DOI:
10.1039/D4SC02998D
(Edge Article)
Chem. Sci., 2024,
15, 13942-13948
Palladium-catalyzed decarboxylative (4 + 3) cycloadditions of bicyclobutanes with 2-alkylidenetrimethylene carbonates for the synthesis of 2-oxabicyclo[4.1.1]octanes†
Received
7th May 2024
, Accepted 1st August 2024
First published on 6th August 2024
Abstract
While cycloaddition reactions of bicyclobutanes (BCBs) have emerged as a potent method for synthesizing (hetero-)bicyclo[n.1.1]alkanes (usually n ≤ 3), their utilization in the synthesis of bicyclo[4.1.1]octane derivatives (BCOs) is still underdeveloped. Here, a palladium-catalyzed formal (4 + 3) reaction of BCBs with 1,4-O/C dipole precursors for the synthesis of oxa-BCOs is described. Unlike previous catalytic polar (3 + X) cycloadditions of BCBs, which are typically achieved through the activation of BCB substrates, the current reaction represents a novel strategy for realizing the cycloaddition of BCBs through the activation of the “X” cycloaddition partner. Moreover, the obtained functionalized oxa-BCOs products can be readily modified through various synthetic transformations.
Introduction
Bridged ring systems have long been coveted by organic and medicinal chemists due to their versatility as the basic structures of natural products and pharmaceuticals.1 Given the success of the “escape from flatland” concept in medicinal chemistry,2 there is a growing interest in bicyclo[n.1.1]alkanes as synthetic targets due to their rigid conformation and metabolic stability, which show great potential in replacing benzene rings.3 For instance, bicyclo[1.1.1]pentanes (BCPs),4 bicyclo[2.1.1]hexanes (BCHs),5 and bicyclo[3.1.1]heptanes (BCHeps)6 can mimic para-, ortho-, meta- and multi-substituted benzenoids in drug design, contingent on their substitution patterns. Additionally, Mykhailiuk's survey showed that incorporating heteroatoms (O- or N-) into BCH and BCHep analogues generally resulted in positive changes in water solubility, metabolic stability, and lipophilicity.5a–c,6a Therefore, developing new strategies to synthesize bicyclo[n.1.1]alkane derivatives is highly desirable and will expedite their application in new drug discovery.
The first cycloaddition reaction of bicyclo[1.1.0]butanes (BCBs) was reported in 1966 by Blanchard.7 Only in recent years has it emerged as a crucial synthetic platform for constructing (hetero-)bicyclo[n.1.1]alkane skeletons, owing to the pioneering scientific contributions of Wipf,8 Glorius,9 Brown,10 Leitch,11 Procter,12 Molander,13 Li,14 Wang,15 Waser,16 Studer,17 L. Deng,18 Feng,19 W.-P. Deng,20 Bach,21 Jiang22 and others.23 Generally, there are five state-of-the-art strategies for the cycloaddition of BCBs to obtain (hetero-)bicyclo[n.1.1]alkanes: (a) the formal (3 + 1) cycloaddition of BCBs with dihalocarbenes for synthesizing BCPs pioneered by Applequist,24a Mykhailiuk,24b,c Ma24d,e and Anderson;24f (b) thermally-driven (3 + 2) cycloaddition of BCBs for the synthesis of (hetero-)BCHs;7,8,23a–c (c) energy transfer [2π + 2σ]9a–d,10,21 and [2σ + 2σ]16 photocycloadditions; (d) electron transfer catalysis to construct BCHs and BCHeps via formal (3 + 2)12,14a,15,23d,e and (3 + 3)13,14b cycloaddition of BCBs. (e) Polar cycloadditions of BCBs.9f,g,11,17-20 Despite substantial advancements, the cycloadditions of BCBs continue to encounter numerous obstacles. For example, (i) in contrast to the well-established formal (3 + 1), (3 + 2), and (3 + 3) cycloadditions of BCBs, the higher-order (3 + n) (n > 3) cycloaddition of BCBs to saturated bicyclo[n.1.1]alkanes is still uncommon in the literature (Scheme 1a).25 Currently, there is only one documented formal (5 + 3) example of BCBs reacting with thiophenes to form unsaturated bicyclic rings through a photoredox-induced radical pathway (Scheme 1a, left).9h This rarity may be attributed to the high degree of transannular strain and unfavorable entropic factors during the formation of medium-sized rings. Although Grygorenko's elegant research has shown that bicyclo[4.1.1]octane frameworks (BCOs) are potential bioisosteres of substituted benzene,3c there is no report on the (4 + 3) cycloaddition of BCBs for preparing these valuable C(sp3)-rich bridged ring systems. (ii) The seminal contributions of Leitch's group11 in the realm of polar cycloadditions of BCBs led to the expeditious advancement of formal (3 + n) (n = 2 or 3) cycloadditions between BCBs and imines,11 aldehydes,9f ketenes,17 indoles18,19 and nitrones20 over the past two years, providing a wide range of valuable (hetero-)BCHs and BCHeps. However, all these Lewis acid catalytic systems were used to activate BCB substrates to generate the cycloadducts. The strategy for synthesizing bicyclo[n.1.1]alkanes by catalytically activating the “X” cycloaddition partner in the polar (3 + X) cycloadditions of BCBs remains unknown (Scheme 1a, right). Therefore, it is highly desirable to develop a new cycloaddition strategy to achieve the challenging (4 + 3) cycloaddition of BCBs.
