Associations of coke oven emission exposure with pulmonary function, blood pressure, blood cell parameters, and biochemical indices in coking workers: a cross-sectional pilot study†
Received
28th May 2024
, Accepted 4th November 2024
First published on 25th November 2024
Abstract
Background and objective: Coke oven emissions (COEs) are formed in the process of coking production, mainly composed of polycyclic aromatic hydrocarbons (PAHs) and benzene; however, the health impacts of COE exposure in coking workers are not fully clear so far. We aimed to explore the associations of occupational COE exposure with pulmonary function, blood pressure, blood cell parameters, and blood biochemical indices, and to bolster health surveillance and disease prevention and control in coking workers. Methods: We investigated 566 coking workers at a large state-owned enterprise coking plant in Taiyuan, Shanxi, China, measured the concentrations of plasma 16 PAHs and urinary phenol, assessed the health outcomes including pulmonary function, blood pressure, the levels of peripheral hematologic parameters and biochemical indices, and examined the associations of PAH and phenol concentrations with the health outcomes using multiple linear regressions, least absolute shrinkage and selection operator regression (LASSO), and Bayesian kernel machine regression (BKMR). Results: After adjustment for confounders, plasma ∑15PAH concentration was significantly associated with increases in hemoglobin (HGB) and triglyceride (TG) levels in coking workers, and urinary phenol concentration was significantly associated with increases in the diastolic blood pressure (DBP) level, and decreases in platelet (PLT) count. When phenol concentration and PAH concentration were simultaneously included in the multiple linear regression model, both of them were associated with the level of HGB. LASSO and BKMR indicated that the PAHs with four rings and above contributed to the HGB level. Conclusion: PAH exposure could damage hematological parameters and blood lipids, and benzene exposure could increase blood pressure and decrease PLT count.
Environmental significance
China is the largest coke producer and exporter worldwide. A large amount of coke oven emissions (COEs) were emitted from the coke production process, including polycyclic aromatic hydrocarbons (PAHs), benzene, sulfur dioxide, carbon dioxide, and so on. Despite the concentrations of COEs and other pollutants in coking plants being strictly controlled within the allowable range of Chinese national standards, the potential health hazard of long-term exposure at low doses should still be considered. The findings indicated the potential health impacts of occupational COE exposure on pulmonary function, hematologic parameters, and blood lipids in coking workers, which suggested we better understand and attach more importance to potential multiple health impacts of occupational COE exposure among coking workers in routine health monitoring. In addition, the findings may hint to researchers to further tighten the national standards related to coking workplaces, to protect the health of the occupational population as much as possible.
|
1 Introduction
China is the largest coke producer and exporter all over the world. A large amount of coke oven emissions (COEs) are emitted from the coke production process, such as polycyclic aromatic hydrocarbons (PAHs), benzene, sulfur dioxide, carbon dioxide, and so on.1 The COEs in the workplace can easily enter the human body via inhalation and skin absorption, causing cancer and other serious health risks. COEs were identified as a confirmed human carcinogen by the International Agency for Research on Cancer (IARC) over four decades ago.2,3 Despite the concentrations of COEs and other pollutants in coking plants being strictly controlled within the allowable range of Chinese national standards, the potential health hazard of long-term exposure at low doses should still be considered.
PAHs are a class of more than 200 compounds containing two or more benzene rings and are mainly from the pyrolysis and incomplete combustion of carboniferous organic matter in coking production.4 It is reported that PAHs contributed to the morbidity of asthma,5 obstructive lung diseases,6 lung cancer,7 bladder cancer,8 breast cancer,9 reproductive function damage,10,11etc. Occupational PAH exposure can increase the mortality risk of ischemic heart disease and cardiovascular disease.12,13 More recently, PAH exposure has been reported to be associated with changes in liver and kidney function.14,15 Benzene (C6H6) widely exists in gasoline, engine exhausts, wood, and tobacco smoke, and is produced in the industrial processes of coal charging, hot coke pushing, and tar distillation in coking plants.1,16 Urinary phenol concentration is used to reflect the benzene exposure level in the workplace.17 Higher levels of benzene exposure were closely associated with high morbidity of blood system diseases such as myelodysplastic syndrome in petroleum workers,18 acute leukemia in rubber workers,19 leukemia in chemical dye workers,20 and aplastic anemia in shoemakers.21 Lower levels of benzene exposure in the workplace were associated with various hematological parameters (the numbers of white blood cells, red blood cells, and lymphocytes) in workers from gasoline stations,22 shoe factories,23 and petroleum refineries.24–26
However, the multiple health impacts of occupational PAH and benzene exposure in coking workers are not fully clear so far. Considering the critical primary parts of the respiratory and vascular system for the air pollutants entering the body, and the early sensitive warning function of hematologic and biochemical indices for the disease or health status in human beings, we aimed to examine the associations of PAH and benzene exposure with pulmonary function, blood pressure, hematologic parameters, and biochemical indices in coking workers in this study. The findings are expected to reveal the potential health impact of occupational COE exposure on coking workers, promote health surveillance and disease prevention, and provide a clue or basis for hypothesis development, specific design, and the protocol in a prospective cohort study.
2 Subjects and methods
2.1 Subjects
We investigated 566 coking workers at a large state-owned enterprise coking plant in Taiyuan, Shanxi, P.R. China, from April to June 2019. All eligible staff were >20 years of age, employed in the coking plant for more than 1 year, and provided written informed consent before the investigation. The exclusion criteria are (1) who had previously or currently been occupationally exposed to formaldehyde, organic solvents, or heavy metals, (2) who had a personal or family history of tumor or blood system diseases, (3) who lived in a room that had not been renovated and redecorated in the last 2 years, and (4) the residential community is 2.5 km away from the coking plant and not downwind of it. The protocol was conducted by the Declaration of Helsinki and was approved by the Ethics and Human Subjects Committees of Shanxi Medical University (2019LL111).
2.2 Questionnaire investigation
All subjects were interviewed face-to-face using a specialized questionnaire to collect their age (years), gender, education, tobacco smoking and alcohol drinking habits, work type, employment duration (duration of employment at the coking plant and year), personal vocational history, personal illness and medication history, family history of diseases, and usage of occupational protection measures. Tobacco smoking was divided into current tobacco smoking and non-smoking. Current tobacco smoking was defined as smoking cigarettes or other smoked tobacco products at least once in the last 30 days. Otherwise, it is considered non-smoking. Alcohol drinking was defined as drinking more than 40 g of alcohol per day for men and 20 g for women based on alcohol consumption according to the World Health Organization (WHO).27 Otherwise, it is considered non-drinking.
2.3 Exposure assessment
2.3.1 Airborne COEs, benzene, benzene homologs, and monitoring.
A total of 51 air samples were routinely collected using active carbon tubes or glass fiber filters for 15 min at the height of the breathing zone from 7 workshops in the coke plant, including 1# coking, 2# coking, cold drum electric capture, crude benzene, ammonium sulfate, a water pump, and a desulfurization workshop, according to the method recommended by the air sampling specifications for hazardous substance monitoring in the workplace (GBZ159-2004). The COE concentrations were determined using the gravimetric method (as benzene soluble matter) according to the health standard for COEs in air of the workplace (GB17054-1997) and presented as the time-weighted average (TWA, mg m−3) and short-term exposure limit (STEL, mg m−3). Airborne benzene and benzene homolog concentrations were determined using a gas chromatograph equipped with a flame ionization detector (FID) according to the determination of air-aromatic hydrocarbons in workplace air (GBZ/T160.42-2007). They were expressed as the TWA (mg m−3) and STEL (mg m−3).
2.3.2 Blood sample collection and plasma PAH determination.
Fasting elbow venous blood samples were drawn in the morning from each participant into two vacuum tubes. One tube sample was non-anticoagulated and centrifuged to obtain serum for the biochemical indicator determination. The other tube sample was anticoagulated, the routine hematological parameters were detected, and then centrifuged to obtain plasma for PAH determination.
16 plasma PAHs were separated using n-hexane extraction, concentrated to near dryness using a nitrogen blower, and determined using a gas chromatography-mass spectrometry analysis (GC-MS) based on the methods of Pleil J. D. et al.,28 including naphthalene (Nap), acenaphthene (Ace), acenaphthylene (Acy), fluorene (Flu), phenanthrene (Phe), fluoranthene (Flt), anthracene (Ant), pyrene (Pyr), benzo[a]anthracene (BaA), chrysene (Chr), benzo[k]fluoranthene (BkF), benzo[b]fluoranthene (BbF), benzo[a]pyrene (BaP), dibenzo[a,h]anthracene (DhA), indeno[1,2,3-cd]pyrene (ICP), and benzo[g,h,i]perylene (BgP). Each PAH homolog was identified and quantified based on the retention time and peak area. PAHs multi compound standard – 16 compound mix (Reagecon, Ireland) and internal standards were used for the quality control. Concentrations below the LOD were substituted with the LOD divided by the square root of 2.29
2.3.3 Urine sampling and phenol determination.
Midstream urine samples after the night shift were collected in the fasting morning, the specific gravity was determined, and frozen in small packages at −80 °C until use. The urine sample was digested with perchloric acid, and extracted with anhydrous diethyl ether, and the phenol concentration was determined using a gas chromatograph equipped with a flame ionization detector (FID) according to the Chinese national standard methods (WS/T49-1996, WS/T50-1996, China). The measurement conditions were shown (ESI, Table S1†). The phenol standard (Sigma-Aldrich, USA) was dissolved in anhydrous diethyl ether (0–180 μg mL−1) as a series of working solutions.
