Polycyclic aromatic hydrocarbons in silicone wristbands of Uruguayan children: measurement and exposure source exploration†
Received
29th November 2023
, Accepted 26th March 2024
First published on 5th April 2024
Abstract
Polycyclic aromatic hydrocarbons (PAHs) pose health risks to children, potentially resulting in stunted growth, obesity, and cognitive deficits, but lack of reliable and noninvasive means to measure PAHs results in poor understanding of exposure patterns and sources in this vulnerable population. In this study, 24 children aged ∼7 years (9 boys and 15 girls) from Montevideo, Uruguay wore silicone wristbands for 8 days to monitor the exposure of 27 PAHs. Wristbands were extracted using a modified ethyl acetate tandem solid phase extraction clean up and then analyzed via gas chromatography with tandem mass spectrometry. This analysis has reported LODs for 27 PAHs between 0.05 and 3.91 μg L−1. Eighteen PAHs were detected in >50% of the samples with concentration medians ranging 1.2–16.3 ng g−1 of wristband. Low molecular weight PAHs (2–3 rings) such as naphthalene and its alkyl derivatives were highly correlated (0.7–0.9) in the wristbands, suggesting exposure from related sources. Exposure source exploration focused on secondhand tobacco smoke, potentially through caregivers who reported on smoking habits in an associated survey. A principal components analysis (PCA) was conducted to examine patterns in PAH compounds detected in the wristbands; subsequently, the resulting components were compared according to current smoking among caregivers. The PCA analysis revealed a grouping of participants based on higher exposure of 1-methyl naphthalene, pyrene, fluoranthene, 1-methylphenanthrene, dibenzothiophene and 2-phenyl naphthalene. The derived components did relate with parental smoking, suggesting that some participants experienced exposure to a common source of certain PAHs outside of parental smoking. This is the first study to assess PAH exposure in young children from South America. Using wristbands, our study indicates exposure to multiple, potentially harmful chemicals. Wristbands could provide a comprehensive picture of PAH exposure in children, complementing other non-invasive biomonitoring approaches.
Environmental significance
Polycyclic aromatic hydrocarbons (PAHs) are pollutants of concern due to their ubiquitous environmental presence and the elevated risk they can present to children's health. Continuing to advance monitoring techniques for PAH levels in children is important for taking steps in mitigating exposure. In this initial study we are one of the first groups to analyze PAH exposure in children using silicone wristbands as passive samplers. We detected 27 unique PAHs including some recognized to be carcinogenic which were quantified, and a paired statistical analysis explored secondhand tobacco smoke as a potential exposure source.
|
1. Introduction
Polycyclic aromatic hydrocarbons (PAHs) are byproducts of incomplete combustion of fossil fuels, biomass, cigarettes,1 and other sources. Low molecular weight PAHs (2 or 3 rings) such as naphthalene and anthracene are products of low temperature combustion, whereas high temperature combustion leads to larger PAHs with 5 or 6 rings.2 PAHs in the environment can also originate from industrial/commercial production of pigments, plastics, pharmaceuticals, and pesticides.3 Furthermore, several common foods, including grilled/roasted foods,4,5 dairy, and vegetables5 could contain PAHs. In one study, bread and potatoes were contaminated with naphthalene, acenaphthene and fluorene, although the origin of these contaminants was not clear.6 In Kuwait, 16 PAHs were detected in 60–100% of food products tested, including meats and vegetables, and indicated that children could potentially ingest over 1000 ng PAHs per day.7 The United States (US) Environmental Protection Agency (EPA) considers 16 PAHs to pose significant human health risks,8 and has set the permissible exposure levels of PAHs in air at 0.2 mg m−3 and maximum contaminant level in water at 0.1–0.4 μg L−1.9
Children are more at risk of and vulnerable to PAH exposure compared to adults due to their greater sensitivity and lower body weight, which increases the dose effect of a chemical.10 Children also ingest more food, water, and air than adults by body weight.11 Additionally, infants can have high exposure to PAHs from dust when they explore their environments by crawling, frequently touching surfaces, and placing objects in their mouths.12 Once PAHs are absorbed by the body, they can undergo biotransformation through cytochrome P450 to form phenols, which can further transform to radicals and epoxides including hydroxylated PAHs.13 PAHs have been linked to several adverse health outcomes in children. For example, prenatal exposure to PAHs was negatively associated with nonverbal reasoning and intelligence in 5 year old children.14 In another study, PAH metabolites in air and cord blood were positively related with anxiety, attention disorders, and learning disabilities.15 There is some evidence from school children in Malaysia living close to petrochemical industrial plants that higher PAH exposure may be related to DNA damage.16 Because of these health risks, it is important to have non-invasive and easily implemented methods to rapidly and efficiently evaluate chemical pollutant exposure in large populations.
Silicone wristbands are increasingly used as passive personal samplers for organic pollutants due to their low cost and minimal burden to the participants. Anderson and colleagues employed silicone wristbands to detect occupational exposure to PAHs, among other organic pollutants, over periods of days to weeks.17 Since then, multiple studies have evaluated organic pollutants including PAHs in wristbands among various age groups, geographic settings, and time frames.18–22 Only 2 papers have reported on analyses of PAHs in wristbands to assess exposure in children (age 3–17 years).23,24 One study, conducted among students aged 15–17 years in Chile, measured multiple semi volatile organic compounds including PAHs.24 Another reported PAHs and environmental contaminants in wristbands of participants as young as 3 years across three continents.23 Recent reviews by our group25 and others26 highlight the lack of studies on PAH exposure in children.
Our objective was to expand the understanding of the frequency and concentrations of PAHs detected in wristbands worn by children aged ∼7 years living in an urban setting. Furthermore, with 45–54% of mothers and fathers being current smokers, we hypothesized that PAH exposure in children is related to smoking habits of caregivers.
2. Materials and methods
2.1 Scola-Exposome study
The Scola-Exposome study was previously described in detail by Travis et al.27 Briefly the study was conducted in Montevideo, Uruguay among first-grade children (ages 6.0–7.8 years). The children (n = 24) were recruited for this pilot study during ongoing research activities of the Salud Ambiental Montevideo (SAM) research program conducted by the Catholic University of Uruguay and the University at Buffalo. Caregivers provided separate informed consent to participate in Scola-Exposome, under IRB protocols approved by the University at Buffalo (STUDY #2664) and the Catholic University of Uruguay. Demographic information was obtained through the larger SAM assessment and included family work history and structure, hygiene habits, income, education, and smokers in the home.
2.2 Wristband deployment and collection
Silicone wristbands were purchased from https://24hourwristbands.com (Houston, TX). Wristbands were cleaned before deployment with solvent washes to remove any potential contamination.17 Briefly, wristbands were soaked in ethyl acetate/hexane (1
:
1 v/v) and shaken with an INNOVA platform shaker at 60 RPM for 2.5 hours. This was repeated 3 times. Two more extractions were performed with ethyl acetate/methanol (1
:
1 v/v) and shaken at 60 RPM for 2.5 hours. Air dried wristbands were stored in acid washed amber glass jars and sent to Montevideo for deployment. Participating children put on their own wristbands and were instructed to wear them continuously during all activities, including sleep, for 8 consecutive days. At the end of the sampling period, each participant returned their own band to a corresponding acid washed amber jar. The study took place between July and December 2018. Upon conclusion of field work, all wristbands were shipped to the University at Buffalo, where they were stored at −40 °C.
