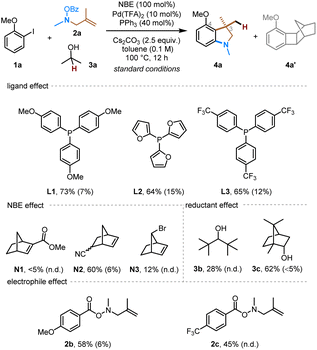
|
Entry |
Change from the “standard conditions” |
Yield of 4ab |
Yield of 4a′b |
Unless otherwise noted, all reactions were carried out with 1a (0.1 mmol), 2a (0.2 mmol) and 3a (0.12 mmol), in 1.0 mL of solvent for 12 h. The number in parentheses refers to the yield of side-product 4a′.
NMR yields were determined using 1,1,2,2-tetrachloroethane as the internal standard.
|
1 |
None |
83% |
10% |
2 |
No PPh3 |
n.d. |
n.d. |
3 |
PPh3 (25 mol%) |
50% |
<5% |
4 |
Other ligands instead of PPh3 |
Listed above |
5 |
No NBE or no Pd(TFA)2 |
n.d. |
n.d. |
6 |
NBE (50 mol%) |
68% |
<5% |
7 |
Other NBEs instead of simple NBE |
Listed above |
8 |
Other reductants instead of 3a |
Listed above |
9 |
Other electrophiles instead of 2a |
Listed above |
10 |
Pd2dba3 instead of Pd(TFA)2 |
58% |
<5% |
11 |
Pd(OAc)2 instead of Pd(TFA)2 |
71% |
10% |
12 |
1,4-Dioxane instead of toluene |
39% |
18% |
With the optimized conditions established, we next proceeded to explore the substrate scope for the formation of 3,3-disubstituted indolines (Table 2). Aryl halides with both electron-donating (4a–4f, 4i) and – withdrawing (4g, 4h, 4j–4l) groups proceeded smoothly, and the corresponding indoline products were obtained in good yields. Besides ortho-substituted aryl iodides, ortho-, meta-disubstituted and ortho-, para-disubstituted substrates (4q–4y) also worked well. A wide range of common functional groups are well tolerated in the reaction, including chloro (4g), fluoro (4h), biphenyl (4i),18 ester (4j, 4u), nitro (4k, 4r), trifluoromethyl (4l), bromo (4q), tertiary alcohol (4t), nitrile (4w), amine (4x), and amide (4y) groups. The tolerance of aryl bromide allows the products to potentially undergo various downstream transformations. Besides monocyclic aryl iodides, 1-iodonaphthalene (4n) and heteroaromatic ones, such as substituted pyridine (4m), quinoline (4o) and dibenzofuran (4p), are also suitable substrates. Regarding the side products of these reactions, in addition to the norbornyl-benzocyclobutene type, the NBE-attached uncyclized products have also been detected.
Unless otherwise noted, all reactions were carried out with 1 (0.2 mmol), 2 (0.4 mmol) and 3 (0.24 mmol) in 2.0 mL of toluene for 12 h; all yields are isolated yields.
P(4-CF3C6H4)3 (L3) as ligand.
120 °C with N4.
Phenylboronic acid was used as the nucleophile.
|
|
Other alkene-tethered O-benzoyl hydroxylamines were explored next. Bulkier olefin substituents, i.e., those bearing isopropyl (4z), tert-butyl (4aa), and phenyl groups (4ab), are tolerated in this reaction. These substrates generate an all-carbon quaternary centre at the C3 position. Besides forming N-methyl substituted indolines, the corresponding removable benzyl group (4ac) or para-methoxybenzyl group (4ad) can also be introduced. The increased steric with these substrates reduced the reactivity substantially; however, using a smNBE (N4) under an elevated reaction temperature led to decent efficiency.
In addition to hydride-mediated conjunctive couplings, other nucleophilic species, such as masked acetylides (4ae-4aj) and organoboronates (4ak-4am), can all undergo the desired transformations. These reactions also afforded an all–carbon quaternary centre in the desired indoline products, and the main side products are the direct ipso Sonogashira or Suzuki coupling without cyclization. As such, this multi-component strategy can rapidly increase the molecular complexity from simple building blocks with high chemoselectivity.