Phenol was identified and quantified based on the retention time and the peak area, respectively. The phenol concentration was calibrated with urine-specific gravity and presented as μg mL−1. The lowest limit of detection (LOD) of phenol in the urine sample was 0.045 μg mL−1. Concentrations below the LOD were substituted with the LOD value divided by the square root of 2.29 Sampling blank, experimental blank, and matrix quality control samples were determined daily to control the measurement. During the whole test period, we detected phenol standard solution (15.0 μg mL−1) twice per day and judged the measurement reliability based on the average quality control chart.
2.4 Pulmonary function and blood pressure assessment
The forced vital capacity (FVC) and forced expiratory volume of each participant was measured in 1 second (FEV1) using a spirometer (HI-801, CHEST, Japan) according to the guidelines recommended by the joint statements on lung function testing issued by the American Thoracic Society and the European Respiratory Society (ATS-ERS),30 and the systolic blood pressure (SBP) and diastolic blood pressure (DBP) were determined in a sitting position using a standard sphygmomanometer (HEM-7133, OMRON, Japan) after a 5 min rest period based on the guidelines recommended by the World Health Organization/International Society of Hypertension (WHO/ISH).31 The body mass index (BMI) value (kg m−2) was obtained by dividing the body weight (kg) by the square of height (m2).
2.5 Blood cell parameters, and glucose, and lipid detection
As previously mentioned, the routine hematological parameters of the anticoagulated blood samples were detected using a fully automated five-class blood cell analyzer (Sysmex XT1800i, SYSMEX, Japan), including white blood cell (WBC) counts, red blood cell (RBC) counts, and hemoglobin (HGB) and platelet (PLT) levels. Serum from the non-anticoagulated blood was used to determine the concentrations of glucose (GLU), total cholesterol (TC), triglyceride (TG), high-density lipoprotein (HDL), and low-density lipoprotein (LDL) using an automatic blood biochemistry analyzer (Cobas 8000, Roche, Switzerland).
2.6 Statistical analysis
We presented continuous variables as the mean ± standard deviation (SD) or median (interquartile range, IQR), and expressed categorical variables as numbers and percentages (%). Plasma PAH concentration and urinary phenol concentration were normalized by using logarithm transformation due to their skew distributions and numerical ranges. We used multiple linear regression to analyze the association of COE exposure (indicated by PAH and phenol concentrations) with health outcomes (pulmonary function, blood pressure, and blood glucose and lipids).32 The entry and removal criteria were 0.10 and 0.15 for variable selection, respectively. Covariates for adjustment were identified based on their significant relationships with the exposure concentrations and outcome scores using analysis of variance (ANOVA) or the t-test for continuous variables, and the chi-square (χ2) test for categorical variables. Based on the tertile cut-off values, we classified age into 3 categorical variables including <43 years (n = 206), 43–49 years (n = 195), and ≥49 years (n = 165), and classified employment duration into <24 years (n = 225), 24–30 years (n = 158), and ≥30 years (n = 183). Based on BMI values, we stratified subjects as normal (BMI < kg m−2, n = 211), overweight (BMI ≥ 24 kg m−2 and <28 kg m−2, n = 259), and obesity (BMI ≥ 28 kg m−2, n = 96) according to the guidelines recommended by Working Group of Obesity, China (WGOC), International Life Science Association. SPSS 22.0 software (SPSS Inc., Chicago, IL) was used for data processing and statistical analysis. SAS 9.4 (SAS Institute Inc., Cary, NC) was adopted for restricted cubic spline (RCS) regression, while R studio software (version 4.0.2; R Development Core Team) was used for least absolute shrinkage and selection operator (LASSO) regression and Bayesian kernel machine regression (BKMR), with a glmnet package for LASSO analysis, and packages of bkmr, readxl, corrplot, and gglpot2 for BKMR analysis. We set the statistical significance at a two-sided P <0.05 and reported the regression coefficients (β) and 95% confidence intervals (CIs) from the multiple regression analyses.
3 Results
3.1 Questionnaire information and workplace monitoring
The subjects were predominantly males (n = 529, 93%), with an average age of (44.5 ± 7.9) years and employment duration of (25.2 ± 8.9) years. The majority of them had college degrees or above (n = 228, 40.28%), were tobacco smokers (n
=
323, 57.07%), and were non-alcohol consumers (n
=
345, 60.95%). The TWA and STEL concentrations of COEs, benzene, methylbenzene, and xylene from 7 workshops in the coke plant were listed (Table 1). The average and individual TWA and STEL concentrations of each pollutant did not exceed the occupational exposure limit (OEL) stipulated by the Chinese national standard (GBZ 2.1-2007).
Table 1 Concentrations of airborne benzene, benzene homologs, and COEs in the coking plant (mg m−3)a
Workshop |
n
|
COEs |
Benzene |
Methylbenzene |
Xylene |
TWA |
STEL |
Excursion limits |
TWA |
STEL |
TWA |
STEL |
TWA |
STEL |
The OEL refers to the permissible concentration–time weighted average (PC-TWA), permissible concentration-short-term exposure limit (PC-STEL), and maximum excursion limits set by Chinese occupational exposure limits for hazardous agents in the workplace part 1 chemical hazardous agents (GBZ 2.1-2007).
|
1# coking |
8 |
0.0007 ± 0.0002 |
<0.005 |
0.03 |
0.15 ± 0.05 |
<0.6 |
0.25 ± 0.05 |
<1.2 |
0.30 ± 0.10 |
<3.3 |
2# coking |
13 |
0.0009 ± 0.0002 |
<0.005 |
0.03 |
0.28 ± 0.21 |
<0.6 |
0.28 ± 0.04 |
<1.2 |
0.60 ± 0.25 |
<3.3 |
Cold drum electric capture |
10 |
0.0008 ± 0.0001 |
<0.005 |
0.03 |
0.10 ± 0.02 |
<0.6 |
0.20 ± 0.06 |
<1.2 |
0.45 ± 0.12 |
<3.3 |
Crude benzene |
11 |
— |
<0.005 |
0.03 |
0.10 ± 0.03 |
<0.6 |
0.21 ± 0.05 |
<1.2 |
0.58 ± 0.10 |
<3.3 |
Ammonium sulfate |
7 |
— |
<0.005 |
0.03 |
0.11 ± 0.02 |
<0.6 |
0.30 ± 0.05 |
<1.2 |
0.69 ± 0.14 |
<3.3 |
Water pump |
1 |
0.0001 |
<0.005 |
0.03 |
0.01 |
<0.6 |
0.02 |
<1.2 |
0.05 |
<3.3 |
Desulfurization |
1 |
— |
<0.005 |
0.03 |
0.01 |
<0.6 |
0.02 |
<1.2 |
0.05 |
<3.3 |
Average |
|
0.0008 ± 0.0003 |
<0.005 |
0.03 |
0.17 ± 0.16 |
<0.6 |
0.24 ± 0.09 |
<1.2 |
0.48 ± 0.28 |
<3.3 |
Occupational exposure limit (OEL) |
— |
0.01 |
— |
3 |
6 |
10.0 |
50 |
100.0 |
50 |
100 |
Exceed the OEL (yes/no) |
|
No |
|
No |
No |
No |
No |
No |
No |
No |
3.2 Plasma PAH and urinary phenol concentrations
3.2.1 Plasma PAH concentrations.
The median (P25, P75) concentrations of 16 PAHs are shown in Table 2. Among them, Nap was excluded in the subsequent analysis, due to its large numerical variation, a wide range of sources, high background value and volatility, rapid biological metabolism, etc. We used the sum of the remaining 15 PAHs (∑15PAHs) to represent total PAH exposure for subsequent analysis, which was significantly or marginally correlated with each PAH homolog (Table S2†). Plasma ∑15PAH concentration was moderately higher in males than females (P < 0.10) (Fig. 1a), slightly increased with the educational level, and statistically decreased with age, employment duration, and BMI. No significant difference was found in ∑15PAH concentrations with tobacco smoking or alcohol drinking habits (P > 0.05).
