DOI:
10.1039/D4SC01649A
(Edge Article)
Chem. Sci., 2024,
15, 9703-9708
Palladium-catalyzed asymmetric [4 + 3] cycloaddition of methylene-trimethylenemethane: access to seven-membered exocyclic axially chiral allenes†
Received
11th March 2024
, Accepted 15th May 2024
First published on 17th May 2024
Abstract
A palladium-catalyzed asymmetric [4 + 3] cycloaddition of the methylene-trimethylenemethane donor with an azadiene has been developed, affording benzofuro[3,2-b]azepine-derived exocyclic chiral allene with control of axial and point chirality. The target compounds were generated in good to excellent yields and with high diastereoselectivities and enantioselectivities (up to >20
:
1 dr, 99% ee). Furthermore, this cycloaddition reaction could be efficiently scaled-up and several synthetic transformations were accomplished for the construction of useful chiral allenol and chiral spirocyclic derivatives.
Introduction
Axially chiral allenes are important structural features in a variety of natural products, bioactive compounds, pharmaceuticals, ligands, organo-catalysts, and functional materials.1 The reactive orthogonal π-bonds of chiral allenes render them versatile chiral building blocks in organic synthesis.2 Developing concise approaches to synthesize axially chiral allene-containing compounds asymmetrically using readily available and inexpensive starting materials has attracted much attention.3 Among the identified allenic natural products and pharmaceuticals, chiral exocyclic allenes constitute a major subclass, for example, allenic carbacyclin,4 Neoxanthin,5 Grasshopper ketone,6 and Citroside A7 (Fig. 1). However, compared with linear allenes, strategies to construct chiral exocyclic allenes are still rare and remain in high demand.8
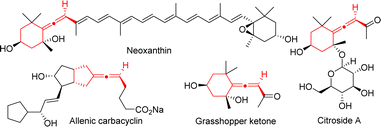 |
| Fig. 1 Bioactive chiral exocyclic allenes. | |
Cycloaddition reactions involving palladium-trimethylenemethane (Pd-TMM) are extremely valuable in the construction of cyclic compounds9 and total synthesis of natural products.10 In the asymmetric Pd-TMM chemistry (Scheme 1a), allyl trimethylsilanes have extensively been employed as TMM donors11 since Trost's important contribution in 1979.12 In recent years, a number of novel self-deprotonated TMM donors have been gradually developed for the asymmetric Pd-TMM cycloadditions.13 All these TMM donors generally behave as three-carbon synthons to react with a multitude of acceptors, giving chiral five,11a–g,13a–i six,11h seven13j–o and nine-membered11i carbo- and heterocyclic compounds. On the basis of the above, in 2013, Trost and co-workers reported a novel methylene-TMM donor, which could react with α, β-unsaturated N-acyl pyrroles in the palladium-catalyzed asymmetric [3 + 2] cycloaddition to provide chiral substituted vinylidenecyclopentanes.14 And then in 2018, they developed a palladium-catalyzed asymmetric [3 + 2] cycloaddition reaction between the racemic methylene-TMM donor and electron-deficient olefins through a dynamic kinetic asymmetric transformation process, furnishing cyclopentane-derived chiral exocyclic allenes.15 In 2019, our group also applied it in asymmetric tandem [3 + 2] cycloaddition/allylation to produce chiral hexahydropyrazolo[5,1-a]isoquinoline derivatives (Scheme 1b).16 To the best of our knowledge, the catalytic asymmetric [4 + 3] cycloaddition of the methylene-TMM donor has never been achieved. On the other hand, seven-membered azepines are privileged skeletons in many natural products and pharmaceuticals.17 Introducing an axially chiral allene moiety into the exocyclic backbone of the chiral azepine is highly challenging and may produce unexpected biological and pharmacological properties. In conjunction with our interest in palladium-catalyzed cycloaddition reaction and allene chemistry,18 herein, we present the first palladium-catalyzed asymmetric [4 + 3] cycloadditions of methylene-TMM donors with benzofuran derived azadienes to furnish benzofuro[3,2-b]azepine-derived exocyclic chiral allenes bearing axial and central chirality (Scheme 1c).
