DOI:
10.1039/D4RA07438F
(Review Article)
RSC Adv., 2025,
15, 688-711
Cracking the code: the clinical and molecular impact of aminopyridines; a review (2019–2024)
Received
17th October 2024
, Accepted 16th December 2024
First published on 8th January 2025
Abstract
Aminopyridines belong to a class of compounds that are monoamino and diamino derivatives of pyridine. They work primarily by blocking voltage-gated potassium channels in a dose-dependent manner. Essential heterocycles used extensively in synthetic, natural products, and medicinal chemistry are aminopyridine and its derivatives. A vast array of biological and pharmacological effects can result from the interaction of aminopyridine rings with different enzymes and receptors, due to their unique structural properties. Aminopyridine research is continually growing, and there are now greater expectations for how it may aid in the treatment of numerous disorders. This review article will serve as an innovative platform for researchers investigating aminopyridine compounds, intending thoroughly to examine both traditional and novel synthesis strategies in addition to investigating the various biological characteristics displayed by these adaptable heterocycles. We attempt to provide valuable insights that will contribute to further progress in the synthesis and utilization of aminopyridines in various fields.
1 Introduction
N-heterocyclic complexes exhibit a huge spectrum of remarkable biological properties.1 The nitrogen-bearing heterocycle pyridine in all of its similar forms is an important source of pharmaceuticals with therapeutic action in medicinal chemistry research. The Food and Drug Administration (FDA) has regularly authorized this privileged scaffold along with a long list of other pharmaceutical candidates. Due to its ease of parallelization and potential for chemical space testing, this moiety is gaining interest in a variety of disease scenarios.2,3 Besides, it is a key solvent and reagent,4 also essential to the advancement of drugs.5–7 The majority of pyridine derivatives are playing a crucial role in HIV antiviral therapy,8 as well as in hypnosis,9 sedation,10 bone calcium,11 cholesterol,12 and triglyceride regulation,13 as antidiabetic,14 antihistaminic,15 antiulcerate,16 antineoplastic,17 and anticancer agents.18 A versatile surface modification for immobilizing nanoparticles is poly vinyl pyridine 1.19 Niacin, or nicotinic acid 2, is a vitamin B-3 and an essential component of food for humans.20 Tryptophan is an amino acid that can be produced by both plants and animals.21 Nicotine 3 is a stimulant that speeds up the messages from the brain to the body.22 An alkaloid called anabasine 4, containing piperidine and pyridine, is found in the stems and leaves of tobacco plants.23 It is both chemically and structurally identical to nicotine, mostly used as an insecticide in industry.24 Toxalbumin ricinine 5 is obtained from Ricinus communis, produces castor beans.25 Atazanavir 6 is used along with other medications to treat human immunodeficiency virus (HIV) infections.26 Imatinib mesylate 7 is used for gastrointestinal stromal tumors.27 Pyridoxal 8 is one of the regular forms available of vitamin B-6, for treating dietary shortages or imbalances (Fig. 1).28,29
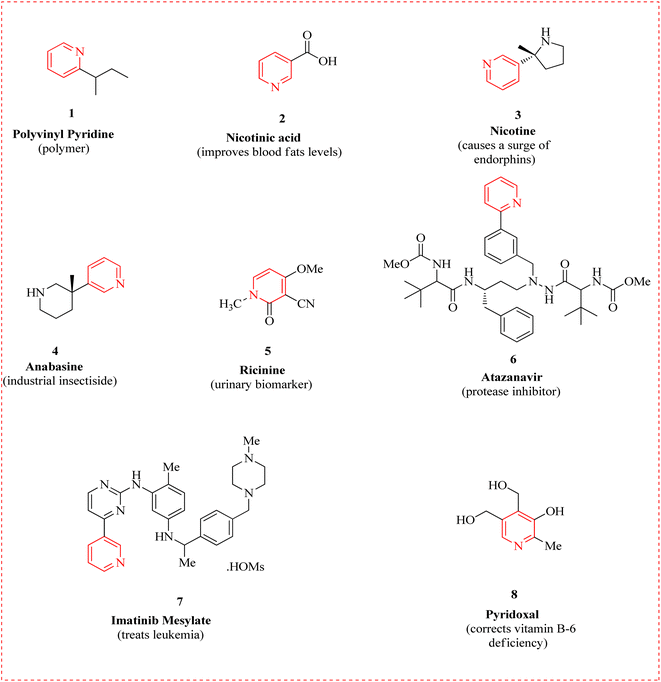 |
| Fig. 1 Naturally occurring pyridine derivatives. | |
Aminopyridine, a derivative of pyridine, first came to prominence through scientific studies in the mid-20th century.30 2-Aminopyridine (2-AP) 9 is a completely functionalized, low molecular weight moiety used in the synthesis of many different biological compounds,31 being synthesized by a large number of pharmaceutical companies worldwide.32–36 2-AP functions as an ideal propellant in pulling these molecules in the direction of their pharmacological purposes.37 The first aminopyridine to be applied in a therapeutic setting is 4-aminopyridine (4-AP) 10.38 That stimulates the release of acetylcholine and conceivably noradrenaline at nerve terminals by blocking potassium channels.39 When the pharmacological characteristics were first reported in 1924, remarks were made about the drug's excitatory effects on the central nervous system and its vasopressor activity.40 In the 1960s and 1970s, research began to reveal its potential therapeutic uses, particularly in neurology.
Ampyra 10, also known as dalfampridine, is an aminopyridine that facilitates walking in multiple sclerosis (MS) patients.41 This potassium channel blocker is used to treat neuromyelitis optica spectrum disorder (NMOSD) and MS in order to improve motor function.42 Sulfasalazine 11, as an Immunomodulator and Crizotinib 12 is a tyrosine kinase inhibitor of the anaplastic lymphoma kinase (ALK), receptor tyrosine enzyme (ROS1) and mesenchymal epithelial transition (MET) kinases.43 Crizotinib is a more operative for initial treatment for patients with newly identified advanced ALK-positive non-small cell lung cancer (NSCLC) than the platinum-based double-agent chemotherapy.44 Amifampridine 13 is a medication that belongs to the aminopyridine class.45 A histamine antagonist H1 called tripelennamine is used against coughs, colds, and hypersensitivity reactions.46 Mepyramine 14 is an antihistamine that is used to treat allergy symptoms, hypersensitivity responses, and urticaria.47 Urinary tract analgesic phenazopyridine 15, also referred to as pyridium, is used to treat urinary tract irritation in the short term and its unpleasant side effects, as burning and pain during urination (Fig. 2).48 Pyrilamine acts on the H1 receptor and is an antihistamine of the first generation.49 Aminopyridines are expected to be extensively used in next-generation drugs that address a wide range of diseases, including cancer, heart disease, and neurological complications.50,51
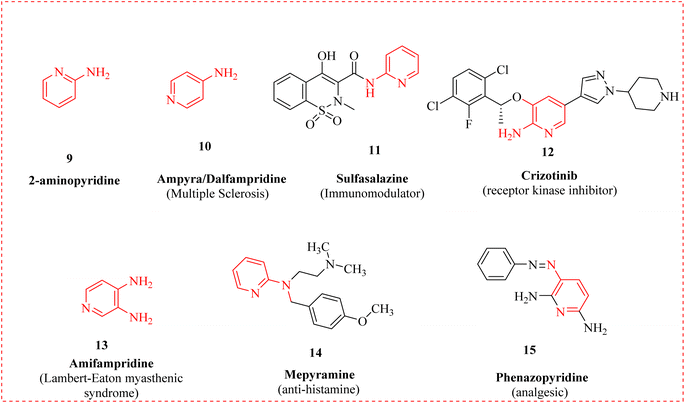 |
| Fig. 2 FDA-approved aminopyridine-containing drugs. | |
Improvements in synthetic chemistry and drug delivery strategies will augment the medicinal potential of aminopyridines and pave the way for the development of more effective, targeted medications with reduced side effects and improved bioavailability.52,53 Aminopyridines are becoming essential parts of customized therapy techniques, which use drugs based on a patient's genetic composition and the specifics of their condition, as personalized medicine continues to gain traction.54 By using the unique properties of aminopyridines and state-of-the-art technology, upcoming drug development initiatives are well-positioned to open up new routes for treating unmet medical needs and improving patient outcomes.55 A higher proportion of innovative aminopyridine-based therapeutic candidates is anticipated in the upcoming years. The latest developments in novel aminopyridine-based molecular frameworks and their distinct therapeutic significance, as documented over the previous years, are combined in this study. It draws attention to a trend toward the usage of aminopyridine-based compounds in therapeutic development and the subsequent rise of a number of strong and promising candidates against a variety of expanding diseases.