Table 2 The concentrations of plasma PAHs (ng mL−1) and urinary phenol (μg mL−1)a
Items |
Median |
P
25
|
P
75
|
∑15PAHs refers to the addition of 15 PAHs, excluding Nap.
|
∑15PAHs |
8.85 |
4.8 |
18.83 |
Naphthalene (Nap) |
20.24 |
2.25 |
59.52 |
Acenaphthylene (Acy) |
0.24 |
0.11 |
0.45 |
Acenaphthene (Ace) |
1.79 |
0.78 |
2.69 |
Phenanthrene (Phe) |
0.64 |
0.04 |
2.04 |
Fluorene (Flu) |
1.41 |
0.05 |
5.74 |
Fluoranthene (Flt) |
0.11 |
0.05 |
0.36 |
Anthracene (Ant) |
0.14 |
0.03 |
0.54 |
Pyrene (Pyr) |
0.09 |
0.04 |
0.36 |
Benzo[a]anthracene (BaA) |
0.63 |
0.26 |
1.58 |
Chrysene (Chr) |
0.62 |
0.19 |
1.59 |
Benzo[k]fluoranthene (BkF) |
0.45 |
0.13 |
0.95 |
Benzo[b]fluoranthene (BbF) |
0.85 |
0.6 |
1.24 |
Benzo[a]pyrene (BaP) |
0.04 |
0.01 |
0.06 |
Dibenzo[a,h]anthracene (DhA) |
0.06 |
0.02 |
0.07 |
Indeno[1,2,3-cd]pyrene (InP) |
0.035 |
0.01 |
0.042 |
Benzo[g,h,i]perylene (BgP) |
0.056 |
0.027 |
0.056 |
Phenol |
6.00 |
0.03 |
31.00 |
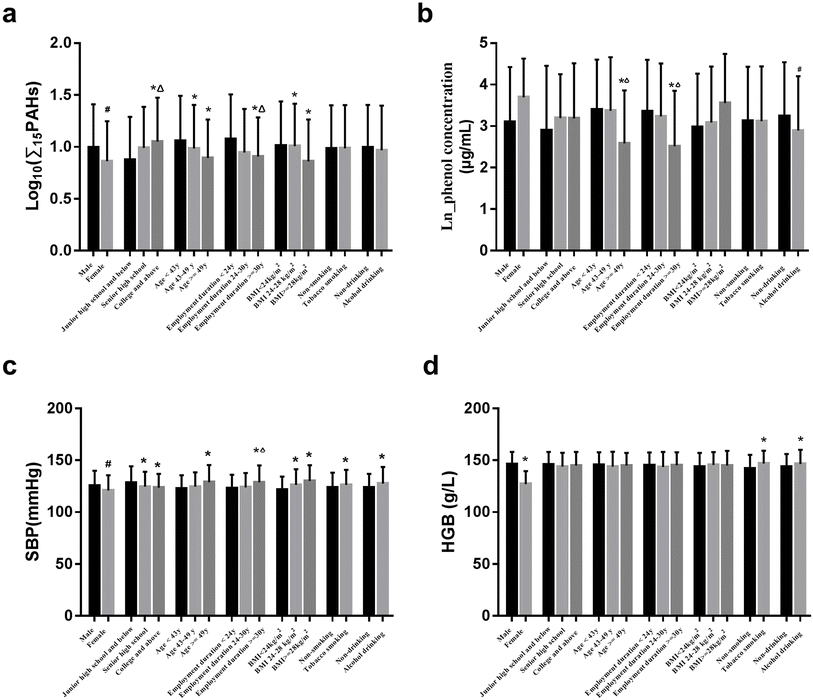 |
| Fig. 1 Changes of log10∑15PAHs concentrations, Ln_phenol concentrations, SBP, and HGB levels with the sociodemographic characteristics in the subjects. Panel a: changes of log10∑15PAHs concentrations with the sociodemographic characteristics in coking workers. Panel b: changes of Ln_phenol concentrations with the sociodemographic characteristics in coking workers; Panel c: changes of SBP levels with the sociodemographic characteristics in coking workers. Panel d: changes of HGB concentrations with the sociodemographic characteristics in coking workers. *: P < 0.05, compared to the first subgroup. #: P < 0.10, compared to the first subgroup. Δ: P < 0.05, compared to the second subgroup. | |
3.2.2 Urinary phenol concentrations.
The median (P25, P75) of urinary phenol concentration was 6.00 (0.03, 31.00) μg mL−1 (Table 2), which was significantly decreased with age and employment duration (P < 0.05) (Fig. 1b), and was marginally lower in drinkers than non-drinkers (P < 0.10). No significant difference was found in phenol concentrations with gender, educational level, BMI, and tobacco smoking habits (P > 0.10).
3.3 Health outcomes
The multiple health outcomes (pulmonary function, blood pressure, blood parameters, and blood glucose and lipids) were significantly varied with gender, age, duration of employment, BMI, and tobacco smoking, and alcohol drinking habits. For instance, the levels of SBP were significantly higher in those who were males, older, with a longer duration of employment, and people who are overweight, smokers, and drinkers (P < 0.05) (Fig. 1c).
3.4 Associations of coke oven emissions with health outcomes
3.4.1 Plasma PAHs and health outcomes.
Spearman correlation analysis found that ∑15PAH concentration was positively correlated with the HGB level (Table S3, see the ESI†). We categorized subjects into two subgroups of low ∑15PAHs (<8.85 ng mL−1) and high ∑15PAHs (≥8.85 ng mL−1) according to the median of plasma ∑15PAH concentration. The two subgroups were balanced in tobacco smoking and alcohol drinking habits (Table S4, see the ESI†), but were significantly different in gender, educational level, age, employment duration, and BMI. After adjusting for covariates including gender, educational level, employment duration, and BMI, covariance analysis indicated that the HGB levels and TG concentrations were significantly higher in the high ∑15PAH subgroup compared to the low ∑15PAH subgroup (P < 0.05) (Table 3). No significant difference was found in levels of FVC, FEV1, FEV1/FVC, SBP, DBP, WBC, RBC, PLT, GLU, TC, HDL, and LDL (P > 0.10). We used multiple linear regression to analyze the association of ∑15PAH concentration in categorical or continuous variables with the outcome after adjusting for covariates. Compared to the low ∑15PAHs (<8.85 ng mL−1), high ∑15PAH concentration (≥8.85 ng mL−1) was significantly associated with a 2.71-point (95% CI: 0.56, 4.86) increase in the HGB level and a 0.26-point (95% CI: 0.01, 0.50) increase in TG concentration (P < 0.05) (Table 4, Model 1). And the higher log10∑15PAH concentration was significantly associated with increases in HGB [3.14 (95% CI: 0.54, 5.74) g L−1] and TG levels [0.40 (95% CI: 0.11, 0.70) mmol L−1] (P < 0.05) (Table 4, Model 2). Then, restricted cubic spline (RCS) regression found the approximate linear relationships in TG, FVC, and PLT concentrations with PAH exposure, and the non-linear relationship of HGB levels with ∑15PAH concentrations (Pnon-linear < 0.05) after adjusting for covariates (Fig. S1, see the ESI†).
Table 3 Covariance analysis results of the health outcomes between the low PAHs group and high PAHs group (mean ± SD)a
Health items |
Low PAHs group (n = 283) |
High PAHs group (n = 283) |
Adjusted for gender, employment duration at the coking plant, BMI, and educational level. *: P < 0.05.
|
FVC |
80.91 ± 9.74 |
81.77 ± 10.01 |
FEV1 |
89.13 ± 10.49 |
90.38 ± 10.76 |
FEV1/FVC (%) |
111.87 ± 19.73 |
112.39 ± 20.62 |
SBP (mmHg) |
126.23 ± 15.47 |
124.03 ± 13.49 |
DBP (mmHg) |
87.28 ± 10.37 |
87.07 ± 8.77 |
WBC (× 109 L−1) |
6.19 ± 1.68 |
6.38 ± 1.69 |
RBC (× 1012 L−1) |
4.77 ± 0.37 |
4.82 ± 0.35 |
HGB (g L−1) |
143.27 ± 12.08 |
146.34 ± 13.64* |
PLT (× 109 L−1) |
224.37 ± 52.76 |
221.48 ± 52.25 |
GLU (mmol L−1) |
5.66 ± 1.64 |
5.49 ± 1.34 |
TC (mmol L−1) |
4.42 ± 0.69 |
4.37 ± 1.09 |
TG (mmol L−1) |
1.61 ± 1.16 |
1.77 ± 1.76* |
HDL (mmol L−1) |
1.09 ± 0.29 |
1.07 ± 0.26 |
LDL (mmol L−1) |
2.61 ± 0.59 |
2.56 ± 0.76 |
Table 4 Adjusted β coefficients and 95% CIs in the multiple linear regressions of the health outcomes with coke oven emissionsa
Model |
Independent variable |
FVC |
HGB |
PLT |
TG |
Models 1 and 2: adjusted for gender, employment duration at coking plant, BMI, and educational level. Model 1 is based on the ∑15PAHs concentrations in the categorical variable, and Model 2 is based on the log10∑15PAHs concentrations in the continuous variable. Models 3 and 4: adjusted for employment duration at the coking plant. Model 3 is based on the urinary phenol concentrations median sub-grouping, and Model 4 is based on the Ln_phenol concentrations. Model 5: adjusted for employment duration at the coking plant and BMI. *: P < 0.05, #: P < 0.10.