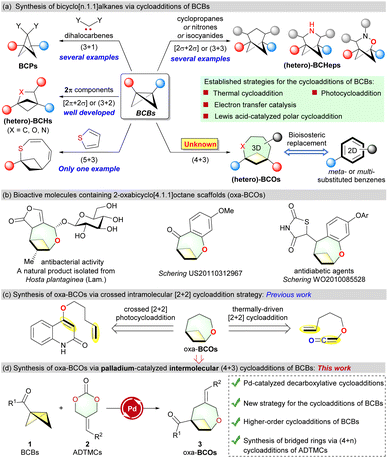 |
| Scheme 1 Synthesis of 2-oxabicyclo[4.1.1]octanes and its scientific context. | |
2-Oxabicyclo[4.1.1]octane scaffolds (oxa-BCOs) exist in both natural and pharmacologically relevant compounds (Scheme 1b).26 However, only a limited number of preparative methods have been established so far, and they are limited in scope and lack convergence. Current syntheses of oxa-BCOs primarily rely on the intramolecular crossed [2 + 2] cycloaddition of 4-(pent-4-en-1-yloxy)quinolin-2(1H)-ones27a,b or alkenyloxyketenes (Scheme 1c).27c,d
In the field of O-heterocyclic synthesis, Pd-catalyzed (4 + n) dipolar cycloadditions of 2-alkylidenetrimethylene carbonates (ADTMCs)28 have emerged as a powerful method for synthesizing monocyclic rings,29a,b fused rings,29c–e and spirocyclic scaffolds.29f–j However, their application in constructing bridged ring systems is extremely rare. As part of our ongoing research program on designing new reactions involving strained rings,19,30 this paper presents the first hetero-(4 + 3) cycloadditions of BCBs with 1,4-O/C dipole species generated catalytically from ADTMCs. The innovative palladium catalytic strategy in BCB chemistry was used to facilitate the cycloaddition reactions,31 resulting in the production of functionalized oxa-BCOs that were unattainable by other methods.
Results and discussion
Unlike relatively stable 1,3-dipoles, 1,4-dipoles, which are not fully conjugated chemical species, are frequently highly reactive and short-lived, resulting in numerous unexpected reaction pathways.28 Initially, we studied the cycloaddition of ADTMC 2a with 1,3-disubstituted BCB ketone using a palladium catalysis strategy. Regrettably, we failed to achieve the desired cycloadduct as 2a rapidly decomposed.32 Therefore, we opted for the monosubstituted BCB ketone 1a with reduced steric hindrance as the model substrate (Table 1; see the ESI† for the complete set of optimization data). Ligands L1–L3, L6–L8, and L10 were tested as indicated in Table 1. However, none of these ligands resulted in the formation of the desired product 3aa, and 2a underwent rapid decomposition. Phosphinooxazoline (PHOX)-type ligands L4 and L5 produced the (4 + 3) cycloadduct 3aa, albeit with a low yield (<15%). The current Pd-catalyzed cycloaddition of BCB was highly ligand-dependent (entries 1–10). Although Segphos L8 did not produce oxa-BCO 3aa, the sterically-hindered and electron-rich DTBM-Segphos L9 successfully yielded the desired product with a 49% NMR yield (entry 8 versus 9). Further improvement of the yield was achieved with EtOAc, THF or 1,4-dioxane instead of toluene as the solvent (entries 11–15). Besides ligands, the solvent also had substantial effect on the yield but no improvement over 1,4-dioxane was seen. Therefore, the final reaction conditions are described as follows: 1 (1.0 equiv.), 2 (1.2 equiv.), Pd2(dba)3.CHCl3 (5 mol%), commercial available ligand L9 (10 mol%), 1,4-dioxane, 25 °C, and 12 h (conditions A). Moreover, we conducted condition-based sensitivity screening,33 revealing that the O2 level had no significant impact on the reaction. However, this reaction exhibited moderate sensitivity to concentration, moisture, temperature, and scale.
Table 1 Selected examples of the optimization of reaction conditionsa
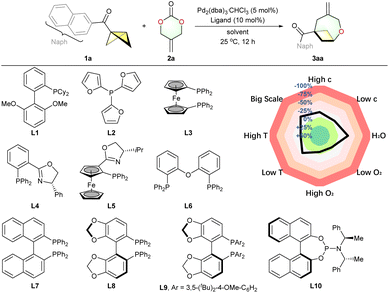
|
Entry |
Ligand |
Solvent |
Conversion of 1ab (%) |
Conversion of 2ab (%) |
Yieldb (%) |
Reaction conditions: 1a (0.10 mmol), 2a (0.12 mmol), Pd2(dba)3·CHCl3 (5 mol%) and ligand (10 mol%), solvent (2 mL), 25 °C, under N2 for 12 h.
