DOI:
10.1039/D4RA04301D
(Paper)
RSC Adv., 2025,
15, 1713-1720
Full-component extract of Crithmum maritimum and its effect on epidermal regeneration
Received
12th June 2024
, Accepted 1st October 2024
First published on 20th January 2025
Abstract
As a well-known aromatic herb rich in various bioactive molecules, the extract of Crithmum maritimum is widely used in cosmetics. However, the extraction process for Crithmum maritimum is far from perfect. Moreover, the water- and oil-soluble components are too complex to be compatible with each other. Thus, the traditional water- or oil-based extracts of Crithmum maritimum encompass only water- or oil-soluble components, respectively. The effective components being incomplete results in significantly reduced effects. Therefore, obtaining a full-component extract of Crithmum maritimum is imperative to ensure its full functionality. Herein, a full-component extract of Crithmum maritimum based on miscibility between polyol and oil was developed by extracting water-soluble active substances with a ternary solvent, followed by mixing them with oil extracts of Crithmum maritimum. In ternary solvents, most water-soluble compounds of Crithmum maritimum can be extracted by ethanol. During evaporation, the water-soluble components are transferred and dissolved in hexanediol from the solvent. Thus, by blending hexanediol with caprylic/capric triglyceride (GTCC), we successfully created a potent full-component extract of Crithmum maritimum. Through the study of the barrier-weakening 3D full-skin model, it was found that the full-component extract of Crithmum maritimum, rich in chlorogenic acid, could significantly promote the epidermal regeneration of UV-induced skin damage compared to the oil-based extract components.
Introduction
Crithmum maritimum is an edible halophyte that grows in the coastal areas of the Mediterranean, Black Sea, and Atlantic Europe. This plant has both edible and medicinal value because of several major bioactive components inside it, including vitamin C, carotenoids, and fatty acids. In the water-soluble compounds of sea fennel, carbohydrates account for the largest proportion, mainly sucrose and glucose, followed by organic acids and polyphenolic substances.1 Its polyphenolic substances are secondary metabolites of Crithmum maritimum with antioxidative properties. Especially, chlorogenic acid is a well-known polyphenolic secondary metabolite with various biological activities. It can eliminate the activity of hydroxyl radicals and superoxide anions in tissues by forming hydrogen radicals with antioxidant effects.2 In the oil-soluble compounds of sea fennel, the proportion of fatty acids is high, and it has high contents of flavonoids and carotenoids. Its essential oil is antimicrobial and insecticidal because it is rich in γ-terpinene, β-phellandrene, carvacrol methylether, and β-ocimene.3–5 Based on these abundant active components, Crithmum maritimum can be used for dermal repair and epidermal barrier regeneration in cosmetics.6,7 However, the extraction process for Crithmum maritimum is not perfect as the water- and oil-soluble components are too complex to be compatible with each other. Traditional sea fennel extracts usually involve methanol or ethanol as solvents, and the active substances obtained are phenolic acids, flavonoids, etc. The extraction methods of sea fennel essential oil with antibacterial and insecticidal properties are generally distillation or supercritical extraction.8 Thus, the traditional extracts of Crithmum maritimum encompass only water- or oil-soluble components, resulting in incomplete effective components and significantly reduced effects. Obtaining a full-component extract of Crithmum maritimum is imperative to ensure its total functionality.
Ultraviolet (UV) radiation can directly damage cellular DNA and activate oxidative stress, leading to skin aging phenomena, such as dry skin and skin wrinkles. However, recent studies have indicated that chlorogenic acid can effectively reduce the damage of UV radiation to the skin by protecting cells and inhibiting the oxidative stress state of cells.9–11 Chlorogenic acid extracted from green tea, coffee, and Artemisia can protect collagen in the skin from oxidative damage and reduce UV radiation damage to the skin because of anti-oxidation from chlorogenic acid.12 A study by Li et al.13 found that after irradiation of human skin fibroblasts with 40 J cm−2 and 70 J cm−2 of UVA and UVB, respectively, and treating them with different concentrations of chlorogenic acid, the activity of photoaging cells is enhanced significantly.