|
Model 1 |
Low ∑15PAHs |
|
— |
|
0.26 (0.01, 0.50)* |
High ∑15PAHs |
2.71 (0.56, 4.86)* |
Model 2 |
log10∑15PAHs |
|
3.14 (0.54, 5.74)* |
|
0.40 (0.11, 0.70)* |
Model 3 |
Low phenol subgroup |
— |
|
−13.08 (−24.83, −1.32)* |
|
High phenol subgroup |
1.94 (−0.37, 4.25)# |
Model 4 |
Ln_phenol |
0.39 (0.05, 0.73)* |
|
−2.40 (−4.12, −0.68)* |
Model 5 |
Ln_phenol |
0.45 (0.01, 0.88)* |
|
|
log10∑15PAHs |
3.25 (−0.47, 6.98)# |
3.4.2 Associations of urinary phenol with health outcomes.
Likewise, Pearson correlation analysis found that urinary phenol concentration was positively correlated with the levels of FVC and HGB, and was negatively associated with the PLT level and HDL concentration (P < 0.01) (Table S2, see the ESI†). Subjects were categorized into the low phenol subgroup (<6 μg mL−1) and high subgroup (≥6 μg mL−1) according to the median of urinary phenol concentration. As compared to the low phenol subgroup, the high phenol subgroup was significantly younger and had a significantly shorter employment duration at the coking plant (P < 0.05) (Table S4†). The two subgroups were balanced in gender, BMI, educational level, and tobacco smoking, and alcohol drinking habits (P > 0.10). After adjusting for employment duration, covariance analysis showed that the FVC level was marginally increased in the high phenol subgroup compared to the low subgroup (P < 0.10), the DBP level was significantly enhanced (P < 0.05), and PLT count was significantly reduced (P < 0.05) (Table 5). No significant difference was found in any other outcome between the two subgroups (P > 0.10). Multiple linear regression demonstrated that compared to the low phenol subgroup, phenol concentration was significantly associated with a 13.08-point (95% CI: −24.83, −1.32) decrease in PLT count (P < 0.05), and was marginally associated with a 1.94-point (95% CI: −0.37, 4.25) increase in FVC (P < 0.10) (Table 4, Model 3). And a higher Ln_phenol concentration was significantly associated with a 0.39-point (95% CI: 0.05, 0.73) increase in the FVC level and a 2.40-point (95% CI: −4.12, −0.68) decrease in PLT count, after adjusting for the employment duration at the coking plant (Table 4, Model 4). No significant association was found for urinary phenol concentration with other health outcomes.
Table 5 Covariance analysis results of the health outcomes between the low phenol group and the high phenol group (mean ± SD)a
Health outcomes |
Low phenol subgroup (n1 = 283) |
High phenol subgroup (n2 = 283) |
Adjusted for employment duration at coke plant; *: P < 0.05, #: P < 0.10.
|
FVC |
79.71 ± 9.28 |
81.65 ± 10.84# |
FEV1 |
89.73 ± 10.41 |
90.37 ± 11.65 |
FEV1/FVC (%) |
114.19 ± 19.23 |
112.58 ± 20.42 |
SBP (mmHg) |
123.07 ± 12.97 |
124.98 ± 15.35 |
DBP (mmHg) |
85.75 ± 9.10 |
87.63 ± 9.98* |
WBC (× 109 L−1) |
6.20 ± 1.66 |
6.23 ± 1.64 |
RBC (× 1012 L−1) |
4.79 ± 0.40 |
4.83 ± 0.33 |
HGB (g L−1) |
144.54 ± 14.46 |
146.48 ± 11.25 |
PLT (× 109 L−1) |
228.74 ± 52.36 |
215.66 ± 50.17* |
GLU (mmol L−1) |
5.61 ± 1.71 |
5.44 ± 1.05 |
TC (mmol L−1) |
4.35 ± 0.80 |
4.37 ± 0.80 |
TG (mmol L−1) |
1.72 ± 1.81 |
1.83 ± 1.31 |
HDL (mmol L−1) |
1.09 ± 0.29 |
1.03 ± 0.25 |
LDL (mmol L−1) |
2.54 ± 0.66 |
2.52 ± 0.65 |
3.4.3 Sensitivity analysis.
To further explore the relationships between COEs and health outcomes, we simultaneously included phenol concentration and PAH concentration in the multiple linear regression model and found a significant positive association of Ln_phenol concentration with the HGB level [0.45 (95% CI: 0.01, 0.88) g L−1] after adjusting for the employment duration and BMI (P < 0.05) (Table 4, Model 5), and a marginally positive association of log10∑15PAHs with the HGB level [3.25 (95% CI: −0.47, 6.98) g L−1] (P < 0.10). LASSO regression analysis was performed to screen out the major PAH homologs affecting the HGB level and indicated the main contributor of high ring PAHs (HR-PAHs, with 4 benzene rings and above) to the HGB level. More precisely, LASSO results showed the positive contributions of Inp (β = 1.81, P = 0.01) and Bbf (β = 1.49, P = 0.06) concentrations to the higher HGB level, and a negative association of Chr concentration with the HGB level (β = −1.02, P = 0.04) (Table 6). Furthermore, we used the Bayesian Kernel Machine Regression (BKMR) model to analyze the association of HR-PAHs with the HGB level. There was a positive overall effect of HR-PAHs on the HGB level when all six HR-PAHs were kept at or above their 50th percentile, as compared to when all HR-PAHs were at their median values (50th percentiles) (Fig. 2a). The concentration changes in BbF, BkF, and IcP from the 25th to 75th percentile were significantly associated with a significant increase in the HGB level (Fig. 2b), yet changes in BaP, DhA, and BgP concentrations were significantly negatively associated with a decrease in the HGB level. To explore the potential nonlinearity of the exposure-response function, the univariate relationship between each HR-PAH homolog and HGB level was estimated when all the other homologs were fixed at the 50th percentile. The results indicated that homologs of BbF, BkF, and IcP had significantly increased HGB levels, yet BaP, DhA, and BgP had decreased it (Fig. 2c), which was consistent with the findings in Fig. 2b. Subsequently, we observed the significant potential interactions between BkF and BbF, and between IcP and BbF, due to their intersectant correlation curves.
Table 6 The estimated coefficients for LASSO regression between 15 PAHs and HGB level
Variables |
Coefficients |
P
|
Bbf |
1.49 |
0.06 |
Inp |
1.81 |
0.01 |
Chr |
−1.02 |
0.04 |
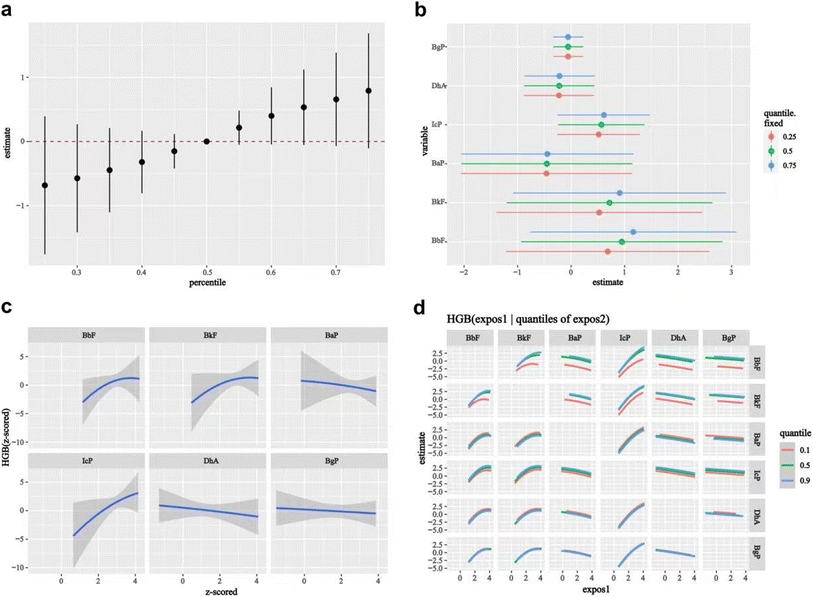 |
| Fig. 2 Bayesian Kernel Machine Regression (BKMR) analysis of HR-PAHs and HGB level. Panel a: overall effect (95% credible interval) of the HR-PAHs mixture on HGB level by BKMR model when all the exposures are at particular percentiles (as shown on the x-axis) were compared to when all the exposures are at the 50th percentile. Panel B: individual exposure contribution (95% credible interval) to the overall effect of the HR-PAHs on HGB by BKMR model as indicated by the change in HGB level when exposure is at the 25th compared to the 75th percentile, while all the other chemicals within the same class are fixed at either 25th, 50th, or 75th percentile (as indicated by fixed. quantile). Panel c: univariate exposure-response function (shaded area indicates 95% credible interval) between selected exposure and HGB, while the other exposures within the same class are fixed at the 50th percentile (i.e., median value). Panel d: bivariate exposure-response function for HGB. When the level of another HR-PAHs is fixed at 10%, 50%, and 90% (the other 9 pollutants are fixed at the median), the dose–response curve between one HR-PAHs homolog and HGB level. | |
4 Discussion
In this cross-sectional study, we analyzed the associations of occupational COE exposure with multiple health outcomes including pulmonary function, blood pressure, hematologic parameters, and blood glucose and lipids in coking workers. Although the average concentrations of COEs, benzene, methylbenzene, and xylene did not exceed the OEL stipulated by the Chinese national standard (GBZ 2.1-2007), plasma ∑15PAH concentration was found to be positively associated with the HGB level and TG concentration. Urinary phenol concentration was significantly associated with an increase in the FVC level and a decrease in the PLT level. The findings indicated the potential health impacts of occupational COE exposure on pulmonary function, hematologic parameters, and blood lipids in coking workers. It is suggested that we better understand and give more importance to the potential multiple health impacts of occupational exposure to COEs among coking workers in routine health monitoring. In addition, the findings may hint to researchers to further tighten the national standards related to coking workplaces, to protect the health of the occupational population as much as possible.