2 Pharmacology
2.1 Anti-bacterial and anti-inflammatory potential
Inflammation is a process stimulated against pathogen infections,56 is regulated by several molecules having anti-inflammatory activities.57 The continuous production of inflammatory agents can lead to tissue and DNA damage by stimulating cell proliferation to cancer.58 A novel mononuclear copper complex [Cu (C5H6N2)4] Br2.2 (C3H7NO) 17, called Cu-4AP-Br was reported by the reaction of copper bromide 16 and 4-amino pyridine 10 hydrothermally in DMF solution. That shows remarkable antioxidant and antibacterial activities. For measuring its anti-inflammatory activity naproxen (NAP) 18 a known, effective anti-inflammatory drug was taken as reference. The inhibition of 5-lipoxygenase (5-LOX) activity of NAP and Cu-4AP-Br are shown in Table 1. The anti-inflammatory activity of this pure molecule was 4.6 times higher than that of the synthetic material at a dose of 25 μg mL−1. The finding has shown that, at a test concentration of 100 μg mL−1, Cu-complex exhibited high anti-inflammatory activity (IP = 54.2 ± 2.08%). The standards were provided as the mean ± SD of three independent measures (n = 3). Using Cu-4AP-Br and NAP as an optimistic regulator (Scheme 1).59
Table 1 Anti-inflammatory activity of mononuclear copper complex and NAP at several concentrations
Compounds |
Concentration (μg mL−1) |
Inhibition |
NAP |
2.5 |
15.01 ± 0.47 |
5 |
31.64 ± 0.82 |
25 |
95.00 ± 2.3 |
Cu-complex |
25 |
20.51 ± 0.7 |
50 |
37.96 ± 0.39 |
100 |
54.20 ± 2.08 |
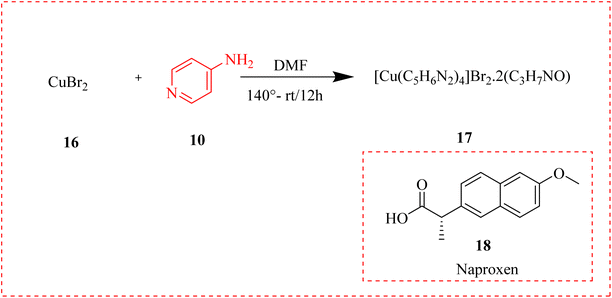 |
| Scheme 1 Synthesis of mononuclear copper complex from copper bromide and 4-AP. | |
Antibiotics are the “magical shots” that fight bacteria, their discovery is regarded as a medical marvel. With the growing use, microorganisms have established antimicrobial resistance (AMR).60 AMR refers to the potential of microorganisms to flourish in the middle of drugs designed to kill them. Antibiotics are a class of antimicrobials specially used to contest bacterial infections.61 A novel and effective multicomponent one-pot technique for the preparation of derivatives of 2-amino-3-cyanopyridine from 3-dimethylamino phenylprop-2-en-1-one 19, malononitrile 20 and substituted amines 21 was proposed. The synthesized molecules (22a–d) were examined, and based on their antimicrobial activity, compound 22c showed the maximum antibacterial action specifically against S. aureus and B. subtilis, with MIC values of 39 ± 0.000 μg mL−1 (Scheme 2).62
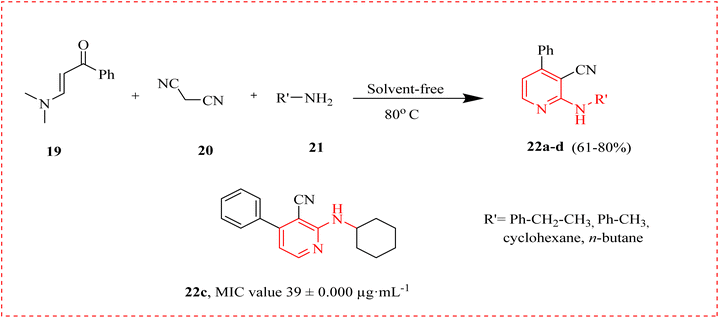 |
| Scheme 2 Synthesis of 2-amino-3-cyanopyridine derivatives. | |
Three novel zinc(II) complexes containing 4-aminopyridine (4-NH2py) were reported. These complexes, [Zn(4NH2py)2(NCS)2], [Zn(4-NH2py)2Cl2] and [Zn(4-NH2py)2(NCS)Cl], were synthesized from methyl zinc(III)chloride hydrate 23, methanol compound with 4-AP 24 and thiocyanate derivative 25. The antibacterial screening of every complex was assessed in vitro using two different bacteria, Staphylococcus aureus and Escherichia coli. According to the findings, 26 has the strongest antibacterial activity of all the others. This variation results from variations in the interactions that the anion ligands elicit (Scheme 3).63
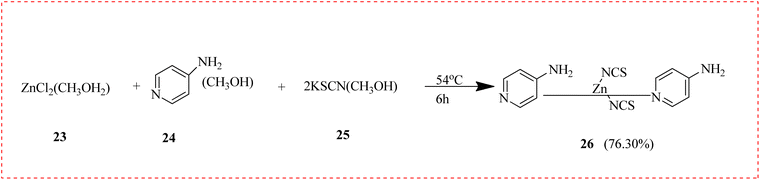 |
| Scheme 3 Synthesis of complex [Zn(4NH2py)2(NCS)2]. | |
Al-Fakeh et al., synthesized complexes by mentioned metal salts: CoCl2·6H2O MnCl2·4H2O, CuCl2·2H2O, PdCl2 and CrCl3·6H2O with Schiff base 27 and 2-amino-4,6-dimethyl pyrimidine (ADMPY) 28, produced when benzaldehyde reacted with p-phenylenediamine and hydroxy-naphthaldehyde. The antioxidant and antibacterial activities of the complexes were investigated. Cu(II) and Pd(II) complexes exhibited extraordinary activity, cobalt and chromium complexes presented temperate antioxidant activity, when compared to the standard (ascorbic acid) while Pd(II) and Cu(II) complexes were best antibacterial agents using gentamycin as standard (Fig. 3).64
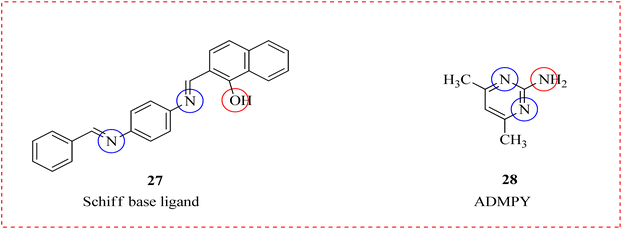 |
| Fig. 3 Structures of Schiff base ligand and ADMPY. | |
Tosylated 4-AP 30 created from 10 and 29 latter complexed with different metals and employed as antibacterial agents. Compared to the coordinated imine of the complexes, the free imine nitrogen in the ligands vibrated at a higher frequency. This implied that the metal ions were receiving lone pair electrons from the free azomethine nitrogen. The antibacterial properties of the ligand and its complexes were assessed using a range of microorganisms. The outcome showed that tosylated 4-aminopyridine's antibacterial activity was increased by complexing with Co(II), Fe(II), and Ni(II) ions (Scheme 4).65
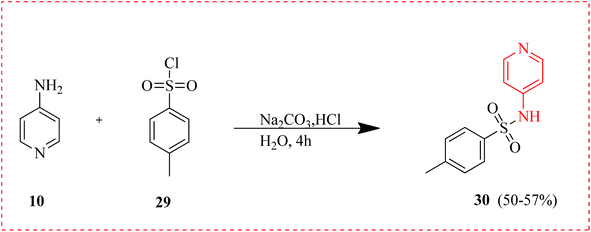 |
| Scheme 4 Synthesis of monotosylated 4-AP. | |
İlkimen et al., reported two new salts (32 and 35) obtained from 2-methoxy-5sulfamoylbenzoic acid (31) and 2-aminopyridine (9) or 2-amino-4methylpyridine (33) and their Cu(II) complexes. Levofloxacin, Vancomycin, Cefepime, and Fluconazole were the standard antibiotics. All moieties exhibited antibacterial property. The compounds with the highest activity are Cu (OAc)2·2H2O (31.25 μg mL−1) for Candida albicans, 32 (31.25 μg mL−1) for Lactobacillus monocytogens, all compounds (7.60 μg mL−1) for Bacillus subtilis, 9 (31.25 μg mL−1) for Enterobacter coli, Cu complex (15.60 μg mL−1) for Staphylococcus aureus. All antibacterial drugs were active against L. monocytogens (Scheme 5 and Table 2).66
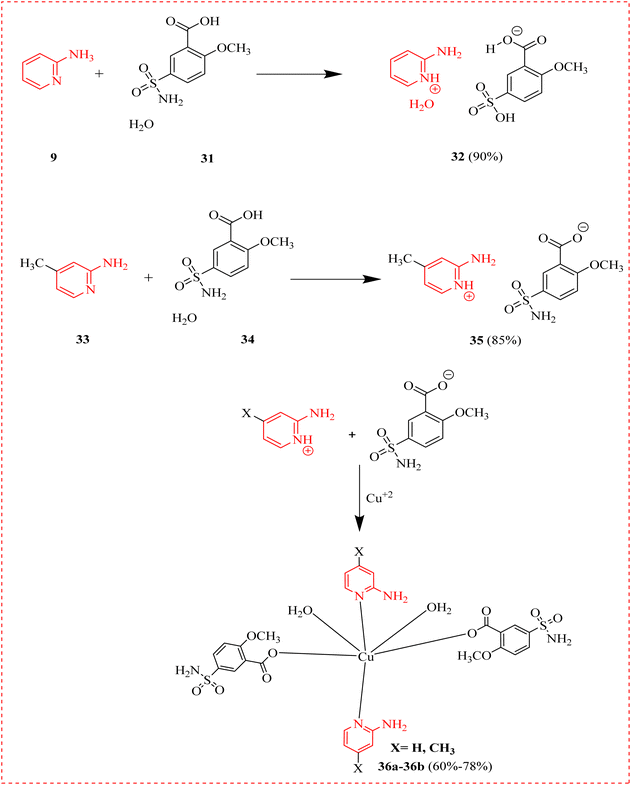 |
| Scheme 5 Synthesis of methoxy-sulfamoylbenzoate and amino-methylpyridinium salts and their copper metal complexes. | |