2.3 Blood collection for hemoglobin and lead analysis
Fasting venous blood was collected by an experienced nurse. A drop of venous blood was removed from one of the tubes immediately after collection and used to measure hemoglobin via a hemoglobinometer (HemoCue Inc, Lake Forest, CA). Standard controls provided by the manufacturer were used to perform daily quality control checks. For lead analysis, a second sample was collected into a heparin-coated tube (Vacutainer, Becton Dickinson, Franklin Lakes, NJ). Blood was transported on ice to the Toxicology Laboratory “CEQUIMTOX” (Specialized Center for Chemical Toxicology), of the Faculty of Chemistry, University of the Republic of Uruguay. Blood was analyzed by Graphite Furnace Atomic Absorption Spectrometry (GF-AAS, VARIAN SpectrAA-55B). The LOD ranged 0.6–0.8 μg dL−1 during the study period. The laboratory participates in the Lead and Multi-Element Proficiency Program (LAMP) and the Interlaboratory Program of Quality Control for Lead in Blood, Spain (PICC Pb-S).
2.4 Wristband extraction
The following solid standards were purchased from Accustandard® (New Haven CT); anthracene, benz(a)anthracene, phenanthrene, pyrene, fluoranthene, 1,2-dimethyl naphthalene, 1-methyl naphthalene, 2-methyl naphthalene, acenaphthene, dibenzothiophene, 2-methyl anthracene, 1,6-dimethyl naphthalene, triphenylene, 1,6,7-trimethyl naphthalene, 1-methylphenanthrene, 2-phenyl naphthalene, fluoranthene-D10, fluorene-D10. The following standards were purchased from Accustandard® in 1 mL ampules; acenaphthene-D10 (4 mg mL−1), benz(a)anthracene-D12 (0.2 mg mL−1), naphthalene-D8 (4 mg mL−1), anthracene-D10 (2 mg mL−1), chrysene-D12 (4 mg mL−1), phenanthrene-D10 (0.2 mg mL−1), pyrene-D10 (0.5 mg mL−1), 1-phenyl naphthalene (50 μg mL−1), naphthalene (0.2 mg mL−1), fluorene (0.2 mg mL−1), chrysene (0.2 mg mL−1), acenaphthylene (0.2 mg mL−1), benzo(c)phenanthrene (50 μg mL−1), benz(a)pyrene (2 mg mL−1), benz(e)pyrene (50 μg mL−1), 3,6-dimethylphenanthrene (50 μg mL−1), 1-methyl pyrene (50 μg mL−1), 2-methylphenanthrene (50 μg mL−1). HPLC grade ethyl acetate was purchased from Supelco® (Bellefonte, PA), hexanes and HPLC grade methanol were purchased from Fisher Chemical® (Pittsburg, PA), and 2,2,4 trimethyl pentane was purchased from VWR Chemicals® (Radnor, PA). A Nanopure Diamond™ system was used for ultra-high purity water (18.2 MΩ cm). Nitrogen gas and ultra-high-purity argon and helium were purchased from Airgas® (Radnor, PA).
A modified ethyl acetate extraction was used for this analysis.17 Wristbands were cut into quarters and weighed. Fragments of each wristband were placed into an acid washed 50 mL (pre-marked for 300 μL) glass tube and fortified with a 2.5 ng internal standard spike by adding and drying a 12.5 μL spike of a 1 ppm standard solution on said wristband. The internal standard mixture can be seen in Table S1.† Blank wristbands which were previously solvent washed as described earlier were also extracted to eliminate risk for false positives. Wristband fragments were shaken at 60 RPM for 2 hours, together with 20 mL ethyl acetate; then the solvent was collected and set aside. Further extraction occurred with fresh 20 mL ethyl acetate for 2 hours. The ethyl acetate extracts were pooled, then evaporated under a slow stream of N2 gas to approximately 300 μL, which ultimately was diluted to 3.3 mL with acetonitrile. Solid phase extraction (SPE) was employed as a further clean up step. For this, each acetonitrile extract was passed through a C18 SPE cartridge and the eluent was collected for analysis. This step was done to remove phospholipids and other contaminants that may interfere in the GC-MS analysis. A C18 cartridge (500 mg, 6 cm3) was conditioned with 6 mL of acetonitrile, then 3.3 mL of wristband acetonitrile extract was loaded onto the cartridge and the flow through was collected (eluate). Two additional times, 3 mL of acetonitrile was added to the original sample tube, vortexed for 30 seconds and quantitatively transferred into the same SPE cartridge, where the eluates were collected and combined (about 9.3 mL). The combined SPE eluates (collected in a pre-marked 15 mL glass tube) were evaporated to approximately 300 μL under an N2 stream, and later extracted with 1 mL of 2,2,4-trimethyl pentane spiked with 13C12-PCB-138. This extra step was done to prevent loss of volatile PAHs and to solvent exchange to an ideal solvent for GC-MS analysis. The 2,2,4-trimethyl pentane layer was taken and evaporated to 250 μL for GC-MS/MS analysis.
2.5 Gas chromatography with tandem mass spectrometry (GC-MS/MS) analysis
A detailed description of the GC-MS/MS analysis can be found in the ESI.† Briefly, analytical separation of 27 PAH analytes and 9 deuterated PAH internal standards (Table S1†) was achieved using a Thermo Scientific® (Waltham, MA) TG-5MS 30M column I.D. 0.25 mm, film thickness 0.25 μM with a 5% phenyl methyl polysiloxane stationary phase. The GC-MS/MS system used was a Thermo Scientific® TRACE GC Ultra and Thermo Scientific® TSQ Quantum Ultra MS (Waltham, MA) with an AS 3000 autosampler. Limits of detection (LOD) and limits of quantitation (LOQ) are provided in Table S1.† LODs ranged from 0.04 to 3.91 part per billion (ppb). A recovery study was done using pre-washed “blank wristbands” that were spiked before and after extraction with 100 ng L−1 for each PAH; recoveries for the 27 PAHs were determined to be 48.3–92.3%. A summary of these recoveries can be found in Table S1.† Fig. S1† shows structures for all 27 PAHs considered in this study. Quantitation was done by one-point standard addition with a 27 PAH mixture.
2.6 Statistical analysis
The mean, median, distribution plots and box plots of the PAH compounds in wristbands were generated using Graph Pad prism v.9.4. Mean, median and distribution plots were generated with values > LOD. R Studio was used to calculate the Spearman correlation coefficients (rho) among PAH compounds in wristbands, and to generate correlation matrices. Primary correlation analyses only considered PAHs which were detected in >50% of participants. STATA version 14 was employed to calculate participant characteristics (mean ± SD, range, frequencies of categories).