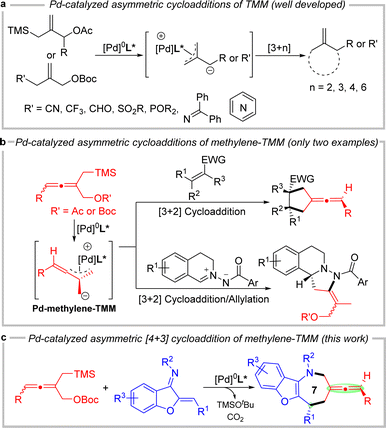 |
| Scheme 1 Profile of Pd-catalyzed asymmetric cycloadditions involving TMM and methylene-TMM. | |
Results and discussion
To test the feasibility of the palladium-catalyzed [4 + 3] cycloaddition, we investigated the model reaction of the racemic tri-substituted allene TMM donor 1a and benzofuran-derived azadiene 2a in 1,4-dioxane at room temperature (Table 1). On the basis of our previous work on palladium-catalyzed cycloadditions,18a–c Pd2(dba)3 was used as the precatalyst and axially chiral BINAP- or BINOL-based phosphines as the ligand. To our delight, under catalysis of a complex of Pd2(dba)3 (2.5 mol%) and L1 (11 mol%), the [4 + 3] cycloaddition proceeded to deliver the desired cycloadduct 3aa in 28% yield with low stereoselectivity (1
:
1.5 dr and −20% ee) (entry 1). Then several axially chiral bisphosphine ligands L2 and L3 were examined and did not promote the reaction (entry 2 and entry 3). Subsequently, considering that phosphoramidite ligands containing different substitutions on the nitrogen atom had a huge impact on the diastereoselectivity and enantioselectivity, several axially chiral phosphoramidite ligands L4–L7 were then examined (entries 4–7). The reaction proceeded smoothly in the presence of L7 to give 3aa in 73% yield with moderate diastereoselectivity (1
:
4 dr) and a significant improvement of enantioselectivity (96% ee) (entry 7). A quick screening of several solvents such as tetrahydrofuran (THF), toluene and mesitylene (entries 8–10) revealed that mesitylene is the optimal solvent, leading to the cycloadduct 3aa in 80% yield with 1
:
5 dr and 98% ee (entry 10). Then several palladium catalysts were also evaluated, and Pd(dmdba)2 displayed a better catalytic activity, resulting in the formation of the product in 90% yield with 1
:
5.5 dr and 98% ee (entry 12). Decreasing the loading of the catalyst led to a slight improvement of diastereoselectivity and yield, but the reaction time could be extended to 36 hours. Finally, the optimal reaction conditions were determined as follows: the use of Pd(dmdba)2 (2.5 mol% or 5 mol%) and chiral ligand L7 (5.5 mol% or 11 mol%) as the catalyst in mesitylene at room temperature.
Table 1 Optimization of reaction conditionsa

|
Entry |
Pd source |
L |
Solvent |
t h−1 |
Yieldb (%) |
drc |
eed (%) |
Unless otherwise indicated, all reactions were performed with 1a (0.20 mmol) and 2a (0.10 mmol) in the presence of [Pd] (5 mol%) and a ligand (5.5 mol% for diphosphines, 11 mol% for the phosphoramidite ligand) in 1 mL of solvent under an Ar atmosphere at room temperature. Abbreviations: dba, dibenzylidene acetone; dmdba, 3,5,3′,5′-dimethoxydibenzylidene acetone.
Isolated yield.
dr values were determined by 1 H NMR analysis.
ee values of major diastereomers were determined by HPLC analysis using a chiral stationary phase.