Table 2 MIC results of the synthesized salts and their metal complexes with standards (μg mL−1)
Molecules |
C. albicans |
L. monocytogens |
B. subtilis |
S. aureus |
E. coli |
32 |
62.50 |
62.50 |
62.50 |
62.50 |
62.50 |
35 |
62.50 |
62.50 |
125.00 |
62.50 |
125.00 |
36a |
62.50 |
62.50 |
125.00 |
125.00 |
125.00 |
36b |
62.50 |
31.25 |
62.50 |
62.50 |
62.50 |
Vancomycin |
— |
125 |
250 |
62.50 |
31.25 |
Levofloxacin |
— |
31.25 |
62.50 |
31.25 |
31.25 |
Cefepime |
— |
31.25 |
62.50 |
62.50 |
62.50 |
Fluconazole |
62.50 |
— |
— |
— |
— |
2.2 Antitrypanosomal and anti-malarial agents
Antitrypanosomal therapy of revived diseases in immunosuppressed patients results in declined intensity of parasitemia.67 Human African trypanosomiasis (sleeping sickness) is a parasitic infection that leads to death.68 A methoxyphenol derivative 37 was reported as priority antitrypanosomal and selective antiplasmodial agent as inhibitors of Trypanosoma brucei. One of the compounds in the collection with promising drug-like properties produced was methylsulfonyl bipyridine amine 38 is undergoing clinical testing to treat malaria. With a view on in vivo mouse testing, they proposed an o-disubstituted phenyl B-ring to optimize the equilibrium between antitrypanosomal activity, solubility, and metabolic stability of this group. This was accomplished by joining molecules with an extra ring nitrogen atom on the C-ring of a p-cyclic amide with an o-disubstituted B-ring pattern (Fig. 4).69
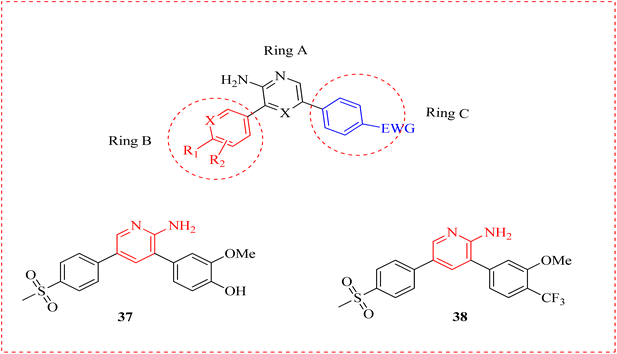 |
| Fig. 4 Structures of reported compounds methoxyphenol 37 and methyl sulfonyl bipyridine amine 38. | |
Oliveira et al., examined the effects of novel aminopyridine derivatives complexed with Cu2+ against T. cruzi trypomastigote forms: cis-aquadichloro pyridinemethanamino copper (39a) and its glycosylated ligand, cis-dichloro (glucopyranosyloxy)phenyl methyl pyridinemethamino copper (39b). These metallodrugs pre-treated trypomastigotes inhibiting the association index with LLC-MK2 cells. When tested on mammalian cells, both complexes shown minimal toxicity (CC50 >100 μM). When tested on intracellular amastigotes, the IC50 values were found to be 14.4 μM for compound 39a and 27.1 μM for complex 39b. Results determined the potential of these aminopyridines complexed with Cu2+ as promising aspirants for additional antitrypanosomal drug development (Fig. 5).70
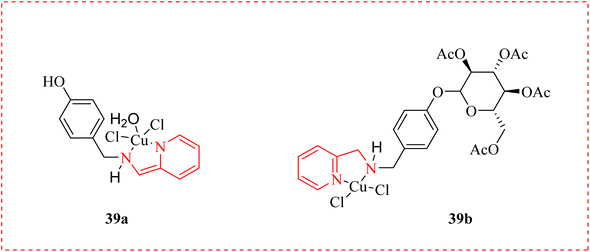 |
| Fig. 5 Structures of the metal-based amino pyridines cis-aquadichloro pyridinemethamino copper (39a) and cis-dichloro tetra-O-acetyl-β-D-glucopyranosyloxy phenyl methyl pyridinemethamino copper (39b). | |
2.3 Anti-multiple sclerosis
The autoimmune disease MS is the primary cause of nontraumatic neurological illness in adults.71 MS is a global problem, and is very predominant.72 Yet there is no cure for multiple sclerosis. Treatment usually emphases on speedy recovery from attacks, reduced radiographic and clinical relapses, reducing the progression of the disease.73 It has been proved that 4-AP that is accepted for the MS. As possible options for positron emission tomography (PET) imaging, they looked into four new 4-AP derivatives. Three out of the four molecules were potent to block the voltage-gated potassium channels KV1. In particular, it was discovered that 3-methyl4-aminopyridine (3Me4-AP) was roughly 7 times more effective than 4-AP (10) and 3-fluoromethyl-4-aminopyridine (3F4-AP) (41a); 3-methyl-4-aminopyridine (3Me4-AP), 3-methoxy-4-aminopyridine (3MeO4AP) (41b and 41c) was about 3–4 times less potent than 4-AP; and 2-trifluoromethyl-4-aminopyridine (2CF34-AP) (41d, 41e) was approximately 60-fold less active. Their findings implied that these new compounds has found use in imaging and therapy (Table 3).74
Table 3 IC50 of 4-AP derivatives and confidence interval. N is the number of times each was tested
Drug |
IC50 (μM) |
95% C.I. (μM) |
N |
10 |
293 |
258–328 |
6 |
41a |
244 |
185–303 |
5 |
41b |
40 |
36–43 |
4 |
41c |
807 |
762–853 |
4 |
41d |
1061 |
914–1209 |
6 |
41e |
12 776 |
8838–16 715 |
4 |
Aydoğmuş et al., established the involvement of K+ channels in the cell cycle by using 4-AP in conjunction with paclitaxel (PTX) (42) in MCF-7 and MDA MB-231 cell lines. To estimate viability, trypan blue was utilized. For understanding the mechanism, intracellular K+ concentration, intracellular Ca2+ concentration, and transmembrane potential measurements were made with fluorescent dyes. According to cell cycle analysis, 4-AP therapy made the MCF-7 and MDA MB-231 cell lines go into G1 arrest. PTX caused G1 stop in MCF-7 cells and S phase in other cells. Combination therapy caused S phase arrest in MCF-7 cells and both G2/M and S phase arrest in MDA MB-231 cells. After every treatment, the intracellular K+ content increased in both cell lines. The Ca2+ concentration significantly improved after combo conduct. This work indicated that the grouping of 4AP and PTX was a viable substitute, taking into account the findings for Ca2+, K+, and membrane potential (Fig. 6).75
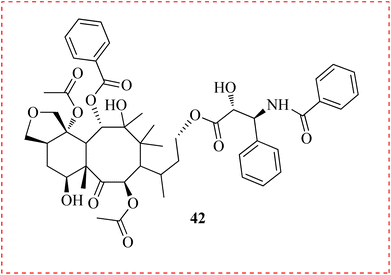 |
| Fig. 6 Structure for paclitaxel. | |
4-AP is a selective voltage blocker channel used for multiple sclerosis patients. The investigation was divided into two phases: after 4-AP underwent H/D exchange, serum monoamine oxidase activity was measured by applying both deuterated and non-deuterated 4-AP to MS patients' serum. There were 120 subjects in total for the trial, split into two groups of 60: MS sufferers and control. Patients' monoamine oxidase (MAO) activity was considerably (P < 0.01) higher than the control groups. When 4-AP was present, there was a significant (P < 0.01) drop in MAO activity, and this drop was further pronounced when deuterated 4-AP was present. In conclusion, 4-AP has an inhibitory effect on MAO activity, and this effect was enhanced when deuterium was used in place of hydrogen (Scheme 6).76