To further understand correlations among compounds detected in wristbands and discern common exposure patterns, we subjected the 18 compounds detected in at least 50% of the study wristbands to principal component analysis (PCA). Calculations were performed using Minitab Statistical Software (V23.2.1). Values below the limit of detection were replaced with LOD/SQRT(2). The 18 variables were normalized to the standard deviation of each variable using the equation below:
Xf = normalized measurement,
Xi = initial measurement of PAH in wristband (ng g
−1),
![[X with combining tilde]](https://www.rsc.org/images/entities/i_char_0058_0303.gif)
= average measurement of PAH in wristband among 24 participants,
sx = standard deviation measurement of PAH in wristband among 24 participants.
Results of the PCA analysis (Eigenvalues, eigenvectors, scores) are provided in Table S2.† As smoking is an important source of PAH exposure, we hypothesized that PAH compounds detected in children's wristbands would be related to caregiver smoking. To test this, we selected the two components from the PCA that explained the highest amount of variability in PAH levels and created a scatterplot. We further indicated whether the component scores were from children whose caregivers did not smoke or from households with one or both caregivers currently smoking. These graphs were completed in Microsoft Excel and Origin.
3. Results
3.1 Participant characteristics
Participant characteristics for this study can be found in Table 1 below. This study deployed wristbands to 24 participants aged 6–7.8 years old that included 37.5% boys and 62.5% girls. In nearly 50% of households both caregivers were smokers. For most children, hemoglobin was in a range considered healthy for this age group (Hb > 12.0 g dL−1).28 Lead in blood samples was detected at concentrations between 0.3 and 6.4 μg dL−1, but >80% of children had levels below the US Centers for Disease Control reference value of 3.5 μg dL−1.29
Table 1 Characteristics of the 24 children and their caregivers participating in Scola-Exposome
Characteristic |
% or mean ± SD |
Range |
Crowding defined as 2+ persons per room living in the home.
In the second half of 2018, when the study was conducted, this monthly income was equivalent to roughly 465 U.S. dollars; according to CEIC (https://www.ceicdata.com/en), the average monthly household income in Montevideo was just shy of 74 000 Uruguayan pesos.
Score derivation was previously described;30 higher score indicates greater disadvantage.
|
Child characteristics
|
Boys |
37.5% |
|
Age (months) |
82.0 ± 4.6 |
72–94 |
Years living in current house |
4.9 ± 2.4 |
0–7 |
Hemoglobin (g dL−1) |
13.2 ± 0.9 |
11.3–14.6 |
Blood lead concentration (μg dL−1) |
2.1 ± 1.8 |
0.3–6.4 |
≥ 3.5 μg dL−1 |
16.7% |
![[thin space (1/6-em)]](https://www.rsc.org/images/entities/char_2009.gif) |
Household characteristics
|
Mother's age (years) |
32.8 ± 5.9 |
24–47 |
Maternal education (years) |
8.8 ± 2.5 |
3–13 |
Mother unemployed or stay-at-home |
37.5% |
|
Parents are married or cohabitating |
75.0% |
|
Child lives with: |
|
|
Both parents |
43.5% |
Mother only |
13.0% |
Other arrangement |
43.5% |
Households with children <5 years of age |
20.8% |
|
Crowded householdsa |
29.2% |
|
Mother currently smokes |
45.8% |
|
Father currently smokes |
54.2% |
|
Families with at least 1 smoker |
70.8% |
Monthly family income < 15 000b Uruguayan pesos |
50.0% |
|
Neighborhood disadvantage factor scorec |
1.6 ± 1.1 |
−0.1 to 3.4 |
Distance to nearest bus line (m) |
165 ± 145 |
0.3–484 |
Distance to nearest gas station (m) |
1017 ± 536 |
205–1877 |
3.2 PAHs in wristbands
The wristbands were analyzed for 27 PAHs. Table S3† provides detected concentrations of each PAH in each wristband, expressed as ng g−1 of wristband. PAHs were detected in every wristband analyzed, with the number of compounds ranging 7–23. Of the 27 compounds examined, 18 were detected in more than 50% of wristbands (Table 2). The most frequently detected compounds were, in increasing order, pyrene (75%), 2-phenyl naphthalene (75%), anthracene (75%), fluorene (75%), naphthalene (83%), and 1-methyl naphthalene (96%).
Table 2 Detections of 27 PAHs in 24 wristbands including mean, median, minimum, maximum, number of detections and detection frequency. Values given as ng g−1 wristband
PAH |
Mean |
Median |
Min |
Max |
# of detections |
% detected |
Compound monitored by the EPA because of high frequency of environmental occurrence and known/suspected toxicity.
|
Naphthalenea |
3.8 |
2.8 |
0.2 |
17.0 |
20 |
83% |
1-Methyl naphthalene |
4.5 |
3.5 |
0.3 |
17.3 |
23 |
96% |
2-Methyl naphthalene |
2.7 |
2.1 |
0.0 |
10.6 |
14 |
58% |
Acenaphthylenea |
2.6 |
1.6 |
0.0 |
11.2 |
13 |
54% |
Acenaphthenea |
2.1 |
1.5 |
0.0 |
7.1 |
7 |
29% |
1,2-Dimethyl naphthalene |
2.8 |
1.9 |
0.4 |
9.7 |
8 |
33% |
1,6-Dimethyl naphthalene |
1.6 |
1.2 |
0.6 |
3.7 |
12 |
50% |
Fluorenea |
7.2 |
7.3 |
1.7 |
15.0 |
18 |
75% |
1,6,7-Trimethyl naphthalene |
5.4 |
4.7 |
1.1 |
16.7 |
12 |
50% |
Anthracenea |
21.7 |
12.1 |
0.1 |
148.6 |
18 |
75% |
Phenanthrenea |
21.4 |
4.0 |
0.5 |
67.4 |
9 |
38% |
Dibenzothiophene |
2.9 |
2.6 |
0.2 |
8.5 |
15 |
63% |
2-Methyl anthracene |
20.2 |
6.3 |
0.3 |
135.7 |
12 |
50% |
1-Methylphenanthrene |
23.6 |
10.4 |
0.3 |
132.4 |
14 |
58% |
2-Methylphenanthrene |
30.4 |
16.3 |
1.2 |
217.4 |
16 |
67% |
Pyrenea |
12.4 |
9.0 |
3.3 |
28.4 |
18 |
75% |
Fluoranthenea |
12.3 |
9.7 |
2.0 |
30.1 |
17 |
71% |
1-Phenyl naphthalene |
4.8 |
4.2 |
2.2 |
10.2 |
12 |
50% |
2-Phenyl naphthalene |
10.6 |
5.8 |
1.4 |
52.0 |
18 |
75% |
3,6-Dimethylphenanthrene |
14.5 |
4.5 |
1.1 |
98.2 |
10 |
42% |
1-Methyl pyrene |
28.2 |
10.1 |
0.8 |
152.2 |
16 |
67% |
Benz(a)anthracenea |
21.0 |
12.4 |
0.5 |
81.0 |
9 |
38% |
Benzo(c)phenanthrene |
3.7 |
3.3 |
0.1 |
8.2 |
12 |
50% |
Chrysene/triphenylenea |
4.4 |
3.4 |
1.7 |
10.1 |
11 |
46% |
Benz(a)pyrenea |
5.0 |
3.3 |
0.1 |
22.1 |
10 |
42% |
Benz(e)pyrene |
2.5 |
1.8 |
0.1 |
9.7 |
9 |
38% |
For many PAHs, the range of concentrations detected was narrow, approximately a factor of ten difference between maximum and minimum; however, for some species the lowest and highest concentrations differed by a factor of a hundred or thousand (e.g., anthracene ranged between 0.1 and 148.48 ng g−1) (Table 2).
