Lithium recovery from U.S. oil and gas produced waters: resource quality and siting considerations†
Received
20th May 2024
, Accepted 29th December 2024
First published on 7th January 2025
Abstract
To meet the growing demand for lithium sustainably, secondary resources must be explored. Among nontraditional resource alternatives, brines co-produced from oil and gas (O&G) operations are of particular interest owing to their abundance and considerable lithium concentrations. Whereas previous work has highlighted potential O&G brines of interest for lithium extraction, the criteria to distinguish optimal from suboptimal O&G produced waters are still unclear. In the following, we provide perspectives on assessing the feasibility and challenges of produced waters from key U.S. formations as lithium resources based on their water chemistry, production rates, and geographic placement. Specifically, we clarify the impact of production rates on the estimated lithium resources and how it may aggrandize evaluations. We assess how key secondary cation concentrations and ratios complicate downstream separation, and evaluate the role of the geographic coexistence of lithium resources and lithium consumers (i.e., the manufacturing sector). Among the U.S. O&G formations evaluated herein, the Marcellus shale emerges as an attractive formation for lithium extraction, with an estimated annual lithium metal output of 930 metric tons and lower secondary cation concentrations. The potential feasibility of brines from the Marcellus formation is enabled by its reduced need for downstream separation and purification, as well as its proximity to major lithium end-user facilities (i.e., battery manufacturers). Overall, we provide an initial set of criteria to help evaluate O&G formations for their potential to serve as lithium resources and provide an assessment of the lithium resources in key U.S. O&G plays.
Water impact
Oil and gas (O&G) produced waters, consisting of abundant and lithium-rich wastewaters generated in oilfields, have been identified as an alternative source of lithium. Herein, we clarify factors that must be assessed to determine the suitability of O&G brines for lithium extraction.
|
Introduction
Achieving a low-carbon economy will require unprecedented quantities of lithium metal, one of the most critical components for at-scale manufacturing of clean energy technologies and infrastructure.1,2 Current lithium resources, however, are unable to meet growing demand, with supply deficits expected as early as 2030.3 Moreover, extraction of conventional resources creates environmental stresses that are counterproductive to current sustainability and decarbonization efforts. Lithium extraction from salar brines, for example, alters the hydrogeological conditions of neighboring water systems and consumes ∼500 tons of water for every 1 ton of lithium strip produced.4–6 Hard rock lithium mining (e.g., spodumene, petalite, lepidolite) is also costly to the environment, with high energy requirements, water use, and pollutants released during processing.7 Alternative resources are thus needed to strengthen lithium supply chains while minimizing the environmental footprint of current sourcing operations.
An emerging alternative source of lithium is co-produced brines from oil and gas (O&G) operations, characterized by high production rates8 coupled with notable concentrations of lithium.9,10 Although total lithium resources have been evaluated for O&G brines,11 our understanding of how the overall water chemistry impacts recoverability is still limited. High water production rates may inflate the total resource prediction of lithium-poor formations, and the presence of high total dissolved solids (TDS) content and co-occurrence of competing cations challenge the separation of Li from the brine waste. Moreover, existing discussions on lithium sourcing from produced waters do not include the geographic location of current and upcoming lithium markets and how this alternative resource may be integrated into the existing lithium infrastructure.
In this perspective, we evaluate key U.S. O&G water-producing sites for lithium production based on their water chemistry and their geographic potential to serve as lithium suppliers for end-use industries. Parsed water chemistry data from the USGS National Produced Waters Geochemical Database,12,13 and water production data obtained from public records14–16 and EPA estimates17 are used to assess how lithium concentrations, water production rates, and the overall water chemistry of O&G formations impact total resource estimation and recoverability. We evaluate these criteria within the context of 4 key U.S. O&G formations: Bakken, Wolfcamp, Marcellus, and Smackover. The first three consist of unconventional resources with prolific O&G operations and high water production outputs, and the latter is a conventional play with known high lithium concentration brines.11,18,19 Data for the Salton Sea geothermal brine formation is also presented here for comparative purposes owing to their known Li potential. Moreover, geographic and environmental considerations are also discussed in this perspective, including the regional distribution of domestic lithium markets and potential proximity to lithium-producing O&G brines. Overall, this work provides a set of initial criteria to evaluate prospective resources and potential challenges arising from lithium recovery from produced waters.
