DOI:
10.1039/D5PY00144G
(Paper)
Polym. Chem., 2025,
16, 1813-1821
The origin of the thermally stable white-light emission property of POSS-conjugated polymer hybrid films†
Received
14th February 2025
, Accepted 14th March 2025
First published on 21st March 2025
Abstract
We have previously reported thermally stable white-light luminescence from polymer hybrid films consisting of tetraphenylethene (TPE)-tethered polyhedral oligomeric silsesquioxane (POSS) and poly(1,4-phenylenevinylene) (PPV). We observed that the intensity ratios between the dual emission bands from the POSS and the conjugated polymer, and thus the color balance, were maintained even in the higher-temperature region. In this paper, the origin of the thermally stable dual-emission properties of these white-light-emitting hybrid films is investigated using a series of POSS derivatives with different tethered luminophores and various conjugated polymer matrices. We obtained homogeneous hybrid films and revealed that one of the key factors for the expression of dual-emission properties is the relationship between the photoluminescence quantum yields of the energy donors (fillers) (ΦD) and acceptors (polymers) (ΦA). When the emission quantum yield of either the donor or acceptor molecules is high, only an emission band originating from the molecule with the higher emission quantum yield can be observed. Dual emission from both the donor and acceptor is detectable only when the ΦD is as high as the ΦA or slightly higher than the ΦA. Based on these findings, we have demonstrated a logical design for dual-emissive materials. We also found that the affinity between POSS and polymers is responsible for maintaining the emission color balance at high temperatures. It was observed that POSS substituted with bulky groups can hybridize with conjugated polymer chains, and that the thermal behavior of the polymer is dominated by the POSS. As a result, the thermal stability can be enhanced. We revealed the origin of the thermally stable white-light luminesce properties of POSS hybrids based on two aspects.
Introduction
π-Conjugated polymers have attracted attention as a platform for constructing modern optoelectronic materials owing to their unique material, electrical and optical properties.1–4 However, the durability of organic materials including conjugated polymers is generally lower than that of inorganics. Therefore, it is still strongly required to reinforce the durability and extend the lifetimes of organic devices. To meet these demands, we have focused on the formation of hybrid materials with polyhedral oligomeric silsesquioxane (POSS).5 POSS is an organic–inorganic hybrid molecule consisting of a cubic silica core and organic substituents at eight vertices.6–14 By connecting organic dyes to POSS, various functional optical materials and sensors can be created.15–26 In addition, the thermal stability of the dyes can be enhanced.27–29 Furthermore, by selecting appropriate substituents, it is possible to tune various physical properties, such as miscibility in polymer matrices. In particular, the thermal stability of polymer matrices can be enhanced by simple mixing with POSS because of the suppression of molecular motions by POSS.30–38 Some POSS derivatives can even show high miscibility with π-conjugated polymers, and the resulting polymer hybrids can act as thermally stable luminescent materials.39–43 Therefore, we have regarded POSS derivatives as a versatile element-block, which is a minimum functional unit containing a heteroatom, for creating designable hybrid materials through facile preparation methods.44–49
White-light-emitting materials are versatile for a variety of applications, such as surface light source illuminators that cast few shadows and lightweight car lamps in motor vehicles.50 There are several strategies for preparing white-light-luminescent materials based on dye-encapsulated micelles51–54 or vesicles,55–57 hybrids containing various dyes,58–62 fixation into gels,63–66 and arrangement with metal–organic frameworks.67–72 To induce white-light emission, it is essential to mix two or more luminophores in a single material and preserve their luminescence properties in the condensed state of the material. In these multiple-dye-doped materials, critical phase separation occasionally occurs during manufacturing or at the elevated temperatures caused by device operation. Therefore, it is still challenging to enhance the thermal durability and suppress the degradation of white-light luminescence at high temperature.
In a previous report, we demonstrated that a hybrid film composed of 10 wt% poly[2-methoxy-5-(2-ethylhexyloxy)-1,4-phenylenevinylene] (MEH-PPV) and 90 wt% tetraphenylethene-tethered POSS (TPE-POSS) exhibited white-light luminescence consisting of dual-emission properties originating from the emission band of TPE-POSS and that of MEH-PPV.43 In particular, the hybrid film retained white-light luminescence even at high temperature without unexpected changes in the intensity ratios of the emission bands. Although we observed these intriguing thermo-optical properties of POSS hybrid materials, the origin of these unique properties was unclear. To achieve control of their optical properties and the further improvement of their thermal stability, it is essential to comprehend the mechanism and explore further combinations of POSS derivatives and conjugated polymers.
In this report, we prepared hybrid films containing various combinations of a series of luminophore-tethered POSS fillers and conjugated polymer matrices. From optical measurements, we clarified the rule for obtaining dual-emission to produce white-light emission. Based on this information, we demonstrated how to predict optical characteristics based on the optical data of the components. Moreover, from thermal analyses, we obtained the structure–property relationship for explaining the thermal stability of white-light emission. Based on the optical and thermal studies, we propose the origin of the thermally stable white-light emission of POSS-conjugated polymer hybrids.