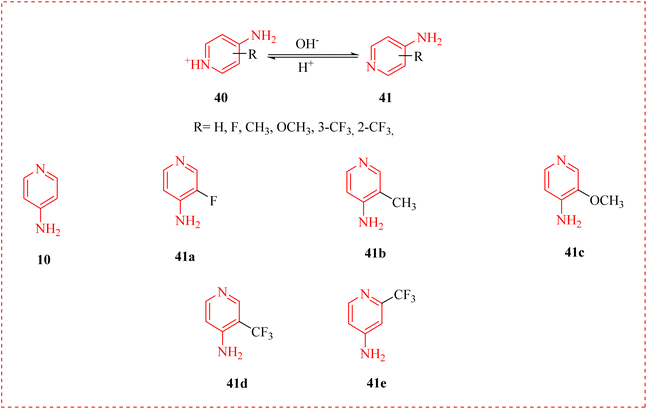 |
| Scheme 6 Acid–base equilibrium of 4-AP analogues. | |
4-AP was reported as symptomatic treatment in several neurologic illnesses because it is an antagonist of Kv channels. 4-AP is linked to MS patients for improved motor function, improved vision, and reduced fatigue. For the characteristic treatment of walking deficiency in MS, its sustained release formulation fampridine 10 has received approval. The enhancement of conduction along demyelinated axons was attributed to the blockage of axonal Kv channels, which accounted for positive effects. Nonetheless, a growing amount of data indicated that fampridine has offered benefits in addition to its symptomatic mechanism of action (Fig. 7).77
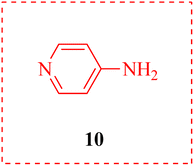 |
| Fig. 7 Structure of fampyridine/4-AP (10) a formulation against MS. | |
2.4 Against metabolic enzymes
Türker et al., studied the enzyme inhibition properties of the Pd-based complexes having both N-heterocyclic carbene (NHC) ligands and 2-AP. The 3-cyanobenzyl group in the NHC ligand of these complexes has inhibitory effects on a few metabolic enzymes, including xanthine oxidase (XO) and carbonic anhydrase (hCA). For hCA I, hCA II, hCA, plus XO the IC50 ranges were found to be 0.325–0.707, 0.238–0.636, and 0.576–1.693 μM, respectively. Pd(II)–NHC compounds containing 2-AP might be effective hCA and XO inhibitors. The novel Pd-based compounds 44a–44f comprised a combination of NHC ligand 42 and 2-AP 9. PdBr2 (pyridine) complexes from the starting material NHC have a weak attachment between the pyridine ligand and the palladium centre. Their findings pointed to the implementation of effective medicine for XO (Scheme 7 and Table 4).78
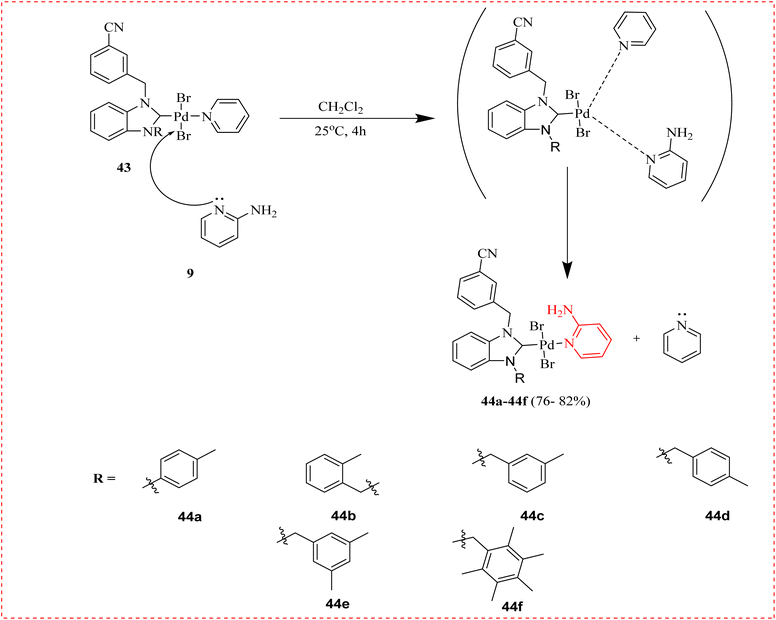 |
| Scheme 7 (NHC)PdBr2 (2-aminopyridine) complexes: synthesis and structures for their derivatives. | |
Table 4 The IC50 values of the NHC PdBr2 2AP compounds 44a–44f on HCA (I) and XO
Compound |
XO (μM) |
hCAI (μM) |
hCAI (μM) |
44a |
1.08 ± 0.04 |
0.47 ± 0.03 |
0.54 ± 0.04 |
44b |
0.66 ± 0.01 |
0.35 ± 0.05 |
0.46 ± 0.04 |
44c |
1.69 ± 0.06 |
0.71 ± 0.04 |
0.64 ± 0.05 |
44d |
0.65 ± 0.02 |
0.41 ± 0.03 |
0.34 ± 0.03 |
44e |
0.58 ± 0.02 |
0.41 ± 0.04 |
0.24 ± 0.03 |
44f |
0.59 ± 0.05 |
0.33 ± 0.02 |
0.32 ± 0.03 |
Aminopyridine containing thiourea derivatives have developed as a vital class of bioactive pharmacophores in the realm of medicinal chemistry.79 Rizvi et al., reported the synthesis of aminopyridine thiourea derivatives (47a–47p) as a powerful, non-competitive inhibitors of α-glucosidase enzyme with a half-maximal inhibitory concentration ranges from 24.62 ± 0.94 to 142.18 ± 2.63 μM in vitro assays (Table 5). Here, compound 47e, 47g, 47h, 47n and 47o exhibited most potent activity (Table 6). Results were supported by computational studies that showed important interactions between enzymes through hydrophobic and π–π assembling forces. Molecular docking and density functional theory were used in kinetic experiments to examine the type of contacts, free energy, and manner of inhibition (Scheme 8).51,80
Table 5 The IC50 values of the 1-phenyl-3-(5-(trifluoromethyl)pyridin-2-yl)thiourea derivatives against α-glucosidase
Compound |
α-Glucosidase inhibition IC50 ± SEM (μM) |
Compounds |
α-Glucosidase inhibition IC50 ± SEM (μM) |
47a |
92.1 ± 3.07 |
47i |
56.81 ± 2.41 |
47b |
— |
47j |
97.91 ± 0.68 |
47c |
81.31 ± 0.86 |
47k |
142.18 ± 2.63 |
47d |
59.76 ± 1.36 |
47l |
121.61 ± 1.36 |
47e |
24.62 ± 0.94 |
47m |
68.91 ± 1.72 |
47f |
63.21 ± 0.96 |
47n |
28.63 ± 2.04 |
47g |
41.87 ± 1.16 |
47o |
38.85 ± 2.17 |
47h |
39.86 ± 1.73 |
47p |
109.31 ± 1.89 |
Acarbose |
875.85 ± 2.03 |
|
|
Table 6 Results of kinetic inhibition studies of most potent compounds (47e, 47g, 47h, 47n, 47o)
Compounds |
Ki ± SEM (μM) |
Type of inhibition |
47e |
19.96 ± 0.0318 |
Non-competitive |
47g |
36.17 ± 0.0112 |
Non-competitive |
47h |
34.21 ± 0.0036 |
Non-competitive |
47n |
47.23 ± 0.0076 |
Non-competitive |
47o |
24.97 ± 0.0231 |
Non-competitive |
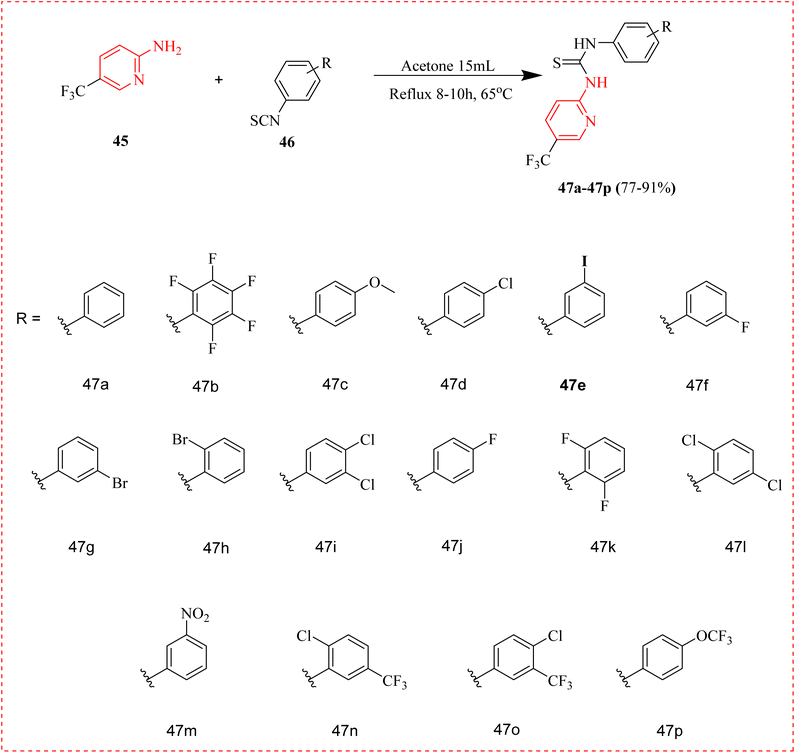 |
| Scheme 8 Synthesis of 1-phenyl-3-(5-(trifluoromethyl)pyridin-2-yl)thiourea derivatives. | |
A novel set of kojic acid derivatives conjugated to amino pyridine intended to investigate its tyrosinase-inhibiting potential was presented. Each derivative showed a distinct inhibitory potency when tested against tyrosinase in comparison to kojic acid, which served as the positive control. Every derivative's antioxidant activity was computed. Compound (48) binds to the enzyme in an uncompetitive manner, according to the kinetics of the most active agent. As a result of their strong binding affinity and notable interactions with the tyrosinase enzyme to target the melanogenesis pathway, the analysis suggests that they could be effective future candidates to regulate hyperpigmentation (Fig. 8).81
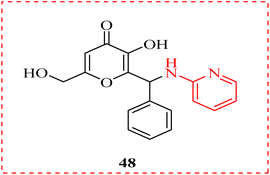 |
| Fig. 8 Structure of methyl-pyran-4-one (48). | |
2.5 Inhibitor of Janus kinase 2 gene
Janus kinase-2 (JAK2) is a non-receptor tyrosine kinase that works as the cytokine receptor's intracellular signalling effector, modulating the effects of growth hormone, leptin, erythropoietin, and interferon.82 3-Methoxypyridine-2-amine (53) reported as the encouraging inhibitor of JAK2 which exhibits great inhibitory effect against myeloproliferative neoplasms IC50 = 6 nM and rheumatoid arthritis and 3 nM, and selectivity for JAK 2 and displayed effective antiproliferative activities against human erythroleukemia cell line human erythroleukemia cells (HEL). Its more effective versions as 54a and 54b. Furthermore, 54a reduced the signs and symptoms in rats with the collagen induced arthritis (CIA model). The freshly synthesized s compounds were screened at 50 μM to see if they could bind to JAK2 (Scheme 9 and Table 7).83