Impact of water production and chemistry on resource evaluation
Lithium resources, quantified based on production rates and chemistry of the O&G brines, provide an overview of the production potential of subsurface systems (Fig. 1a). Total lithium production potential was estimated using average lithium concentrations obtained from the USGS produced waters database12 and the water production rates reported for the formations of interest by state agencies. Since produced water for the Wolfcamp shale was not available, we approximated its production using EPA production rate estimates and EIA well count data.17 The USGS database was also parsed to minimize bias by removing entries in the database with no identifiers (e.g., latitude and longitude, or API information), wells designated as injectors, and averaging duplicate entries into a single entry, similar to previous work.13
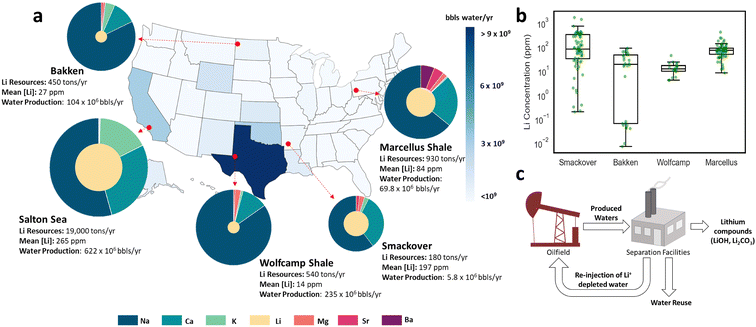 |
| Fig. 1 (a) Map highlighting annual water production from O&G fields by state, as well as the elemental concentrations and total lithium resources for key O&G plays. Total annual lithium resources, in metric tons of lithium metal, are predicted using the USGS geochemical database12 and produced water volumes, estimated from the EPA17 and publicly available data.14–16 The U.S. map color scale reflects the total statewide O&G water production in 2017, and exemplifies how known regional high water production rates do not match expected Li potential of specific formations of interest.8 The pie charts for select locations showcase the major elemental makeup of the brines (outer circle); the lithium inner circle and the major cations outer circle are sized to reflect the lithium content, multiplied by a factor of 100, relative to major cations content. The Salton Sea formation, albeit a geothermal brine field rather than an O&G field, was added here for comparative purposes owing to their notable Li concentrations. (b) Corresponding lithium concentrations for key O&G plays. (c) Simplified workflow for lithium exploration and recovery from produced waters. | |
Our analyses indicate that the Marcellus shale is expected to produce ∼930 tons of lithium metal annually, the Wolfcamp and Bakken shales possess estimated annual production potentials of ∼540 and 450 tons, respectively, whereas the Smackover is expected to produce 180 tons of lithium annually.
Although O&G brines originating from unconventional formations are expected to produce more lithium on a volume basis than conventional formations, massive water production rates in some unconventional plays may inflate the attractiveness of their low-grade lithium brines. For example, despite similar total lithium resources, the average lithium concentration in Wolfcamp brines ([Li] ∼14 ppm) is only half that of the Bakken shale ([Li] ∼27 ppm), and ∼1/6th that of the Marcellus shale ([Li] ∼84 ppm) (Fig. 1a and b). Such a trend underscores a key paradox in lithium recovery from produced waters: the total lithium resources do not always correlate to the lithium grade of brines and, thus, their suitability for downstream processing (Fig. 1c). In other words, for low-grade brines, greater volumes of O&G produced waters must be processed to output the same amount of lithium compound. As we extend our analysis from formation-specific data to regional estimates, production rates may further exaggerate lithium resources valuation. For instance, high water production rates in Texas (∼140 times greater than that of the median U.S. state, Fig. 1a) suggests that the Permian basin outputs considerable quantities of lithium. A closer look, however, reveals that the top water-producing formations in the Permian basin are particularly lithium-poor, with a lithium content ranging between 1 to ∼30 ppm, falling considerably short of those in the Williston and Appalachian basins (Fig. 2). Therefore, while unconventional formations with high water production rates should be assessed for resource estimates, lithium-rich brines such as the ones found in the Marcellus shale are more attractive for efficient lithium recovery.