Results and discussion
Synthesis and sample preparation
The hybrid films were prepared according to the following procedure. Fig. 1 shows the structures of the POSS derivatives (TPE-POSS, DPFL-POSS, DPA-POSS, TPA-POSS, BT-POSS, Btz-POSS, anth-POSS, and DA-POSS) used in this study. We also prepared N-propylmethanimine-modified models for comparison and polymers as a matrix (Fig. S1 and S2†). Each POSS was obtained in high isolated yield through the condensation reaction of octakis(3-aminopropyl) POSS hydrochloride (Amino-POSS) and the corresponding aromatic aldehyde.73,74 The model compounds were prepared using the same condensation reaction between propylamine and the respective aromatic aldehydes. The luminescent π-conjugated polymer MEH-PPV was synthesized according to the Glich route.43,75,76 Poly(9,9-dihexylfluorene-2,7-diyl) (PF) and poly[2,1,3-benzothiadiazole-4,7-diyl(3,3′-didodecyl[2,2′-bithiophene]-5,5′-diyl)] (PDA) were synthesized by cross-coupling polymerization. Poly(3-hexylthiophene-2,5-diyl) (P3HT) was prepared according to a method in the literature.77 The molecular weights were determined using size-exclusion chromatography with polystyrene standards and chloroform as an eluent. All polymers had sufficient molecular weights to show good film formability and sufficient length to exclude molecular-weight effects on the electronic properties of the polymers. The structures of all new compounds were confirmed using 1H, 13C and 29Si NMR spectroscopy (Charts S1–S41†), high-resolution mass spectrometry and elemental analyses.
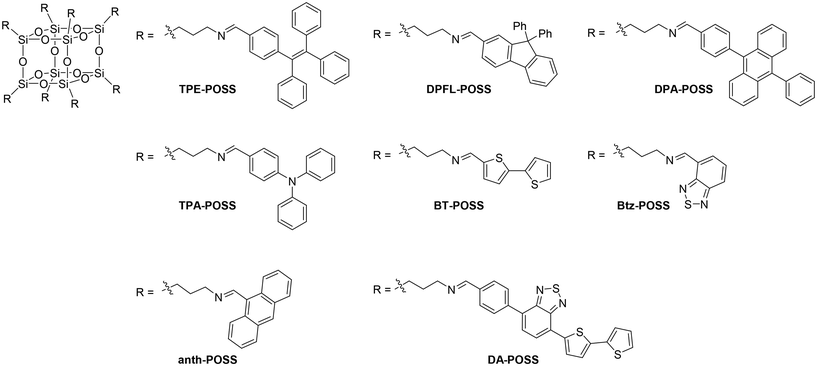 |
| Fig. 1 Chemical structures of POSS derivatives. | |
Hybrid films were fabricated using a spin-coating method (1000 rpm, 30 s) on quartz substrates (0.9 cm × 5 cm) from the chloroform solutions. The concentration of polymers in chloroform was fixed at 1.0 mg per 300 μL. The concentration of POSS derivatives or model compounds in chloroform was also 1.0 mg per 300 μL for preparing neat films. The optical properties of the POSS derivatives and polymers are summarized in Tables S1 and S2.† The POSS derivatives and polymers are expected to act as energy donors and acceptors, respectively, except for DA-POSS and PF. For each combination, hybrid films containing 0, 50, 90, and 100 wt% POSS derivatives were prepared. We obtained homogenous thin films, indicating that POSS-conjugated polymer hybrids can be obtained from the various combinations, and used them for further measurements (Fig. S3†). Although the luminophores are connected by imine bonds, which can potentially be hydrolyzed, the hybrid films can be handled and stored stably in air. Owing to the radially arranged hydrophobic luminophores on POSS, degradation could be suppressed by the restricted accessibility of water molecules.
Evaluation of dual-emission properties
For almost all the combinations, the hybrid films showed brighter emission with higher POSS content (Table 1, Fig. S4 and S5†). Some combinations exhibited dual emission at 90 wt% POSS content, while others showed only POSS emission or polymer emission. MEH-PPV showed dual emission in the presence of TPA-POSS, whereas only polymer emission was observed from the BT-POSS hybrids. P3HT showed dual emission with BT-POSS, although only POSS emission was detected from the TPA-POSS hybrids. These differences seemed to result from the differences between the quantum yields of the components. To examine the relationships between the dual emission properties and Φfilm, the optical properties of the film samples for each combination were investigated (Fig. 2). The data can be classified into three areas: I (ΦPOSS > Φpolymer), II (ΦPOSS ≅ Φpolymer) and III (ΦPOSS < Φpolymer). In area I, the hybrid films showed only the emission band of POSS even when they contained 90 wt% POSS. According to our previous report, POSS should form domains in polymer matrices.43 As a result, energy transfer should be disturbed. In area III, only the emission band attributable to the polymer was observed regardless of the POSS content. This is because the luminescence ability of the energy donor should be too weak to exhibit its own emission after energy transfer. In area II, it should be noted that dual emission from both the POSS and the polymer was detected when the POSS content was over 50 wt% (PDA or P3HT hybrid films) or 90 wt% (MEH-PPV hybrid films). It is suggested that, similarly to the samples categorized in area I, the energy transfer from POSS should be suppressed, and the emission from POSS can be detected.