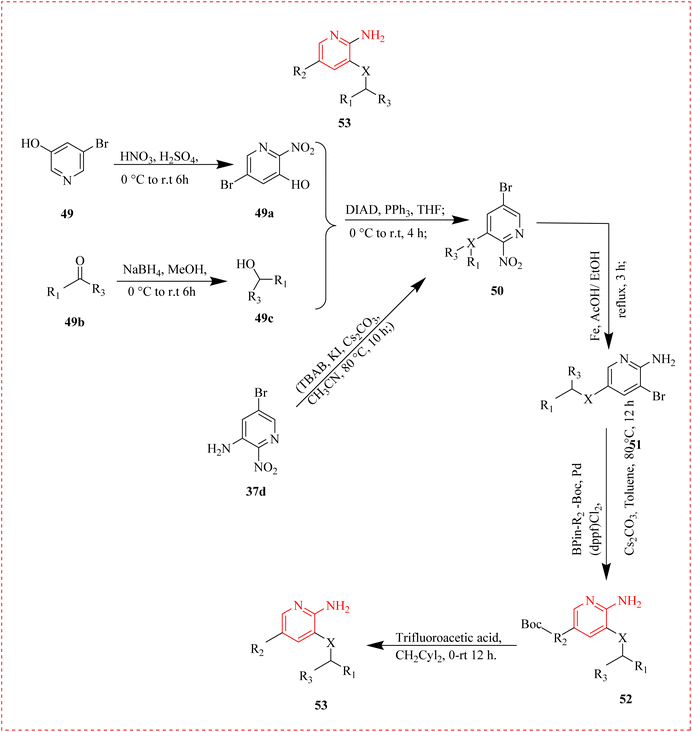 |
| Scheme 9 Synthesis of 3-methoxypyridine-2-amine derivatives. | |
Table 7 The activitity prophiles of (54a), (54b) and ruxolitinib against JAK2 and their antiproliferative activity
Compound |
Against JAK2 (IC50 μM−1) |
Antiproliferative (IC50.μM−1) |
54a |
0.092 ± 0.007 |
15.024 ± 0.032 |
54b |
0.572 ± 0.020 |
4.914 ± 0.056 |
Ruxolitinib |
0.006 ± 0.0001 |
7.639 ± 0.363 |
Munir et al., examined how 2-AP compounds bind when used as JAK2 inhibitors. Credible 3D-quantitative structure–activity relationship (QSAR) models were created and validation showed that the models could be used to predict the bioactivities of JAK2 inhibitors. One hundred novel JAK2 inhibitors with increased potency were computationally developed using the structural criteria supplied by the model's contour maps. A 100 ns molecular dynamic (MD) simulation was performed on the chosen complexes, which helped to forward the investigation of the binding interactions. Positive outcomes were found for internal library compound in the initial in silico study. The conclusions provided insightful advice for the development of powerful and novel JAK2 inhibitors (Fig. 9).84
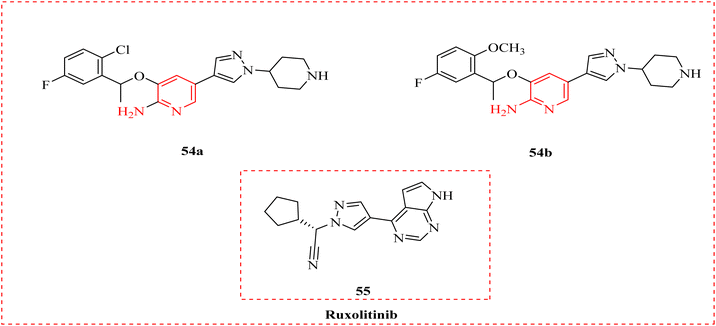 |
| Fig. 9 Structures of compound 3-methoxypyridine-2-amine (54a), (54b) and ruxolitinib. | |
2.6 Anti-neurodegenerative
Neurodegenerative disorders are characterized by loss of selectively vulnerable populations of neurons.85 4-AP was reported as a potential treatment for trigger point injection (TPI) using regular mouse models with functional outcomes including sensory indices, sciatic-functional-index and electro diagnostics. 4-AP has also improved nerve conduction velocity recovery, promoted remyelination, extended the quantity of axonal area after injury, and enabled rapid differentiation between nerve lesions. Accordingly, 4-AP provided a novel prospective therapy to enhance healing and remyelination in cases of significant peripheral nerve injury in addition to a special technique for detecting lesions where the therapy was most likely to be successful.86
The beneficial effects of 4-AP were validated, in promoting remyelination and the restoration of motor function, nerve conduction velocity, and sciatic nerve crush injury in mice. The sciatic nerve crush injury and no-injury groups of mice were divided into groups, and observed with or without 4-AP and saline treatment evaluating the skeletal muscle's morphological, functional, and transcriptional characteristics. 4-AP markedly decreased muscle atrophy while increasing muscle thickness and contractile force, in addition to enhance in vivo function. The results offered fresh perspectives on 4AP's possible therapeutic benefits in contradiction of nerve injury-induced muscle atrophy and dysfunction.87
Vasu et al., reported Blood–Brain Barrier penetration (BBB) of neuronal nitric oxide synthase (nNOS) inhibitors toward novel medications for neurodegenerative illnesses. The crystal structures of the majority of newly developed complexes complexed to different nitric oxide synthase isoforms were examined in addition to the inhibitor efficacy and selectivity. An efflux ratio of 0.8 in the Caco-2 bidirectional experiment indicated a considerably low substrate liability for P-glycoprotein and cancer resistant proteins, while a novel derivative (56) showed good potency (K < 30 nM) in nNOS inhibition. It was detrimental to increase their lipophilicity in order to increase permeability. As a result, they looked into the lead chemical 56's metabolic stability in human liver microsomes (HLM) and mouse liver microsomes (MLM). For HLM and MLM, terfenadine and imipramine employed as controls respectively. The findings showed that 56, with a half-life (t1/2) of 29 minutes, exhibited moderate stability in MLM. In contrast, both compounds showed excellent stability in HLM, with half-lives longer than 60 minutes (Fig. 10 and Table 8).88
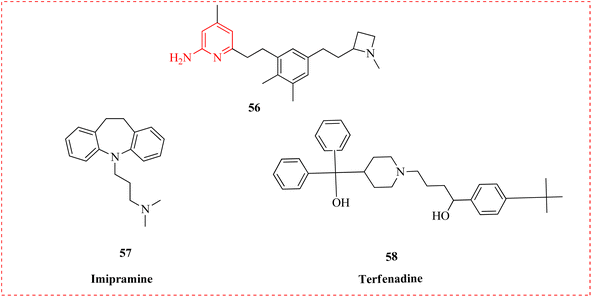 |
| Fig. 10 Structure of human neuronal NOS inhibitors having a 2-AP scaffold and the drugs. | |
Table 8 Metabolic constancy of nNOS inhibitors in mouse and HLM
Compounds |
Half-life (t1/2, min) MLM |
HLM |
56 |
29 |
>60 |
Terfenadine |
|
15 |
Imipramine |
9 |
|
2.7 Anti-tranquilizing and antidepressant activity
Antidepressants are common prescriptions that helps to treat depression and other conditions like anxiety89 and the tranquilizers are used to treat anxiety and insomnia.90 New compound arylmethylamino phenylpyridine for a tranquilizing activity was reported.91 Numerous derivatives with greater neurotropic activity compared to drugs Mexidol and Amitriptyline were recognized. In order to create new aminopyridone derivatives and to check them for nootropic activity, the synthesis of compounds 61 based on amino-methyl—phenylpyridine was performed. Pyridones 61a–c were obtained in higher yields by reacting pyridine-2-one 59 with a number of aldehydes by reduction of imines 60 with sodium borohydride in 2-propanol at room temperature (Scheme 10 and Fig. 11).92
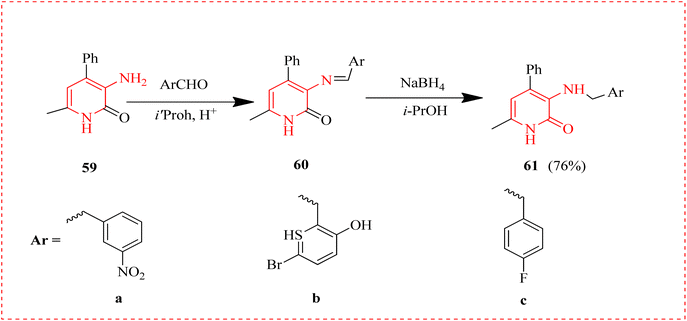 |
| Scheme 10 Synthesis of 3-(arylmethylamino) pyridones derivatives 61a–c. | |
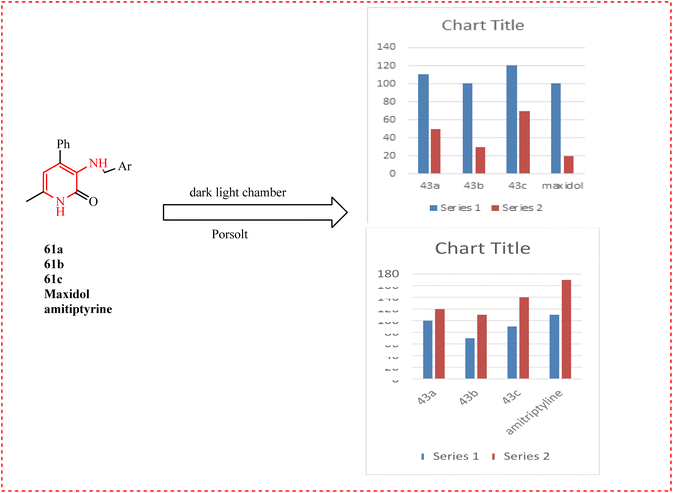 |