Chlorogenic acid is commonly used as a quality marker to verify the activity of extracts. However, wild Crithmum maritimum cannot stably produce these active substances, prompting the exploration of new alternative methods. Against this background, a new culture was discovered called a dedifferentiated cell culture, which relies on the ability of plant cells to reach totipotency after differentiation, meaning that a leaf cell can transform into a root cell based on the signals it receives. In addition, using dedifferentiated cells as raw materials for extraction can also avoid risks such as pesticide residues and heavy metals.
Currently, there is no way to make the water-soluble substances represented by chlorogenic acid in Crithmum maritimum fully compatible with ointments. Thus, based on the existing research, the callus cells of fennel were selected as the extraction object, and a complex solvent composed of ethanol, hexanediol, and caprylic/capric triglyceride was used to extract the water-soluble components from the callus cells of fennel. Then, the ethanol was then removed by rotary distillation to obtain the water-soluble extract. Finally, the extracted water-soluble active components were mixed with the oil extract of Crithmum maritimum to obtain the full-component extract of Crithmum maritimum. Chlorogenic acid and its analogues were used as quality markers to verify the content of active components in the extract, and the effect of the full-component extract was verified by discussing changes in active substances in a skin model test.
Experimental
Instrument, reagents and raw materials
Instrument. All extracts from Crithmum maritimum were analyzed by UPLC (Ultra Performance Liquid Chromatography) from Waters Acquity. In addition, all evaluations of skin were performed by applying a fluorescence microscope from Leica (DM2500) and an upright microscope from Olympus (BX53).
Reagents. Methanol, ethanol, acetonitrile, acetic acid, eosin, xylene, hexanediol, and caprylic/capric triglyceride (GTCC) were all in chemical purity level and used without further purification.
Raw materials. Crithmum maritimum from Seppic (Shanghai) Special Chemicals Co., Ltd was used as the raw material, and the oil extract of Crithmum maritimum was produced by Codif Technologie Naturelle.
Cell culture. Primary cultures of keratinocytes and fibroblasts were established from human skin obtained from patients undergoing surgery, following ethical and safety guidelines approved by local institutional review boards. All skin samples were obtained with the informed consent of the donor.
Preparation of the full-component extract of Crithmum maritimum
Solvents were prepared according to the proportions shown in Table 1. The raw material (dedifferentiated cell of Crithmum maritimum) was added to the solvent and dispersed for 0.5 h to obtain a uniform slurry (raw material
:
solvent = 1
:
10 (w/w)). Then, the slurry was poured into a beaker, and an ultrasonic cell crusher (LC-1200E) was used to crush the cell at 40 °C for 30 minutes, stopping 3 s after each 3 s of ultrasonic dispersion to obtain the homogenized solution. After that, the homogenized solution was performed by suction filtration through a 0.25 μm filter membrane, collecting and combining all the filtered solutions. Rotate and evaporate the filtrate at 45 °C for 60 minutes to remove all ethanol. Then, the liquid left in the bottle was filtered by passing through a 0.25 μm filter membrane, and a clear and transparent liquid with the extract of water-soluble active substances from Crithmum maritimum was obtained.
Table 1 Ratio of extraction solvents
Solvent |
Content (wt%) |
Ethanol |
50 |
GTCC |
47.5 |
Hexanediol |
2.5 |
The oil extract of Crithmum maritimum was mixed with the extract from ternary solvent and filtered through a 0.25 μm filter membrane to obtain the full-component extract of Crithmum maritimum.
Analytical method of full-component extract of Crithmum maritimum
Sample processing method. Approximately 0.2 mL of the sample is taken and weighed precisely. The sample is then placed in a stoppered conical flask, and 1.8 mL of 90% methanol is added precisely. The flask is weighed again. The mixture is sonicated (power: 500 W, frequency 40 kHz) for 30 minutes. After sonication, the flask is weighed again, and 90% methanol is used to compensate for any weight loss. The mixture is shaken well and filtered, and the filtrate is collected.