2.5 mol% Pd and 5.5 mol% ligand were used.
No reaction.
|
1 |
Pd2(dba)3 |
L1
|
1,4-Diox |
48 |
28 |
1 : 1.5 |
−20 |
2 |
Pd2(dba)3 |
L2
|
1,4-Diox |
48 |
NRf |
— |
— |
3 |
Pd2(dba)3 |
L3
|
1,4-Diox |
48 |
NR |
— |
— |
4 |
Pd2(dba)3 |
L4
|
1,4-Diox |
48 |
Trace |
— |
— |
5 |
Pd2(dba)3 |
L5
|
1,4-Diox |
24 |
50 |
1 : 3 |
87 |
6 |
Pd2(dba)3 |
L6
|
1,4-Diox |
6 |
72 |
1 : 6 |
−87 |
7 |
Pd2(dba)3 |
L7
|
1,4-Diox |
10 |
73 |
1 : 4 |
96 |
8 |
Pd2(dba)3 |
L7
|
THF |
2 |
78 |
1 : 3 |
96 |
9 |
Pd2(dba)3 |
L7
|
Toluene |
3 |
76 |
1 : 4 |
97 |
10 |
Pd2(dba)3 |
L7
|
Mesitylene |
2 |
80 |
1 : 5 |
98 |
11 |
Pd(dba)2 |
L7
|
Mesitylene |
2 |
77 |
1 : 4 |
95 |
12 |
Pd(dmdba)2 |
L7
|
Mesitylene |
6 |
90 |
1 : 5.5 |
98 |
13e |
Pd(dmdba)2 |
L7
|
Mesitylene |
36 |
92 |
1 : 6 |
98 |
After the optimized conditions were established, we investigated the scope of allene TMM donors 1 in the Pd-catalyzed asymmetric [4 + 3] cycloaddition (Scheme 2). Generally, the reaction proceeded smoothly to give the corresponding products in good to excellent yields (50–96%) with diastereoselectivities ranging from 5.7
:
1 to >20
:
1 and good enantioselectivities of 85–99%. The allene 1 with whether straight-chain alkyl, branch-chain alkyl or cycloalkyl substituents worked efficiently in the reaction (3aa–3ha). It is worth noting that higher diastereoselectivities were obtained for straight-chain alkyl substituents on the allene, probably due to the lower steric hindrance, which makes it easier to fit the spatial structure of transition states. Furthermore, the alkyl group bearing protected heteroatoms (3ia) and the benzyl group (3ja) were also well accepted, leading to the corresponding products with good to excellent levels of diastereo-and enantioselectivities. The absolute configuration of the cycloadduct was unambiguously determined through X-ray crystallographic analysis of the product 3ba.21
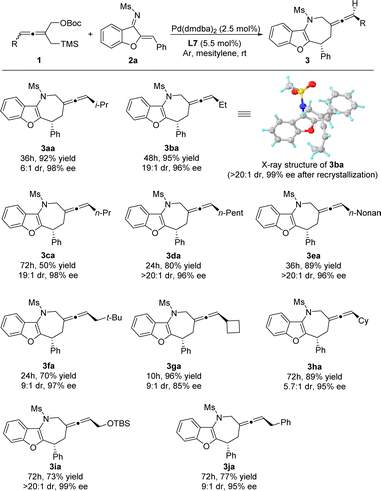 |
| Scheme 2 Substrate scope of allene TMM donors 1.a aUnless otherwise indicated, all reactions were carried out with 1 (0.20 mmol) and 2a (0.10 mmol) in 1 mL of mesitylene under an Ar atmosphere at room temperature. Isolated yields were reported. The dr values were determined by 1H NMR analysis, and ee values were determined by HPLC analysis using a chiral stationary phase. | |
Following exploration of the variation of substituents on the allenes in Scheme 2, the generality of the asymmetric [4 + 3] cycloaddition with ethyl allene donor 1b was investigated. As shown in Scheme 3, a series of benzofuran derived azadienes 2 were suitable substrates for this cycloaddition and afforded benzofuro[3,2-b]azepine-derived exocyclic axially chiral allenes 3 in moderate to excellent diastereoselectivities (9
:
1 to >20
:
1 dr) and very good enantioselectivities (92% to 99% ee). Azadienes bearing electron-donating or -withdrawing groups at the ortho, meta, and para positions of the aromatic R1 group were converted into the expected products 3bb–3br in good to excellent yields, dr, and ee. The heteroaryl substrate 3s also performed the reaction well to produce the corresponding product 3bs in 92% yield with 13