COEs are a major occupational hazard factor for coking workers, and contain a large number of carcinogenic PAHs, benzene, and benzene homologs.33 In this study, the airborne COE, benzene, methylbenzene, and xylene concentrations in the workplace were far below the OEL set by the Chinese national standards of occupational exposure limits for hazardous agents in the workplace (GBZ 2.1-2007), yet the results indicated that the current standards may still not be effective in preventing workers from suffering from potential health threats.
PAHs are a large group of chemicals with 2 to 7 fused aromatic rings and account for the largest proportion of COEs. Since plasma PAHs are not affected by differences in metabolism and excretion among individuals, it can be used as a biological monitoring indicator to reflect occupational PAH exposure. Measurement of PAHs in plasma or serum was useful for assessing accurate, quantitative personal exposure levels and potential toxic effects on the body.28,34–36 Due to the good correlation with each PAH homolog, we used the plasma ∑15PAH concentration to reflect total PAH exposure. In our study, we observed that the plasma ∑15PAH concentration was associated with gender, educational level, age, employment duration, and BMI, and we corrected these covariates in subsequent analyses to minimize confounding effects. The percentage of males and females (93% and 7%) and differences in the type and intensity of work may contribute to the difference. 40.28% of the subjects were highly educated (a college degree or above), which has a higher concentration of plasma ∑15PAHs and was inconsistent with previous research that showed higher PAH concentrations in less-educated subjects.37 Generally, an individual employee's job or working task is dominantly dependent on the educational level, even in the same workplace. This phenomenon forces us to prioritize the impact of educational attainment on exposure and health status in the upcoming follow-up study, from viewpoints of the exposure source in the working environment (job category, working duration, and occupational health protections), previous changes of activity in the workplace, personal habits of living, diet, behavior, and even emerging pollutant co-exposure, etc. Employment duration, a time-dependent confounder38 was found to have a negative association with the plasma ∑15PAH concentration, because the workers with longer employment duration likely having a more favorable health profile or more experience can be transferred to positions with less exposure.
Some PAHs are well-known as carcinogens, mutagens, and teratogens and therefore pose a serious threat to human health and well-being. For workers exposed to PAH mixtures and other occupational chemicals, a series of health problems (an increased risk of skin, lung, bladder, and gastrointestinal cancers) have been reported.39–41 PAH exposure was revealed to alter the hematological parameters (such as WBC, RBC, HGB, and PLT), which play critical roles in the process of occurrence, development, diagnosis, and treatment of diseases and disorders;23 however, the hematotoxicity of PAHs remains controversial.42–44 Among blood cells, RBCs and WBCs are universally measured, since RBCs are mainly responsible for the transportation of oxygen in the body, while WBCs are part of the body's defense system, and can protect the body against both infectious diseases and foreign invaders. With PAH exposure increasing, a decreased dose–response of counts for WBCs, eosinophils, monocytes, and lymphocytes was discovered in the occupational population in a two-year follow-up study.43 Urinary PAH metabolite was demonstrated to be associated with WBCs, HGB, lymphocyte counts, the neutrophil–lymphocyte ratio (NLR), and the platelet–lymphocyte ratio (PLR) among coke oven workers, which were more robust in females than males.45 The finding suggested that in addition to PAHs, gender also has a significant effect on blood cell count. Studies indicated a positive association of PAH exposure with increased PLR among the general population.46 Urinary PAH metabolite (9-OHPhe) was associated with an increment in WBC and neutrophil counts among male coke oven workers,45 which was consistent with studies among other male workers.44,47 However, in this study, no association was found in PAH exposure with WBC, RBC, and PLT counts, which may be related to gender differences, PAH exposure, and other potential factors. In this study, we found that the HGB level was higher in males, smokers, and drinkers, which was consistent with previous research.48,49 Also, the HGB level presented a non-linear relationship with ∑15PAH concentration. LASSO and BKMR analysis demonstrated that BbF and IcP homologs contributed positively to the HGB level, while Chr contributed negatively. HGB is considered to be an oxygen sensor, providing information to organisms to adjust metabolism or biosynthesis to meet oxygen needs. HGB was significantly increased in both male and female rats when they were subchronically subjected to oral benzo[a]pyrene (BaP) exposure.50 Studies have reported an association of PAH metabolite with a reduced HGB level among female coking workers45 and brick kiln workers.51 The divergence is possibly due to the difference in PAH exposure, population characteristics, co-exposure to other contaminants, and other potential confounding factors.
This study also indicated a significant positive association of PAH exposure with serum TG concentration in coking workers, which was consistent with the increased TG concentration in the high PAH exposure adults52 and garage workers.32 High TG is a risk factor for cardiovascular disease (CVD), and investigations have discovered a positive relationship between PAH exposure at work and CVD risk in humans.53 Serum TG mediated the association between PAHs and nonalcoholic fatty liver disease.54 Following intratracheally instilled BaP (5 mg mL−1), hepatic TG concentration was markedly increased in C57BL/6 mice in a time-dependent pattern.55 No association was found between PAH exposure and other health outcomes in coking workers.
Benzene, another important component of COEs, has been classified as one of the human carcinogens by the International Agency for Research on Cancer (IARC) since 1982.2 Recent studies have found that low-concentration benzene exposure was associated with some non-cancerous diseases.22 To further reveal the potential health effects of benzene exposure in coking workers, we used the urinary phenol concentration as the benzene biological exposure monitoring indicator, since phenol accounts for 70–85% of benzene metabolites in urine when the airborne benzene concentration is in the range of 0.1–10 ppm.56 In this study, we observed that the employment duration and age were significantly associated with urinary phenol concentration, and was adjusted as a covariate in the following analysis. FVC is the most important indicator of respiratory resistance among FVC, FEV1, and FEV1/FVC. In this study, we found that benzene exposure was significantly associated with an increase in FVC level, but was not associated with FEV1 or FEV1/FVC levels. This finding in our study is inconsistent with previous results that indicated the significantly decreased FVC in workers who were occupationally exposed to benzene, toluene, ethylbenzene & xylene (BTEX),57 and the significant association of a cumulative benzene soluble fraction (BSF) or benzene exposure with the decreases in the FVC, FEV1, and FEV1/FVC in coking workers or in petrol pump workers.58 The divergence is possibly due to the distinct difference in benzene exposure characteristics and levels, co-exposure to other contaminants, the demographic characteristics of the population, and other potential confounding factors. The increased FVC of coking workers may be related to their lifestyle (including physical activity). Still, compensatory pulmonary function factors cannot be excluded, and need further investigation in the upcoming cohort study. Blood pressure refers to arterial blood pressure including SBP and DBP, and can be affected by multiple factors. The higher SBP was observed in males with lower education levels, age, longer employment duration, who were obese and overweight, smoking, or drinking. In this study, we found a significant positive association of benzene exposure with an increase in DBP in coking workers, which is consistent with the finding of Ataro Z. et al. in garage workers.59 Higher urine phenol concentration was significantly associated with a higher incidence of hypertension in subjects.60 Benzene exposure was associated with a higher prevalence of hypertension (prevalence ratio (PR) = 1.05, 95% confidence interval (CI) = 1.02, 1.08).61 A possible mechanism of benzene-induced hypertension could be a disturbance of the nitric oxide process.
Recently accelerating studies have indicated that PLT plays a critical role in immune and inflammatory processes, for instance, PLT participates in the regulation of leukocyte recruitment by releasing numerous inflammatory mediators.62 In this study, we found a negative association between urinary phenol concentration and the PLT level in coking workers, which was concordant with a significant decrease in PLT counts in Egyptian taxi drivers.63 Long-term exposure to low concentrations of PAHs was also associated with alterations in PLT indices in a longitudinal study in China.64 PLT counts were significantly lower in workers exposed to benzene (below 1 ppm in air).65 PLT parameters of large-platelet cell ratios (P-LCR), platelet distribution width (PDW), mean platelet volume (MPV), and platelet-associated immunoglobulin levels were significantly elevated in benzene-exposed workers.66 Benzene exposure induced an immunosuppressive effect on the adaptive immune system and activation of the innate immune system to cause inflammation in humans.67 Exposure to a mixture of formaldehyde, benzene, toluene, and xylene significantly decreased RBC and PLT counts in peripheral blood in mice.68 The reduced PLT in this study indicated the potential immunosuppression and inflammation in coking workers.