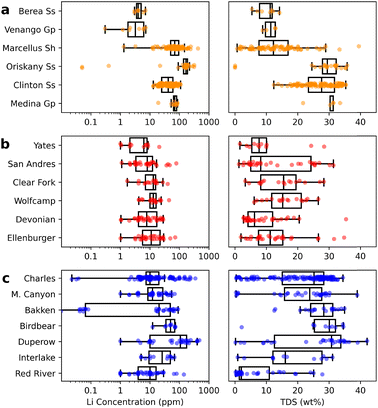 |
| Fig. 2 Lithium concentration and total dissolved solid (TDS) content for key formations in the (a) Appalachian basin, (b) Permian basin, and (c) Williston basin. | |
Moreover, we note that while the data at the formation level provides initial screening of prospective lithium resources, significant compositional variations within a formation imply that siting decisions should consider stratigraphic characteristics. Lithium concentrations in brines produced from different depths of the Bakken and Smackover formations, for example, vary by several orders of magnitude (Fig. 1b). Thus, although play-based estimates offer an assessment of regional lithium resources, determining a specific site for lithium recovery requires a more localized understanding of the geochemistry within the targeted formation.
Co-occurrence of secondary cations
Although several technologies, both commercially available as well as those under development, have shown promising potential for lithium extraction, the coexistence of secondary cations poses a significant challenge, hindering efficient lithium-ion enrichment and separation. For example, adsorbents, exchange resins, solvent-based extraction, membrane filtration and electrochemical approaches (e.g., ion pumping, electrodialysis) all exhibit limited Li extraction efficiency under high salinity conditions, where large concentrations of competing cations reduce selectivity towards Li ions.9,20–22 Thus, brines with lower concentrations of secondary cations, and lower concentration ratios of those ions to Li are desirable to ensure high-yield, high-purity output lithium compounds with lower separation processing requirements.
Among the O&G brines evaluated here, the Marcellus shale and Smackover formations present the lowest major metals-to-lithium and divalent ions-to Li ratios, with values that are similar to, or slightly greater than, those of the Salton Sea geothermal brines (Fig. 1a and 3). In contrast, Bakken and Wolfcamp produced waters present the least amenable compositions for downstream separations, with elevated major metals-to-lithium ratios, Mg concentrations that are 40 to 100 times greater than Li concentrations, and Ca concentrations that more than 400 times greater than Li concentrations (Fig. 1a and 3). Lower major metal-to-Li ratios observed in the Marcellus and Smackover formations, thus, make those formations more attractive options for lithium production from a technical feasibility standpoint.
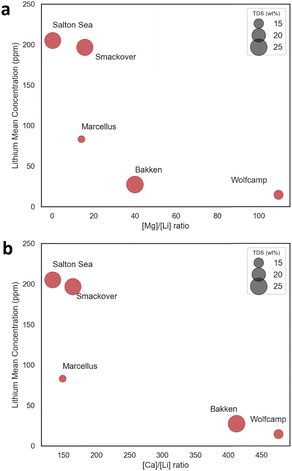 |
| Fig. 3 Average lithium concentration vs. (a) Mg/Li and (b) [Ca]/[Li] ratios for key O&G plays. The formation bubble size corresponds to the TDS values for each formation. | |
Moreover, although not evaluated here, other key technical parameters that should be accounted for include the removal of contaminants (e.g., organics, radioactive elements) prior to Li processing, the physical and chemical properties of the O&G brine, and any required adjustments to ensure that the incoming brine is compatible with surface processing units.
Geographic considerations
The colocation of natural, human, and infrastructure resources improves the economics of lithium extraction by reducing the costs related to materials transport and workforce availability. Current and upcoming investments in battery manufacturing, the largest end-use of lithium metal in the U.S.,23 offer a proxy to evaluate geographic proximity between lithium end-users and potential O&G brine resources. For instance, among O&G lithium resources, the Marcellus shale presents one of the most favorable locations for ongoing and upcoming lithium end-use manufacturing, whereas the Bakken play lacks similar geographic proximity to key facilities. Battery manufacturing efforts, evaluated based on state-level sector jobs for the year 2022,24 are heavily concentrated in Midwestern and Southern states (Fig. 4). Announced investments in battery production facilities25 suggest that the region will continue to grow their production capacity in upcoming years (Fig. 4). Thus, transportation costs and integration into existing supply chains are expected to be more accessible for lithium production from Marcellus O&G brines than for Bakken lithium operations.