:
1 dr and 94% ee. Moreover, R3 substitutions on 2 bearing halogens or a methyl group at either the C5- or C6-position of the benzofuran ring were amenable to this cycloaddition, furnishing the corresponding products 3bt–3bw. Azadiene 2x with a different sulfonamide substituent (phenylsulfonyl) could also deliver 3bx in 99% yield with 9
:
1 dr and 95% ee. Gratifyingly, the alkyl-substituted benzofuran-derived azadiene 2y was also compatible for this asymmetric [4 + 3] cycloaddition, thereby resulting in product 3by in 86% yield with 6
:
1 dr and 92% ee.
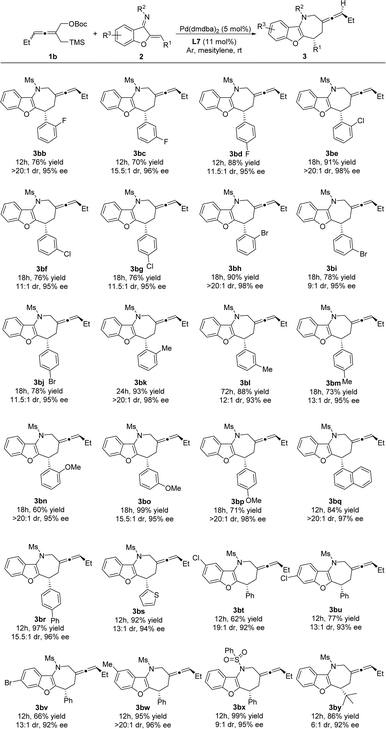 |
| Scheme 3 Substrate scope of benzofuran-derived azadienes 2.a aUnless otherwise indicated, all reactions were carried out with 1b (0.20 mmol) and 2 (0.10 mmol) in 1 mL of mesitylene under an Ar atmosphere at room temperature. Isolated yields were reported. The dr values were determined by 1H NMR analysis, and ee values were determined by HPLC analysis using a chiral stationary phase. | |
As shown in Scheme 4a, Pd-catalyzed asymmetric [4 + 3] cycloaddition reaction of substrates 1b and 2a could be scaled-up with loadings of the palladium catalyst (5 mol%) and L7 (11 mol%), producing the product 3ba in good yield (90%) with excellent selectivities (19
:
1 dr and 96% ee). In order to further demonstrate the potential of the asymmetric [4 + 3] cycloaddition, subsequent transformations of the product 3ia and 3ba have been investigated (Scheme 4b). Treatment of the chiral product 3ia with TBAF afforded the alcohol product 4 in 97% yield. Then, the gold catalyzed cyclization19,20 of 4 gave the spirocycle product 5 in good yield with moderate diastereoselectivity and the same enantiomeric excess as that of the starting material. Finally, the seven-membered exocyclic axially chiral allene 3ba could be efficiently converted to the five-membered spirocycle 6 by a TfOH-mediated ring contraction and the absolute configuration of the product 6 was determined by X-ray crystallographic analysis.21
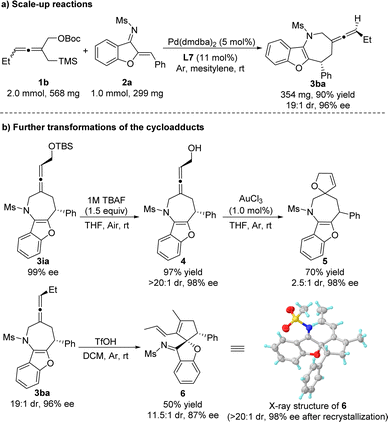 |
| Scheme 4 Scale-up experiment and further transformations of the cycloadducts. | |
On the basis of experimental results and previous mechanistic studies,14–16 a plausible mechanism for the reaction is proposed in Scheme 5. In the presence of the palladium catalyst, allene TMM donors 1 were transformed into Pd-TMM complex A or A′ with simultaneous release of TMSOtBu and CO2. The dynamic kinetic asymmetric transformation process may occur between A and A′. The R-substituent of the allene and the bulky palladium complex on opposing faces leads to the more stable intermediate A (more details in the ESI†). According to the transition state TS-1, the sterically crowded chiral ligand dominates so that the carbanion of the intermediate A attacks at the Si face of the olefinic