High-performance liquid chromatography (HPLC). The mobile phase consists of A-acetonitrile and B-0.5% acetic acid solution, with gradient elution as illustrated in Table 2. Octadecylsilane chemically bonded silica is used as the filler mobile phase. The column temperature is maintained at no higher than 25 °C, the flow rate is set to 1.0 mL min−1, and the detection wavelength is 327 nm.
Table 2 Mobile phase gradient elution condition
Time (min) |
A (%) |
B (%) |
0–20 |
13–50 |
87–50 |
20–25 |
50–100 |
50–0 |
Evaluation of the skin regeneration effect from full component extract
Experimental setup. The model was transferred to a 6-well plate, and 2 mL of 3D full-thickness skin model culture medium was added to each well. Simultaneously, the model was divided into a blank control group (CON), negative control group (UVA), and test groups: full-component extract of Crithmum maritimum (FCE) and oil-extracts of Crithmum maritimum (OE), as shown in Table 3, with three replicates per group. Test samples were administered every other day for a total of 3 times. The sample groups received surface administration with a volume of 20 μL by a gentle circular motion. After applying the test samples to the surface of the model in the testing group, the model was incubated for 24 hours in a CO2 incubator (37 °C, 5% CO2 by volume). After incubation, the residual test examples on the model surface were washed with sterile PBS solution, and the residual liquid inside and outside the model was wiped off with a sterile cotton swab. Subsequent tests were then conducted.
Table 3 Test groups and detection indicators
Group |
Sample name |
Dosage concentration |
Stimulus conditions |
Detection indicators |
Con |
|
|
|
Organizational form, collagen fibers, collagen I, LOR |
UVA |
|
|
UVA (35 J cm−2) |
FCE |
Full-component extract |
30% |
OE |
Oil extract |
H&E staining. After cleaning the model, it was fixed with 4% paraformaldehyde (POM) for 24 hours, then embedded in paraffin and sliced. The middle section of the slice was baked at 58 °C for 30 minutes. After dehydration and hydration, it was stained with hematoxylin for 3 to 5 minutes, differentiated with hydrochloric acid, returned to blue with ammonia water, washed with water, and sliced into 85% and 95% gradient alcohol for dehydration. The section was stained with eosin for 5 minutes and then sealed with neutral resin after dehydration. The condition of the skin wound was observed under a microscope.
Masson staining. After cleaning the model, it was fixed with 4% POM for 24 hours, followed by paraffin embedding and sectioning. The middle section of the section was selected and baked at 58 °C for 30 minutes. After dehydration and dewaxing, the composite staining solution was applied for 5 minutes. Then, the section was washed with distilled water, treated with phosphomolybdic acid hydrate, stained with aniline blue for 10 minutes, differentiated with 1% acetic acid, dehydrated with anhydrous ethanol, and subjected to microscopic examination after sealing the slide to detect the collagen fibers.
Immunofluorescence staining (IF). The model used for detection was fixed with 4% POM for 24 hours, followed by gradient dehydration and paraffin-embedded sections. The paraffin-embedded sections were dewaxed and hydrated. Endogenous peroxidase activity was blocked by adding H2O2 and incubating at room temperature. After washing, serum homologous to the secondary antibody was added and blocked at 37 °C without rinsing. The primary antibody working solution was added and incubated overnight at 4 °C. After washing with PBS, the secondary antibody was added and incubated at room temperature for 1 hour. ABC composite solution was then added and incubated at room temperature, followed by diaminobenzidine (DAB) staining according to the instructions. The cell nucleus was restained with hematoxylin for 1 minute, washed thoroughly with water, decolorized with 1% hydrochloric acid ethanol, reversed blue with 1% ammonia water, washed thoroughly with water, subjected to gradient dehydration, sealed with neutral resin, and examined microscopically.