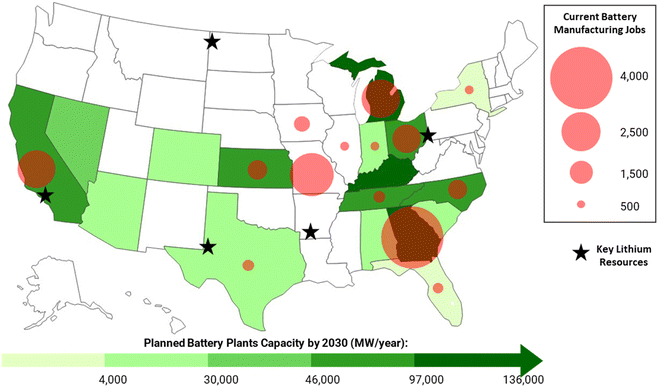 |
| Fig. 4 Predicted battery plant output by 2030, according to the Argonne National Lab.22 Private battery manufacturing jobs for the year of 2022, on average, according to the Bureau of Labor Statistics.21 | |
Environmental considerations
Whereas transforming produced waters into a valuable resource presents a possible revenue-generating path for waste management, the environmental impact of lithium extraction must be examined. First, brines produced from O&G operations are considered waste material since their high salinity, radioactivity, heavy metals content, and presence of organic compounds make them unsuitable for immediate reuse. As a result, produced waters are typically reinjected into nonproducing formations that increase the risk of fault activation and induced microseismicity.26 Lithium extraction processes typically involve the pre- or co-extraction of major cations and, thus, processing produced waters for lithium recovery may reduce the brine salinity and offer a pathway toward water reuse (e.g., irrigation, industrial use, etc.). Moreover, recovering lithium from a waste product would lead to lower demands for lithium mining from virgin sites, reducing the need for energy and water consumption and the disruption of local ecosystems.6 We note, however, that lithium extraction from produced brines is not without its own set of potential environmental challenges. Principally, since produced waters host lower concentrations of lithium than traditional resources (i.e., hard rocks and salars), extracting lithium from O&G brines may require greater volumes of reagents and energy input to achieve high-purity lithium products. Life cycle assessments are therefore required to fully elucidate the environmental impact of lithium extraction from any produced waters.
Concluding remarks
The perspectives above provide a set of aspects that should be considered when choosing a prospective site for lithium production from O&G brines. Namely, for an O&G water-producing formation to be a compelling lithium resource, they should possess substantial lithium contents, low concentrations of competing cations, and moderate TDS to enable cost- and energy-efficient separations downstream. Moreover, whereas predicted lithium productions are useful to define the resource potential, care should be taken to ensure that resource potentials, embellished commonly by high water production levels, are distinguished from the recoverable Li. Among the O&G formations described here, for instance, the Marcellus shale possesses one of the most attractive lithium resource potentials, producing on average low salinity brines with considerable lithium content. Additionally, its geographic proximity to battery manufacturing infrastructure, the main lithium metal end-user in the U.S., places the Marcellus play in a prime position to be incorporated into existing and upcoming value chains. While the other O&G plays also demonstrated a potential for substantial lithium production, enrichment in unsought cations in their produced brines makes them less attractive for lithium exploration.
Lastly, it is important to note that siting evaluation is not a stagnant process. Updated and more localized geochemical data may reveal formations and locations that have not been considered yet. Advances in separation and purification technologies may improve the economics of recovering lithium, converting locations that are less attractive into viable operations. Environmental considerations and policies are also ever-evolving and may either incentivize lithium operations from produced waters or restrict such activities. A continuous alignment of these factors is therefore needed to promote the sustainable development of lithium from produced waters.
Data availability
The data underlying this study are available in the published article and its ESI.†
Conflicts of interest
There are no conflicts to declare.
Acknowledgements
This material is based upon work supported by the U.S. Department of Energy's Office of Energy Efficiency and Renewable Energy (EERE) Advanced Materials & Manufacturing Technologies Office under grant DE-EE0009440 and by the National Science Foundation's Division of Chemical, Bioengineering, Environmental, and Transport Systems under grant 2145374.
References
- P. Greim, A. A. Solomon and C. Breyer, Assessment of lithium criticality in the global energy transition and addressing policy gaps in transportation, Nat. Commun., 2020, 11(1), 1–11 CrossRef PubMed.
- D. Bogdanov,
et al., Radical transformation pathway towards sustainable electricity via evolutionary steps, Nat. Commun., 2019, 10(1), 1–16 CrossRef PubMed.
- S. Han, M. Zhenghao, L. Meilin, Y. Xiaohui and W. Xiaoxue, Global supply sustainability assessment of critical metals for clean energy technology, Resour. Policy, 2023, 85, 103994 CrossRef.