Determined by 1H NMR spectroscopy using CH2Br2 as the internal standard.
|
1 |
L1
|
Toluene |
36 |
100 |
0 |
2 |
L2
|
Toluene |
<5 |
100 |
0 |
3 |
L3
|
Toluene |
34 |
100 |
0 |
4 |
L4
|
Toluene |
42 |
100 |
11 |
5 |
L5
|
Toluene |
58 |
100 |
14 |
6 |
L6
|
Toluene |
44 |
100 |
0 |
7 |
L7
|
Toluene |
50 |
100 |
0 |
8 |
L8
|
Toluene |
10 |
100 |
0 |
9 |
L9
|
Toluene |
66 |
100 |
49 |
10 |
L10
|
Toluene |
8 |
73 |
0 |
11 |
L9
|
PhCl |
75 |
100 |
40 |
12 |
L9
|
CH2Cl2 |
30 |
100 |
10 |
13 |
L9
|
EtOAc |
64 |
100 |
53 |
14 |
L9
|
THF |
85 |
100 |
71 |
15 |
L9
|
1,4-Dioxane |
>99 |
100 |
96 |
With optimized reaction conditions established, we first examined the substrate scope with respect to the BCBs 1. As shown in Scheme 2, in addition to naphthyl BCB ketone 1a, phenyl BCB ketones (3ba–da) with substituents in the para- and meta-positions were compatible with our catalyst system and afforded the corresponding oxa-BCOs in moderate to good yield. The cycloadditions of furan-2-yl- and thiophen-2-yl-containing BCB ketones were also successful (3ea–fa). Aliphatic BCB ketones, such as 1p, are also tolerated, yet with eroded yield. Notably, the functionalized alkynyl-substituted Malins's BCB,34 not previously reported in cycloaddition reactions, selectively forms the desired cycloadduct 3ha with a 63% yield.
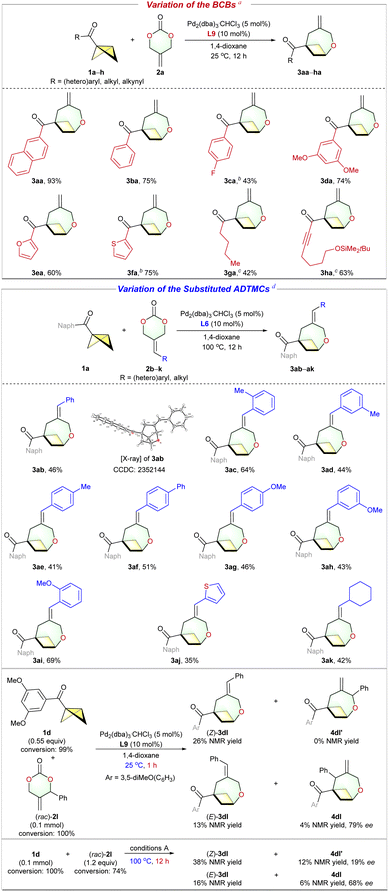 |
| Scheme 2 Substrate scope of the Pd-catalyzed (4 + 3) cycloaddition. a Standard conditions A: 1 (0.20 mmol), 2 (0.24 mmol), Pd2(dba)3·CHCl3 (5 mol%), and L9 (10 mol%) in 1,4-dioxane (4 mL) at 25 °C under a N2 atmosphere for 12 h. Isolated yields of 3 were reported. b Reaction was run at 0.15 mmol scale. c Run at 100 °C. d Standard conditions B: 1 (0.10 mmol), 2 (0.20 mmol), Pd2(dba)3·CHCl3 (5 mol%), and L6 (10 mol%) in 1,4-dioxane (2 mL) at 100 °C under a N2 atmosphere for 12 h. | |
Although (4 + 2) cycloadditions of ADTMCs are well established,28 the corresponding (4 + 3) reactions are relatively rare. Currently, there have been only two successful examples.29g,h However, the (4 + 3) reactions reported independently by Chen29g and Li29h are not compatible with substituted ADTMCs. This result can be attributed to the additional challenges in controlling regioselectivity (such as the by-products 4ab and 5ab in Scheme 3) and Z/E selectivity when using the substituted ADTMCs as oxa-1,4-dipole synthons. Under conditions A, 1a and phenyl-2-alkylidenetrimethylene carbonate 2b were converted into product 3ab, but the yield was low (13% NMR yield). The structure and E/Z geometry of the C
C bond in 3ab were determined using X-ray single crystal diffraction.35 After numerous attempts, the optimized reaction conditions B successfully facilitated the (4 + 3) cycloaddition of 1a and 2b, resulting in a 53% NMR yield of the desired 3ab together with some unidentified by-products (<10% NMR yield) (Scheme 3 bottom, see the ESI for details). Next, we investigated the reaction of the substituted ADTMCs with substituents at the ortho-, meta- or para-position of the phenyl ring (Scheme 2, middle, 3ab–ai). In general, substrates (3ac and 3ai) with a substituent group at the ortho-position of the phenyl ring showed higher yields compared to substrates with meta- or para-substituted phenyl rings. Gratifyingly, the reaction was not limited to ADTMCs with substituted phenyl group; the heteroaryl substrate 2j, and the cycloalkyl substrate 2k also underwent the reaction smoothly. Moreover, the reaction between BCB 1d (0.55 equiv.) and racemic 5-methylene-4-phenyl-1,3-dioxan-2-one 2l yielded a mixture of (Z)-3dl and (E)-3dl, along with chiral (4 + 3) cycloadduct 4dl, with a 4% NMR yield and 79% ee after 1 hour at room temperature. When the reaction was conducted at 100 °C with 1.2 equivalents of (rac)-2l, it produced four isomers, namely (Z)-3dl, (E)-3dl, 4dl′ (19% ee), and 4dl (68% ee), in a ratio of about 52
:
24
:
16
:
8. The unreacted ADTMC 2l was obtained with an enantiomeric excess of 1% (Scheme 2 bottom).
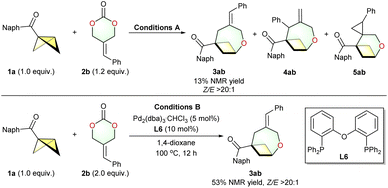 |
| Scheme 3 Optimized reaction conditions B for the (4 + 3) cycloadditions of BCBs with the substituted ADTMCs. | |
To demonstrate the synthetic utility of these (4 + 3) cycloadducts, several transformations of 3aa were investigated (Scheme 4). Firstly, we found that the dihydroxylation of the exo-methylene moiety of 3aa using K2OsO4–2H2O/NMO afforded diol 6 in 93% yield. Moreover, the epoxidation of the double bond was accomplished with m-CPBA (3aa → 7). Alternatively, the double bond could be hydrogenated to produce the corresponding 8 in good yield. In addition, adduct 3aa could be converted into a variety of oxa-bicyclo[4.1.1]octane derivatives by Wittig olefination (9), reduction (10) and condensation reaction (11).
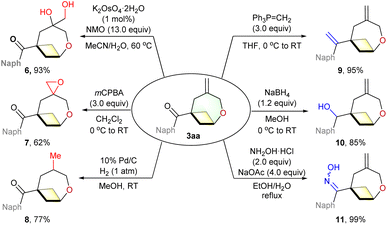 |
| Scheme 4 Synthetic transformations. | |
Based on our experimental observations and relevant literature studies, we proposed a plausible mechanism to illustrate the catalytic cycle (Scheme 5). First, Pd2(dba)3.CHCl3 and the P-ligand formed a [Pd0]/L complex. Then, oxidative addition of the ADTMCs 1 to palladium(0) gives π-allylpalladium carbonate int-I. Successive decarboxylation forms π-allyl palladium 1,4-O/C dipole species int-II. The oxygen anion in the zwitterionic allylpalladium intermediates int-II facilitates nucleophilic ring-opening of BCBs, generating intermediate int-III. Finally, intramolecular allyation of int-III affords the target (4 + 3) product 3 and regenerates the palladium catalyst. Therefore, in the O-homoconjugate addition process (int-II → int-III), the monosubstituted BCB ketones could serve as more effective electrophiles compared to the 1,3-disubstituted BCB ketones for producing the desired cycloadducts.
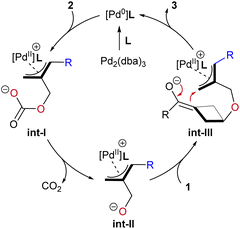 |
| Scheme 5 Proposed mechanism. | |
Conclusions
In conclusion, a new strategy based on the palladium catalysis toward the atom-economic synthesis of uncommon yet significant 2-oxabicyclo[4.1.1]octane frameworks (oxa-BCOs) has been presented. This represents the first hetero-(4 + 3) cycloaddition of BCBs.36 The utilization of easily accessible starting materials, gentle reaction conditions, broad functional group tolerance, and versatile functionalizations of the functionalized oxa-BCOs products renders this approach highly practical and appealing. The increasing significance of bicyclo[4.1.1]octanes in the quest for new bridged bicyclic molecules with bioisosteric properties leads us to believe that our protocol would not only enrich the toolkit of synthetic chemists but also greatly enlarge the (hetero-)bicyclo[n.1.1]alkanes library accessible for drug discovery.
Data availability
The data supporting this article have been included as part of the ESI.† All detailed procedures, characterization data and NMR spectra are available in the ESI.†
Author contributions
X.-Y. G. and L. T. performed all of the experiments and analysed their results. X.-Y. G., X. Z. and J.-J. F. wrote the manuscript. J.-J. F. conceived the catalytic system and directed the project.
Conflicts of interest
There are no conflicts to declare.
Acknowledgements
We are grateful to the Fundamental Research Funds for the Central Universities. The 1H, 13C NMR spectra, HRMS (ESI) and single crystal X-ray diffraction were performed at Analytical Instrumentation Center of Hunan University.