In addition, we observed that multiple health outcomes (such as systolic blood pressure, hemoglobin, etc.) varied significantly with tobacco smoking habits as shown in Fig. 1, but the two subgroups were balanced in terms of tobacco smoking habits in the later analysis. The possible reasons are various, such as the different basis of sample selection, male-female ratio, etc. Nonetheless, the impact of smoking on the health of the occupational population is still not negligible. It is reported that the combined effect of smoking and occupational hazards was evident from the significant differences in morbidity between smokers and non-smokers across many occupations, and the interaction between these two types of hazards increases the risk of various diseases, particularly chronic obstructive pulmonary diseases, lung cancer, and cardiovascular diseases, as well as disabilities.69–71 We will further explore the potential impact of smoking on the relationship between COE exposure and health outcomes in our future studies.
This study has notable strengths and limitations. First of all, we conducted a cross-sectional pilot study to investigate the potential association of COE exposure with multiple health outcomes in coking workers. Secondly, we comprehensively evaluated PAH and benzene exposure levels in terms of both external and internal exposure, used plasma ∑15PAH concentration to reflect total PAH exposure due to their relative stability and good correlation with each PAH homolog, and adopted urinary phenol concentration to represent benzene exposure. Thirdly, the internal controls of the same coking plant were selected for comparison to enhance comparability between different groups. Fourthly, we used covariance analysis, the multiple linear regression model, and RCS to analyze the association of COE exposure with multiple health outcomes, and further used BKMR to analyze the combined effects, dose responses, and interactions of PAH homologs on health outcomes. In addition, LASSO and BKMR were used for sensitivity analysis to enhance the credibility of the results. Lastly, a moderate to large sample size (n = 566) was used to ensure statistical validity as much as possible.
We recognized that our study has some limitations. We didn't measure other internal exposure biomarkers of benzene exposure, such as trans,trans-muconic acid (tt-MA), or S-phenyl mercapturic acid (S-PMA), considering that the phenol concentrations can represent 70–85% of benzene metabolites in urine when the airborne benzene concentrations ranged from 0.1–10 ppm,56 which will further be determined in the subsequent study. Secondly, we neglected to measure blood lymphocyte levels or other immune response parameters, such as immunoglobulin E and interleukin 4, which could serve as early indicators of pathological conditions and are important for a more comprehensive assessment of immune responses related to lung function injury. Besides, there were also some metal compounds in COEs that could induce hematological disturbances. Then, physical activity was not included in the questionnaire items in this study and should be included in the subsequent investigation since it is becoming a concern for human health in the study field of modern public health. The findings suggest we better understand and attach more importance to the potential multiple health impacts of occupational COEs among coking workers, even though the exposure was far below the OEL. The findings also suggest we further tighten the national standards in the workplace and proceed with a well-designed prospective cohort study in the future.
5 Conclusion
In conclusion, our population study clearly showed that PAH exposure could damage hematological parameters and blood lipids, and benzene exposure could increase blood pressure and decrease PLT count.
Ethical statement
The protocol was conducted by the Declaration of Helsinki and was approved by the Ethics and Human Subjects Committees of Shanxi Medical University (2019LL111), and was performed in line with the principles of the Declaration of Helsinki.
Consent to participate
Informed consent was obtained from all individual participants included in the study.
Consent to publish
Neither the entire paper nor any part of its content has been published or has been accepted elsewhere. All authors give their consent to publish all submitted materials.
Data availability
There are ethical restrictions on the sharing of de-identified data for this study. The ethics committee has not agreed to the public sharing of data as we do not have the participants' permission to share their anonymous data.
Author contributions
All authors contributed to the study's conception and design. Material preparation, acquisition of data, and data curation were performed by Xin Li, Xiaoling Zhou, Huixiang Nie, Guoshun Huang, and Xuhong Wang. Data analysis was performed by Min Sun, Xin Li, Mengmeng Geng, Zhiyan Zhang, and Na Xia. The first draft of the manuscript was written by Min Sun and all authors commented on previous versions of the manuscript. Hongmei Zhang revised the manuscript and approved the final version for publication. All authors read and approved the final manuscript.
Conflicts of interest
There are no conflicts of interest to declare.
Acknowledgements
This work received financial support from the National Natural Science Foundation of China (NSFC81973093) and the Shanxi Provincial Basic Research Program (202403021211123 and 202403021211124). The authors would like to appreciate and acknowledge the support and efforts from the staff of the 6th affiliated hospital of Shanxi Medical University. We would like to honestly thank the participants for their great contributions to this study.
References
- Q. He, Y. Yan, Y. Zhang, X. Wang and Y. Wang, Coke workers' exposure to volatile organic compounds in northern China: a case study in Shanxi Province, Environ. Monit. Assess., 2015, 187, 359 CrossRef PubMed.
- D. B. Clayson, IARC monographs on the evaluation of the carcinogenic risk of chemicals to humans. Volume 34, polynuclear aromatic compounds, part 3. Industrial exposures in aluminium production, coal gasification, coke production and iron and steel founding: Internation, Cancer Lett., 1985, 28, 243 Search PubMed.
- L. Tomatis, IARC benzene report, Science, 1982, 218, 214 CrossRef CAS.
- X. Zhang, L. Yang, H. Zhang, W. Xing, Y. Wang, P. Bai, L. Zhang, K. Hayakawa, A. Toriba, Y. Wei and N. Tang, Assessing Approaches of Human Inhalation Exposure to Polycyclic Aromatic Hydrocarbons: A Review, Int. J. Environ. Res. Public Health, 2021, 18, 3124 CrossRef CAS PubMed.
- S. L. Gale, E. M. Noth, J. Mann, J. Balmes, S. K. Hammond and I. B. Tager, Polycyclic aromatic hydrocarbon exposure and wheeze in a cohort of children with asthma in Fresno, CA, J. Exposure Sci. Environ. Epidemiol., 2012, 22, 386–392 CrossRef CAS PubMed.
- I. Burstyn, P. Boffetta, D. Heederik, T. Partanen, H. Kromhout, O. Svane, S. Langard, R. Frentzel-Beyme, T. Kauppinen, I. Stucker, J. Shaham, W. Ahrens, S. Cenee, G. Ferro, P. Heikkila, M. Hooiveld, C. Johansen, B. G. Randem and W. Schill, Mortality from obstructive lung diseases and exposure to polycyclic aromatic hydrocarbons among asphalt workers, Am. J. Epidemiol., 2003, 158, 468–478 CrossRef.
- B. Moorthy, C. Chu and D. J. Carlin, Polycyclic aromatic hydrocarbons: from metabolism to lung cancer, Toxicol. Sci., 2015, 145, 5–15 CrossRef CAS PubMed.
- I. Burstyn, H. Kromhout, C. Johansen, S. Langard, T. Kauppinen, J. Shaham, G. Ferro and P. Boffetta, Bladder cancer incidence and exposure to polycyclic aromatic hydrocarbons among asphalt pavers, Occup. Environ. Med., 2007, 64, 520–526 CrossRef CAS.
- D. G. Lee, I. Burstyn, A. S. Lai, A. Grundy, M. C. Friesen, K. J. Aronson and J. J. Spinelli, Women's occupational exposure to polycyclic aromatic hydrocarbons and risk of breast cancer, Occup. Environ. Med., 2019, 76, 22–29 CrossRef.
- H. Jeng, W. Y. Lin, M. R. Chao, W. Y. Lin and C. H. Pan, Semen quality and sperm DNA damage associa -revised - final-finalted with oxidative stress in relation to exposure to polycyclic aromatic hydrocarbons, J. Environ. Sci. Health, Part A: Toxic/Hazard. Subst. Environ. Eng., 2018, 53, 1221–1228 CrossRef CAS.
- U. Luderer, F. Christensen, W. O. Johnson, J. She, H. S. Ip, J. Zhou, J. Alvaran, E. J. Krieg and J. S. Kesner, Associations between urinary biomarkers of polycyclic aromatic hydrocarbon exposure and reproductive function during menstrual cycles in women, Environ. Int., 2017, 100, 110–120 CrossRef CAS.
- I. Burstyn, H. Kromhout, T. Partanen, O. Svane, S. Langard, W. Ahrens, T. Kauppinen, I. Stucker, J. Shaham, D. Heederik, G. Ferro, P. Heikkila, M. Hooiveld, C. Johansen, B. G. Randem and P. Boffetta, Polycyclic aromatic hydrocarbons and fatal ischemic heart disease, Epidemiology, 2005, 16, 744–750 CrossRef.
- M. A. Mallah, M. A. Mallah, Y. Liu, H. Xi, W. Wang, F. Feng and Q. Zhang, Relationship Between Polycyclic Aromatic Hydrocarbons and Cardiovascular Diseases: A Systematic Review, Front. Public Health, 2021, 9, 763706 CrossRef.