bond in the benzofuran-derived azadienes 2, leading to the transition state TS-2. There were three possible pathways for the formation of different cyclized products in TS-2. For path (b), the steric effect between the N-Ms group and the intermediate carbon (C3) of allene blocked the cyclization reaction. For path (c), due to steric hindrance of the C4 point and its distance from the reaction site, it is difficult for the nitrogen anion to attack C4 to afford an eight-membered ring. Therefore, the transition state preferred path (a) to give rise to the seven-membered heterocyclic product 3 by intramolecular linear regioselective allylic substitution.
 |
| Scheme 5 Proposed reaction mechanism for palladium-catalyzed asymmetric [4 + 3] cycloaddition. | |
Conclusions
In summary, palladium-catalyzed asymmetric [4 + 3] cycloadditions of readily available racemic methylene-TMM donors with benzofuran-derived azadienes have been achieved under mild reaction conditions to give the benzofuro[3,2-b]azepine-derived exocyclic chiral allenes bearing axial and central chirality in good to excellent yields with good to excellent diastereoselectivities and enantioselectivities. This is the first example of asymmetric [4 + 3] cycloadditions of Pd-methylene-TMM. The cycloaddition reaction can be scaled-up, and the synthetic utility of chiral allenes has been demonstrated by their further transformations. The bactericidal activities of the novel compounds synthesized by our methodology are being evaluated.
Data availability
Experimental details and characterization of the complexes can be found in the ESI.†
Author contributions
B. M. conceived and directed the project. Y. W. performed reaction experiments and synthesis of substrates. Z. W., Y. S., Y. M. and T. L. performed synthesis of substrates and some data collection. C. Y. helped with the crystallographic data analysis. B. M., H. G., C. Y. and Y. W. cowrote the manuscript. All authors discussed the results and commented on the manuscript.
Conflicts of interest
There are no conflicts to declare.
Acknowledgements
This work was supported by the National Natural Science Foundation of China (no. 22101156), the Natural Science Foundation of Shandong Province, China (no. ZR2021QB032) and the Taishan Scholars Program of Shandong Province, China (No. tsqnz20221153).
Notes and references
-
(a)
N. Krause and A. S. K. Hashmi, Modern Allene Chemistry, Wiley-VCH, Weinheim, Germany, 2004, pp. 877–923 CrossRef;
(b) A. Hoffmann-Röder and N. Krause, Angew. Chem., Int. Ed., 2004, 43, 1196–1216 CrossRef PubMed;
(c) X. Pu, X. Qi and J. M. Ready, J. Am. Chem. Soc., 2009, 131, 10364–10365 CrossRef CAS PubMed;
(d) F. Cai, X. Pu, X. Qi, V. Lynch, A. Radha and J. M. Ready, J. Am. Chem. Soc., 2011, 133, 18066–18069 CrossRef CAS PubMed;
(e) S. Yu and S. Ma, Angew. Chem., Int. Ed., 2012, 51, 3074–3112 CrossRef CAS PubMed;
(f) P. Rivera-Fuentes and F. Diederich, Angew. Chem., Int. Ed., 2012, 51, 2818–2828 CrossRef CAS PubMed.