Notes and references
-
(a) Y. Xue and G. Dong, Acc. Chem. Res., 2022, 55, 2341–2354 CrossRef CAS PubMed;
(b) L. Min, X. Liu and C.-C. Li, Acc. Chem. Res., 2020, 53, 703–718 CrossRef CAS PubMed;
(c) J. Liu, X. Liu, J. Wu and C.-C. Li, Chem, 2020, 6, 579–615 CrossRef CAS;
(d) C.-H. Liu and Z.-X. Yu, Angew. Chem., Int. Ed., 2017, 56, 8667–8671 CrossRef CAS;
(e) T. P. Stockdale and C. M. Williams, Chem. Soc. Rev., 2015, 44, 7737–7763 RSC;
(f) M. Ruiz, P. López-Alvarado, G. Giorgi and J. C. Menéndez, Chem. Soc. Rev., 2011, 40, 3445–3454 RSC.
-
(a) F. Lovering, J. Bikker and C. Humblet, J. Med. Chem., 2009, 52, 6752–6756 CrossRef CAS;
(b) F. Lovering, MedChemComm, 2013, 4, 515–519 RSC.
-
(a) M. A. M. Subbaiah and N. A. Meanwell, J. Med. Chem., 2021, 64, 14046–14128 CrossRef CAS;
(b) P. K. Mykhailiuk, Org. Biomol. Chem., 2019, 17, 2839–2849 RSC;
(c) V. V. Semeno, V. O. Vasylchenko, I. M. Fesun, L. Yu. Ruzhylo, M. O. Kipriianov, K. P. Melnykov, A. Skreminskyi, R. Iminov, P. Mykhailiuk, B. V. Vashchenko and O. O. Grygorenko, Chem.–Eur. J., 2023, e202303859 Search PubMed.
- For representative reviews, see:
(a) B. R. Shire and E. A. Anderson, JACS Au, 2023, 3, 1539–1553 CrossRef CAS PubMed;
(b) J. M. Anderson, N. D. Measom, J. A. Murphy and D. L. Poole, Angew. Chem., Int. Ed., 2021, 60, 24754–24769 CrossRef CAS;
(c) M. M. D. Pramanik, H. Qian, W.-J. Xiao and J.-R. Chen, Org. Chem. Front., 2020, 7, 2531–2537 RSC;
(d) F.-S. He, S. Xie, Y. Yao and J. Wu, Chin. Chem. Lett., 2020, 31, 3065–3072 CrossRef CAS;
(e) J. Kanazawa and M. Uchiyama, Synlett, 2019, 30, 1–11 CrossRef CAS ; For selected examples, see:;
(f) J.-X. Zhao, Y.-X. Chang, C. He, B. J. Burke, M. R. Collins, M. D. Bel, J. Elleraas, G. M. Gallego, T. P. Montgomery, J. J. Mousseau, S. K. Nair, M. A. Perry, J. E. Spangler, J. C. Vantourout and P. S. Baran, Proc. Natl. Acad. Sci. U. S. A., 2021, 28, e2108881118 CrossRef;
(g) X. Zhang, R. T. Smith, C. Le, S. J. McCarver, B. T. Shireman, N. I. Carruthers and D. W. C. MacMillan, Nature, 2020, 580, 220–226 CrossRef CAS;
(h) S. Yu, C. Jing, A. Noble and V. K. Aggarwal, Angew. Chem., Int. Ed., 2020, 59, 3917–3921 CrossRef CAS;
(i) Y. Yang, J. Tsien, J. M. E. Hughes, B. K. Peters, R. R. Merchant and T. Qin, Nat. Chem., 2021, 13, 950–955 CrossRef CAS PubMed.
-
(a) A. Denisenko, P. Garbuz, N. M. Voloshchuk, Y. Holota, G. Al-Maali, P. Borysko and P. K. Mykhailiuk, Nat. Chem., 2023, 15, 1155–1163 CrossRef CAS;
(b) V. V. Levterov, Y. Panasyuk, V. O. Pivnytska and P. K. Mykhailiuk, Angew. Chem.,
Int. Ed., 2020, 59, 7161–7167 CrossRef CAS;
(c) V. V. Levterov, Y. Panasiuk, O. Shablykin, O. Stashkevych, K. Sahun, A. Rassokhin, I. Sadkova, D. Lesyk, A. Anisiforova, Y. Holota, P. Borysko, I. Bodenchuk, N. M. Voloshchuk and P. K. Mykhailiuk, Angew. Chem., Int. Ed., 2024, e202319831 CAS;
(d) A. Denisenko, P. Garbuz, S. V. Shishkina, N. M. Voloshchuk and P. K. Mykhailiuk, Angew. Chem., Int. Ed., 2020, 59, 20515–20521 CrossRef CAS;
(e) A. Denisenko, P. Garbuz, Y. Makovetska, O. Shablykin, D. Lesyk, G. Al-Maali, R. Korzh, I. V. Sadkova and P. K. Mykhailiuk, Chem. Sci., 2023, 14, 14092–14099 RSC;
(f) R. Jeyaseelan, M. Utikal, C. G. Daniliuc and L. Næsborg, Chem. Sci., 2023, 14, 11040–11044 RSC;
(g) M. Reinhold, J. Steinebach, C. Golz and J. C. L. Walker, Chem. Sci., 2023, 14, 9885–9891 RSC;
(h) J. M. Posz, N. Sharma, P. A. Royalty, Y. Liu, C. Salome, T. C. Fessard and M. K. Brown, J. Am. Chem. Soc., 2024, 146, 10142–10149 CrossRef CAS PubMed.