Immunohistochemical staining (IHC). The model used for detection was fixed with 4% POM for 24 hours, followed by paraffin embedding and sectioning. The paraffin-embedded sections were dewaxed and hydrated. Endogenous peroxidase activity was blocked by adding H2O2 and incubating at room temperature. After washing, serum homologous to the secondary antibody was added and blocked at 37 °C without rinsing. The primary antibody working solution was added and incubated overnight at 4 °C. After washing, the secondary antibody was added and incubated at room temperature for 1 hour. Nuclear staining was performed with Hoechst, followed by incubation at room temperature. The sections were sealed with a drop of anti-quenching agent, and fluorescence microscopy photography was performed within 24 hours.The enhancing rate was calculated using the following formula:
Results and discussion
Miscibility mechanism of chlorogenic acids and oil
Chlorogenic acid contains multiple hydroxyl groups that are not miscible in oil. The miscibility between oil and chlorogenic acid can be promoted by hexanediol, which has a specific solubility for chlorogenic acids and is readily miscible in oil. During the entire extraction process, active substances with high polarity, such as chlorogenic acid, are extracted with ethanol. Subsequently, after rotary evaporation, these active substances are transferred from ethanol to hexanediol. The specific process is shown in Fig. 1.
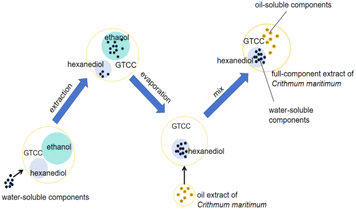 |
| Fig. 1 Miscibility mechanism. | |
Influence of the ternary solvent on the extract of Crithmum maritimum
Chlorogenic acid and isochlorogenic acid A were identified by comparing their retention times. The results show that both extraction solvents have absorption peaks of these two active substances in their HPLC spectra with some differences (Fig. 2). In comparison to traditional ethanol extraction, although the content of some water-soluble active substances may be affected, ternary solvents can still extract water-soluble active components from Crithmum maritimum.
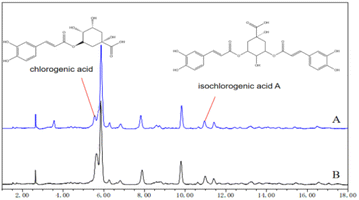 |
| Fig. 2 HPLC of the ternary solvent extract (A) and ethanol extract (B) from Crithmum maritimum. | |
Differences between the oil extract and the full component extract of Crithmum maritimum
The differences between the oil extract and the full-component extract of Crithmum maritimum were compared by HPLC, as shown in Fig. 3. In the spectra, chlorogenic acid and isochlorogenic acid A were identified in the full-component extract of Crithmum maritimum by HPLC with retention times of 5–6 minutes and 11 minutes, respectively. In contrast, the spectra of the oil extract of Crithmum maritimum showed no absorption peak of chlorogenic acid. Additionally, isochlorogenic acid A was present in the spectra of the oil extract, but its concentration was notably lower.
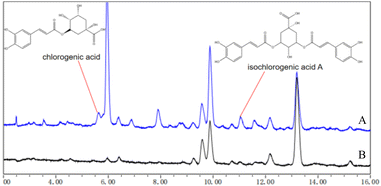 |
| Fig. 3 HPLC of the full-component extract (A) and oil extract (B) of Crithmum maritimum. | |
Evaluation of the skin regeneration effect from full component extract
Skin model. A skin model was prepared as described previously.14 Briefly, a dermal equivalent was prepared by adding a suspension of 250
000 fibroblasts per cm2 on the collagen–glycosaminoglycan–chitosan porous scaffold. All dermal equivalents were cultured for 21 days in a fibroblast medium containing 10 μg mL−1 L-ascorbic acid 2-phosphate. The medium was changed daily. On day 21, keratinocytes were seeded on the dermal equivalent at a density of 250
000 cells per cm2. After 7 days of submerged culture in the keratinocyte medium, the skin equivalent model was raised to the air–liquid interface and cultured in a 3D full-thickness skin model culture medium. UVA irradiation was started on the day the model was received. In addition to group CON, group (UVA) and sample group (OE, FCE) were irradiated with a UVA irradiator from Philips with a 35 J cm−2 irradiation dose. The UVA irradiation dose was 35 J cm−2. The frequency of irradiation was one time per day, and the total irradiation was performed 4 times, as shown in Fig. 4.