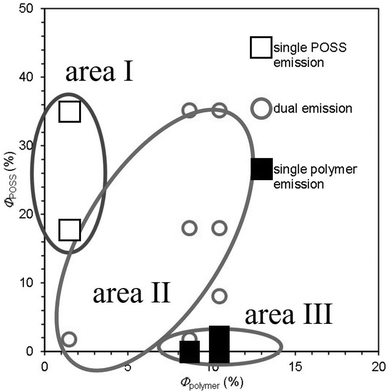 |
| Fig. 2 Plots of the emission efficiencies of hybrid films containing POSSs. The horizontal and vertical axes represent Φfilm,polymer and Φfilm,POSS, respectively. The samples in area II showed dual emission from both the POSS and polymer. The samples in areas I and III showed emission from only the POSS or only the polymer, respectively. | |
Table 1 Luminescence behaviors of the combinations of POSSs and polymersa
|
TPE-POSS
|
TPA-POSS
|
DPA-POSS
|
BT-POSS
|
anth-POSS
|
DPFL-POSS
|
Btz-POSS
|
Circles indicate combinations that showed dual emission from both the POSS and polymer. White and black squares indicate combinations that showed the emission from only the POSS or only the polymer, respectively. Various contents of POSS (0, 50, 90, and 100 wt%) were loaded for testing each combination.
The test was not conducted.
|
MEH-PPV
|
○ |
○ |
○ |
■ |
■ |
■ |
■ |
PDA
|
○ |
○ |
—b |
○ |
—b |
—b |
■ |
P3HT
|
□ |
□ |
—b |
○ |
—b |
—b |
—b |
To investigate the influence of POSS on luminescent properties, hybrid films were prepared with model compounds and other polymer matrices (Fig. S6–S8, Tables S3 and S4†). Using the same method as that for preparing POSS hybrids, film samples containing 0, 50, 90, and 100 wt% of each model compound were fabricated. Interestingly, the films showed dual emission or only polymer emission. In light of the fact that the hybrid films containing POSS tended to exhibit dual emission or only POSS emission, these results can also be explained by the formation of domain structures, similar to our previous results.43 In the case of the hybrids including model compounds, the luminophores and polymer chains can interact. As a result, efficient energy transfer should proceed in the film, resulting in emission annihilation of a single luminophore molecule. In contrast, the bulky structure of the POSS core makes it likely that each luminophore will be isolated from the polymer chains at the molecular level. Moreover, POSS forms domain structures with sizes of several tens of nanometers in the polymer matrices.43 Consequently, in the presence of POSS, energy transfer should be restricted in the films, and dual emission can be induced.
Next, we also investigated reverse energy transfer between PF and DA-POSS, which should act as an energy donor and acceptor, respectively. The values of ΦPF,film and ΦDA-POSS,film are 13% and 2%, respectively, and this combination is found in area II in Fig. 2, meaning that white-light dual emission can be expected. To confirm the validity of this estimation, the hybrid films were prepared using the same method as previous examples with variable content of DA-POSS (0, 10, 25, 50, 75, 90, and 100 wt%), and their optical properties were investigated. Accordingly, as seen in Fig. 3, white-light luminescence was detected from the film containing 10 wt% of DA-POSS. This result indicates that dual emission can be realized if the balance of Φdonor,film and Φacceptor,film is optimized.
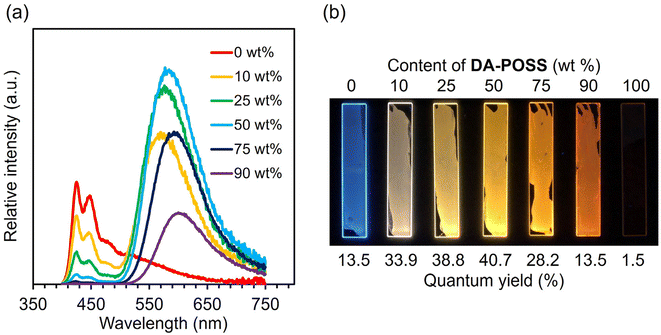 |
| Fig. 3 (a) Relative photoluminescence spectra of PF and DA-POSS hybrid films excited at λabs,PF (385 nm). Numbers in the legend indicate the content ratios of DA-POSS. (b) Photos of PF and DA-POSS hybrid films irradiated by a UV lamp (365 nm). | |
Influence of thermal stability of color balance
First, to investigate the contribution of each material to the thermal stability of the color balance of the white-light emission reported in the previous study, we evaluated the effect of thermal stability by replacing the individual components in the hybrid film based on TPE-POSS and MEH-PPV. Instead of TPE-POSS or MEH-PPV, TPA-POSS or PDA was used, respectively. The emission spectrum with changing temperature was monitored, and the emission intensities from the POSS and polymer moieties were plotted versus temperature. As shown in Fig. 4, the hybrid film with 90 wt% TPA-POSS and 10 wt% MEH-PPV exhibited low thermal stability in terms of color balance. The emission bands decreased individually with heating. Conversely, the film containing 90 wt% TPE-POSS and 10 wt% PDA showed the desired thermally stable emission behavior (Fig. 5). The emission of TPE-POSS and PDA attenuated in a concerted fashion in the film, similar to the film consisting of TPE-POSS and MEH-PPV in the previous report (Fig. S9†).43 The partial emission peak shift of PDA around 676 nm with increasing temperature should be attributed to the intramolecular conformational change of the polymer main-chain. As the intermolecular main-chain interactions are suppressed by bulky POSS, the spectral changes should be small and hardly affect the color balance of the white-light emission. In the hybrid film with the model compound, the concerted decrease in the emission intensity with increasing temperature was not observed (Fig. S10 and S11†), similarly to in our previous report.43 These results imply that TPE-POSS could play an important role in maintaining color balance at high temperatures.