- Y. S. Min, H. S. Lim and H. Kim, Biomarkers for polycyclic aromatic hydrocarbons and serum liver enzymes, Am. J. Ind. Med., 2015, 58, 764–772 CrossRef CAS PubMed.
- T. H. Yuan, D. Y. Ke, J. E. Wang and C. C. Chan, Associations between renal functions and exposure of arsenic and polycyclic aromatic hydrocarbon in adults living near a petrochemical complex, Environ. Pollut., 2020, 256, 113457 CrossRef CAS.
- C. Fenga, S. Gangemi, F. Giambo, C. Tsitsimpikou, K. Golokhvast, A. Tsatsakis and C. Costa, Low-dose occupational exposure to benzene and signal transduction pathways involved in the regulation of cellular response to oxidative stress, Life Sci., 2016, 147, 67–70 CrossRef CAS PubMed.
- O. Inoue, K. Seiji, M. Kasahara, H. Nakatsuka, T. Watanabe, S. G. Yin, G. L. Li, C. Jin, S. X. Cai, X. Z. Wang and A. Et, Quantitative relation of urinary phenol levels to breathzone benzene concentrations: a factory survey, Br. J. Ind. Med., 1986, 43, 692–697 CAS.
- A. R. Schnatter, D. C. Glass, G. Tang, R. D. Irons and L. Rushton, Myelodysplastic syndrome and benzene exposure among petroleum workers: an international pooled analysis, J. Natl. Cancer Inst., 2012, 104, 1724–1737 CrossRef CAS.
- R. A. Rinsky, R. J. Young and A. B. Smith, Leukemia in benzene workers, Am. J. Ind. Med., 1981, 2, 217–245 CrossRef CAS.
- T. Sorahan, L. J. Kinlen and R. Doll, Cancer risks in a historical UK cohort of benzene exposed workers, Occup. Environ. Med., 2005, 62, 231–236 CrossRef CAS.
- E. Paci, E. Buiatti, A. S. Costantini, L. Miligi, N. Pucci, A. Scarpelli, G. Petrioli, L. Simonato, R. Winkelmann and J. M. Kaldor, Aplastic anemia, leukemia and other cancer mortality in a cohort of shoe workers exposed to benzene, Scand. J. Work, Environ. Health, 1989, 15, 313–318 CrossRef CAS.
- T. Tunsaringkarn, S. Soogarun and A. Palasuwan, Occupational exposure to benzene and changes in hematological parameters and urinary trans, trans-muconic acid, Int. J. Occup. Environ. Med., 2013, 4, 45–49 CAS.
- L. L. Ye, G. H. Zhang, J. W. Huang, Y. Li, G. Q. Zheng, D. T. Zhang, L. F. Zhou, X. D. Tao, J. Zhang, Y. J. Ye, P. Sun, A. Frank and Z. L. Xia, Are polymorphisms in metabolism protective or a risk for reduced white blood cell counts in a Chinese population with low occupational benzene exposures?, Int. J. Occup. Environ. Health, 2015, 21, 232–240 CrossRef CAS PubMed.
- D. H. Koh, H. K. Jeon, S. G. Lee and H. W. Ryu, The relationship between low-level benzene exposure and blood cell counts in Korean workers, Occup. Environ. Med., 2015, 72, 421–427 CrossRef PubMed.
- S. A. Robert, P. J. Kerzic, Y. Zhou, M. Chen, M. J. Nicolich, K. Lavelle, T. W. Armstrong, M. G. Bird, L. Lin, H. Fu and R. D. Irons, Peripheral blood effects in benzene-exposed workers, Chem. Biol. Interact., 2010, 184, 174–181 CrossRef.
- M. A. D'Andrea and G. K. Reddy, Benzene exposure from the BP refinery flaring incident alters hematological and hepatic functions among smoking subjects, Int. J. Occup. Med. Environ. Health, 2017, 30, 849–860 Search PubMed.
-
E. B. R. Desapriya, T. Stockwell, S. R. Doll and R. Room, International Guide for Monitoring Alcohol Consumption and Related Harm, World Health Organization, Geneva, 2000 Search PubMed.
- J. D. Pleil, M. A. Stiegel, J. R. Sobus, S. Tabucchi, A. J. Ghio and M. C. Madden, Cumulative exposure assessment for trace-level polycyclic aromatic hydrocarbons (PAHs) using human blood and plasma analysis, J. Chromatogr. B: Anal. Technol.
Biomed. Life Sci., 2010, 878, 1753–1760 CrossRef CAS.
- R. W. Hornung and L. D. Reed, Estimation of Average Concentration in the Presence of Nondetectable Values, Appl. Occup. Environ. Hyg., 1990, 5, 46–51 Search PubMed.
- M. R. Miller, J. Hankinson, V. Brusasco, F. Burgos, R. Casaburi, A. Coates, R. Crapo, P. Enright, C. P. van der Grinten, P. Gustafsson, R. Jensen, D. C. Johnson, N. MacIntyre, R. McKay, D. Navajas, O. F. Pedersen, R. Pellegrino, G. Viegi and J. Wanger, Standardisation of spirometry, Eur. Respir. J., 2005, 26, 319–338 CrossRef CAS.
- J. A. Whitworth, 2003 World Health Organization (WHO)/International Society of Hypertension (ISH) statement on management of hypertensionJ. Hypertens., 2003, 21, 1983–1992 Search PubMed.
- Z. Ataro, A. Geremew and F. Urgessa, Chemical exposure in garage workers and related health risks on the biochemical levels: A comparative study in Harar town, eastern Ethiopia, SAGE Open Med., 2019, 7, 2106918792 Search PubMed.
- K. Zou, P. Wang, X. Duan, Y. Yang, H. Zhang, S. Wang, L. Shi, Y. Wang, W. Yao and W. Wang, Benchmark dose estimation for coke oven emissions based on oxidative damage in Chinese exposed workers, Ecotoxicol. Environ. Saf., 2020, 202, 110889 CrossRef CAS PubMed.
- X. F. Song, Z. Y. Chen, Z. J. Zang, Y. N. Zhang, F. Zeng, Y. P. Peng and C. Yang, Investigation of polycyclic aromatic hydrocarbon level in blood and semen quality for residents in Pearl River Delta Region in China, Environ. Int., 2013, 60, 97–105 CrossRef CAS.
- X. Xia, A. Carroll-Haddad, N. Brown, M. J. Utell, C. T. Mallon and P. K. Hopke, Polycyclic Aromatic Hydrocarbons and Polychlorinated Dibenzo-p-Dioxins/Dibenzofurans in Microliter Samples of Human Serum as Exposure Indicators, J. Occup. Environ. Med., 2016, 58, S72–S79 CrossRef CAS.
- X. Zhang, X. Li, Y. Jing, X. Fang, X. Zhang, B. Lei and Y. Yu, Transplacental transfer of polycyclic aromatic hydrocarbons in paired samples of maternal serum, umbilical cord serum, and placenta in Shanghai, China, Environ. Pollut., 2017, 222, 267–275 CrossRef CAS PubMed.
- E. L. Sabbath, M. M. Glymour, C. Berr, A. Singh-Manoux, M. Zins, M. Goldberg and L. F. Berkman, Occupational solvent exposure and cognition: does the association vary by level of education?, Neurology, 2012, 78, 1754–1760 CrossRef CAS PubMed.
- Y. Ko, S. C. Howard, A. P. Golden and B. French, Adjustment for duration of employment in occupational epidemiology, Ann. Epidemiol., 2024, 94, 33–41 CrossRef PubMed.
- D. L. Diggs, A. C. Huderson, K. L. Harris, J. N. Myers, L. D. Banks, P. V. Rekhadevi, M. S. Niaz and A. Ramesh, Polycyclic aromatic hydrocarbons and digestive tract cancers: a perspective, J. Environ. Sci. Health, Part C: Environ. Carcinog. Ecotoxicol. Rev., 2011, 29, 324–357 CrossRef CAS.
- L. Yang, K. Yan, D. Zeng, X. Lai, X. Chen, Q. Fang, H. Guo, T. Wu and X. Zhang, Association of polycyclic aromatic hydrocarbons metabolites and risk of diabetes in coke oven workers, Environ. Pollut., 2017, 223, 305–310 CrossRef CAS.
- C. Barul and M. E. Parent, Occupational exposure to polycyclic aromatic hydrocarbons and risk of prostate cancer, Environ. Health, 2021, 20, 71 CrossRef CAS PubMed.
- J. R. Clark, B. Serdar, D. J. Lee, K. Arheart, J. D. Wilkinson and L. E. Fleming, Exposure to polycyclic aromatic hydrocarbons and serum inflammatory markers of cardiovascular disease, Environ. Res., 2012, 117, 132–137 CrossRef CAS.