-
(a) D. Dibchak, M. Snisarenko, A. Mishuk, O. Shablykin, L. Bortnichuk, O. Klymenko-Ulianov, Y. Kheylik, I. V. Sadkova, H. S. Rzepa and P. K. Mykhailiuk, Angew. Chem., Int. Ed., 2023, e202304246 CAS;
(b) A. S. Harmata, T. E. Spiller, M. J. Sowden and C. R. J. Stephenson, J. Am. Chem. Soc., 2021, 143, 21223–21228 CrossRef CAS PubMed;
(c) T. Iida, J. Kanazawa, T. Matsunaga, K. Miyamoto, K. Hirano and M. Uchiyama, J. Am. Chem. Soc., 2022, 144, 21848–21852 CrossRef CAS;
(d) N. Frank, J. Nugent, B. R. Shire, H. D. Pickford, P. Rabe, A. J. Sterling, T. Zarganes-Tzitzikas, T. Grimes, A. L. Thompson, R. C. Smith, C. J. Schofield, P. E. Brennan, F. Duarte and E. A. Anderson, Nature, 2022, 611, 721–726 CrossRef CAS PubMed.
- A. Cairncross and E. P. Blanchard Jr, J. Am. Chem. Soc., 1966, 88, 496–504 CrossRef CAS.
-
(a) P. Wipf and M. A. A. Walczak, Angew. Chem., Int. Ed., 2006, 45, 4172–4175 CrossRef CAS;
(b) M. A. A. Walczak, T. Krainz and P. Wipf, Acc. Chem. Res., 2015, 48, 1149–1158 CrossRef CAS PubMed.
-
(a) R. Kleinmans, T. Pinkert, S. Dutta, T. O. Paulisch, H. Keum, C. G. Daniliuc and F. Glorius, Nature, 2022, 605, 477–482 CrossRef CAS PubMed;
(b) Y. Liang, R. Kleinmans, C. G. Daniliuc and F. Glorius, J. Am. Chem. Soc., 2022, 144, 20207–20213 CrossRef CAS PubMed;
(c) R. Kleinmans, S. Dutta, K. Ozols, H. Shao, F. Schäfer, R. E. Thielemann, H. T. Chan, C. G. Daniliuc, K. N. Houk and F. Glorius, J. Am. Chem. Soc., 2023, 145, 12324–12332 CrossRef CAS PubMed;
(d) S. Dutta, Y.-L. Lu, J. E. Erchinger, H. Shao, E. Studer, F. Schäfer, H. Wang, D. Rana, C. G. Daniliuc, K. N. Houk and F. Glorius, J. Am. Chem. Soc., 2024, 146, 5232–5241 CrossRef CAS PubMed;
(e) S. Dutta, D. Lee, K. Ozols, C. G. Daniliuc, R. Shintani and F. Glorius, J. Am. Chem. Soc., 2024, 146, 2789–2797 CrossRef CAS PubMed;
(f) Y. Liang, F. Paulus, C. G. Daniliuc and F. Glorius, Angew. Chem., Int. Ed., 2023, e202305043 CAS;
(g) Y. Liang, R. Nematswerani, C. G. Daniliuc and F. Glorius, Angew. Chem., Int. Ed., 2024, e202402730 CAS;
(h) H. Wang, H. Shao, A. Das, S. Dutta, H. T. Chan, C. Daniliuc, K. N. Houk and F. Glorius, Science, 2023, 381, 75–81 CrossRef CAS PubMed.
- R. Guo, Y.-C. Chang, L. Herter, C. Salome, S. E. Braley, T. C. Fessard and M. K. Brown, J. Am. Chem. Soc., 2022, 144, 7988–7994 CrossRef CAS PubMed.
-
(a) K. Dhake, K. J. Woelk, J. Becica, A. Un, S. E. Jenny and D. C. Leitch, Angew. Chem., Int. Ed., 2022, 61, e202204719 CrossRef CAS;
(b) K. J. Woelk, K. Dhake, N. D. Schley and D. C. Leitch, Chem. Commun., 2023, 59, 13847–13850 RSC.
- S. Agasti, F. Beltran, E. Pye, N. Kaltsoyannis, G. E. M. Crisenza and D. J. Procter, Nat. Chem., 2023, 15, 535–541 CrossRef CAS PubMed.
- Y. Zheng, W. Huang, R. K. Dhungana, A. Granados, S. Keess, M. Makvandi and G. A. Molander, J. Am. Chem. Soc., 2022, 144, 23685–23690 CrossRef CAS PubMed.
-
(a) M. Xu, Z. Wang, Z. Sun, Y. Ouyang, Z. Ding, T. Yu, L. Xu and P. Li, Angew. Chem., Int. Ed., 2022, 61, e202214507 CrossRef CAS PubMed;
(b) T. Yu, J. Yang, Z. Wang, Z. Ding, M. Xu, J. Wen, L. Xu and P. Li, J. Am. Chem. Soc., 2023, 145, 4304–4310 CrossRef CAS PubMed.
- Y. Liu, S. Lin, Y. Li, J.-H. Xue, Q. Li and H. Wang, ACS Catal., 2023, 13, 5096–5103 CrossRef CAS.