- B. C. Gibb, The rise and rise of lithium, Nat. Chem., 2021, 13(2), 107–109 CrossRef CAS PubMed.
- X. Yang,
et al., Emerging Research Needs for Characterizing the Risks of Global Lithium Pollution under Carbon Neutrality Strategies, Environ. Sci. Technol., 2023, 57, 5103–5106 CrossRef CAS PubMed.
- V. Flexer, C. F. Baspineiro and C. I. Galli, Lithium recovery from brines: A vital raw material for green energies with a potential environmental impact in its mining and processing, Sci. Total Environ., 2018, 639, 1188–1204 CrossRef CAS PubMed.
- S. Jiang,
et al., Environmental impacts of lithium production showing the importance of primary data of upstream process in life-cycle assessment, J. Environ. Manage., 2020, 262, 110253 CrossRef CAS PubMed.
-
J. Veil, U.S. Produced Water Volumes and Management Practices in 2017, Report Prepared for the Ground Water Research and Education Foundation, 2020 Search PubMed.
- A. Kumar, H. Fukuda, T. A. Hatton and J. Lienhard, Lithium Recovery from Oil and Gas Produced Water: A Need for a Growing Energy Industry, ACS Energy Lett., 2019, 4(6), 1471–1474 CrossRef CAS.
- Q. Liu,
et al., Lithium recovery from oil and gas produced water: Opportunities, challenges, and future outlook, J. Water Process Eng., 2023, 55, 104148 CrossRef.
- E. J. M. Dugamin,
et al., Groundwater in sedimentary basins as potential lithium resource: a global prospective study, Sci. Rep., 2021, 11, 21091 CrossRef PubMed.
-
M. S. Blondes, K. D. Gans, M. A. Engle, Y. K. Kharaka and M. E. Reidy, U.S. Geological Survey National Produced Waters Geochemical Database v2.3 [Data set], U.S. Geological Survey, 2019 Search PubMed.
- B. R. Scanlon,
et al., Can we beneficially reuse produced water from oil and gas extraction in the U.S.?, Sci. Total Environ., 2020, 717, 137085 CrossRef CAS PubMed.
-
North Dakota Mineral Resources, Historical monthly Bakken oil production statistics, 2019 Search PubMed.
-
Pennsylvania Department of Environmental Protection, Oil and Gas Reports, 2019 Search PubMed.
-
Arkansas Oil and Gas Commission, Monthly and Annual Production Graphs, 2019 Search PubMed.
-
United States Environmental Protection Agency, Technical Development Document for the Effluent Limitations Guidelines and Standards for the Oil and Gas Extraction Point Source Category, 2016 Search PubMed.
- R. Darvari,
et al., Controls on lithium content of oilfield waters in Texas and neighboring states (USA), J. Geochem. Explor., 2024, 257, 107363 CrossRef CAS.
- W. T. Stringfellow and P. F. Dobson, Technology for the Recovery of Lithium from Geothermal Brines, Energies, 2021, 14, 6805 CrossRef CAS.
- M. A. Alkhadra,
et al., Electrochemical Methods for Water Purification, Ion Separations, and Energy Conversion, Chem. Rev., 2022, 122, 13547–13635 CrossRef CAS PubMed.
- M. L. Vera, W. R. Torres, C. I. Galli, A. Chagnes and V. Flexer, Environmental impact of direct lithium extraction from brines, Nat. Rev. Earth Environ., 2023, 4(3), 149–165 CrossRef CAS.
- L. Wu,
et al., Lithium recovery using electrochemical technologies: Advances and challenges, Water Res., 2022, 21, 118822 CrossRef PubMed.
-
U.S. Geological Survey, Mineral Commodity Summaries 2023, 2023 Search PubMed.
-
U.S. Bureau of Labor Statisticso, 2022 Quarterly Census of Employment and Wages, 2022 Search PubMed.
-
D. Gohlke, Y. Zhou, X. Wu and C. Courtney, Assessment of Light-Duty Plug-in Electric Vehicles in the United States, Argonne National Lab, 2022 Search PubMed.
-
Pennsylvania Department of Environmental Protection, Oil and Gas Reports, 2019 Search PubMed.
|
This journal is © The Royal Society of Chemistry 2025 |
Click here to see how this site uses Cookies. View our privacy policy here.