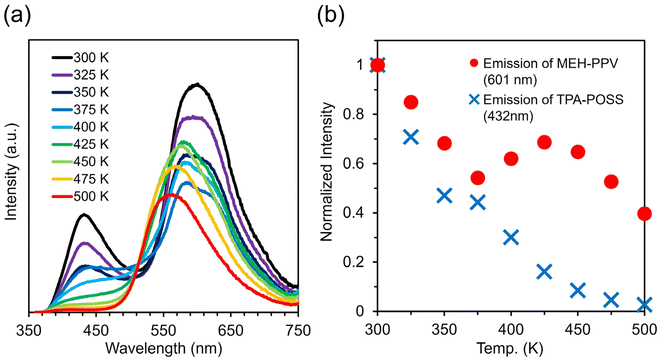 |
| Fig. 4 (a) Variable-temperature emission spectra with excitation at 350 nm and (b) intensity ratios at 432 nm for TPA-POSS and 601 nm for MEH-PPV in the hybrid film containing 10 wt% MEH-PPV and 90 wt% TPA-POSS. | |
 |
| Fig. 5 (a) Variable-temperature emission spectra with excitation at 330 nm and (b) intensity ratios at 492 nm for TPE-POSS and 676 nm for PDA in the hybrid film containing 10 wt% PDA and 90 wt% TPE-POSS. | |
TPE-POSS has several distinctive characteristics, namely, a bulky steric structure, aggregation-induced emission properties, and affinity with π surfaces.43 To elucidate which properties were important to the thermally stable color balance, other POSSs with one of the same properties as TPE-POSS were hybridized with MEH-PPV, and their optical and thermal properties were evaluated. DPFL-POSS, which has a bulky steric structure similar to that of TPE-POSS, and BT-POSS, which is expected to have a higher affinity with π surfaces than TPE-POSS, were applied. The color balance of the hybrid films with changing temperature was monitored to clarify the POSS substituent effect on thermal stability.
Fig. S12† shows the emission spectra and peak-top decay plots at various temperatures for hybrid films containing 90 wt% BT-POSS and 10 wt% MEH-PPV. The emission of MEH-PPV decreased with increasing temperature until 375 K and then increased. This behavior was similar to the emission decay of MEH-PPV in the neat film (Fig. S13†) and TPA-POSS hybrid film (Fig. 4). In these films, the emission intensities decreased or remained the same until 375 K, then increased until 425 K, and then decreased again. This behavior indicates that MEH-PPV retains its bulk characteristics, and that MEH-PPV and POSSs exhibit their optical thermal properties individually. Hence, these materials do not preserve the color balance at high temperature.
In the case of the hybrid film containing 90 wt% DPFL-POSS and 10 wt% MEH-PPV, due to the almost complete lack of luminescence from DPFL-POSS, we were unable to plot the peak tops of DPFL-POSS emission (Fig. S14†). In this film, the emission of MEH-PPV decreased monotonically. This decrease is similar to that of the TPE-POSS hybrid film, as shown in Fig. S9.† The emission of MEH-PPV in the TPE-POSS hybrid film decreased more drastically than that in the DPFL-POSS hybrid film. This is explained by its energy donation ability. As TPE-POSS is more luminescent than DPFL-POSS, MEH-PPV has a relatively higher emission intensity at 300 K in the TPE-POSS hybrid film than in the DPFL-POSS hybrid film. As a result, the difference between its intensity at 300 K and 500 K is larger compared to that of the TPE-POSS hybrid. Based on the similarity between the TPE-POSS and DPFL-POSS hybrid film, the bulky steric substituents of the POSS should be regarded as the key to the thermally stable color balance. In the hybrid film, MEH-PPV should be isolated by the surrounding excess bulky POSS, and its thus, its bulk characteristics as a polymer are no longer observed. Therefore, it is likely that the molecular motion of MEH-PPV should be restricted by the POSS. At high temperatures, it is proposed that the emission intensities of MEH-PPV and TPE-POSS could decrease concertedly when molecular motions occur in the POSS units due to heating.