The five species with the highest detected concentrations in increasing order were 1-methylphenanthrene (max value: 132.4 ng g−1), 2-methyl anthracene (135.6 ng g−1), anthracene (148.5 ng g−1), 1-methyl pyrene (152.1 ng g−1), and 2-methyl phenanthrene (217.3 ng g−1). Of these, 1-methyl phenanthrene also had the highest mean concentration (30.4 ng g−1). Fig. 1 shows the range of concentrations for all 27 PAH species across the 24 wristbands. Fig. S3† gives an alternate view of this data in terms of pmol PAH per g wristband.
 |
| Fig. 1 Concentration ranges of 27 PAH compounds displayed using a box plot with the lower 5th and upper 95th percentile represented by the whiskers, the 25th and 75th percentiles represented by the box edges, and the median represented by a line bisecting each box. Statistics were calculated using observed PAH measurements and detections below LOD were excluded. PAHs ordered by lowest to highest molecular weight. | |
A Spearman correlation matrix was generated comparing PAHs detected in wristbands (Fig. 2). Several compounds were correlated suggesting a common source of exposure. All correlation values and corresponding p-values are detailed in Table S4.†
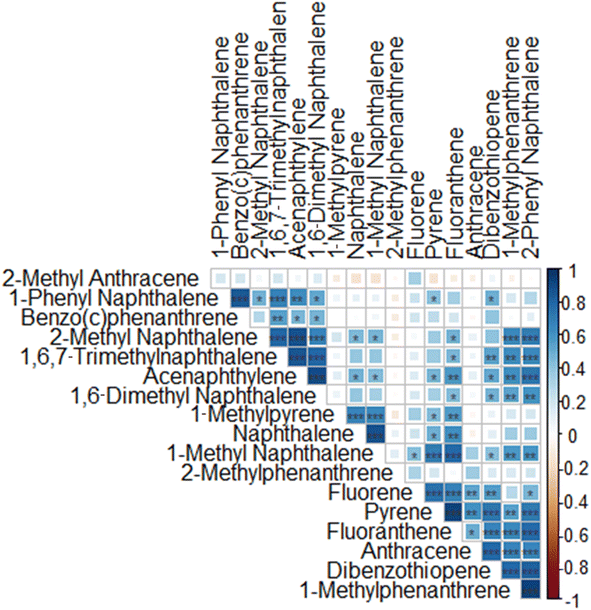 |
| Fig. 2 A Spearman correlation matrix showing the relationships between 18 PAHs which were detected in at least 50% of the analyzed wristbands. Statistics were calculated using observed values, and values below LOD or not detected were replaced with LOD/SQRT(2). Coefficients shown were calculated in R studio. Statistical significance is represented by p-values (* < 0.05. ** < 0.01. *** < 0.001). Blank cells are not considered significant. | |
3.3 PAH compounds in wristbands of children whose caregivers do or do not smoke
A PCA analysis was conducted on the 18 compounds detected in wristbands from over 50% of participants (N > 12). Fig. S2† is a scree plot showing the distribution of eigenvalues for each principal component in this analysis. The first two components plotted together explained nearly 60% of variability (PC1-43% and PC2-16% in PAH levels detected in the wristbands (Table S2B†)). A comprehensive list of eigenvectors is included in Table S2C.† PC 1–2 had eigenvectors ranging [0.01–0.32] for PC1 and [0.064–0.347] for PC2.
Fig. 3 illustrates the relationships between the two top principal components and individual loadings of the PAHs. Compounds in the right middle section are more strongly correlated with only PC1 and include 1-methyl naphthalene, pyrene, fluoranthene, 1-methylphenanthrene, dibenzothiophene and 2-phenyl naphthalene. Compounds in the upper and lower left sections correlate more closely with PC2 and include benzo(c)phenanthrene, 1-phenyl naphthalene, fluorene, anthracene, and 2-methyl anthracene. In turn, 1,6-dimethyl naphthalene, acenaphthylene, 2-methyl naphthalene and 1,6,7-trimethylnaphthalene were correlated with both PC1 and PC2. Due to the larger weight of principal component 1, we will focus on its impact on data variance.
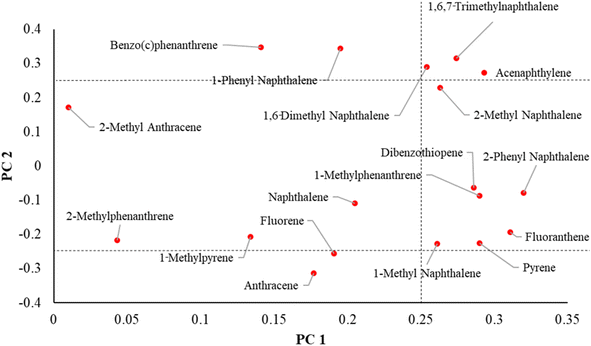 |
| Fig. 3 A 2-dimensional plot of PC1 and PC2 with PAH metabolites in wristbands and their corresponding eigenvectors plotted on the x and y-axis. Dashed lines define subjective thresholds (0.25 or −0.25) based on the magnitude of the eigenvectors observed. PAHs with rho values > [0.25] were considered to be the most important in describing either principle component 1 or 2. | |
Principal component scores were plotted, and participants were distinguished by the current smoking status of their caregivers (neither, one, or both caregivers smoke) to visualize potential clustering by smoking as a source of PAH exposure (Fig. 4). There was no discernible pattern of clustering in relation to caregiver smoking status. Several children from households with smokers had low PC1 and/or PC2 scores while some children with non-smoking caregivers had scores indicating higher level of exposure. Thus, the two principal components and/or 3-category smoking variable do not specifically capture exposure patterns from caregiver smoking and other variables may be contributing. Other sources of PAH exposure are likely at play. This may include secondhand smoke exposure outside the home, as well as use of grills or car emissions.
