PFAS surveillance within a highly militarized island: a case study of Okinawa, Japan†
Received
29th August 2024
, Accepted 28th October 2024
First published on 3rd December 2024
Abstract
Per- and polyfluoroalkyl substances (PFAS) are a resilient class of anthropogenic contaminants of emerging concern with over 12
000 individual compounds that have been noted for industrial applications, consumer goods, and food packaging materials. In general, the most common contributors to PFAS environmental pollution are aviation facilities, specifically those that use aqueous film forming foams (e.g., at military bases and airports). In this study, we examined the presence of PFAS across Okinawa Island (Japan) due to its large-scale U.S. military presence throughout the island. Surface water was collected at 61 sites across the island to achieve maximum geographical coverage of the island while also collecting near suspected PFAS sources; 31 PFAS were monitored using a 12 min HPLC-MS/MS method. A total of 15 PFAS were detected and quantified around the island with a mean Σ15PFAS of 16.3 ng L−1 and a maximum site concentration of 164.3 ng L−1. Region-specific PFAS profiles were observed across the island, including the overwhelming presence of PFHpA in the northern region of the island, revealing the possibility of multiple PFAS source points in Okinawa. The resultant data herein provides the first island-wide examination of PFAS “hotspots” across Okinawa and is a critical first step toward increasing PFAS awareness and action.
Environmental significance
Per- and polyfluoroalkyl substances (PFAS) are an expanding class of over 14 000 anthropogenic organofluorine chemicals utilized for their thermal stability and physiochemical properties. Their long-term use, specifically relating to military and aviation industries, has led to their persistence in the environment. As the toxicological effects of PFAS have been increasingly explored over the last decade, the overwhelming U.S. military presence on the small island of Okinawa, Japan has left the local population worried about the human and environmental health impacts these known sources may pose. This study provides the first comprehensive analysis of PFAS spanning the entire island of Okinawa, Japan. A targeted HPLC-MS/MS approach was employed to determine presence and concentrations of 31 PFAS in coastal and inland waters.
|
1 Introduction
The island of Okinawa, Japan, encompasses approximately 1200 km2 of land, making it the largest of the Ryukyus Islands with a population of approximately 1.3 million people.1,2 Juxtaposed to this robust population density, the island is surrounded by vulnerable fringing reefs and has a collection of highly biodiverse primary and semi-primary forests that contain many endemic species.3,4 Like many subtropical islands, the typical climate is long and wet summers, promoting conditions ideal for runoff pollution, increasing opportunities for transport of anthropogenic contaminants into soil, sediment, and surface water. In Okinawa, this potential runoff is problematic, as the groundwater aquifer was noted to be extremely permeable.5
Tracking sources and the fate of pollutants on islands can be challenging, due to the myriad of anthropogenic activities commonly present near water systems, and often times human biomonitoring is used as a tool for assessing region-specific pollutant burdens. Agriculture has long been a significant activity in Okinawan culture, with a diverse array of crops.6–8 Wastewater treatment plants and industrial effluents may also contribute to the presence of anthropogenic pollutants in Okinawa's more densely populated areas.9 However, perhaps one of the most interesting potential sources of pollution on Okinawa is the heavy United States (US) military presence, with at least 32 US military installations located on the island. To date, few studies have reported values for pollutants in the environment of Okinawa; those published have mostly focused on the presence of heavy metals and persistent organic pollutants, such as organochlorine chemicals/pesticides and polychlorinated biphenyls (PCBs).10–13 In these previous studies, there has been some indication that a relationship exists between the observed environmental concentrations of pollutants and the site's proximity to military installations, water treatment facilities, and/or agricultural areas;11,12,14 however, more studies are needed to better define these relationships.
Per- and polyfluoroalkyl substances (PFAS) are a ubiquitous class of thousands of anthropogenic chemicals characterized by high persistence and mobility in the environment and a myriad of adverse health effects associated with exposure/burden.15–17 While there are numerous pathways for PFAS to enter the environment, perhaps the most prevalent source is using aqueous film forming foams (AFFF), which are commonly employed firefighting suppressants at many military and aviation facilities.18,19 Okinawa is predicted to have elevated presence of PFAS due to the high number of military bases on the island. To date, the characterization of PFAS on Okinawa has been limited, and there has not been a study that has comprehensively examined the presence of PFAS across this vulnerable island.