To gather information on the isolation effect on the polymer chains, differential scanning calorimetry (DSC) measurements were performed. The films were fabricated by the drop-casting method. Fig. S15† shows the DSC curves of the MEH-PPV neat film and hybrid films containing 10 wt% MEH-PPV and 90 wt% POSS derivatives. The shape of the endothermic peak in the DSC curve, which is assigned to the glass transition, was distinctive. Compared with the MEH-PPV neat film, the hybrid films with non-bulky POSSs such as TPA-POSS and BT-POSS showed sharp peaks, indicating that the relaxation time of MEH-PPV should be little affected by the POSS. In contrast, relatively broad endothermic peaks were observed for the hybrid films containing the bulky POSS derivatives such as TPE-POSS and DPFL-POSS, meaning that the distribution of the relaxation time should be widened due to hybridization. The fact that the difference in the relaxation times of the polymers is dispersed means that the properties of the polymer chains are not averaged, in other words, the MEH-PPV chains could be well separated in these hybrid films.
To estimate the affinity between a polymer and filler, the Flory–Huggins interaction parameters (χ) are often used.78–87 As has been conducted in several studies, the interaction parameters were calculated from the contact angles (Tables S5–S7 and Fig. S16†).81–83 To qualitatively discuss the affinity between the POSS derivatives and MEH-PPV, we estimated the interaction parameter using this indirect method. The trend in the interaction parameters reasonably explains the relationship between the affinity and bulkiness of the substituents on the POSS. The derivatives having bulky substituents, including TPE-POSS, DPFL-POSS and DPA-POSS, show relatively smaller χMEH-PPV,POSS values, while those with less-bulky substituents, including TPA-POSS, BT-POSS, anth-POSS and Btz-POSS, exhibit larger χMEH-PPV,POSS values. Based on these results, it is suggested that bulky substituents show a positive effect in improving affinity toward polymer chains, leading to the isolation of the polymer chains (Fig. 6). In this state, the molecular motions of the polymer chains are dominated by POSS, and therefore, the emissions of the polymer chains can be annihilated correspondingly with the decrease in the emission intensity of the POSS by heating. Moreover, it should be noted that the POSS derivatives having bulky substituents are favorable for controlling the thermo-optical properties of the polymer by hybridization.
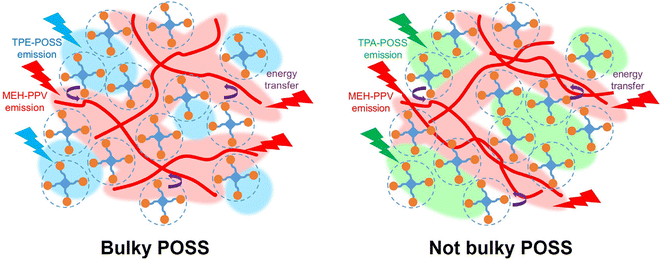 |
| Fig. 6 Schematic models of hybrid films containing a bulky-group-substituted POSS (such as TPE-POSS) and a less-bulky-group-substituted POSS (such as TPA-POSS). | |
In summary, the structure–property relationship for the thermo-optical properties in POSS hybrid materials is explained as follows. As shown in Fig. 6, the bulky substituents on the POSS derivative promote the hybridization of the POSS with polymer chains. In this state, the polymer chains are isolated from each other, and the properties of the bulk materials should no longer be observed. In particular, the degree of molecular motion is determined by the surrounding POSS molecules. Therefore, the thermal decay of the emission intensity from the polymer chains proceeds monotonically, corresponding with that of the POSS, and the ratio between their emission intensity is maintained during the luminescence decay of the overall hybrid material. Thus, the hybrid film maintains the color balance of the white emission even at high temperatures.
Conclusion
In this study, the key factors for the unique phenomenon of thermally stable white-light luminescence were revealed using two approaches: the origin of the dual emission and that of the thermally stable color balance. The balance between the luminescence quantum yields of the POSS and the polymer, which can act as a donor and an acceptor in the energy transfer system, respectively, has been found to be important. When one is much larger than another, emission is only observed from one component. If both values are similar, dual emission from both the POSS and polymer components can be detected. In other words, the dual-emission properties of the hybrid materials can be predicted based on the relative magnitudes of ΦPOSS,film and Φpolymer,film. We also found that the affinity between the POSS and polymers is the key to maintaining the emission color balance at high temperatures. DSC measurements revealed that POSS derivatives containing bulky substituents have good affinity with polymers. When such POSS derivatives are hybridized with a polymer, the thermal behavior of the polymer is dominated by the POSS. Polymers hybridized with a bulky POSS cannot behave as bulk materials and are influenced by the surrounding POSS. The emission from the polymer decreases monotonically upon heating, and the behavior corresponds to the decay of the POSS. As a result, the hybrid film, as a single material, decreases in emission without change in its emission color. Flory–Huggins interaction parameters (χ) are available for estimating the affinity of several POSSs toward MEH-PPV. This means that by using χPOSS,polymer, it is possible to predict whether a hybrid material will maintain its color balance when heated. Our findings may have potential applications in developing thermally stable light emitters as well as for tailoring the properties of materials based on hybrids by designing POSS structures.