A plausible and simplified catalytic cycle is proposed in Scheme 2. The reaction starts with oxidative addition of the aryl halide to form Int I (step A), which then undergoes migratory insertion into NBE to generate Int II (step B), followed by concerted metalation deprotonation (CMD) to give the key ANP intermediate Int III (step C). Afterwards, oxidative addition of the ANP with the nitrogen electrophile (step D) and then reductive elimination (step E) introduce an amino group at the ortho position. Subsequent extrusion of NBE via β-carbon elimination (step F) sets the stage for the intramolecular migratory insertion with the alkene group (step G). The resulting Pd(II) intermediate is then trapped by the external nucleophile, which leads to the conjunctive coupling at the ipso position and regeneration of the Pd(0) catalyst after the reductive elimination (step H).
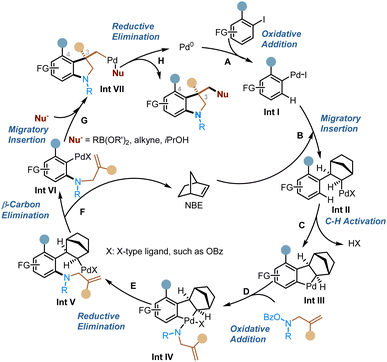 |
| Scheme 2 Proposed catalytic cycle. | |
The utility of this method was exemplified in the synthesis of compound 7, which is the key intermediate in the preparation of a diaryl rhodamine dye (Scheme 3). This type of dyes are useful fluorescent labels of nucleosides, nucleotides, polynucleotides, and polypeptides, and can be applied in the field of fluorescent nucleic acid analysis, including automated DNA sequencing.19 Intermediate 7 was previously prepared in eight steps from expensive 1,3-dihydroxynaphthalene (8).19 We envisioned that our method should allow for a more straightforward and modular access to this intermediate. Indeed, starting from relatively inexpensive 2-methoxynapthalene, the directed lithiation and subsequent iodination afforded the aryl iodide (6),20 which was then subjected to the Pd/NBE-catalyzed four-component coupling under the modified condition. Note that, compared to other aryl iodide substrates in Table 2, compound 6 is a more challenging substrate due to the meta constraint (the steric hindrance caused by the meta substituent, which hampers the ortho C–H activation).14b Ultimately, the use of a more stable amine electrophile (2b), together with a more electron-deficient tri(2-furyl)phosphine ligand (L2) and a smNBE (N1), delivered the target compound (7) in a satisfactory yield (for details, see ESI†).
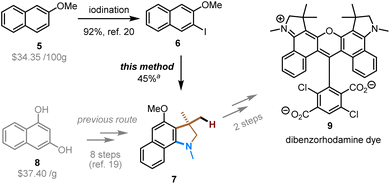 |
| Scheme 3 Synthetic application. aThe reaction was carried out with 6 (0.1 mmol), 2b (0.2 mmol), 3b (0.12 mmol), Pd(TFA)2 (15 mol%), L2 (35 mol%), N1 (100 mol%), Cs2CO3 (0.25 mmol) in 1.0 mL of toluene at 100 °C for 12 h. | |
Finally, we conducted the preliminary exploration of the asymmetric version of this transformation (Table 3). Gu and coworkers reported the first enantioselective Pd/NBE catalysis via asymmetric ipso Heck coupling using a carefully optimized chiral phosphoramidite ligand;21 however, other asymmetric ipso termination reactions remain challenging.22 At this stage, a range of different classes of readily available chiral ligands were evaluated in this conjunctive ipso coupling reaction, including phosphoramidites (L4, L5), monodentate phosphines (L6, L8), bidentate phosphines (segphos, L7), and Zhang's sulfinamide-containing phosphine (Sadphos, L9–L12) ligands.23 It is encouraging to observe that the chiral monodentate phosphoramidite ligand, i.e., L5, can afford the desired product (4z) in 89% e.e. While the yield remains low at this stage, this result indicates the feasibility to control the enantioselectivity in the conjunctive ipso coupling.
Table 3 Preliminary evaluation of chiral ligands for the enantioselective reactiona,b
Unless otherwise noted, all reactions were carried out with 1a (0.1 mmol), 2d (0.2 mmol) and 3a (1.2 mmol), Pd(TFA)2 (10 mol%), ligand (40 mol%), NBE (100 mol%), Cs2CO3 (0.25 mmol) in 1.0 mL of toluene at 85 °C for 12 h.