The purpose of this study was to characterize the presence of 31 PFAS in surface water collected from 61 sites across the entire island employing isotope dilution and targeted high performance liquid chromatography-tandem mass spectrometry (HPLC-MS/MS). This surveillance effort across Okinawa Island aimed to help identify potential PFAS “hotspots” and provide a comparison between locations with varying anthropogenic activities. These data will also serve as important initial baseline concentrations that may be used to assist in prospective monitoring (e.g., post episodic weather events, such as typhoons) and will help raise awareness toward initiating efforts to study the impact of PFAS around the island.
2 Materials and methods
2.1 Sample collection and extraction
Surface water samples (n = 61) were collected in June 2023, from 46 coastal, 13 inland, and 2 groundwater sites, covering the entire island of Okinawa. Sampling locations (ESI Fig. S1;† coordinates and site notes are shown in ESI Table S2†) were strategically chosen based on geographical coverage of the island, which included sites near potential PFAS sources (i.e. military areas, industrial plants, and water treatment facilities) and sites suspected to be pristine (i.e. rural areas and national parks). Collections were achieved using 250 mL high density polyethylene (HDPE) bottles. Field blanks (n = 4) and quality control QC samples (n = 3) were also implemented. QC samples were prepared in the laboratory by transferring 250 mL of optima water into clean HDPE bottles which were subsequently spiked with 25 μL of a native PFAS standard solution containing the 31 PFAS targeted in this study. The concentration of native PFAS standards in solution were approximately 49 ng mL−1. Surface water samples and field blanks were transported from the field the same day and stored at 4 °C until extraction, which occurred within 1–3 days of collection. Surface water samples were extracted as previously described.20 In brief, Strata-XL-AW, weak anion exchange cartridges (Phenomenex), were preconditioned with 0.3% methanolic ammonium acetate, methanol, and acetic acid/ammonium acetate buffer solution prior to sample loading. Cartridges were washed with acetic acid/ammonium acetate buffer prior to elution with methanol and 0.3% methanolic ammonium hydroxide. Extracts were subsequently concentrated to 1 mL under nitrogen evaporation prior to HPLC-MS/MS analysis. For the detailed extraction protocol (including sample normalization and internal standard addition), see ESI.†
2.2 High performance liquid chromatography-tandem mass spectrometry (HPLC-MS/MS)
PFAS analyses were conducted using a Thermo Scientific Vanquish HPLC with a Phenomenex Gemini C18 column (100 mm × 2 mm; 3 μm particle size) coupled to a TSQ Quantis triple quadrupole mass spectrometer, operated with electrospray ionization in negative ion mode. Prior to analysis, the HPLC was fitted with a Vanquish PFAS replacement kit with PFAS-free plumbing and hardware to minimize PFAS background. Selected-Reaction Monitoring (SRM) transitions were used to detect and quantify PFAS, with the most intense transition used to quantify PFAS while the second transition was used to confirm identification (when applicable). This analytical workflow monitored 31 PFAS, including both the linear and branched isomers of PFOS (shown collectively here as ΣPFOS). PFAS abbreviations, scan parameters, and LC-MS/MS parameters (source, mobile phases, chromatographic gradient, and flow rate) can be found in ESI Tables S4–S6.†
2.3 Data analysis
Surface water extracts, method blanks, QC samples and calibrants were queued randomly in the mass spectrometric sequence. Peak integration was performed using Xcalibur v.4.1 software (Thermo Fisher Scientific). A linear regression model for each PFAS (isotope dilution) was built over 19 calibration levels ranging from 0.004 to 47.6 ng mL−1. All calibration curves generated for quantitation had an R2 value greater than 0.995. The resultant concentration of each PFAS was normalized to the mass of the extracted samples, providing results in ng PFAS/g water. ESI Table S4† lists each PFAS with the corresponding internal standard used for quantitation. Limits of detection (LOD) and limits of quantitation (LOQ) for each PFAS were curated visually for each extract using the signal-to-noise defined as 3× for LOD and 10× for LOQ. Extraction recovery, as a percentage, was calculated using the QC samples. PFAS for which the corresponding recovery percentages were not within an 80–120% range were excluded from further data discussion. PFAS for which the quality control samples had a percent relative standard deviation (% RSD) > 25% were also excluded from data discussion (all recovery % RSD values for PFAS discussed in this study are in ESI Table S7†). Field blanks were assessed for background contamination and the mean of any background for a given PFAS was subtracted from sample extracts before quantitation was performed. A geographic information system was used to spatially analyze the data and produce a predictive heat map (more details found in ESI†).