Data availability
The data supporting this article have been included as part of the ESI.†
Conflicts of interest
There are no conflicts to declare.
Acknowledgements
This work was partially supported by the ENEOS Tonengeneral Research/Development Encouragement & Scholarship Foundation, Japan (for M. G.) and JSPS KAKENHI Grant Numbers 24K01570 (for K. T.).
References
- J. H. Burroughes, D. D. C. Bradley, A. R. Brown, R. N. Marks, K. Mackay, R. H. Friend, P. L. Burns and A. B. Holmes, Nature, 1990, 347, 539–541 CAS.
- H. Sirringhaus, P. J. Brown, R. H. Friend, M. M. Nielsen, K. Bechgaard, B. M. W. Langeveld-Voss, A. J. H. Spiering, R. A. J. Janssen, E. W. Meijer, P. Herwig and D. M. de Leeuw, Nature, 1999, 401, 685–688 CrossRef CAS.
- K. Mahesh, S. Karpagam and F. Goubard, eXPRESS Polym. Lett., 2018, 12, 238–255 CAS.
- A. C. Grimsdale, K. Leok Chan, R. E. Martin, P. G. Jokisz and A. B. Holmes, Chem. Rev., 2009, 109, 897–1091 CAS.
- K. Tanaka and Y. Chujo, J. Mater. Chem., 2012, 22, 1733–1746 CAS.
- D. B. Cordes, P. D. Lickiss and F. Rataboul, Chem. Rev., 2010, 110, 2081–2173 CAS.
- J. J. Schwab and J. D. Lichtenhan, Appl. Organomet. Chem., 1998, 12, 707–713 CAS.
- F. K. Wang, X. Lu and C. He, J. Mater. Chem., 2011, 21, 2775–2782 CAS.
- K. Naka and Y. Irie, Polym. Int., 2017, 66, 187–194 CAS.
- Y. Kaneko, Chem. Rec., 2023, 23, e202200291 CAS.
- Y. Liu, K. Koizumi, N. Takeda, M. Unno and A. Ouali, Inorg. Chem., 2022, 61, 1495–1503 CAS.
- R. Kajiya, H. Wada, K. Kuroda and A. Shimojima, Chem. Lett., 2020, 49, 327–329 CAS.
- H. Sakai, T.-M. Yung, T. Mure, N. Kurono, S. Fujii, Y. Nakamura, T. Hayakawa, M.-C. Li and T. Hirai, JACS Au, 2023, 3, 2698–2702 CAS.
- S. Morimoto, H. Imoto and K. Naka, Chem. Commun., 2017, 53, 9273–9276 CAS.
- M. Gon, K. Tanaka and Y. Chujo, Chem. – Asian J., 2022, 17, e202200144 CrossRef CAS PubMed.
- H. Narikiyo, D. Iizuka, M. Gon, K. Tanaka and Y. Chujo, Eur. J. Inorg. Chem., 2025, 28, e202400731 CrossRef CAS.
- H. Narikiyo, M. Gon, K. Tanaka and Y. Chujo, Asian J. Org. Chem., 2025, 14, e202400508 CrossRef CAS.
- H. Narikiyo, T. Kato, M. Gon, K. Tanaka and Y. Chujo, ChemPhotoChem, 2025, 9, e202400273 CrossRef CAS.
- H. Narikiyo, M. Gon, K. Tanaka and Y. Chujo, Bull. Chem. Soc. Jpn., 2024, 97, uoae066 CrossRef.
- H. Narikiyo, M. Gon, K. Tanaka and Y. Chujo, Polym. J., 2024, 56, 661–666 CrossRef CAS.
- D. Iizuka, M. Gon, K. Tanaka and Y. Chujo, Chem. Commun., 2022, 58, 12184–12187 RSC.
- D. Iizuka, M. Gon, K. Tanaka and Y. Chujo, Bull. Chem. Soc. Jpn., 2022, 95, 743–747 CrossRef CAS.
- R. Nakamura, H. Narikiyo, M. Gon, K. Tanaka and Y. Chujo, Mater. Chem. Front., 2019, 3, 2690–2695 RSC.
- H. Narikiyo, M. Gon, K. Tanaka and Y. Chujo, Mater. Chem. Front., 2018, 2, 1449–1455 CAS.
- H. Narikiyo, T. Kakuta, H. Matsuyama, M. Gon, K. Tanaka and Y. Chujo, Bioorg. Med. Chem., 2017, 25, 3431–3436 CAS.
- T. Kakuta, K. Tanaka and Y. Chujo, J. Mater. Chem. C, 2015, 3, 12539–12545 CAS.
- K. Sato, M. Gon, K. Tanaka and Y. Chujo, Bull. Chem. Soc. Jpn., 2024, 97, uoae040 Search PubMed.
- M. Gon, K. Sato, K. Tanaka and Y. Chujo, RSC Adv., 2016, 6, 78652–78660 CAS.