| Fig. 11 Graph presenting comparison of arylmethylamino methyl phenylpyridine and comparator drugs. | |
2.8 Anti-HIV
Human immunodeficiency virus, also called HIV damages the immune system so that the body is unable to combat disease. If HIV isn't treated, fails the immune system enough to become acquired immunodeficiency syndrome (AIDS).93 3-aminopyridine derivatives, on basis of the analogous chloroacetamide and condensed 1H-pyrido [2,3-b] [1,4] oxazine-2(3H)-one prepared by acylation reaction with chloroacetyl chloride. 3-Aminopyridine-2(1H)-one was reacted with several isothiocyanates to get thioureide derivatives. A cyclization of the derivative of carbamothioyl methacrylamide into substituted 1,3-thiazine was demonstrated. The biotesting phase of thiourea derivative 62 was conducted to determine its mode of inhibition of specific transcriptase types and its potential as an anti-HIV agent. 3-aminopyridine-2(1H)-one, acylates efficiently in CH2Cl2 in pyridine to form the chloroacetamide in a good yield. Replacing CH2Cl2 with DMF and an increase in temperature up to 80–100 °C lead to the cyclization of the intermediate 63 to 65 (Scheme 11).94
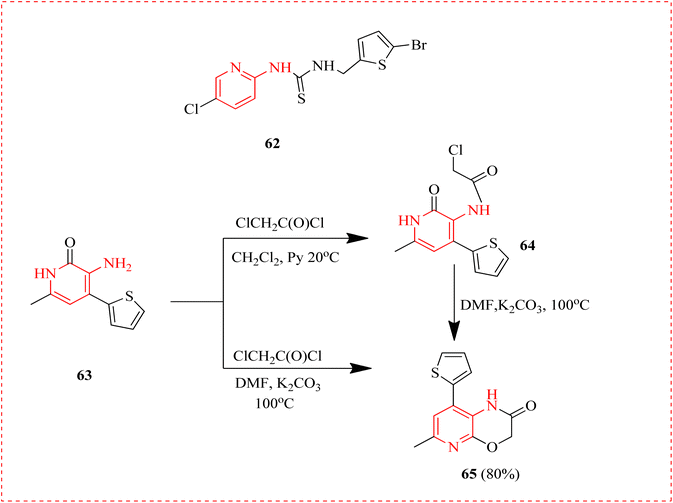 |
| Scheme 11 Synthesis of 1H-pyrido [2,3-b] [1,4] oxazin-2(3H)-one 65. | |
2.9 Muscular and neuronal activity
Govindappa et al., discussed strategies to improve the possible therapeutic outcome of 4-AP in PNI-induced bone loss and muscle repair. The adult male mice's right sciatic nerve, as well as the femoral and sciatic nerves innervating it, were crushed. Animals that had surgery were given 4-AP and regular saline. They discovered that 4-AP completely corrected trauma-induced alterations in muscle fibre type composition and greatly improved muscle morphology, cross-sectional area, and minimum ferret diameter from post-injury day 7. It also dramatically increased SFI, VFT, and hind limb paw grip strength. When compared to the saline group, the 4-AP-induced muscular effects were also linked to a much higher quantity of proliferating cells (Ki67+) and regenerating stem cells (Pax7+).95
Work on the use of thermogelling polymers to create a new local delivery system for 4-AP was reported. A thermosensitive formulation of a block copolymer (Poly-Lactic-Glycolic-Acid–Poly-Ethylene-Glycol (PLGA-PEG)) 68 was improved. Following a spinal nerve crush injury showed a marked improvement in motor and sensory functional recovery using a single dose of (4-AP)–PLGA–PEG. Additionally, immunohistochemistry analyses of injured nerves treated with (4-AP)-PLGA-PEG showed elevated neurofilament heavy expression. Using cerebral spheroids created from human induced pluripotent stem cells (iPSCs), examined 4AP effects on neuronal activity and related neurogenesis. They reported that 4-AP increases neuronal activity also enhanced the number of neurons and glial cells. These findings indicated that iPSC-derived brain spheroids were desirable for investigating several aspects of activity-induced neurogenesis (Scheme 12).96
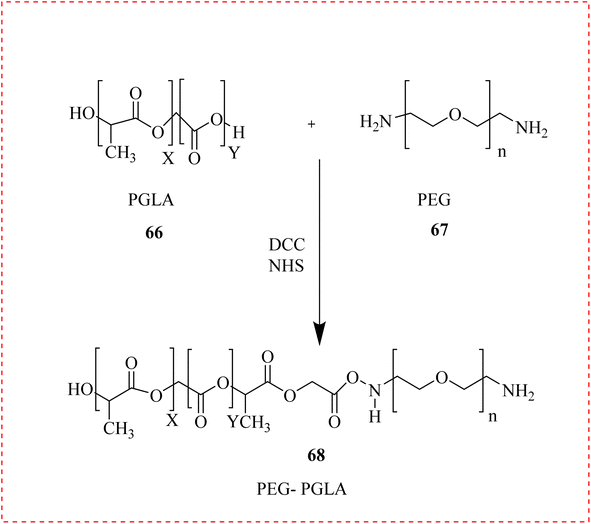 |
| Scheme 12 Synthesis of PGLA-PEG polymer. | |
Moreover, it was demonstrated that both dopamine depletion in the striatum and methyl-phenyl-tetrahydropyridine (MPTP) induced neuronal death in the sensory neurons were prevented by 4-AP. The rotarod test and open field test behaviour index verified that 4-AP reduced the motor impairments brought on by MPTP 69. Additionally, they demonstrated that the MPTP-induced rise in malonaldehyde (MDA) and drop in superoxide dismutase (SOD) levels could be considerably mitigated by 4AP therapy. Furthermore, 4-AP reversed the effects of MPTP-induced neurotoxicity on dopaminergic neurons by substantially reducing B-cell lymphoma-2 expression and increasing caspase-3 activation. According to these findings, 4-AP reduces oxidative stress and apoptosis in order to protect dopaminergic neurons against MPTP. This is a therapeutic agent for treatment of Parkinson disease (Fig. 12).97
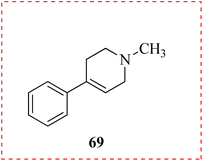 |
| Fig. 12 Structure of MPTP. | |
Yang Sun et al., discussed a new Kv1 channel tracer called [11C] methyl-4-aminopyridine ([11C]3Me4-AP) 75. Through the effective Stille cross-coupling of a stannyl precursor with a free amino group mediated by Pd (0)–Cu(I), [11C]3Me4-AP was produced. In rats and nonhuman primates, evaluation of [11C] 3Me4-AP's imaging properties revealed that it had slow kinetics and a moderate level of brain permeability. A one-tissue section model precisely simulated the regional brain time–activity curves, and further testing in monkeys shown that the tracer is metabolically stable. [11C] methoxy-4-aminopyridine ([11C]3MeO4-AP) 73 and [18F], ([18F]3F4-AP) 71, two similar tracers, are less common than [11C] with slower kinetics and lower initial brain absorption. That indicated reduced permeability to the blood–brain barrier. 3Me4-AP (75) suggests a higher binding affinity that is in line with in vitro studies. Conversely, the tracer's less desirable characteristics were brought about by the gentle kinetics and high binding affinity. That indicated reduced permeability to the blood–brain barrier and slower kinetics (Scheme 13).98
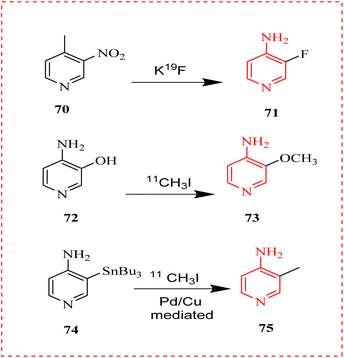 |
| Scheme 13 Synthesis of novel tracer for potassium Kv1 channels11 C 3Me4-AP (75). | |
2.10 Anticancer