- For relevant reviews on chiral allenes in synthetic organic chemistry, see:
(a) A. S. K. Hashmi, Angew. Chem., Int. Ed., 2000, 39, 3590–3593 CrossRef CAS;
(b) R. W. Bates and V. Satcharoen, Chem. Soc. Rev., 2002, 31, 12–21 RSC;
(c) S. Ma, Chem. Rev., 2005, 105, 2829–2871 CrossRef PubMed;
(d) J. M. Alonso and P. Almendros, Chem. Rev., 2021, 121, 4193–4252 CrossRef CAS PubMed.
- For selected reviews on the catalytic asymmetric synthesis of axially chiral allenes, see:
(a) M. Ogasawara, Tetrahedron: Asymmetry, 2009, 20, 259–271 CrossRef CAS;
(b) R. K. Neff and D. E. Frantz, ACS Catal., 2014, 4, 519–528 CrossRef CAS;
(c) J. Ye and S. Ma, Org. Chem. Front., 2014, 1, 1210–1224 RSC;
(d) W.-D. Chu, Y. Zhang and J. Wang, Catal. Sci. Technol., 2017, 7, 4570–4579 RSC;
(e) X. Wang, X. Chen, W. Lin, P. Li and W. Li, Adv. Synth. Catal., 2022, 364, 1212–1222 CrossRef CAS;
(f) W. Xiao and J. Wu, Org. Chem. Front., 2022, 9, 5053–5073 RSC.
- P. W. Collins and S. W. Djuric, Chem. Rev., 1993, 93, 1533–1564 CrossRef CAS.
- A. Baumeler and C. H. Eugster, Helv. Chim. Acta, 1992, 75, 773–790 CrossRef CAS.
- A. Baumeler, W. Brade, A. Haag and C. H. Eugster, Helv. Chim. Acta, 1990, 73, 700–715 CrossRef CAS.
- K. Umehara, I. Hattori, T. Miyase, A. Ueno, S. Hara and C. Kageyama, Chem. Pharm. Bull., 1998, 36, 5004–5008 CrossRef.
- For selected examples, see:
(a) A. Hölzl-Hobmeier, A. Bauer, A. V. Silva, S. M. Huber, C. Bannwarth and T. Bach, Nature, 2018, 564, 240–243 CrossRef PubMed;
(b) C.-Y. He, Y.-X. Tan, X. Wang, R. Ding, Y.-F. Wang, F. Wang, D. Gao, P. Tian and G.-Q. Lin, Nat. Commun., 2020, 11, 4293–4301 CrossRef CAS PubMed;
(c) S. Tang, P. Zhang, Y. Shao and J. Sun, Nat. Commun., 2022, 13, 3146–3157 CrossRef CAS PubMed;
(d) S. Zou, B. Yu and H. Huang, Angew. Chem., Int. Ed., 2023, 62, e202215325 CrossRef CAS PubMed;
(e) P. Luo, L. Li, X. Mao, Z. Sun, Y. Wang, F. Peng and Z. Shao, Chem. Sci., 2023, 14, 10812–10823 RSC.
- For selected reviews, see:
(a) B. M. Trost, Angew Chem. Int. Ed. Engl., 1986, 25, 1–114 CrossRef;
(b) B. M. Trost, Pure Appl. Chem., 1988, 60, 1615–1626 CrossRef CAS;
(c) M. Lautens, W. Klute and W. Tam, Chem. Rev., 1996, 96, 49–92 CrossRef CAS PubMed;
(d) J. P. A. Harrity, B. Allen and C. Lakeland, Chem. - Eur. J., 2017, 23, 13830–13857 CrossRef PubMed;
(e) B. M. Trost and G. Mata, Acc. Chem. Res., 2020, 53, 1293–1305 CrossRef CAS PubMed;
(f) J. Dua, Y.-F. Li and C.-H. Ding, Chin. Chem. Lett., 2023, 34, 10840–10858 Search PubMed.