- K. Suenaga, K. Tanaka and Y. Chujo, Chem. – Eur. J., 2017, 23, 1409–1414 CAS.
- E. Ayandele, B. Sarkar and P. Alexandridis, Nanomaterials, 2012, 2, 445–475 CAS.
- I. Blanco and F. A. Bottino, Polym. Compos., 2013, 34, 225–232 CAS.
- K. Tanaka, S. Adachi and Y. Chujo, J. Polym. Sci., Part A: Polym. Chem., 2010, 48, 5712–5717 CAS.
- J.-H. Jeon, K. Tanaka and Y. Chujo, J. Polym. Sci., Part A: Polym. Chem., 2013, 51, 3583–3589 CAS.
- H. Kozuka, K. Tanaka and Y. Chujo, Eur. Polym. J., 2022, 175, 111360 CAS.
- K. Ueda, K. Tanaka and Y. Chujo, Polym. J., 2020, 52, 523–528 CAS.
- K. Ueda, K. Tanaka and Y. Chujo, Bull. Chem. Soc. Jpn., 2017, 90, 205–209 CAS.
- K. Ueda, K. Tanaka and Y. Chujo, Polymers, 2018, 10, 1332 Search PubMed.
- K. Tanaka, H. Kozuka, K. Ueda, J.-H. Jeon and Y. Chujo, Mater. Lett., 2017, 203, 62–67 CAS.
- K. Ueda, K. Tanaka and Y. Chujo, Polym. J., 2016, 48, 1133–1139 CrossRef CAS.
- K. Ueda, K. Tanaka and Y. Chujo, Polymers, 2019, 11, 44 Search PubMed.
- M. Gon, K. Sato, K. Kato, K. Tanaka and Y. Chujo, Mater. Chem. Front., 2019, 3, 314–320 CAS.
- M. Gon, K. Kato, K. Tanaka and Y. Chujo, Mater. Chem. Front., 2019, 3, 1174–1180 CAS.
- M. Gon, S. Saotome, K. Tanaka and Y. Chujo, ACS Appl. Mater. Interfaces, 2021, 13, 12483–12490 CAS.
- Y. Chujo and K. Tanaka, Bull. Chem. Soc. Jpn., 2015, 88, 633–643 CAS.
- M. Gon, K. Tanaka and Y. Chujo, Bull. Chem. Soc. Jpn., 2017, 90, 463–474 CAS.
- M. Gon, K. Tanaka and Y. Chujo, Polym. J., 2018, 50, 109–126 CAS.
- K. Tanaka and Y. Chujo, Polym. J., 2020, 52, 555–566 CAS.
- M. Gon, S. Ito, K. Tanaka and Y. Chujo, Bull. Chem. Soc. Jpn., 2021, 94, 2290–2301 CAS.
- K. Tanaka and Y. Chujo, Polym. J., 2023, 55, 353–364 CAS.
- E. Fresta and R. D. Costa, Adv. Funct. Mater., 2020, 30, 1908176 CAS.
- P. Pallavi, B. Sk, P. Ahir and A. Patra, Chem. – Eur. J., 2018, 24, 1151–1158 CAS.
- X. Zhang, D. Görl and F. Würthner, Chem. Commun., 2013, 49, 8178–8180 CAS.
- R. Wang, J. Peng, F. Qiu and Y. Yang, Chem. Commun., 2011, 47, 2787–2789 CAS.
- M. Bälter, S. Li, M. Morimoto, S. Tang, J. Hernando, G. Guirado, M. Irie, F. M. Raymo and J. Andréasson, Chem. Sci., 2016, 7, 5867–5871 RSC.
- X. Zhang, S. Rehm, M. M. Safont-Sempere and F. Würthner, Nat. Chem., 2009, 1, 623–629 CAS.
- J. Huang, Y. Yu, L. Wang, X. Wang, Z. Gu and S. Zhang, ACS Appl. Mater. Interfaces, 2017, 9, 29030–29037 CAS.
- P. Xing, Z. Zhao, A. Hao and Y. Zhao, Chem. Commun., 2016, 52, 1246–1249 RSC.
- H.-T. Feng, X. Zheng, X. Gu, M. Chen, J. W. Y. Lam, X. Huang and B. Z. Tang, Chem. Mater., 2018, 30, 1285–1290 CAS.
- X.-L. Ni, S. Chen, Y. Yang and Z. Tao, J. Am. Chem. Soc., 2016, 138, 6177–6183 CAS.
- T. Ono and Y. Hisaeda, J. Mater. Chem. C, 2019, 7, 2829–2842 CAS.
- W. Yuan, L. Shu, J. Xu, C. Hua and J. Huang, ACS Macro Lett., 2025, 14, 51–56 CAS.
- X. Li, L. Liu, L. Jia, Z. Lian, J. He, S. Guo, Y. Wang, X. Chen and H. Jiang, Nat. Commun., 2025, 16, 467 CAS.
- D. Podder, S. K. Nandi, S. Sasmal and D. Haldar, Langmuir, 2019, 35, 6453–6459 CAS.