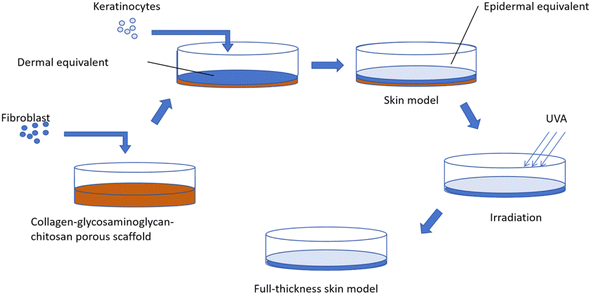 |
| Fig. 4 Process of the full-thickness skin model. | |
Evaluation. Exposure to ultraviolet radiation can induce damage to the skin barrier and collagen depletion. However, chlorogenic acid has a free radical scavenging effect, which can protect the skin by reducing oxidative stress in cells by scavenging free radicals.15,16 Herein, using a full-thickness skin model with weakened barriers by UV is suitable for the evaluation of the skin regeneration effect from full-component extract, the histological morphology, loricrin (LOR) content, collagen content, and collagen fiber content of the epidermal layer in this model were observed to determine whether the extract of Crithmum maritimum had cell repair and anti-wrinkle effects. Compared to the UVA group, all test groups demonstrated increased thickness of the living cell layer and higher LOR content of the epidermis based on the barrier-weakening 3D full-skin model test. At the same UVA dose, the thickness of the epidermis in the OE group reached 64.69 μm with a 6.17% increase, and the content of collagen fibers, collagen I and LOR increased 250%, 105%, and 77.27%. Similarly, the FCE group showed an improved epidermal thickness increase from 27.33% to 77.58 μm along with significant increases of 416.67% in collagen fiber content, 135% in collagen I, and 125% in LOR. Detailed results are presented in Fig. 5–8 and Tables 4–7.
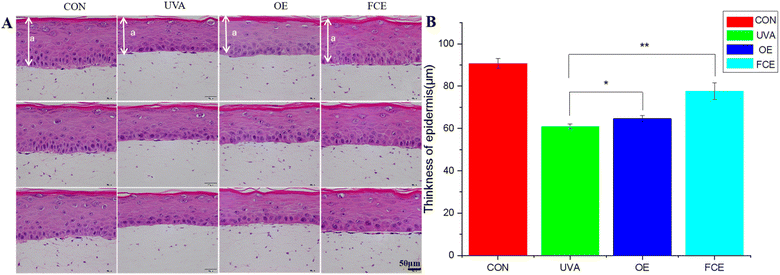 |
| Fig. 5 HE (A), changes in epidermis (B) (a = epidermis,*p < 0.05, **p < 0.01). | |
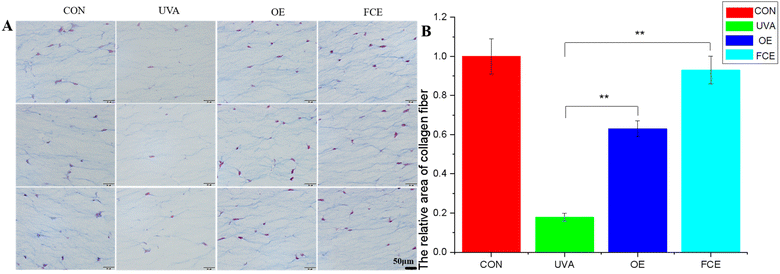 |
| Fig. 6 Masson staining (A), changes in collagen fiber (B) (*p < 0.05, **p < 0.01). | |
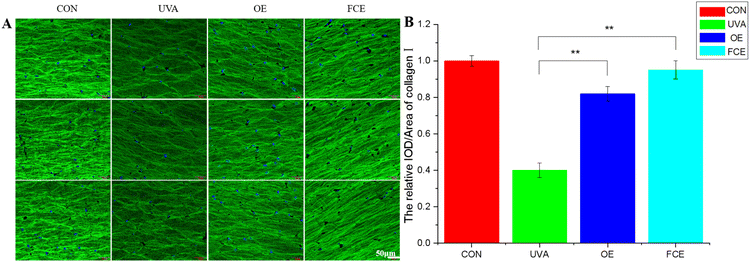 |
| Fig. 7 IF (A), changes in collagen I (B) (*p < 0.05, **p < 0.01). | |
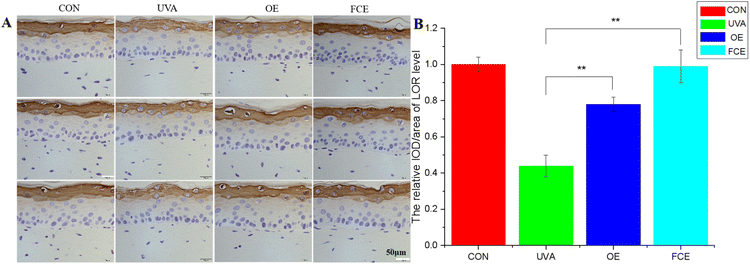 |
| Fig. 8 IHC (A), changes in LOR content (B) (*p < 0.05, **p < 0.01). | |
Table 4 Thickness of the epidermis
Groups |
Mean thickness of living epidermal cells (μm) |
SD |
Increase (%) |
CON |
90.79 |
2.20 |
|
UVA |
60.93 |
1.26 |
|
OE |
64.69 |
1.40 |
6.17 |
FCE |
77.58 |
3.88 |
27.33 |
Table 5 Changes in collagen fibers
Groups |
Average of relative area |
SD |
Increase (%) |
CON |
1.00 |
0.09 |
|
UVA |
0.18 |
0.02 |
|
OE |
0.63 |
0.04 |
250.00 |
FCE |
0.93 |
0.07 |
416.67 |
Table 6 Changes in collagen I in skin modela
Groups |
Relative IOD/area of collagen I |
SD |
Increase (%) |
IOD is short for integrated optical density. |
CON |