- For selected examples, see:
(a) B. M. Trost and M. L. Crawley, J. Am. Chem. Soc., 2002, 124, 9328–9329 CrossRef CAS PubMed;
(b) W. J. Moran, K. M. Goodenough, P. Raubo and J. P. A. Harrity, Org. Lett., 2003, 5, 3427–3429 CrossRef CAS PubMed;
(c) N. C. Mancey, N. Sandon, A.-L. Auvinet, R. J. Butlin, W. Czechtizky and J. P. A. Harrity, Chem. Commun., 2011, 47, 9804–9806 RSC;
(d) B. M. Trost, D. A. Bringley, T. Zhang and N. Cramer, J. Am. Chem. Soc., 2013, 135, 16720–16735 CrossRef CAS PubMed.
-
(a) B. M. Trost, J. P. Stambuli, S. M. Silverman and U. Schwörer, J. Am. Chem. Soc., 2006, 128, 13328–13329 CrossRef CAS PubMed;
(b) B. M. Trost, S. M. Silverman and J. P. Stambuli, J. Am. Chem. Soc., 2007, 129, 12398–12399 CrossRef CAS PubMed;
(c) B. M. Trost and S. M. Silverman, J. Am. Chem. Soc., 2010, 132, 8238–8240 CrossRef CAS PubMed;
(d) B. M. Trost, D. A. Bringley and S. M. Silverman, J. Am. Chem. Soc., 2011, 133, 7664–7667 CrossRef CAS PubMed;
(e) B. M. Trost, D. A. Bringley and P. S. Seng, Org. Lett., 2012, 14, 234–237 CrossRef CAS PubMed;
(f) B. M. Trost and S. M. Silverman, J. Am. Chem. Soc., 2012, 134, 4941–4954 CrossRef CAS PubMed;
(g) B. M. Trost, T. M. Lam and M. A. Herbage, J. Am. Chem. Soc., 2013, 135, 2459–2461 CrossRef CAS PubMed;
(h) R. Shintani, S. Park, W. L. Duan and T. Hayashi, Angew. Chem., Int. Ed., 2007, 46, 5901–5903 CrossRef CAS PubMed;
(i) B. M. Trost and P. J. McDougall, Org. Lett., 2009, 11, 3782–3785 CrossRef CAS PubMed.
-
(a) B. M. Trost and D. M. T. Chan, J. Am. Chem. Soc., 1979, 101, 6429–6432 CrossRef CAS;
(b) P. Binger and U. Schuchardt, Angew Chem. Int. Ed. Engl., 1977, 16, 249–250 CrossRef.