3 Results and discussion
Of the 31 PFAS targeted, 15 PFAS were detected and quantified in at least one of the 61 surface water samples (Table 1). The mean Σ15PFAS across the entire island was 16.3 ng L−1. The highest Σ15PFAS sampled at one site was 164.3 ng L−1. Of the 15 unique PFAS detected, there were seven perfluoroalkyl carboxylic acids (PFCAs), four perfluoralkyl sulfonic acids (PFSAs), two perfluorinated sulfonamides, and two fluorotelomers. The top three PFAS, by frequency detected (>LOD) and frequency quantified (>LOQ) were ΣPFOS, PFOA, and PFNA. Interestingly, five PFAS had mean concentrations greater than 4.0 ng L−1: PFHpA > PFHxS > ΣPFOS > PFPeA > FHxSA, with several having maximum concentrations greater than 20 ng L−1: PFHpA > ΣPFOS > PFOA > PFHxS > PFPeA. Of the four PFSAs detected, the longer-chain species had higher mean concentrations than the short-chain species.
Table 1 Summary statistics of the 15 PFAS monitored in surface water samples collected in and around Okinawa, Japanc
PFAS |
Frequency detection |
Frequency quantified |
Mean (ng L−1) |
Min (ng L−1) |
Max (ng L−1) |
Median (ng L−1) |
Represents the sum of the linear and branched isomers of PFOS.
Represents the total PFAS, a combined sum of all individual PFAS monitored for a given sample.
Values < LOQ were not included in ∑PFAS calculations. PFAS detected, defined as LOD < x < LOQ, were included in frequency detected summary statistics but were not assigned concentration values (and thus were not included in concentration summary statistics). Non-detects (ND) were not included in any sums or summary statistics.
|
PFPeA |
21% |
13% |
5.1 |
0.5 |
20.8 |
1.9 |
PFHxA |
43% |
28% |
3.1 |
0.4 |
19.4 |
0.8 |
PFHpA |
26% |
21% |
30.7 |
2.1 |
122.4 |
11.5 |
PFOA |
67% |
54% |
1.9 |
0.1 |
40.4 |
0.3 |
PFNA |
66% |
66% |
0.2 |
0.1 |
1.3 |
0.1 |
PFDA |
25% |
20% |
0.6 |
0.6 |
0.7 |
0.6 |
PFUdA |
8% |
8% |
0.3 |
0.2 |
0.3 |
0.2 |
PFBS |
11% |
8% |
2.0 |
0.4 |
4.6 |
1.9 |
PFPeS |
5% |
5% |
2.1 |
1.4 |
3.0 |
2.0 |
PFHxS |
18% |
15% |
8.8 |
1.5 |
26.3 |
2.5 |
ΣPFOSa |
100% |
95% |
5.9 |
0.1 |
73.1 |
1.5 |
FBSA |
5% |
5% |
2.4 |
1.9 |
2.9 |
2.5 |
FHxSA |
7% |
7% |
4.2 |
2.9 |
7.8 |
3.1 |
4:2 FTS |
31% |
3% |
0.1 |
0.1 |
0.1 |
0.1 |
8:2 FTS |
20% |
15% |
0.1 |
0.0 |
0.2 |
0.1 |
ΣPFASb |
NA |
NA |
16.3 |
0.0 |
164.3 |
2.1 |
A predictive heat map (Fig. 1), based on the measured Σ15PFAS values for 61 sampling locations, pinpoints areas with elevated Σ15PFAS levels, i.e., “hotspots”. These hotspots are locations that should elicit continued monitoring and/or mitigation and remediation efforts. Examining the heat map revealed an interesting hotspot along the southwestern coast of central Okinawa. This high Σ15PFAS area is near Kadena Airforce Base, and was dominated by the presence of ΣPFOS, PFHxS, PFHxA, and PFPeA, with the site with the highest overall Σ15PFAS in the study (164.3 ng L−1). This area has been shown previously to have elevated PFAS levels, ranging from 65–195 ng L−1 for PFOA, PFHxA, and PFOS, reported by Mitchell 2020
21 and Yukioka et al. 2020.14 These studies monitored seven and eight sites, respectively, in this area of Okinawa. For reference, ESI Fig. S2† shows the eight river and groundwater samples collected by Yukioka et al. 2020