NMR yields were determined using 1,1,2,2-tetrachloroethane as the internal standard. The absolute stereochemistry of the major enantiomer is unknown yet.
|
|
Conclusions
In summary, we have developed a Pd/NBE-catalyzed four-component reaction to incorporate conjunctive coupling of an alkene and a nucleophile at the arene ipso position. The use of an alkene-tethered amine-electrophile in this reaction allows for a rapid, chemoselective, and modular synthesis of 3,3-disubstituted indolines, which is distinct from other approaches. The unique reaction mode discovered here could inspire the development of diverse synthetic tactics based on the Pd/NBE catalysis for broader applications. Efforts towards optimizing the enantioselective version using carefully engineered chiral ligands are in progress and will be reported in due course.
Data availability
The data underlying this study are available in the published article and its ESI.† Crystallographic data for compound 4i has been deposited at the CCDC under CCDC ID 2310334. See DOI: https://doi.org/10.1039/D3SC06409C.
Author contributions
A. R., X. L. and G. D. conceived and designed the experiments. A. R. and R. Y. performed the experiments. A. R., R. Y., X. L. and G. D. wrote the manuscript. X. L. and G. D. directed the research.
Conflicts of interest
There are no conflicts to declare.
Acknowledgements
We thank the financial support from the University of Chicago and the National Institute of General Medical Sciences (R01GM124414). We also acknowledge S. Anferov and A. Filatov (University of Chicago) for the X-ray crystallography.
Notes and references
- For recent reviews on multi-component reactrions, see:
(a) I. Ugi, A. Dömling and W. Hörl, Endeavour, 1994, 18, 115–122 CrossRef CAS;
(b) B. Ganem, Acc. Chem. Res., 2009, 42, 463–472 CrossRef CAS PubMed;
(c) A. Dömling, W. Wang and K. Wang, Chem. Rev., 2012, 112, 3083–3135 CrossRef PubMed.
- For selected reviews on Pd/NBE cooperative catalysis, see:
(a) M. Catellani, Synlett, 2003, 0298–0313 CrossRef CAS;
(b)
M. Catellani, in Palladium in Organic Synthesis, ed. J. Tsuji, Springer Berlin Heidelberg, Berlin, Heidelberg, 2005, vol. 14, pp. 21–53 Search PubMed;
(c) M. Catellani, E. Motti and N. Della Ca', Acc. Chem. Res., 2008, 41, 1512–1522 CrossRef CAS PubMed;
(d)
A. Martins, B. Mariampillai and M. Lautens, in C–H Activation, ed. J.-Q. Yu and Z. Shi, Springer Berlin Heidelberg, Berlin, Heidelberg, 2009, vol. 292, pp. 1–33 Search PubMed;
(e) J. Ye and M. Lautens, Nat. Chem., 2015, 7, 863–870 CrossRef CAS PubMed;
(f) N. Della Ca', M. Fontana, E. Motti and M. Catellani, Acc. Chem. Res., 2016, 49, 1389–1400 CrossRef PubMed;
(g) Z. Liu, Q. Gao, H. Cheng and Q. Zhou, Chem.–Eur. J., 2018, 24, 15461–15476 CrossRef CAS PubMed;
(h) J. Wang and G. Dong, Chem. Rev., 2019, 119, 7478–7528 CrossRef CAS PubMed;
(i) K. Zhao, L. Ding and Z. Gu, Synlett, 2019, 30, 129–140 CrossRef CAS;
(j) R. Li and G. Dong, J. Am. Chem. Soc., 2020, 142, 17859–17875 CrossRef CAS PubMed;
(k) Z. Chen and F. Zhang, Tetrahedron, 2023, 134, 133307 CrossRef CAS.
- J. Wang, C. Qin, J.-P. Lumb and X. Luan, Chem, 2020, 6, 2097–2109 CAS.
- X. Qi, J. Wang, Z. Dong, G. Dong and P. Liu, Chem, 2020, 6, 2810–2825 CAS.
- L. Zhang, G. J. Lovinger, E. K. Edelstein, A. A. Szymaniak, M. P. Chierchia and J. P. Morken, Science, 2016, 351, 70–74 CrossRef CAS PubMed.