Cancer is a class of disorders that can begin in any bodily tissue when aberrant cells proliferate out of control and spread to other bodily regions.99,100 Cancer is the leading causes of premature deaths globally.101,102 A new synthesized pyridine methyl naphthalene for cancer treatment against human lung (H-460) and breast (MCF-7) cell lines was reported. The ligand L (76) was prepared by reacting hydroxy-naphthaldehyde with 2-AP. The antidepressant activity was evaluated by raised plus maze model using Diazepam as reference. An IC50 of 23.99 μg mL−1 against H-460 and 31.68 μg ml−1 against MCF-7, respectively, demonstrated 89.20% anticancer activity at the maximal tested dose (500 μg mL−1) and recommended the compound's potential for application as an anticancer agent as well (Scheme 14).103
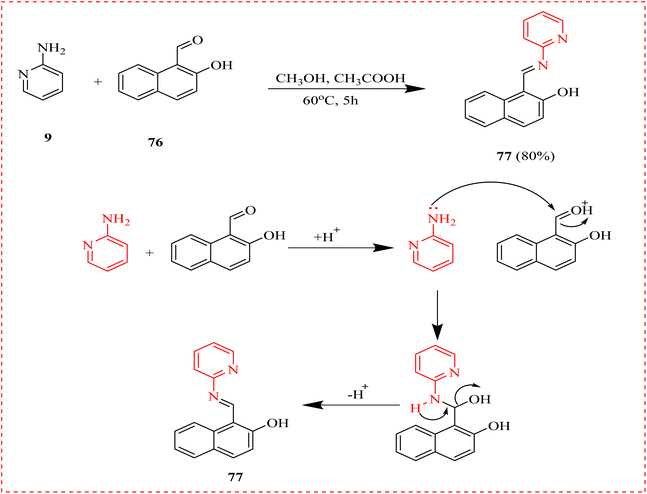 |
| Scheme 14 Synthesis of Schiff base ligand L 1-((pyridin-2-ylimino)methyl)naphthalene-2-ol (77). | |
Naz et al., reported the synthesis of amino acid conjugates of aminopyridine as potential anticancer agents. The parent (A2780) and cisplatin-resistant (A2780CISR) ovarian cancer cell lines were used to assess the cytotoxic activity. In contrast to parent cells, the results indicated that 82 and 83 demonstrated encouraging suppression in cisplatin-resistant cell lines in terms of both resistance factor and IC50 values (Table 7). Moreover, with IC50 values of 31.45 μM and 15.41 μM in the case of the parent and resistant cell lines, respectively, 83 was found to be the most active chemical. Docking experiments confirmed that compounds 82 and 83 exhibited significant binding affinities with several signalling cascade protein targets. In cisplatin-resistant cell lines, the anticancer properties of compounds 82, and 83 indicated that these ligands might serve as lead compounds for enhanced novel anticancer medications (Scheme 15 and Table 9).104
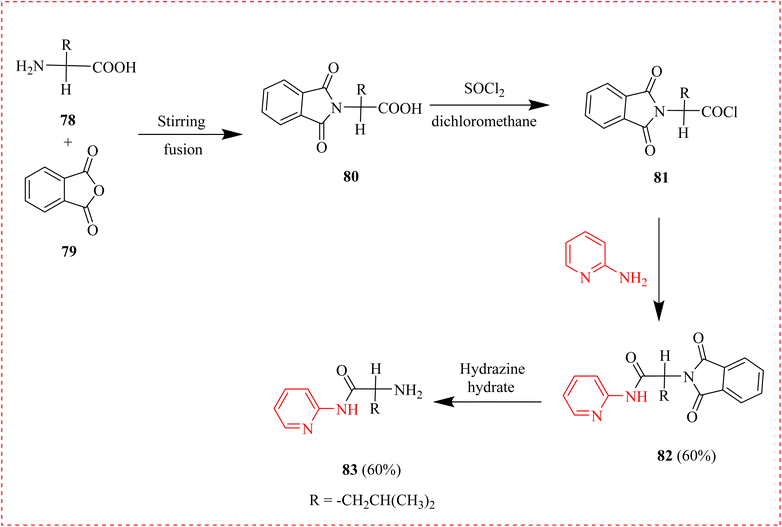 |
| Scheme 15 Synthesis of aminopyridine containing amino acid conjugates as potential anticancer agents. | |
Table 9 Anticancer potential of amino pyridine derivatives against A2780 (parent) and A2780CISR (cisplatin-resistant) ovarian cancer cell lines, expressed as IC50 values and compared to cisplatin, the standard drug
Tested compounds |
IC50 (μM) |
A2780* |
A2780CISR** |
Resistance factor |
82 |
39.14 ± 1.79 |
51.21 ± 1.65 |
1.31 |
83 |
31.45 ± 0.02 |
15.41 ± 1.52 |
0.49 |
Cisplatin |
0.61 ± 0.06 |
16.43 ± 1.45 |
26.93 |
An innovative selective CDK8 inhibitor against colon cancer discovered was reported in vivo. To be more precise, a number of novel 2AP derivatives were produced, and assessed using the structural details of the sorafenib-bound CDK8 structure. Of them, compound urea derivative 84 exhibited good selectivity and substantial inhibitory action against CDK8 with an IC50 value of 46 nM. Additionally, there appeared a relationship between biotinylated-81 and endogenous or overexpressed CDK8. This substance demonstrated antiproliferation efficacy on colon cancer cell lines with elevated levels of CDK8 expression, inhibited WNT/β-catenin activation and TCF family transcriptional activity, and caused G1 phase arrest in HCT-116 cells. Furthermore, this substance exhibited strong efficacy against HCT-116 cells that were resistant to Sorafenib (a potent cancer drug). Additionally, it demonstrated appropriate pharmacokinetic and minimal toxicity (Fig. 13).105
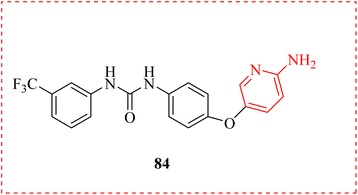 |
| Fig. 13 Structure of reported AP derivative. | |
Metal complexes related with organic ligands have gained much interest due to their structural variety and wide applications.106 Aminopyridines possess anticancerous potential through initiating ribonucleotide reductase, responsible for allowing them to target numerous cancerous diseases mainly gynecologic cancer.107 Mhadhbi et al., published the organic and inorganic fusion complex (2-HAP)2[CoBr4], where 2-HAP = 2-protonated aminopyridinium cation, as explained by a number of physicochemical techniques. Three phase transitions at T1 = 61.9 °C, T2 = 107.6 °C, and T3 = 172 °C were revealed by the differential scanning calorimetric method. Malignant Mat-Ly-Lu and Walker 256/B cell lines were used to assess the anticancer effects and dose response effect in Table 8. Using molecular docking, the antiviral properties of the organic portion of these hybrid compounds were investigated; notably, the binding projected energy was −4.0 vs. −5.3 kcal mol−1 for chloroquine that was used as reference. The drug-likeness, pharmacokinetics, molecular binding and chemo-preventive assays displayed that (2-HAMP)2[CoBr4] has encouraging biological potentials (Table 10).108
Table 10 Anticancer effect of (2-HAMP)2[CoBr4] on malignant Mat-Ly-Lu (prostate cells) and Walker 256/B mammary gland carcinoma cells
Dose-dependent concentration (μg mL−1) |
Proliferative inhibition (%) |
(2-HAMP)2[CoBr4] |
Malignant Mat-Ly-Lu |
Walker 256/B mammary glands |
CTRL |
0.9 |
1.2 |
H2O2 |
99.2 |
98.8 |
10 |
8.17 |
17.2 |
20 |
18.8 |
25.4 |
30 |
29.74 |
36.6 |
50 |
53.51 |
62.5 |
Luan et al., examined the anticancer properties of compact graphene oxide (HRGO) and gold nanomaterial (AuNM)-based (HRGO/Au@AP) nanocomposite for ovarian cancer, as well as the nanomaterials' ability to induce apoptosis and human ovarian cancer cell lines (SKOV3 and A2780). In order to increase the sample's solubility and bioavailability, HRGO was functionalized with 1AP as a potential stabilizing agent. Apoptosis assays, oxygen species measurements on SKOV3 and A2780 cells, and the findings of an anticancer investigation demonstrated a better capacity to trigger apoptosis. According to this study, HRGO/Au@AP may promote apoptosis in human ovarian cancer cells.109