-
(a) B. M. Trost and G. Mata, Angew. Chem., Int. Ed., 2018, 57, 12333–12337 CrossRef CAS PubMed;
(b) B. M. Trost and Y. Wang, Angew. Chem., Int. Ed., 2018, 57, 11025–11029 CrossRef CAS PubMed;
(c) B. M. Trost, Z. Jiao and C. Hung, Angew. Chem., Int. Ed., 2019, 58, 15154–15158 CrossRef CAS PubMed;
(d) B. M. Trost, Y. Wang and C. Hung, Nat. Chem., 2020, 12, 294–301 CrossRef CAS PubMed;
(e) B. M. Trost, Z. Zuo, Y. Wang and J. E. Schultz, ACS Catal., 2020, 10, 9496–9503 CrossRef CAS;
(f) B. M. Trost, A. H. Shinde, Y. Wang, Z. Zuo and C. Min, ACS Catal., 2020, 10, 1969–1975 CrossRef CAS;
(g) B. M. Trost, Z. Zuo and Y. Wang, Org. Lett., 2021, 23, 979–983 CrossRef CAS PubMed;
(h) B. M. Trost, C. Zhu and C. C. Ence, Org. Lett., 2021, 23, 2460–2464 CrossRef CAS PubMed;
(i) B. M. Trost and Z. Zuo, Angew. Chem., Int. Ed., 2020, 59, 1243–1247 CrossRef CAS PubMed;
(j) Y. Liu, Z. Wang, Z. Huang, X. Zheng, W. Yang and W.-P. Deng, Angew. Chem., Int. Ed., 2020, 59, 1238–1242 CrossRef CAS PubMed;
(k) P. Kumari, W. Liu, C. Wang, J. Dai, M. Wang, Q. Yang, Y. Deng and Z. Shao, Chin. J. Chem., 2020, 38, 151–157 CrossRef CAS;
(l) X. Zheng, H. Sun, W.-L. Yang and W.-P. Deng, Sci. China: Chem., 2020, 63, 911–916 CrossRef CAS;
(m) W.-L. Yang, Z. Huang, Y.-Z. Liu, X. Yu and W.-P. Deng, Chin. J. Chem., 2020, 38, 1571–1574 CrossRef CAS;
(n) Y.-Z. Liu, Z. G. Wang, Z. S. Huang, W.-L. Yang and W.-P. Deng, Org. Lett., 2021, 23, 948–952 CrossRef CAS PubMed;
(o) W.-L. Yang, J.-H. Shen, Z.-H. Zhao, Z. Wang and W.-P. Deng, Org. Chem. Front., 2022, 9, 4685–4691 RSC.
- B. M. Trost and A. Maruniak, Angew. Chem., Int. Ed., 2013, 52, 6262–6264 CrossRef CAS PubMed.
- B. M. Trost, D. Zell, C. Hohn, G. Mata and A. Maruniak, Angew. Chem., Int. Ed., 2018, 57, 12916–12920 CrossRef CAS PubMed.
- B. Mao, Y. Xu, Y. Chen, J. Dong, J. Zhang, K. Gu, B. Zheng and H. Guo, Org. Lett., 2019, 21, 4424–4427 CrossRef CAS PubMed.
-
A. R. Katritzky, C. A. Ramsden, E. F. V. Scriven and R. J. K. Taylor, Comprehensive Heterocyclic Chemistry III, Elsevier, Netherlands, 2008, vol. 13 Search PubMed.
-
(a) B. Mao, J. Zhang, Y. Xu, Z. Yan, W. Wang, Y. Wu, C. Sun, B. Zheng and H. Guo, Chem. Commun., 2019, 55, 12841–12844 RSC;
(b) B. Mao, H. Liu, Z. Yan, Y. Xu, J. Xu, W. Wang, Y. Wu and H. Guo, Angew. Chem., Int. Ed., 2020, 59, 11316–11320 CrossRef CAS PubMed;
(c) H. Lin, X. Yang, W. Ning, X. Huang, X. Cao, Y. Ge, B. Mao, C. Wang, H. Guo and C. Yuan, Org. Lett., 2022, 24, 9442–9446 CrossRef CAS PubMed;
(d) Y. Dong, J. Liu, X. Gao, T. Pan, B. Mao, S. Yu, Y. Wu, C. Zhang and H. Guo, Chin. Chem. Lett., 2023, 34, 108297–108301 CrossRef CAS.
- N. Krause and C. Winter, Chem. Rev., 2011, 111, 1994–2009 CrossRef CAS PubMed.
- B. Alcaide and P. Almendros, Acc. Chem. Res., 2014, 47, 939–952 CrossRef CAS PubMed.
- Crystallographic data for 3ba and 6 have been deposited with the Cambridge Crystallographic Data Centre as deposition number CCDC 2335107 and 2335108, respectively.
|
This journal is © The Royal Society of Chemistry 2024 |
Click here to see how this site uses Cookies. View our privacy policy here.