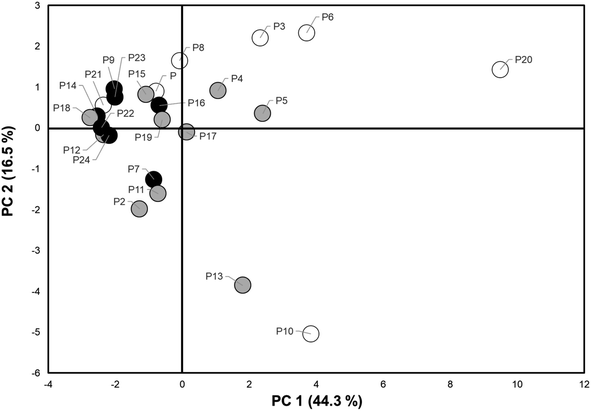 |
| Fig. 4 A two-dimensional score plot of only PC1 and PC2. This plot is further narrated with participant labels and clustering. Clusters were subjectively based on participant score proximity (white = no caregivers smoke, gray = one caregiver smokes, black = both caregivers smoke). | |
4. Discussion
4.1 PAHs in wristbands
We analyzed 27 PAH compounds in wristbands worn by schoolchildren in Montevideo, Uruguay. Eleven of these PAHs are monitored by the US EPA as hazardous and potentially carcinogenic;8 in our sample, they were detected on at least 7 and up to 20 wristbands, with acenaphthene being the least and naphthalene the most common. Naphthalene, acenaphthylene, anthracene, pyrene, fluoranthene, and benz(c)phenanthrene were detected in over half of the wristbands, suggesting a high exposure rate. We analyzed 16 additional PAHs compounds, including alkylated derivatives of the EPA-monitored compounds (e.g., naphthalene with 1-methyl naphthalene, and 2-methyl naphthalene). Of these, 12 were detected in at least 50% of the wristbands. We found 18 strong (rho > 0.70) inter-correlations among PAHs detected in the wristbands, suggesting common exposure sources.
4.2 Detecting PAHs exposure using wristbands
There are limited studies which have detected PAHs in wristbands in various geographic and sociocultural settings, and so direct comparison with past studies is challenging. Furthermore, very few evaluations of PAHs in silicone wristbands worn by children have been reported. One example from 2019 reported on wristbands worn by teenagers ages 15–17 for 5 days in either winter or summer to survey multiple classes of semi volatile organic compounds.24 This study found higher exposure to PAHs in winter months possibly due to increased time indoors with heating systems.24 Studies not done in children18,31,32 which used wristbands to measure PAH exposure among 17 pregnant women aged 21–35 years,18 25 Native American volunteers (age > 18 years),31 and 10 volunteers (18–50 years) from Bloomington Indiana,32 found that in North America phenanthrene is not only one of the most frequently detected PAHs but also had the highest reported concentrations (medians of 89.3–362.8 ng g−1 of wristband). This contrasts with our study, where phenanthrene had the 9th highest detection (ng g−1) of the 27 compounds. The most frequently detected PAH in our study was 1-methyl naphthalene with a median concentration of 3.2 ng g−1.
The five compounds which we observed to have the highest concentrations in wristbands were, in increasing order, 1-methylphenathrene (132.4 ng g−1 wristband; 58% detection), 2-methyl anthracene (135.6 ng g−1; 50%), anthracene (148.5 ng g−1; 75%) 1-methyl pyrene (152.1 ng g−1; 67%), and 2-methyl phenanthrene (217.3 ng g−1; 67% detection). Anthracene is a common byproduct of low combustion reactions but is also intentionally produced for use as a fluorophore, and in polymer synthesis.33 Sources of 1-methylphenathrene, 2-methyl anthracene, 1-methyl pyrene and other alkylated PAHs include but are not limited to, combustion of crude oil and fuels34,35 as well as cigarette smoke.36 Four of these compounds are alkylated derivatives and are not classified by the EPA as hazardous. Alkylated PAHs have higher abundance than their non-alkylated forms in many environmental media, including crude oil,35 ambient air,37,38 cigarette smoke36 and plant and animal tissue samples.39 A recent study in fish found that 3 ring alkylated PAHs were more persistent, bioaccumulated, and toxic than their parent compounds.40 Thus far, only two other studies measured select alkylated PAHs in wristbands.18,31 Among the Swinomish Indian Tribal Community in Washington state, alkylated PAHs were detected as frequently as the parent compounds but at lower concentrations.31 Alternatively, a study in pregnant women living in Texas found that alkylated PAHs such as 2-methyl naphthalene, 1-methyl naphthalene, and 1-methyl phenanthrene were detected at high concentrations, all with medians above 100 ng g−1; however, they were still lower than all other PAHs. The detected concentrations of both alkylated and non-alkylated PAHs were lower in Montevideo first graders compared to the above studies. Nevertheless, direct comparison of PAH concentration between studies is challenging due to differences in quantitation, recoveries, study design and analysis bias such as instruments or analytical techniques used.
4.3 Correlation among PAH compounds in wristbands
Eleven PAHs including 1-methyl naphthalene, acenaphthylene, 1,6-dimethyl naphthalene, 1,6,7-trimethyl naphthalene, dibenzothiophene, 1-methylphenanthrene, pyrene, fluoranthene, 2-phenyl naphthalene, 2-methyl naphthalene, and anthracene (Table S4†) correlated strongly (rho > 0.7) with two or more compounds from the same list of PAHs, indicating a common exposure source. These compounds share a conserved 2 ringed structure but differ in terms of the addition and position of 1 or more alkyl moieties. Of these correlations, six overlapped with compounds described by PC1.
Our analysis identified correlations among PAHs with low molecular weight (2–4 rings). According to prior evidence, the sources of PAHs include diet (which cannot be identified via wristbands) smoking habits, and fuel used in cars and grills.41 PAHs are also a common byproduct of cigarette smoke with microgram amounts of PAHs per cigarette being released.42 The PAHs present in second-hand smoke are almost exclusively (∼95%) comprised of 2–4 ringed compounds, with the other 5% being >5 rings.42 Studies have pointed to cigarette smoke as a source of PAHs (primarily lower molecular weight PAHs). A 2015 study of American-made cigarettes showed a strong correlation (rho > 0.61) among low molecular PAHs (2 rings), suggesting multiple PAHs could come from the same cigarettes.43 The compounds highlighted in our statistical analysis are all 2–3 ring PAHs and could be from secondhand smoke but more studies are needed to confirm this.42
4.4 Correlation among PAH detections between participants with smoking and non-smoking caregivers
Methods for determining sources of PAHs include assessing ratios of low (2–4 rings) and high (5–6 rings) molecular weight PAHs.44 When the ratio of the sum of low molecular weight PAHs to the sum of high molecular weight PAHs (in terms of mass) is greater than 1, the source of those PAHs is more likely a low combustion reaction (wood burning, cigarette smoke, etc.).44 In Uruguayan schoolchildren, the ratio of low molecular weight PAHs to high molecular weight PAHs (based on the sum of median detections for each group) was greater than 1, suggesting a low combustion source. On the other hand, a PCA analysis did not reveal specific clustering of children whose caregivers reported currently smoking. The potential explanations for these discrepant findings include: (1) other sources may be at play; (2) the sample size is too low for definitive conclusions; (3) the questions on smoking were limited to yes/no responses on current smoking status of the caregivers, without querying about other smokers in the household and whether smoking occurs inside of the home or in other places where the children spend considerable time. As such, our analyses represent only the first exploration of these relationships and serve to stimulate future research. Additional studies could, for example, include a corollary nicotine analysis in the wristbands or analyze cotinine levels in urine to make more definitive connections to smoking as an exposure source. Future studies should also investigate other potential sources of PAH exposure among children in different sociocultural settings.