14 (blue pins) on the same map of the 61 sites collected in this study (green pins). Moving beyond this PFAS-laden site, Fig. 1 revealed at least three additional hotspots. Along the east-central coast of Okinawa, near Camp Hansen/Schwab Marine Corps Bases, a similar PFAS profile to Kadena Airforce Base was detected, but with two additional PFAS noted: PFOA and FHxSA. The additional hotspots are located in the northern region of Okinawa. Although concentrations of Σ15PFAS measured here were similar to those reported for the central/southern regions, the PFAS fingerprint observed in the northern sites were substantially different. Surface water collected in the northern region was dominated by PFHpA, which was not detected, or detected at very low levels, in the other PFAS hotspots around the island. The Σ15PFAS for the major hotspots around the island were similar, ranging from 80–164 ng L−1, however; the discovery of unique relationships between the PFAS profiles and different geographical regions of the island suggests there may be multiple sources responsible for Okinawa's PFAS contamination.
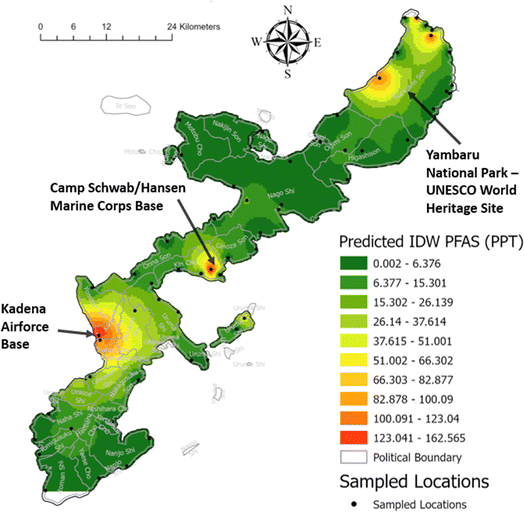 |
| Fig. 1 Predictive heat map of total PFAS levels across Okinawa, Japan, based on the measured concentrations in 61 surface water samples. Dots on the map represent the sampling locations, with two US military bases and the UNESCO World Heritage Site highlighted. | |
The nine most PFAS-laden sites (Σ15PFAS levels 23.3–164.3 ng L−1) are shown in Fig. 2, with the boundaries (red zones) of several military areas on Okinawa. In the figure, the stacked bar graph represents individual PFAS measured at each site by concentration. This figure highlights unique PFAS fingerprints for the different regions of Okinawa. The sites surrounding military areas appear to have similar PFAS compositions, containing PFHxA, PFHxS, PFHpA, PFOA, and ΣPFOS; all of which are consistent with findings from AFFF-impacted areas.19,22 While PFHpA has been detected in groundwater impacted by AFFF usage at US military bases,22 the surface water samples collected around the military bases in Okinawa had very low levels of PFHpA. However, PFHpA appears to dominate the PFAS profile of sampling locations in the northern region, which is predominantly rural and agriculturally used land. Moreover, two of the sites with the highest measured Σ15PFAS concentrations (126.4 and 91.8 ng L−1) were collected from inland water sources within the Yambaru National Park, a UNESCO World Natural Heritage Site. Though sources of PFHpA are commonly linked to stain and grease proof coatings, food packaging materials, textiles, and AFFF-degradation products,23–25 some studies have revealed an increased presence of PFHpA in rural areas. Studies by Simcik et al. 2005
27 and Scott et al. 2006
26 both reported higher concentrations of PFHpA in rural areas compared to more urban locations. Additionally, it has been shown that precipitation can have PFHpA, thus atmospheric precipitation is believed to be the main source of PFHpA in rural areas.26–28 The elevated presence of PFHpA is of unique concern to farmers and/or stakeholders of more rural areas, as a study by Mattsson et al. 2015
29 determined a correlation between PFHpA exposure to rural farmers and coronary heart disease.29 PFHpA was the only PFAS (of the eight analyzed) that had a correlation in their study. At the three most northern sites in Fig. 2 (Σ15PFAS = 91.8, 95.6, and 126.4 ng L−1) PFHpA dominated the PFAS profiles measuring 85.5 ng L−1 (93%), 94.8 ng L−1 (99%), and 122.3 ng L−1 (97%) PFHpA, respectively.