- For recent representative synthesis of 3,3-disubstituted indolines, see:
(a) Y. Nakao, S. Ebata, A. Yada, T. Hiyama, M. Ikawa and S. Ogoshi, J. Am. Chem. Soc., 2008, 130, 12874–12875 CrossRef CAS PubMed;
(b) D. Liang, X. Song, L. Xu, Y. Sun, Y. Dong, B. Wang and W. Li, Tetrahedron, 2019, 75, 3495–3503 CrossRef CAS;
(c) Q. Nguyen, K. Sun and T. G. Driver, J. Am. Chem. Soc., 2012, 134, 7262–7265 CrossRef CAS PubMed;
(d) T. Torigoe, T. Ohmura and M. Suginome, Angew. Chem., Int. Ed., 2017, 56, 14272–14276 CrossRef CAS PubMed;
(e) T. Ohmura, K. Yagi, S. Kusaka and M. Suginome, ACS Catal., 2020, 10, 3152–3157 CrossRef CAS;
(f) X. Sun, Z. Wu, W. Qi, X. Ji, C. Cheng and Y. Zhang, Org. Lett., 2019, 21, 6508–6512 CrossRef CAS PubMed.
-
(a) D. Zhang, H. Song and Y. Qin, Acc. Chem. Res., 2011, 44, 447–457 CrossRef CAS PubMed;
(b) W. Zi, Z. Zuo and D. Ma, Acc. Chem. Res., 2015, 48, 702–711 CrossRef CAS PubMed;
(c) D. Liu, G. Zhao and L. Xiang, Eur. J. Org Chem., 2010, 3975–3984 CrossRef CAS.
- D. M. Whitelaw and H. S. Kim, Can. Med. Assoc. J., 1964, 90, 1385–1389 CAS.
-
(a) Y.-J. Wang, R. J. Kathawala, Y.-K. Zhang, A. Patel, P. Kumar, S. Shukla, K. L. Fung, S. V. Ambudkar, T. T. Talele and Z.-S. Chen, Biochem. Pharmacol., 2014, 90, 367–378 CrossRef CAS PubMed;
(b)
W. J. Boroughf, in Critical Care Toxicology, ed. J. Brent, K. Burkhart, P. Dargan, B. Hatten, B. Megarbane and R. Palmer, Springer International Publishing, Cham, 2016, pp. 1–14 Search PubMed;
(c) K. Biemann, M. Friedmann-Spiteller and G. Spiteller, Tetrahedron Lett., 1961, 2, 485–492 CrossRef.
- For the first ortho amination via the Pd/NBE catalysis, see: Z. Dong and G. Dong, J. Am. Chem. Soc., 2013, 135, 18350–18353 CrossRef CAS PubMed.
- For recent representative examples using alkene-tethered amine electrophiles via the Pd/NBE catalysis, see:
(a) J. Li, Y. Yang, Y. Liu, Q. Liu, L. Zhang, X. Li, Y. Dong and H. Liu, Org. Lett., 2021, 23, 2988–2993 CrossRef CAS PubMed;
(b) A. J. Rago and G. Dong, Org. Lett., 2021, 23, 3755–3760 CrossRef CAS PubMed;
(c) X. Liu, J. Wang and G. Dong, J. Am. Chem. Soc., 2021, 143, 9991–10004 CrossRef CAS PubMed.
- For representative examples of reductive Heck reactions, see:
(a) S. Cacchi and A. Arcadi, J. Org. Chem., 1983, 48, 4236–4240 CrossRef CAS;
(b) A. Amorese, A. Arcadi, E. Bernocchi, S. Cacchi, S. Cerrini, W. Fedeli and G. Ortar, Tetrahedron, 1989, 45, 813 CrossRef CAS;
(c) A. L. Gottumukkala, J. G. de Vries and A. J. Minnaard, Chem.–Eur. J., 2011, 17, 3091 CrossRef CAS PubMed;
(d) S. Raoufmoghaddam, S. Mannathan, A. J. Minnaard, J. G. de Vries and J. N. H. Reek, Chem.–Eur. J., 2015, 21, 18811 CrossRef CAS PubMed;
(e) Z.-M. Zhang, B. Xu, Y. Qian, L. Wu, Y. Wu, L. Zhou, Y. Liu and J. Zhang, Angew. Chem., Int. Ed., 2018, 57, 10373–10377 CrossRef CAS PubMed.