Smoking among Uruguayan adults has declined between 2009 and 2017, particularly among males and the 15–24 year age group.45 Nevertheless, according to the 2017 Global Adult Tobacco Survey, ∼26% of males and 18% of females still smoked around the time of the Scola-Exposome study. Furthermore, the prevalence of secondhand smoke in Uruguayan homes was ∼20% in 2017.45 On the other hand, Scola-Exposome was conducted between the months of July and December 2018, encompassing winter and spring, when heating is used in Uruguayan homes. According to a separate survey administered to ∼250 families participating in the broader cohort, ∼20% of households used gas while 25% used wood as a source of heating.
It is also important to point out that there are no studies which have determined the exact efficacy of silicone wristbands to passively sample PAHs sourced from secondhand smoke. However, previous studies among children used silicone wristbands to evaluate total nicotine exposure in homes of smokers and non-smokers. These studies showed that wristbands in the homes of smokers collected higher levels of nicotine than in homes of non-smokers,46,47 suggesting that wristbands have utility in detecting secondhand smoke exposure.
4.5 Study strengths & limitations
An important strength of our wristband analysis is that we prepared a method for the detection of 27 PAHs with recoveries of 48.3–92.3% and a GC-MS/MS method with LODs between 0.04 and 13.04 ng g−1. Furthermore, the study was conducted in a well-characterized study sample. With available information on smoking, we attempted to relate caregiver smoking status and PAH compound levels in wristbands. With respect to limitations, the chemical structure of PAHs is not easily amenable to fragmentation in GC-MS/MS such that signal-to-noise ratios are not significantly increased even in selected reaction monitoring mode.48 Some studies have employed MS/MS approaches to PAH detection with some success but also report higher limits of detection49 compared to our method. On the other hand, our method cannot differentiate between chrysene and triphenylene; they are isobaric and do not separate on the column. All our statistical analyses grouped chrysene and triphenylene. We were unable to target the other five PAHs that the EPA monitors due to solubility challenges. Additionally, this study had a relatively small sample size of 24 participants. It is also important to highlight that a large source of PAHs may come from ingestion of contaminated food which cannot be reflected in the wristbands.5 As discussed above, our questionnaire-based classification of caregiver smoking may misclassify children's secondhand smoke exposure by not accounting for all smokers in the household or all locations where children may be exposed. Lastly, we were unable to corroborate exposure patterns with urinary markers of PAH metabolites, but plan to do so in future studies.
4.6 Public health/exposure implications & future research directions
This study provides evidence that schoolchildren in Montevideo are exposed to PAHs, with numerous compounds detected in wristbands, although the observed levels appear lower than in other studies. It is challenging to define the health impact of exposures at the levels detected in the wristbands because very few studies have linked wristband detections with health outcomes or with internal biomarkers of exposure.50 Additional research with wristbands is needed and we recently proposed several avenues, including sampling in family-based studies for a wider scope of exposure in homes.25 With initial results suggesting higher exposures in children to low molecular weight PAHs, surveys could help determine potential sources, including the use of grills or smoking indoors. Additional experiments that consider nicotine and its biomarkers in wristbands or urine46,47 could help resolve if low molecular weight PAHs result from secondhand smoke exposure.
5. Conclusion
Noninvasive monitoring of PAHs is essential among children given their vulnerability to these ubiquitous compounds. Among school-age children living in Montevideo, Uruguay, PAHs with highest frequency of detection or concentrations were a mix of alkylated and non-alkylated compounds with low molecular weight. Naphthalene and its alkylated derivatives had strong correlations with other low molecular weight PAHs in wristbands, suggesting common exposure sources, possibly secondhand smoke, or fuel combustion. Wristbands provide a comprehensive view of children's exposures, and complement biomonitoring in urine.
Conflicts of interest
The authors declare that they have no known competing financial interests or personal relationships that could have appeared to influence the work reported in this paper.
Acknowledgements
This study was funded by pilot grants from the RENEW Institute, University at Buffalo and Community for Global Health Equity, University at Buffalo. Additional funding was received from the National Institute of Environmental Health Sciences (R01ES023423, PI: Kordas).
References
-
Agency for Toxic Substances and Disease Registry, Polycyclic Aromatic Hydrocarbons (PAHs), Atlanta Georgia, 1996 Search PubMed.
-
A. N. Gachanja and P. K. Maritim, Polycyclic Aromatic Hydrocarbons | Determination, in Encyclopedia of Analytical Science, ed. Worsfold, P., Poole, C., Townshend, A. and Miró, M., Academic Press, Oxford, 3rd edn, 2019, pp. 328–340 Search PubMed.
- H. I. Abdel-Shafy and M. S. M. Mansour, A review on polycyclic aromatic hydrocarbons: source, environmental impact, effect on human health and remediation, Egypt. J. Pet., 2016, 25(1), 107–123 CrossRef.
- J.-G. Lee, S.-Y. Kim, J.-S. Moon, S.-H. Kim, D.-H. Kang and H.-J. Yoon, Effects of grilling procedures on levels of polycyclic aromatic hydrocarbons in grilled meats, Food Chem., 2016, 199, 632–638 CrossRef CAS PubMed.
- G. R. Sampaio, G. M. Guizellini, S. A. da Silva, A. P. de Almeida, A. C. C. Pinaffi-Langley, M. M. Rogero, A. C. de Camargo and E. Torres, Polycyclic Aromatic Hydrocarbons in Foods: Biological Effects, Legislation, Occurrence, Analytical Methods, and Strategies to Reduce Their Formation, Int. J. Mol. Sci., 2021, 22(11), 6010 CrossRef CAS PubMed.
- M. J. Nieva-Cano, S. Rubio-Barroso and M. J. Santos-Delgado, Determination of PAH in food samples by HPLC with fluorimetric detection following sonication extraction without sample clean-up, Analyst, 2001, 126(8), 1326–1331 RSC.
- H. Alomirah, S. Al-Zenki, S. Al-Hooti, S. Zaghloul, W. Sawaya, N. Ahmed and K. Kannan, Concentrations and dietary exposure to polycyclic aromatic hydrocarbons (PAHs) from grilled and smoked foods, Food Control, 2011, 22(12), 2028–2035 CrossRef CAS.
- E. Hussar, S. Richards, Z.-Q. Lin, R. P. Dixon and K. A. Johnson, Human Health Risk Assessment of 16 Priority Polycyclic Aromatic Hydrocarbons in Soils of Chattanooga, Tennessee, USA, Water, Air, Soil Pollut., 2012, 223(9), 5535–5548 CrossRef CAS PubMed.
-
Agency for Toxic Substances and Disease Registry, Polycyclic Aromatic Hydrocarbons, What Health Effects Are Associated With PAH Exposure?, 2013 Search PubMed.
- J. Jurewicz, K. Polańska and W. Hanke, Chemical exposure early in life and the neurodevelopment of children—an overview of current epidemiological evidence, Ann. Agric. Environ. Med., 2013, 20(3), 465–486 Search PubMed.