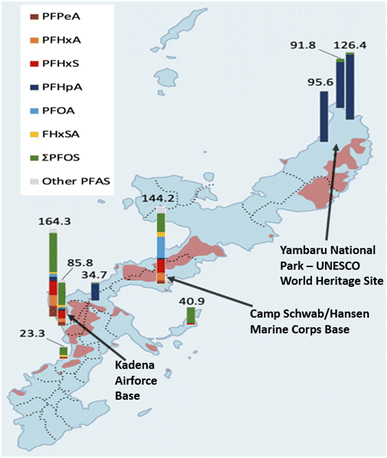 |
| Fig. 2 Map of Okinawa, Japan showing the nine sampling sites with the highest measured total PFAS levels on the Island. Each location is represented by a stacked bar graph showing the most dominant PFAS contributing to the total PFAS concentration for each site (ranging from 23.3–164.3). Above each bar graph, the total PFAS concentration (ng L−1) for each site is listed. Red boundaries indicate US military bases/training facilities, with two US military bases and the UNESCO World Heritage Site highlighted. Map made with Google My Maps. | |
4 Conclusion
There has been a rise in concern amongst the people of Okinawa over the presence of US military bases and their corresponding pollution. To the author's knowledge, this is the first comprehensive island-wide examination of the presence of PFAS across Okinawa Island, Japan. In total, 15 unique species were detected throughout various regions of the island, in both coastal and inland waters. In future studies, nontargeted analyses could be employed to identify a wider breadth of PFAS contamination in Okinawa surface water. Characterization of the PFAS profiles at each site revealed regional changes suggesting the potential of multiple PFAS sources impacting the island. Specifically, unique PFAS profiles were noted in the surface water collected near military bases, whereas water collected in the northern agricultural region (in the UNESCO national park), was surprisingly dominated by the presence of PFHpA. This study is the first step toward alerting local stakeholders of the wider PFAS threat across the island, highlighting sites where additional testing is required and where mitigation and remediation strategies should be considered.
Data availability
The data supporting this article have been included as part of the ESI.†
Author contributions
Camden G. Camacho: conceptualization, methodology, investigation, data curation, writing- original draft, visualization, project administration. Kaylie Anne Costa: conceptualization, investigation, writing – review and editing. Shannon McMahon: resources, writing – review and editing. Jeffrey Jolly: resources, writing – review and editing. Timothy Ravasi: resources, writing – review and editing. Joe Aufmuth: formal analysis, writing – review and editing, visualization. John A. Bowden: resources, methodology, writing – review and editing, funding acquisition.
Conflicts of interest
There are no conflicts to declare.
Acknowledgements
Camden Camacho received support from the University of Florida (UF Graduate Student Council Travel Grant). Timothy Ravasi, Shannon McMahon, and Jeffrey Jolly were supported by the Okinawa Institute of Science and Technology (OIST). The authors declare that they do not possess any known financial interests or personal associations that may have influenced the findings presented in this paper. The authors would also like to express their gratitude to everyone at the Okinawa Institute of Science and Technology (OIST) who helped make this project possible. Additionally, we would like to thank Dr Saki Harii and Dr Fred Sinniger at the University of Ryukyus Tropical Biosphere Research Center for their help and hospitality.
References
- K. Sawada, K. Yamahira and T. Kuriwada, Interpopulation variation in female color-type frequency of Ischnura senegalensis in Okinawa Island, Japan (Odonata: Coenagrionidae), Odonatologica, 2014, 43, 3–4 Search PubMed.
- M. Poulain, Exceptional longevity in Okinawa: A plea for in-depth validation, Demogr Res, 2011, 25, 245–284 CrossRef.