1.00 |
0.03 |
|
UVA |
0.40 |
0.04 |
|
OE |
0.82 |
0.04 |
105.00 |
FCE |
0.95 |
0.05 |
137.50 |
Table 7 Changes in LOR content in the skin model
Groups |
Relative IOD/area of LOR level |
SD |
Increase (%) |
CON |
1.00 |
0.04 |
|
UVA |
0.44 |
0.06 |
|
OE |
0.78 |
0.04 |
125.00 |
FCE |
0.99 |
0.09 |
77.27 |
Based on the above experimental results, it can be observed that the full-component extract of Crithmum maritimum shows better skin repair and anti-aging effects than the oil extract of Crithmum maritimum. Specifically, it increases the thickness of active cells in the epidermis by 19.93%, boosts LOR content by 26.92%, enhances collagen fiber content by 47.62%, and increases collagen I content by 15.85%. The reason for this result may be that the full-component extract could repair cell damage caused by ultraviolet light. Ultraviolet radiation can directly damage cellular DNA and activate oxidative stress, causing cellular DNA damage.17–19 However, the phenolic acids and flavonoids in Crithmum maritimum can repair cell damage caused by ultraviolet radiation. In a recent review, chlorogenic acid from Crithmum maritimum was reported to scavenge oxygen free radicals, resist lipid peroxidation, and protect cells from oxidative damage. In addition, chlorogenic acid can stimulate the upregulation of skin barrier genes, such as filaggrin (FLG), involucrin (IVL), and envoplakin (EVPL), in epidermal keratinocytes.20–22 Except for chlorogenic acid, flavonoids in Crithmum maritimum also have a skin repair effect. It not only possesses certain antioxidant effects but also could promote epidermal self-repair by promoting keratinocyte proliferation.23,24
Conclusion
Chlorogenic acid in Crithmum maritimum can be extracted using a composite solvent of ethanol, GTCC, and hexanediol. Moreover, the resulting extract is designed to be compatible with the oil extract of Crithmum maritimum, resulting in the full-component extract of Crithmum maritimum. This type of extract has been shown to increase the thickness of the epidermis, enhance LOR content, boost collagen fiber content, and increase type I collagen content, which can promote epidermal regeneration. Compared to a single Crithmum maritimum ointment, the effects of the full-component extract are notably more pronounced. The full components of the sea fennel are complex, and the synergistic effect between the components needs to be explored. In addition, this extraction method can be extended to other active plants.