- M. Hauptman and A. D. Woolf, Childhood Ingestions of Environmental Toxins: What Are the Risks?, Pediatr. Ann., 2017, 46(12), e466–e471 Search PubMed.
- J. Moya and L. Phillips, A review of soil and dust ingestion studies for children, J. Exposure Sci. Environ. Epidemiol., 2014, 24(6), 545–554 CrossRef PubMed.
- R. Stading, G. Gastelum, C. Chu, W. Jiang and B. Moorthy, Molecular mechanisms of pulmonary carcinogenesis by polycyclic aromatic hydrocarbons (PAHs): implications for human lung cancer, Semin. Cancer Biol., 2021, 76, 3–16 CrossRef CAS PubMed.
- C. Edwards Susan, W. Jedrychowski, M. Butscher, D. Camann, A. Kieltyka, E. Mroz, E. Flak, Z. Li, S. Wang, V. Rauh and F. Pereira, Prenatal Exposure to Airborne Polycyclic Aromatic Hydrocarbons and Children's Intelligence at 5 Years of Age in a Prospective Cohort Study in Poland, Environ. Health Perspect., 2010, 118(9), 1326–1331 CrossRef PubMed.
- F. Pereira, D. Tang, S. Wang, J. Vishnevetsky, B. Zhang, D. Diaz, D. Camann and V. Rauh, Prenatal Polycyclic Aromatic Hydrocarbon (PAH) Exposure and Child Behavior at Age 6–7 Years, Environ. Health Perspect., 2012, 120(6), 921–926 CrossRef PubMed.
- N. A. Sopian, J. Jalaludin, S. Abu Bakar, T. R. Hamedon and M. T. Latif, Exposure to Particulate PAHs on Potential Genotoxicity and Cancer Risk among School Children Living Near the Petrochemical Industry, Int. J. Environ. Res. Public Health, 2021, 2575 CrossRef CAS PubMed.
- S. G. O'Connell, L. D. Kincl and K. A. Anderson, Silicone Wristbands as Personal Passive Samplers, Environ. Sci. Technol., 2014, 48(6), 3327–3335 CrossRef PubMed.
- I. Mendoza-Sanchez, I. Uwak, L. Myatt, A. Van Cleve, J. C. Pulczinski, K. A. Rychlik, S. Sweet, T. Ramani, J. Zietsman, M. L. Zamora, K. Koehler, G. Carrillo and N. M. Johnson, Maternal exposure to polycyclic aromatic hydrocarbons in South Texas, evaluation of silicone wristbands as personal passive samplers, J. Exposure Sci. Environ. Epidemiol., 2022, 32(2), 280–288 CrossRef CAS PubMed.
- J. P. Koelmel, E. Z. Lin, K. DeLay, A. J. Williams, Y. Zhou, R. Bornman, M. Obida, J. Chevrier and K. J. Godri Pollitt, Assessing the External Exposome Using Wearable Passive Samplers and High-Resolution Mass Spectrometry among South African Children Participating in the VHEMBE Study, Environ. Sci. Technol., 2022, 56(4), 2191–2203 CrossRef CAS PubMed.
- E. Z. Lin, S. Esenther, M. Mascelloni, F. Irfan and K. J. Godri Pollitt, The Fresh Air Wristband: A Wearable Air Pollutant Sampler, Environ. Sci. Technol. Lett., 2020, 7(5), 308–314 CrossRef CAS.
- D. Rohlman, H. M. Dixon, L. Kincl, A. Larkin, R. Evoy, M. Barton, A. Phillips, E. Peterson, C. Scaffidi, J. B. Herbstman, K. M. Waters and K. A. Anderson, Development of an environmental health tool linking chemical exposures, physical location and lung function, BMC Public Health, 2019, 19(1), 854 CrossRef PubMed.
- H. M. Dixon, R. P. Scott, D. Holmes, L. Calero, L. D. Kincl, K. M. Waters, D. E. Camann, A. M. Calafat, J. B. Herbstman and K. A. Anderson, Silicone wristbands compared with traditional polycyclic aromatic hydrocarbon exposure assessment methods, Anal. Bioanal. Chem., 2018, 410(13), 3059–3071 CrossRef CAS PubMed.
- H. M. Dixon, G. Armstrong, M. Barton, A. J. Bergmann, M. Bondy, M. L. Halbleib, W. Hamilton, E. Haynes, J. Herbstman, P. Hoffman, P. Jepson, M. L. Kile, L. Kincl, P. J. Laurienti, P. North, L. B. Paulik, J. Petrosino, G. L. Points 3rd, C. M. Poutasse, D. Rohlman, R. P. Scott, B. Smith, L. G. Tidwell, C. Walker, K. M. Waters and K. A. Anderson, Discovery of common chemical exposures across three continents using silicone wristbands, R. Soc. Open Sci., 2019, 6(2), 181836 CrossRef CAS PubMed.
- C. A. Manzano, N. G. Dodder, E. Hoh and R. Morales, Patterns of Personal Exposure to Urban Pollutants Using Personal Passive Samplers and GC × GC/ToF–MS, Environ. Sci. Technol., 2019, 53(2), 614–624 CrossRef CAS PubMed.
- L. S. Running, K. Kordas and D. S. Aga, Use of wristbands to measure exposure to environmental pollutants in children: Recent advances and future directions, Curr. Opin. Environ. Sci. Health, 2023, 32, 100450 CrossRef.
- L. Hamzai, N. Lopez Galvez, E. Hoh, N. G. Dodder, G. E. Matt and P. J. Quintana, A systematic review of the use of silicone wristbands for environmental exposure assessment, with a focus on polycyclic aromatic hydrocarbons (PAHs), J. Exposure Sci. Environ. Epidemiol., 2022, 32(2), 244–258 CrossRef CAS PubMed.
- S. C. Travis, D. S. Aga, E. I. Queirolo, J. R. Olson, M. Daleiro and K. Kordas, Catching flame retardants and pesticides in silicone wristbands: Evidence of exposure to current and legacy pollutants in Uruguayan children, Sci. Total Environ., 2020, 740, 140136 CrossRef CAS PubMed.
- O. Y. Addo, E. X. Yu, A. M. Williams, M. F. Young, A. J. Sharma, Z. Mei, N. J. Kassebaum, M. E. D. Jefferds and P. S. Suchdev, Evaluation of Hemoglobin Cutoff Levels to Define Anemia Among Healthy Individuals, JAMA Netw. Open, 2021, 4(8), e2119123 CrossRef PubMed.
-
Centers for Disease Control and Prevention, Blood Level Reference Value, https://www.cdc.gov/nceh/lead/data/blood-lead-reference-value.htm, accessed 1-10-2023 Search PubMed.
- S. Frndak, Y. Gallo, E. I. Queirolo, G. Barg, N. Mañay and K. Kordas, A mixed methods study examining neighborhood disadvantage and childhood behavior problems in Montevideo, Uruguay, Int. J. Hyg. Environ. Health, 2021, 235, 113753 CrossRef PubMed.