- A. Nanami, M. Nishihira, T. Suzuki and H. Yokochi, Species-specific habitat distribution of coral reef fish assemblages in relation to habitat characteristics in an Okinawan coral reef, Environ Biol Fishes, 2005, 72(1), 55–65 CrossRef.
- D. M. Olson and E. Dinerstein, The global 200: A representation approach to conserving the earth's most biologically valuable ecoregions, Conserv. Biol., 1998, 12, 502–515 CrossRef.
- J. Yasumoto, M. Abe and T. Nakano, Regional Groundwater Flow Model in Ryukyu Limestone Aquifer, J. Jpn. Soc. Civ. Eng., 2015, 71(4), I_217–I_222 Search PubMed.
- S. Lai, Cooperative Militarization: Agricultural Reform and the U.S. Occupation of Okinawa, Pac Hist Rev., 2022, 91(1), 33–65 CrossRef.
- S. Mambu, S. Sugihara, T. Kawame, T. Nishigaki, K. Toyota and N. Miyamaru,
et al., Effect of green manure application on soil enzyme activity and nutrient dynamics in a sugarcane field of Kitadaito, Okinawa, Japan, Jpn. Agric. Res. Q., 2018, 52(4), 315–324 CrossRef CAS.
- Z. Sano and H. Iwahori, Regional variation in pathogenicity of Meloidogyne incognitapopulations on sweetpotato in Kyushu Okinawa, Japan, J. Nematol., 2005, 35(1), 1–12 CrossRef PubMed.
- H. Kawahata, H. Ohta, M. Inoue and A. Suzuki, Endocrine disrupter nonylphenol and bisphenol A contamination in Okinawa and Ishigaki Islands, Japan - Within coral reefs and adjacent river mouths, Chemosphere, 2004, 55(11), 1519–1527 CrossRef CAS.
- S. T. Imo, M. A. Sheikh, K. Sawano, H. Fujimura and T. Oomori, Distribution and possible impacts of toxic organic pollutants on coral reef ecosystems around Okinawa Island, Japan, Pac. Sci., 2008, 317–326 CrossRef CAS.
- Y. Mukai, A. Goto, Y. Tashiro, S. Tanabe and T. Kunisue, Coastal biomonitoring survey on persistent organic pollutants using oysters (Saccostrea mordax) from Okinawa, Japan: Geographical distribution and polystyrene foam as a potential source of hexabromocyclododecanes, Sci. Total Environ., 2020, 739, 140049 CrossRef CAS PubMed.
- Y. Tashiro, A. Goto, T. Kunisue and S. Tanabe, Contamination of habu (Protobothrops flavoviridis) in Okinawa, Japan by persistent organochlorine chemicals, Environ. Sci. Pollut. Res., 2021, 28(1), 1018–1028 CrossRef CAS PubMed.
- M. A. Sheikh, K. Tsuha, X. Wang, K. Sawano, S. T. Imo and T. Oomori, Spatial and seasonal behaviour of organotin compounds in protected subtropical estuarine ecosystems in Okinawa, Japan, Int. J. Environ. Anal. Chem., 2007, 87(12), 847–861 CrossRef.
- S. Yukioka, S. Tanaka, Y. Suzuki, S. Echigo, A. Kärrman and S. Fujii, A profile analysis with suspect screening of per- and polyfluoroalkyl substances (PFASs) in firefighting foam impacted waters in Okinawa, Japan, Water Res., 2020, 184, 116207 CrossRef CAS PubMed.
- M. Kotthoff, J. Müller, H. Jürling, M. Schlummer and D. Fiedler, Perfluoroalkyl and polyfluoroalkyl substances in consumer products, Environ. Sci. Pollut. Res., 2015, 22(19), 14546–14559 CrossRef CAS PubMed.
- A. East, R. H. Anderson and C. J. Salice, Per- and Polyfluoroalkyl Substances (PFAS) in Surface Water Near US Air Force Bases: Prioritizing Individual Chemicals and Mixtures for Toxicity Testing and Risk Assessment, Environ. Toxicol. Chem., 2021, 40(3), 871–882 CrossRef PubMed.