- For selected reviews on difunctionlization of alkenes, see:
(a) K. H. Jensen and M. S. Sigman, Org. Biomol. Chem., 2008, 6, 4083–4088 RSC;
(b) G. Yin, X. Mu and G. Liu, Acc. Chem. Res., 2016, 49(11), 2413–2423 CrossRef CAS PubMed;
(c) Y. Li, D. Wu, H.-G. Cheng and G. Yin, Angew. Chem., Int. Ed., 2020, 59, 7990–8003 CrossRef CAS PubMed;
(d) Z.-L. Li, G.-C. Fang, Q.-S. Gu and X.-Y. Liu, Chem. Soc. Rev., 2020, 49, 32–48 RSC;
(e) J. Derosaa, O. Apolinara, T. Kanga, V. T. Trana and K. M. Engle, Chem. Sci., 2020, 11, 4287–4296 RSC.
-
(a) T. Wilhelm and M. Lautens, Org. Lett., 2005, 7, 4053–4056 CrossRef CAS PubMed;
(b) For a single example showing ipso reductive Heck termination, see: J. Wang, Y. Zhou, X. Xu, P. Liu and G. Dong, J. Am. Chem. Soc., 2020, 142, 3050–3059 CrossRef CAS PubMed.
- For
the first use of N1, see: P.-X. Shen, X.-C. Wang, P. Wang, R.-Y. Zhu and J.-Q. Yu, J. Am. Chem. Soc., 2015, 137, 11574–11577 CrossRef CAS PubMed.
- For the application of N2, see:
(a) Q. Gao, Y. Shang, F. Song, J. Ye, Z.-S. Liu, L. Li, H.-G. Cheng and Q. Zhou, J. Am. Chem. Soc., 2019, 141, 15986–15993 CrossRef CAS PubMed;
(b) Y.-N. Ding, W.-Y. Shi, C. Liu, N. Zheng, M. Li, Y. An, Z. Zhang, C.-T. Wang, B.-S. Zhang and Y.-M. Liang, J. Org. Chem., 2020, 85, 11280–11296 CrossRef CAS PubMed;
(c) G. Shi, C. Shao, X. Ma, Y. Gu and Y. Zhang, ACS Catal., 2018, 8, 3775–3779 CrossRef CAS.
- X. Liu, Y. Fu, Z. Chen, P. Liu and G. Dong, Nat. Chem., 2023, 15, 1391–1399 CrossRef CAS PubMed.
- X-ray data for 4i CCDC 2310334.
-
D. Sun, J. Y. Lam, W. Zhen, S. C. Benson, B. B. Rosenblum, M. Taing, S. H. Khan and S. M. Menchen, Australia Pat., AU2008200036, A1, January 31, 2008 Search PubMed.
- R. R. Kadiyala, D. Tilly, E. Nagaradja, T. Roisnel, V. E. Matulis, O. A. Ivashkevich, Y. S. Halauko, F. Chevallier, P. C. Gros and F. Mongin, Chem.–Eur. J., 2013, 19, 7944–7960 CrossRef CAS PubMed.
- K. Zhao, S. Xu, C. Pan, X. Sui and Z. Gu, Org. Lett., 2016, 18, 3782–3785 CrossRef CAS PubMed.
-
(a) Z.-S. Liu, G. Qian, Q. Gao, P. Wang, H.-G. Cheng, Q. Wei, Q. Liu and Q. Zhou, ACS Catal., 2018, 8, 4783–4788 CrossRef CAS;
(b) Q. Gao, Z.-S. Liu, Y. Hua, L. Li, H.-G. Cheng, H. Cong and Q. Zhou, Chem. Commun., 2019, 55, 8816–8819 RSC.
- B. Yang, K. Cao, G. Zhao, J. Yang and J. Zhang, J. Am. Chem. Soc., 2022, 144, 15468–15474 CrossRef CAS PubMed.
Footnotes |
† Electronic supplementary information (ESI) available. CCDC 2310334. For ESI and crystallographic data in CIF or other electronic format see DOI: https://doi.org/10.1039/d3sc06409c |
‡ These authors contributed equally. |
|
This journal is © The Royal Society of Chemistry 2024 |
Click here to see how this site uses Cookies. View our privacy policy here.