- T. V. T. Nguyen, A. Bossonnet, M. D. Wodrich and J. Waser, J. Am. Chem. Soc., 2023, 145, 25411–25421 CrossRef CAS PubMed.
- N. Radhoff, C. G. Daniliuc and A. Studer, Angew. Chem., Int. Ed., 2023, e202304771 CAS.
- D. Ni, S. Hu, X. Tan, Y. Yu, Z. Li and L. Deng, Angew. Chem., Int. Ed., 2023, e202308606 CAS.
- L. Tang, Y. Xiao, F. Wu, J.-L. Zhou, T.-T. Xu and J.-J. Feng, Angew. Chem., Int. Ed., 2023, 62, e202310066 CrossRef CAS PubMed.
- J. Zhang, J.-Y. Su, H. Zheng, H. Li and W.-P. Deng, Angew. Chem., Int. Ed., 2024, e202318476 CAS.
- M. de Robichon, T. Kratz, F. Beyer, J. Zuber, C. Merten and T. Bach, J. Am. Chem. Soc., 2023, 145, 24466–24470 CAS.
- Q. Fu, S. Cao, J. Wang, X. Lv, H. Wang, X. Zhao and Z. Jiang, J. Am. Chem. Soc., 2024, 146, 8372–8380 CrossRef CAS PubMed.
-
(a) A. D. Meijere, H. Wenck and F. Seyed-Mahdavi, Tetrahedron, 1986, 42, 1291–1297 CrossRef;
(b) B. D. Schwartz, A. P. Smyth, P. E. Nashar, M. G. Gardiner and L. R. Malins, Org. Lett., 2022, 24, 1268–1273 CrossRef CAS PubMed;
(c) M. Wang, Y. Huang, C. Li and P. Lu, Org. Chem. Front., 2022, 9, 2149–2153 RSC;
(d) H. Yan, Y. Liu, X. Feng and L. Shi, Org. Lett., 2023, 25, 8116–8120 CrossRef CAS PubMed;
(e) H. Ren, T. Li, J. Xing, Z. Li, Y. Zhang, X. Yu and J. Zheng, Org. Lett., 2024, 26, 1745–1750 CrossRef CAS;
(f) L. Yang, H. Wang, M. Lang, J. Wang and S. Peng, Org. Lett., 2024, 26, 4104–4110 CrossRef CAS PubMed.
-
(a) D. E. Applequist and J. W. Wheeler, Tetrahedron Lett., 1977, 18, 3411–3412 CrossRef;
(b) R. Bychek and P. K. Mykhailiuk, Angew. Chem., Int. Ed., 2022, 61, e202205103 CrossRef CAS PubMed;
(c) R. M. Bychek, V. Hutskalova, Y. P. Bas, O. A. Zaporozhets, S. Zozulya, V. V. Levterov and P. K. Mykhailiuk, J. Org. Chem., 2019, 84, 15106–15117 CrossRef CAS PubMed;
(d) X. Ma, D. L. Sloman, Y. Han and D. J. Bennett, Org. Lett., 2019, 21, 7199–7203 CrossRef CAS PubMed;
(e) X. Ma, W. Pinto, L. N. Pham, D. L. Sloman and Y. Han, Eur. J. Org Chem., 2020, 4581–4605 CrossRef CAS;
(f) R. E. McNamee, A. L. Thompson and E. A. Anderson, J. Am. Chem. Soc., 2021, 143, 21246–21251 CrossRef CAS PubMed.
-
(a) S. J. Sujansky and X. Ma, Asian J. Org. Chem., 2024, e202400045 CrossRef CAS;
(b) P. Bellotti and F. Glorius, J. Am. Chem. Soc., 2023, 145, 20716–20732 CrossRef CAS PubMed;
(c) M. Golfmann and J. C. L. Walker, Commun. Chem., 2023, 6, 9 CrossRef CAS PubMed;
(d) C. B. Kelly, J. A. Milligan, L. J. Tilley and T. M. Sodano, Chem. Sci., 2022, 13, 11721–11737 RSC;
(e) J. Turkowska, J. Durkaab and D. Gryko, Chem. Commun., 2020, 56, 5718–5734 RSC;
(f) A. Fawcett, Pure Appl. Chem., 2020, 92, 751–765 CrossRef CAS.
-
(a)
H. B. Josien, J. W. Clader, W. J. Greenlee, M. J. Mayer, J. L. Davis and S. Wan, WO pat. 2010085528 A1, 2010 Search PubMed;
(b)
H. B. Josien, J. W. Clader, W. J. Greenlee, M. J. Mayer, J. L. Davis and S. Wan, US pat. 20110312967 A1, 2011 Search PubMed;
(c) Q. Wang, J. Han and B. Bao, J. Food Biochem., 2017, 41, e12320 CrossRef.