- X. Cao, H. Lan, Z. Li, Y. Mao, L. Chen, Y. Wu and T. Yi, Phys. Chem. Chem. Phys., 2015, 17, 32297–32303 CAS.
- P. Bairi, B. Roy, P. Chakraborty and A. K. Nandi, ACS Appl. Mater. Interfaces, 2013, 5, 5478–5485 CAS.
- C. Vijayakumar, V. K. Praveen and A. Ajayaghosh, Adv. Mater., 2009, 21, 2059–2063 CAS.
- X.-Y. Liu, K. Xing, Y. Li, C.-K. Tsung and J. Li, J. Am. Chem. Soc., 2019, 141, 14807–14813 CAS.
- Z. Wang, C.-Y. Zhu, J.-T. Mo, P.-Y. Fu, Y.-W. Zhao, S.-Y. Yin, J.-J. Jiang, M. Pan and C.-Y. Su, Angew. Chem., Int. Ed., 2019, 58, 9752–9757 CAS.
- Y.-P. Xia, C.-X. Wang, L.-C. An, D.-S. Zhang, T.-L. Hu, J. Xu, Z. Chang and X.-H. Bu, Inorg. Chem. Front., 2018, 5, 2868–2874 CAS.
- C.-Y. Sun, X.-L. Wang, X. Zhang, C. Qin, P. Li, Z.-M. Su, D.-X. Zhu, G.-G. Shan, K.-Z. Shao, H. Wu and J. Li, Nat. Commun., 2013, 4, 2717 Search PubMed.
- W. Newsome, S. Ayad, J. Cordova, E. W. Reinheimer, A. D. Campiglia, J. K. Harper, K. Hanson and F. J. Uribe-Romo, J. Am. Chem. Soc., 2019, 141, 11298–11303 CAS.
- Q. Yang, W. Wang, Y. Yang, P. Li, X. Yang, F. Bai and B. Zou, Nat. Commun., 2025, 16, 696 CAS.
- H. Imoto, S. Wada and K. Naka, Chem. Lett., 2016, 45, 1256–1258 CrossRef CAS.
- M. Janeta, Ł. John, J. Ejfler, T. Lis and S. Szafert, Dalton Trans., 2016, 45, 12312–12321 RSC.
- H.-L. Chow, K.-F. Lin and D.-C. Wang, J. Polym. Sci., Part B: Polym. Phys., 2006, 44, 62–69 CAS.
- H.-L. Chou, K.-F. Lin and D.-C. Wang, J. Polym. Res., 2006, 13, 79–84 CAS.
- R. S. Loewe, S. M. Khersonsky and R. D. McCullough, Adv. Mater., 1999, 11, 250–253 CAS.
- D. R. Kozub, K. Vakhshouri, L. M. Orme, C. Wang, A. Hexemer and E. D. Gomez, Macromolecules, 2011, 44, 5722–5726 CAS.
- S. A. Dowland, M. Salvador, J. D. Perea, N. Gasparini, S. Langner, S. Rajoelson, H. H. Ramanitra, B. D. Lindner, A. Osvet, C. J. Brabec, R. C. Hiorns and H.-J. Egelhaaf, ACS Appl. Mater. Interfaces, 2017, 9, 10971–10982 CrossRef CAS.
- P. Westacott, N. D. Treat, J. Martin, J. H. Bannock, J. C. de Mello, M. Chabinyc, A. B. Sieval, J. J. Michels and N. Stingelin, J. Mater. Chem. A, 2017, 5, 2689–2700 RSC.
- J. Y. Kim, Macromolecules, 2019, 52, 4317–4328 CrossRef CAS.
- J.-H. Kim, A. Gadisa, C. Schaefer, H. Yao, B. R. Gautam, N. Balar, M. Ghasemi, I. Constantinou, F. So, B. T. O'Connor, K. Gundogdu, J. Hou and H. Ade, J. Mater. Chem. A, 2017, 5, 13176–13188 CAS.
- S. Nilsson, A. Bernasik, A. Budkowski and E. Moons, Macromolecules, 2007, 40, 8291–8301 CAS.
- L. Ye, H. Hu, M. Ghasemi, T. Wang, B. A. Collins, J.-H. Kim, K. Jiang, J. H. Carpenter, H. Li, Z. Li, T. McAfee, J. Zhao, X. Chen, J. L. Y. Lai, T. Ma, J.-L. Bredas, H. Yan and H. Ade, Nat. Mater., 2018, 17, 253–260 CAS.
- E. L. Hynes, J. T. Cabral, A. J. Parnell, P. Gutfreund, R. J. L. Welbourn, A. D. F. Dunbar, D. Môn and A. M. Higgins, Commun. Phys., 2019, 2, 112 Search PubMed.
- K. Milczewska, A. Voelkel and J. Jeczalik, Macromol. Symp., 2003, 194, 305–312 CAS.
- K. Milczewska and A. Voelkel, J. Appl. Polym. Sci., 2008, 107, 2877–2882 CAS.
|
This journal is © The Royal Society of Chemistry 2025 |
Click here to see how this site uses Cookies. View our privacy policy here.