- Z. Zhao, Z. Xie, A. Möller, R. Sturm, J. Tang and G. Zhang,
et al., Distribution and long-range transport of polyfluoroalkyl substances in the Arctic, Atlantic Ocean and Antarctic coast, Environ. Pollut., 2012, 170, 71–77 CrossRef CAS PubMed.
- K. A. Barzen-Hanson, S. C. Roberts, S. Choyke, K. Oetjen, A. McAlees and N. Riddell,
et al., Discovery of 40 Classes of Per- and Polyfluoroalkyl Substances in Historical Aqueous Film-Forming Foams (AFFFs) and AFFF-Impacted Groundwater, Environ. Sci. Technol., 2017, 51(4), 2047–2057 CrossRef CAS PubMed.
- Å. Høisæter, A. Pfaff and G. D. Breedveld, Leaching and transport of PFAS from aqueous film-forming foam (AFFF) in the unsaturated soil at a firefighting training facility under cold climatic conditions, J. Contam. Hydrol., 2019, 222, 112–122 CrossRef.
- E. K. Griffin, J. Aristizabal-Henao, A. Timshina, H. L. Ditz, C. G. Camacho and B. F. da Silva,
et al., Assessment of per- and polyfluoroalkyl substances (PFAS) in the Indian River Lagoon and Atlantic coast of Brevard County, FL, reveals distinct spatial clusters, Chemosphere, 2022, 301, 134478 CrossRef CAS.
- J. Mitchell, PFAS contamination from US military facilities in mainland Japan and Okinawa, Asia-Pac. J.-Jpn. Focus, 2020, 18(16), 1–21 Search PubMed.
- W. J. Backe, T. C. Day and J. A. Field, Zwitterionic, cationic, and anionic fluorinated chemicals in aqueous film forming foam formulations and groundwater from U.S. military bases by nonaqueous large-volume injection HPLC-MS/MS, Environ. Sci. Technol., 2013, 47(10), 5226–5234 CrossRef CAS PubMed.
- F. Wang, K. Shih, R. Ma and Li X. yan, Influence of cations on the partition behavior of perfluoroheptanoate (PFHpA) and perfluorohexanesulfonate (PFHxS) on wastewater sludge, Chemosphere, 2015, 131, 178–183 CrossRef CAS PubMed.
- L. M. Weatherly, H. L. Shane, E. Lukomska, R. Baur and S. E. Anderson, Systemic toxicity induced by topical application of perfluoroheptanoic acid (PFHpA), perfluorohexanoic acid (PFHxA), and perfluoropentanoic acid (PFPeA) in a murine model, Food Chem. Toxicol., 2023, 171, 113515 CrossRef CAS PubMed.
- K. C. Harding-Marjanovic, E. F. Houtz, S. Yi, J. A. Field, D. L. Sedlak and L. Alvarez-Cohen, Aerobic Biotransformation of Fluorotelomer Thioether Amido Sulfonate (Lodyne) in AFFF-Amended Microcosms, Environ. Sci. Technol., 2015, 49(13), 7666–7674 CrossRef CAS PubMed.
- B. F. Scott, C. Spencer, S. A. Mabury and D. C. G. Muir, Poly and perfluorinated carboxylates in north American precipitation, Environ. Sci. Technol., 2006, 40(23), 7167–7174 CrossRef CAS.
- M. F. Simcik and K. J. Dorweiler, Ratio of perfluorochemical concentrations as a tracer of atmospheric deposition to surface waters, Environ. Sci. Technol., 2005, 39(22), 8678–8683 CrossRef CAS.
- S. Chen, X. C. Jiao, N. Gai, X. J. Li, X. C. Wang and G. H. Lu,
et al., Perfluorinated compounds in soil, surface water, and groundwater from rural areas in eastern China, Environ. Pollut., 2016, 211, 124–131 CrossRef CAS PubMed.
- K. Mattsson, A. Rignell-Hydbom, S. Holmberg, A. Thelin, B. A. G. Jönsson and C. H. Lindh,
et al., Levels of perfluoroalkyl substances and risk of coronary heart disease: Findings from a population-based longitudinal study, Environ. Res., 2015, 142, 148–154 CrossRef CAS PubMed.
|
This journal is © The Royal Society of Chemistry 2025 |
Click here to see how this site uses Cookies. View our privacy policy here.