Data availability
All relevant data has been included within this manuscript.
Author contributions
Hongbin Huang: conception, revision; Jin Qian: conception, methodology, experiment, analysis, writing; Lanlan Zeng: conception; Jin Zhang: conception; Xiaobo Peng: revision; Xilong Tang: revision; Yanzhang Tang: revision; Meimei Fu: help on experiments, conception, revision, edit; Qi Liu: help on experiment, data presentation, analysis; Fengrui Song: supervision, revision; Jinshan Guo: supervision, conception, methodology, revision; Zhihui Lu: supervision, revision.
Conflicts of interest
There are no conflicts to declare.
Acknowledgements
This work is supported by the funding of the Joint Innovation and Research Laboratory for High tech Raw Materials built by Guangzhou Cadlin Cosmetics Technology Co., Ltd. and Huangpu Institute of Materials.
Notes and references
- L. Meot-Duros and C. Magné, Plant Physiol. Biochem., 2009, 47, 37–41, DOI:10.1016/j.plaphy.2008.09.006.
- N. Nabet, H. Boudries, N. Chougui, S. Loupassaki, S. Souagui, F. Burló, F. Hernández, Á. A. Carbonell-Barrachina, K. Madani and R. Larbat, Int. J. Food Prop., 2017, 20, 1843–1855, DOI:10.1080/10942912.2016.1222541.
- T. Kulisic-bilusic, I. Blažević, B. Dejanović, M. Miloš and G. Pifat, J. Food Biochem., 2010, 34, 286–302, DOI:10.1111/j.1745-4514.2009.00330.x.
- P. Kaan, Ö. C. Karakoç, Y. Y. Yasemin, G. Salih, D. Betül, K. H. C. Başer and F. Demirci, Ind. Crops Prod., 2016, 89, 383–389, DOI:10.1016/j.indcrop.2016.05.032.
- M. M. Ozcan, L. G. Pedro, A. C. Figueiredo and J. G. Barrosol, J. Med. Food, 2006, 9, 128–130, DOI:10.1089/jmf.2006.9.128.
- C. Lequeux, A. Lhoste, M. R. Rovere, C. Montastier and O. Damour, Skin Pharmacol. Physiol., 2011, 24, 75–80, DOI:10.1159/000321991.
- M. Caucanas, C. Montastier, G. E. Piérard and P. Quatresooz, J. Cosmet. Dermatol., 2011, 10, 288–293, DOI:10.1111/j.1473-2165.2011.00584.x.
- M. V. Bratinčević, R. Kovačić, M. Popovi, S. Radman and I. G. Mekinić, Processes, 2023, 11, 2172, DOI:10.3390/pr11072172.
- Y. Wang, Q. Chen, J. Li and N. Zhang, Zhongcaoyao, 2014, 45, 386–391, DOI:10.7501/j.issn.0253-2670.2014.3.016.