-
(a) C. Mülle, A. Bauer, M. M. Maturi, M. C. Cuquerella, M. A. Miranda and T. Bach, J. Am. Chem. Soc., 2011, 133, 16689–16697 CrossRef PubMed;
(b) K. A. B. Austin, E. Herdtweck and T. Bach, Angew. Chem., Int. Ed., 2011, 50, 8416–8419 CrossRef CAS PubMed;
(c) A. Oku, Y. Sawada, M. Schroeder, I. Higashikubo, T. Yoshida and S. Ohki, J. Org. Chem., 2004, 69, 1331–1336 CrossRef CAS PubMed;
(d) B. B. Snider and R. A. H. F. Hui, J. Org. Chem., 1985, 50, 5167–5176 CrossRef CAS;
(e) B. B. Snider, R. A. H. F. Hui and Y. S. Kulkarni, J. Am. Chem. Soc., 1985, 107, 2194–2196 CrossRef CAS.
- H.-J. Cho and J. H. Kim, Org. Biomol. Chem., 2023, 21, 9507–9518 RSC.
-
(a) M.-M. Li, B.-L. Qu, Y.-Q. Xiao, W.-J. Xiao and L.-Q. Lu, Sci. Bull., 2021, 66, 1719–1722 CrossRef CAS PubMed;
(b) H. Xie, L. Chen, Z. Han, Z. Yang, J. Sun and H. Huang, Org. Lett., 2023, 25, 5011–5016 CrossRef CAS PubMed;
(c) Q. Liu, T.-X. Liu, Y. Ru, X. Zhu and G. Zhang, Chem. Commun., 2019, 55, 14498–14501 RSC;
(d) P.-H. Dou, S.-P. Yuan, Y. Chen, J.-Q. Zhao, Z.-H. Wang, Y. You, Y.-P. Zhang, M.-Q. Zhou and W.-C. Yuan, J. Org. Chem., 2022, 87, 6025–6037 CrossRef CAS PubMed;
(e) L. Chen, H. Xie, Y. Xue, Z. Han, J. Sun and H. Huang, Chin. J. Chem., 2024, 42, 829–834 CrossRef CAS;
(f) R. Shintani, K. Moriya and T. Hayashi, Chem. Commun., 2011, 47, 3057–3059 RSC;
(g) Z. Chen, Z.-C. Chen, W. Du and Y.-C. Chen, Org. Lett., 2021, 23, 8559–8564 CrossRef CAS PubMed;
(h) Y. Meng, M. Song, Y. Wang, Y. Wang and E.-Q. Li, Org. Chem. Front., 2023, 10, 2648–2652 RSC;
(i) H. Uno, K. Kawai, T. Araki, M. Shiro and N. Shibata, Angew. Chem., Int. Ed., 2022, 61, e202117635 CrossRef CAS PubMed;
(j) B. Mao, H. Liu, Z. Yan, Y. Xu, J. Xu, W. Wang, Y. Wu and H. Guo, Angew. Chem., Int. Ed., 2020, 59, 11316–11320 CrossRef CAS PubMed.
-
(a) C.-Z. Zhu, J.-J. Feng and J. Zhang, Angew. Chem., Int. Ed., 2017, 56, 1351–1355 CrossRef CAS PubMed;
(b) L. Tang, Q.-N. Huang, F. Wu, Y. Xiao, J.-L. Zhou, T.-T. Xu, W.-B. Wu, S. Qu and J.-J. Feng, Chem. Sci., 2023, 14, 9696–9703 RSC;
(c) Y. Xiao, T.-T. Xu, J.-L. Zhou, F. Wu, L. Tang, R.-Y. Liu, W.-B. Wu and J.-J. Feng, Chem. Sci., 2023, 14, 13060–13066 RSC.
- For Pd-catalyzed ring-opening of BCBs, see:
(a) A. Fawcett, T. Biberger and V. K. Aggarwal, Nat. Chem., 2019, 11, 117–122 CrossRef CAS PubMed;
(b) Z. Zhang and V. Gevorgyan, J. Am. Chem. Soc., 2022, 144, 20875–20883 CrossRef CAS PubMed.
- We presented a compilation of unsuccessful substrate scopes in the ESI. The importance of failed experiments, see: F. Strieth-Kalthoff, F. Sandfort, M. Kühnemund, F. R. Schäfer, H. Kuchen and F. Glorius, Angew. Chem., Int. Ed., 2022, 61, e202204647 CrossRef CAS PubMed.
- L. Pitzer, F. Schäfers and F. Glorius, Angew. Chem., Int. Ed., 2019, 58, 8572–8576 CrossRef CAS PubMed.
- B. D. Schwartz, M. Y. Zhang, R. H. Attard, M. G. Gardiner and L. R. Malins, Chem.–Eur. J., 2020, 26, 2808–2812 CrossRef CAS PubMed.
- CCDC 2352144 (3ab).
- During the peer review of our manuscript, two studies on the (4 + 3) cycloadditions of BCBs were conducted using a Lewis acid catalysis approach
(a) J.-J. Wang, L. Tang, Y. Xiao, W.-B. Wu, G. Wang and J.-J. Feng, Angew. Chem., Int. Ed., 2024, 63, e202405222 CrossRef CAS PubMed;
(b) S. Nicolai and J. Waser, Chem. Sci., 2024, 15, 10823–10829 RSC.
|
This journal is © The Royal Society of Chemistry 2024 |
Click here to see how this site uses Cookies. View our privacy policy here.