- Y. Wang, H. Zhao, T. Wang, X. Liu, Q. Ji, X. Zhu, J. Sun, Q. Wang, H. Yao, Y. Niu, Q. Jia, W. Su, W. Chen, Y. Dai, Q. Zhi, W. Wang, Y. Li, A. Gao and H. Duan, Polycyclic aromatic hydrocarbons exposure and hematotoxicity in occupational population: A two-year follow-up study, Toxicol. Appl. Pharmacol., 2019, 378, 114622 CrossRef CAS.
- T. Zhang, X. L. Zhou, J. Yang, C. Zhang, Q. Q. Meng, Z. W. Xue, W. B. Li, Q. An, Z. Q. Liu and J. Q. Yang, Effect of PAHs on Routine Blood and Immunoglobulin Indices of Residents Living in Areas Polluted by Coking, Biomed. Environ. Sci., 2020, 33, 286–293 CAS.
- C. Liu, M. Wu, M. Fu, H. Wang and J. Nie, Dose-response relationships between polycyclic aromatic hydrocarbon exposure and blood cell counts among coke oven workers: a sex-stratified analysis, BMJ Open, 2021, 11, e46843 Search PubMed.
- C. Yuan, J. Hou, Y. Zhou, C. Hu, H. Sun, W. Chen and J. Yuan, Dose-response relationships between polycyclic aromatic hydrocarbons exposure and platelet indices, Environ. Pollut., 2019, 245, 183–198 CrossRef CAS PubMed.
- A. Kamal, A. Cincinelli, T. Martellini and R. N. Malik, Biomarkers of PAH exposure and hematologic effects in subjects exposed to combustion emission during residential (and professional) cooking practices in Pakistan, Environ. Sci. Pollut. Res., 2016, 23, 1284–1299 CrossRef CAS.
- M. A. Mallah, L. Changxing, M. A. Mallah, S. Noreen, Y. Liu, M. Saeed, H. Xi, B. Ahmed, F. Feng, A. A. Mirjat, W. Wang, A. Jabar, M. Naveed, J. H. Li and Q. Zhang, Polycyclic aromatic hydrocarbon and its effects on human health: overview, Chemosphere, 2022, 296, 133948 CrossRef CAS PubMed.
- E. Shakiba, M. Moradinazar, Z. Rahimi, F. Najafi, Y. Pasdar and M. Kohsari, Tobacco smoking and blood parameters in the Kurdish population of Iran, BMC Cardiovasc. Disord., 2023, 23, 401 CrossRef CAS PubMed.
- M. E. Knuckles, F. Inyang and A. Ramesh, Acute and subchronic oral toxicities of benzo[a]pyrene in F-344 rats, Toxicol. Sci., 2001, 61, 382–388 CrossRef CAS.
- A. Kamal, R. N. Malik, T. Martellini and A. Cincinelli, PAH exposure biomarkers are associated with clinico-chemical changes in the brick kiln workers in Pakistan, Sci. Total Environ., 2014, 490, 521–527 CrossRef CAS.
- S. Srivastava, Effects of Environmental Polycyclic Aromatic Hydrocarbons Exposure and Pro-Inflammatory Activity on Type 2 Diabetes Mellitus in US Adults, Open J. Air Pollut., 2022, 11, 29–46 CrossRef CAS PubMed.
- M. A. Mallah, L. Changxing, M. A. Mallah, S. Noreen, Y. Liu, M. Saeed, H. Xi, B. Ahmed, F. Feng, A. A. Mirjat, W. Wang, A. Jabar, M. Naveed, J. H. Li and Q. Zhang, Polycyclic aromatic hydrocarbon and its effects on human health: An overview, Chemosphere, 2022, 296, 133948 CrossRef CAS.
- Z. Hu, Y. Li, Y. Yang, W. Yu, W. Xie, G. Song, Y. Qian and Z. Song, Serum lipids mediate the relationship of multiple polyaromatic hydrocarbons on non-alcoholic fatty liver disease: A population-based study, Sci. Total Environ., 2021, 780, 146563 CrossRef CAS PubMed.
- F. Li, B. Xiang, Y. Jin, C. Li, S. Ren, Y. Wu, J. Li and Q. Luo, Hepatotoxic effects of inhalation exposure to polycyclic aromatic hydrocarbons
on lipid metabolism of C57BL/6 mice, Environ. Int., 2020, 134, 105000 CrossRef CAS.
- S. Kim, R. Vermeulen, S. Waidyanatha, B. A. Johnson, Q. Lan, M. T. Smith, L. Zhang, G. Li, M. Shen, S. Yin, N. Rothman and S. M. Rappaport, Modeling human metabolism of benzene following occupational and environmental exposures, Cancer Epidemiol., Biomarkers Prev., 2006, 15, 2246–2252 CrossRef CAS.
- B. Harati, S. J. Shahtaheri, A. Karimi, K. Azam, A. Ahmadi, R. M. Afzali and A. Harati, Evaluation of Respiratory Symptoms among Workers in an Automobile Manufacturing Factory, Iran, Iran. J. Public Health, 2018, 47, 237–245 Search PubMed.
- Y. Hu, B. Chen, Z. Yin, L. Jia, Y. Zhou and T. Jin, Increased risk of chronic obstructive pulmonary diseases in coke oven workers: interaction between occupational exposure and smoking, Thorax, 2006, 61, 290–295 CrossRef CAS PubMed.
- Z. Ataro, A. Geremew and F. Urgessa, Occupational health risk of working in garages: a comparative study on blood pressure and hematological parameters between garage workers and Haramaya University community, Harar, eastern Ethiopia, Risk Manag. Healthc. Pol., 2018, 11, 35–44 CrossRef PubMed.
- V. Wiwanitkit, Benzene exposure and hypertension: an observation, Cardiovasc. J. Afr., 2007, 18, 264–265 Search PubMed.
- J. Xu, N. M. Niehoff, A. J. White, E. J. Werder and D. P. Sandler, Fossil-fuel and combustion-related air pollution and hypertension in the Sister Study, Environ. Pollut., 2022, 315, 120401 CrossRef CAS PubMed.
- C. Bakogiannis, M. Sachse, K. Stamatelopoulos and K. Stellos, Platelet-derived chemokines in inflammation and atherosclerosis, Cytokine, 2019, 122, 154157 CrossRef PubMed.
- Z. A. Kasemy, G. M. Kamel, G. M. Abdel-Rasoul and A. A. Ismail, Environmental and Health Effects of Benzene Exposure among Egyptian Taxi Drivers, J. Environ. Public Health, 2019, 2019, 7078024 Search PubMed.
- J. Cui, T. Zhang, C. Zhang, Z. Xue, D. Chen, X. Kong, C. Zhao, Y. Guo, Z. Li, X. Liu, J. Duan, W. Peng, X. Zhou and H. Yu, Long-term exposure to low concentrations of polycyclic aromatic hydrocarbons and alterations in platelet indices: A longitudinal study in China, PLoS One, 2022, 17, e276944 Search PubMed.
- Q. Lan, L. Zhang, G. Li, R. Vermeulen, R. S. Weinberg, M. Dosemeci, S. M. Rappaport, M. Shen, B. P. Alter, Y. Wu, W. Kopp, S. Waidyanatha, C. Rabkin, W. Guo, S. Chanock, R. B. Hayes, M. Linet, S. Kim, S. Yin, N. Rothman and M. T. Smith, Hematotoxicity in workers exposed to low levels of benzene, Science, 2004, 306, 1774–1776 CrossRef CAS PubMed.
- J. Huang, M. Zhao, P. Wang, X. Li, L. Ma, J. Zhang and Y. Zhou, Effects of low concentrations of benzene exposure on levels of platelet-associated antibodies and platelet parameters, J. Occup. Environ. Med., 2014, 56, e92–e97 CrossRef CAS PubMed.
- H. Guo, S. Ahn and L. Zhang, Benzene-associated immunosuppression and chronic inflammation in humans: a systematic review, Occup. Environ. Med., 2021, 78, 377–384 CrossRef.
- F. Wang, F. Liu, H. Liu, W. Chen, X. Si and X. Ma, Effects of immunological and hematological parameter in mice exposed to mixture of volatile organic compounds, Inhalation Toxicol., 2016, 28, 164–169 CrossRef CAS.
- J. P. Mackenbach, R. A. Damhuis and J. V. Been, The effects of smoking on health: growth of knowledge reveals
even grimmer picture, Ned. Tijdschr. Geneeskd., 2017, 160, D869 CAS.
- L. Ma, X. Huo, A. Yang, S. Yu, H. Ke, M. Zhang and Y. Bai, Metal Exposure, Smoking, and the Risk of COPD: A Nested Case-Control Study in a Chinese Occupational Population, Int. J. Environ. Res. Public Health, 2022, 19, 10896 CrossRef CAS PubMed.
- L. L. Roach, The Relationship of Welding Fume Exposure, Smoking, and Pulmonary Function in Welders, Workplace Health & Saf., 2018, 66, 3 Search PubMed.
|
This journal is © The Royal Society of Chemistry 2025 |
Click here to see how this site uses Cookies. View our privacy policy here.