- K. Kunchana, W. Jarisarapurin, L. Chularojmontri and S. K. Wattanapitayakul, Antioxidants, 2021, 10, 703, DOI:10.3390/antiox10050703.
- T. Liu, Y. Liu, Q. Xia, Y. Yu, S. Wang, K. Xiong, Y. Yan and N. Li, Chin. J. Exp. Tradit. Med. Formulae, 2019, 25, 224–230, DOI:10.13422/j.cnki.syfjx.20191207.
- J. Li, L. Song, Q. Chen and Y. Xu, Inf. Tradit. Chin. Med., 2013, 30, 25–27, DOI:10.3969/j.issn.1002-2406.2013.04.011.
- Y. H. Cho, A. Bahuguna, H. H. Kim, D. I. Kim, H. J. Kim, J. M. Yu, H. G. Jung, J. Y. Jang, J. H. Kwak, G. H. Park, O. J. Kwon, Y. J. Cho, J. Y. An, C. Jo, S. C. Kang and B. J. An, J. Photochem. Photobiol., B, 2017, 174, 323–332, DOI:10.1016/j.jphotobiol.2017.08.015.
- F. D. Perrat, O. Damour, C. Montrocher, S. Peyrol, G. Grenier, M. P. Jacob and F. Braye, J. Invest. Dermatol., 2000, 114, 365–370, DOI:10.1046/j.1523-1747.2000.00885.x.
- S. Kang, H. C. Jin, J. H. Lee, G. J. Fisher, Y. S. Wan, E. A. Duell and J. J. Voorhees, J. Invest. Dermatol., 2003, 120, 835–841, DOI:10.1046/j.1523-1747.2003.12122.x.
- S. Wu, M. Yuan, J. Man, Z. Gao and D. Zhang, J. Food Sci. Biotech., 2023, 42, 96–101, DOI:10.3969/j.issn.1673-1689.2023.05.013.
- Z. Assefa, A. V. Laethem, M. Garmyn and P. Agostinis, Biochim. Biophys. Acta, 2005, 1755, 90–106, DOI:10.1016/j.bbcan.2005.04.001.
- M. Ichihashi, M. Ueda, A. Budiyanto, T. Bito, M. Oka, M. Fukunaga, K. Tsuru and T. Horikawa, Toxicology, 2003, 189, 21–39, DOI:10.1016/s0300-483x(03)00150-1.
- C. Cao, L. Shan, R. Kivlin, B. Wallin, E. Card, A. Bagdasarian, T. Tamakloe, W. Chu, K. Guan and Y. Wan, J. Biol. Chem., 2010, 285, 14842, DOI:10.1074/jbc.A109.804144.
- J. W. Cha, M. J. Piao, K. C. Kim, C. W. Yao, J. Zheng, S. M. Kim, C. L. Hyun, Y. S. Ahn and J. W. Hyun, Biomol. Ther., 2014, 22(2), 136–142, DOI:10.4062/biomolther.2014.006.
- K. H. Lee, H. K. Do, D. Y. Kim and W. Kim, Biochem. Biophys. Res. Commun., 2021, 583, 22–28, DOI:10.1016/j.bbrc.2021.10.057.
- W. C. Chen, S. S. Liou, T. F. Tzeng, S. L. Lee and I. M. Liu, Planta Med., 2013, 79, 616–621, DOI:10.1055/s-0032-1328364.
- M. Cho, H. Yoon, M. Park, Y. H. Kim and Y. Lim, Phytomedicine, 2014, 21, 560–569, DOI:10.1016/j.phymed.2013.10.006.
- C. Y. Chen, C. M. Liu, H. C. Yeh, H. M. Wu, W. J. Li and H. T. Li, Chem. Nat. Compd., 2021, 57, 917–920, DOI:10.1007/s10600-021-03512-w.
Footnote |
† These authors contributed equally. |
|
This journal is © The Royal Society of Chemistry 2025 |
Click here to see how this site uses Cookies. View our privacy policy here.