- D. Rohlman, J. Donatuto, M. Heidt, M. Barton, L. Campbell, K. A. Anderson and M. L. Kile, A Case Study Describing a Community-Engaged Approach for Evaluating Polycyclic Aromatic Hydrocarbon Exposure in a Native American Community, Int. J. Environ. Res. Public Health, 2019, 16(3), 327 CrossRef CAS PubMed.
- K. A. Romanak, S. Wang, W. A. Stubbings, M. Hendryx, M. Venier and A. Salamova, Analysis of brominated and chlorinated flame retardants, organophosphate esters, and polycyclic aromatic hydrocarbons in silicone wristbands used as personal passive samplers, J. Chromatogr. A, 2019, 1588, 41–47 CrossRef CAS PubMed.
- J. Van Damme and F. Du Prez, Anthracene-containing polymers toward high-end applications, Prog. Polym. Sci., 2018, 82, 92–119 CrossRef CAS.
- S. Y. Lee and J. H. Kwon, Enhancement of Toxic Efficacy of Alkylated Polycyclic Aromatic Hydrocarbons Transformed by Sphingobium quisquiliarum, Int. J. Environ. Res. Public Health, 2020, 17(17), 6416 CrossRef CAS PubMed.
- S. B. Hawthorne, D. J. Miller and J. P. Kreitinger, Measurement of total polycyclic aromatic hydrocarbon concentrations in sediments and toxic units used for estimating risk to benthic invertebrates at manufactured gas plant sites, Environ. Toxicol. Chem., 2006, 25(1), 287–296 CrossRef CAS PubMed.
- B. Gao, X. Du, X. Wang, J. Tang, X. Ding, Y. Zhang, X. Bi and G. Zhang, Parent, Alkylated, and Sulfur/Oxygen-Containing Polycyclic Aromatic Hydrocarbons in Mainstream Smoke from 13 Brands of Chinese Cigarettes, Environ. Sci. Technol., 2015, 49(15), 9012–9019 CrossRef CAS PubMed.
- M. Moradi, H. Hung, J. Li, R. Park, C. Shin, N. Alexandrou, M. A. Iqbal, M. Takhar, A. Chan and J. R. Brook, Assessment of Alkylated and Unsubstituted Polycyclic Aromatic Hydrocarbons in Air in Urban and Semi-Urban Areas in Toronto, Canada, Environ. Sci. Technol., 2022, 56(5), 2959–2967 CrossRef CAS PubMed.
- J.-J. Lian, C.-L. Li, Y. Ren, T.-T. Cheng and J.-M. Chen, Determination of Alkyl Polycyclic Aromatic Hydrocarbons in Dustfall by Supercritical Fluid Extraction Followed by Gas Chromatography/Mass Spectrum, Bull. Environ. Contam. Toxicol., 2009, 82(2), 189–193 CrossRef CAS PubMed.
- N. Golzadeh, B. D. Barst, J. M. Baker, J. C. Auger and M. A. McKinney, Alkylated polycyclic aromatic hydrocarbons are the largest contributor to polycyclic aromatic compound concentrations in traditional foods of the Bigstone Cree Nation in Alberta, Canada, Environ. Pollut., 2021, 275, 116625 CrossRef CAS PubMed.
- P. N. H. Wassenaar and E. M. J. Verbruggen, Persistence, bioaccumulation and toxicity-assessment of petroleum UVCBs: A case study on alkylated three-ring PAHs, Chemosphere, 2021, 276, 130113 CrossRef CAS PubMed.
- L. Wang, A. Liu, Y. Zhao, X. Mu, T. Huang, H. Gao and J. Ma, The levels of polycyclic aromatic hydrocarbons (PAHs) in human milk and exposure risk to breastfed infants in petrochemical industrialized Lanzhou Valley, Northwest China, Environ. Sci. Pollut. Res., 2018, 25(17), 16754–16766 CrossRef CAS PubMed.
- R. S. Osgood, B. L. Upham, P. R. Bushel, K. Velmurugan, K.-N. Xiong and A. K. Bauer, Secondhand Smoke-Prevalent Polycyclic Aromatic Hydrocarbon Binary Mixture-Induced Specific Mitogenic and Pro-inflammatory Cell Signaling Events in Lung Epithelial Cells, Toxicol. Sci., 2017, 157(1), 156–171 CrossRef CAS PubMed.
- A. T. Vu, K. M. Taylor, M. R. Holman, Y. S. Ding, B. Hearn and C. H. Watson, Polycyclic Aromatic Hydrocarbons in the Mainstream Smoke
of Popular U.S. Cigarettes, Chem. Res. Toxicol., 2015, 28(8), 1616–1626 Search PubMed.
- M. Tobiszewski and J. Namieśnik, PAH diagnostic ratios for the identification of pollution emission sources, Environ. Pollut., 2012, 162, 110–119 CrossRef CAS PubMed.
-
Global Adult Tobacco Survey, National-Statistics-Institute, 2018 Search PubMed.
- P. J. E. Quintana, N. Lopez-Galvez, N. G. Dodder, E. Hoh, G. E. Matt, J. M. Zakarian, M. Vyas, L. Chu, B. Akins, S. Padilla, K. A. Anderson and M. F. Hovell, Nicotine, Cotinine, and Tobacco-Specific Nitrosamines Measured in Children's Silicone Wristbands in Relation to Secondhand Smoke and E-cigarette Vapor Exposure, Nicotine Tob. Res., 2021, 23(3), 592–599 CrossRef CAS PubMed.
- P. J. E. Quintana, E. Hoh, N. G. Dodder, G. E. Matt, J. M. Zakarian, K. A. Anderson, B. Akins, L. Chu and M. F. Hovell, Nicotine levels in silicone wristband samplers worn by children exposed to secondhand smoke and electronic cigarette vapor are highly correlated with child's urinary cotinine, J. Exposure Sci. Environ. Epidemiol., 2019, 29(6), 733–741 CrossRef PubMed.
- D. Shang, M. Kim and M. Haberl, Rapid and sensitive method for the determination of polycyclic aromatic hydrocarbons in soils using pseudo multiple reaction monitoring gas chromatography/tandem mass spectrometry, J. Chromatogr. A, 2014, 1334, 118–125 CrossRef CAS PubMed.
- S.-W. Wang, K.-H. Hsu, S.-C. Huang, S.-H. Tseng, D.-Y. Wang and H.-F. Cheng, Determination of polycyclic aromatic hydrocarbons (PAHs) in cosmetic products by gas chromatography-tandem mass spectrometry, J. Food Drug Anal., 2019, 27(3), 815–824 CrossRef CAS PubMed.
-
WHO, Human Health Effects of Polycyclic Aromatic Hydrocarbons as Ambient Air Pollutants: Report of the Working Group on Polycyclic Aromatic Hydrocarbons of the Joint Task Force on the Health Aspects of Air Pollution, Regional Office for Europe, Copenhagen, 2021 Search PubMed.
|
This journal is © The Royal Society of Chemistry 2024 |
Click here to see how this site uses Cookies. View our privacy policy here.