Liu et al., examined new 2-AP derivatives produced and put through biological testing after being logically generated using molecular simulation. With an IC50 value of 42.3 nM, the chosen spiro derivative C01 demonstrated exceptional activity against CD74-ROS1G2032R cells, 30 times more effective than Crizotinib (cancer growth blocker) (85). Furthermore, C01 (86) exhibited a 10-fold higher potency than Crizotinib and potently suppressed enzymatic activity against clinically resistant ALKG1202R. The susceptibility of C01 to drug-resistant mutant was explained by molecular dynamic, which showed that adding the spiro group might lessen the steric hindrance with huge side chain (arginine) in solvent region of ROS1G2032R. These findings pointed to a future direction for the development of ROS1/ALK dual inhibitors resistant to Crizotinib (Scheme 16).110,111
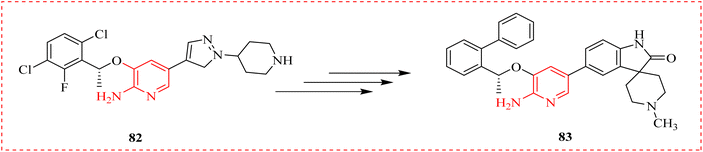 |
| Scheme 16 Novel 2-aminopyridine derivatives Crizotinib (82) and C01 (83) which was about 30 times more potent than Crizotinib. | |
2.11 Anti-tuberculosis
Tuberculosis (TB) is an infection that affects lungs. Caused by bacteria that spreads by air when infected people sneeze, cough or spit. A range of recently synthesized fluorinated chalcones 86, chosen them in vitro antitubercular action, along with its 2AP 3-carbonitrile and 2-amino 3-carbonitrile derivatives was revealed. Out of all synthesized compounds, compound 87b bearing indolyl scaffolds (MIC = 8 μM) had the highest potency. Its potency is comparable to that of broad-spectrum antibiotics such as streptomycin and ciprofloxacin, and it is three times more effective than pyrazinamide. When docking against thymidylate kinase, molecule 87b, which proved to be the most effective, displayed a binding energy of −9.67 kcal mol−1, which was compared with its in vitro MIC value (∼8 μM). The findings suggested that compound 82q, which is the most effective, exhibits selective activity against the H37Rv strain of Mycobacterium TB. Therefore, it is safe to process the molecule further in order to create a new antitubercular drug (Scheme 17).112
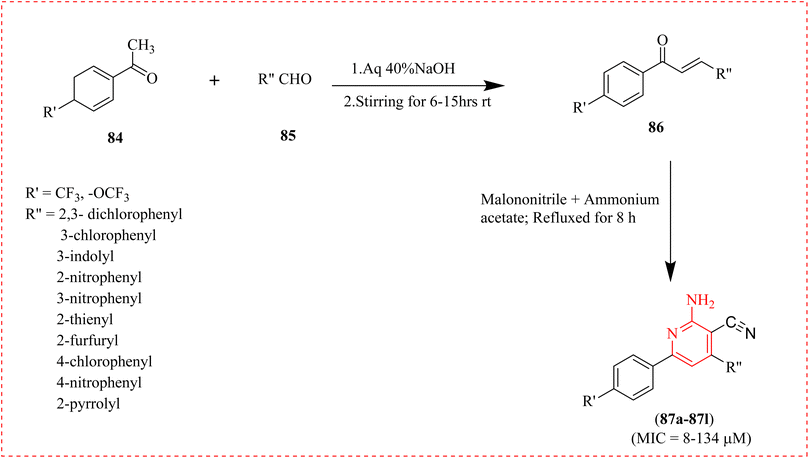 |
| Scheme 17 Synthesis of 2-amino-pyridine-3-carbonitrile derivatives effective against anti-tuberculosis. | |
2.12 Anti-epileptic
Epilepsy is most common serious brain disorder, affecting over 70 million people worldwide.113 Salazar et al., evaluated the effects of chronic intermittent hypoxia (CIH) on spread activity of the hippocampus in vitro, as well as whether these changes persisted or disappeared with normal oxygenation. According to the findings, giving adult rats CIH for 21 days magnifies 4AP-induced epileptiform activity in vitro and raises gamma-band hippocampal network activity. According to their research, CIH-induced changes in the hippocampal network and an increase in seizure was spontaneously reversed. This suggested that surgery or continuous positive airway pressure (CPAP), against obstructive sleep apnea (OSA) in epileptic patients, was beneficial for seizure control (Table 11).114
Table 11 Effects of chronic intermittent hypoxia CIH on body weight and mortality rate
Entries |
CTL |
CIH |
Statistics |
Initial body weight |
155.6 ± 1.7g |
154.2 ± 5.2g |
P= 0.24 (MW = 204.5, df = 20) |
Final body weight |
273.9 ± 4.1g |
258 ± 3.0g |
P < 0.001 (MW = 96, df = 20) |
Mortality rate |
0% (0/8 animals) |
16% (2/14 animals) |
P < 0.001 |
Debilitating diseases known as epileptic and developmental encephalopathies, which were characterized by seizures, intellectual impairment, and additional neuropsychiatric symptoms were reported. They demonstrated how the K+ channel blocker 4-aminopyridine has reduced current amplitudes in KCNA2-encephalopathy and increased transfected neuron firing rate to counteract gain-of-function deficiencies brought on by changes in the KV1.2 subunits in vitro. Except for two who continued to have induced seizures, all six of the patients who had daily absence, myoclonic, or atonic seizures ceased to have seizures. Six people with generalized tonic-clonic seizures displayed no change at all, one deterioration, and three clear improvements.115
Wang et al., employed two models of epileptic attacks: one based on 4-AP and used electrocorticography to confirm the length of an epileptic episode (ECoG). TPM impacted neurovascular coupling in the area of epileptic activity, according to LSCI and ECoG data. TPM significantly reduced the gamma power in ECoG for 4-AP-induced epileptic seizures (4AP + TPM: 0.61 ± 0.13 mV vs. 4-AP: 1.08 ± 0.19 mV; p < 0.05). Results confirmed that the instantaneous cortical LSCI and ECoG approach provided temporal and spatial information on the ictogenesis process and enabled the evaluation of pharmaceutical treatments' effectiveness in treating several types of epileptic seizures in vivo models (Fig. 14).116
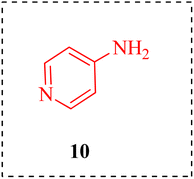 |
| Fig. 14 Structural prophiles for 4-AP. | |
Scalmani et al., conducted recordings in patients with temporal lobe epilepsy and in models of temporal lobe seizures, demonstrating the activation of interneurons at the beginning of focal seizures. Specific interneuron (IN) subtypes were acknowledged as parvalbuminergic (INPV), n = 17, cholecystokinergic (INCCK), n = 13, and somatostatinergic illogical characteristics. When 4AP-induced SLEs began, INPV and INCCK discharged, and their onset patterns were hyper-synchronous. After the SLE, pyramidal neurons were active with varying delays. 50% of the cells in each IN subgroup showed depolarizing block, which lasted longer in iN (4s) than in pyramidal neurons (1s). All IN produced action potential bursts synchronized with the field potential trials that caused SLE termination as the condition progressed. These findings implied that IN played a predominant role in the onset and initiation of focal seizures and corroborate previous in vivo and in vitro data (Fig. 15A and B).117
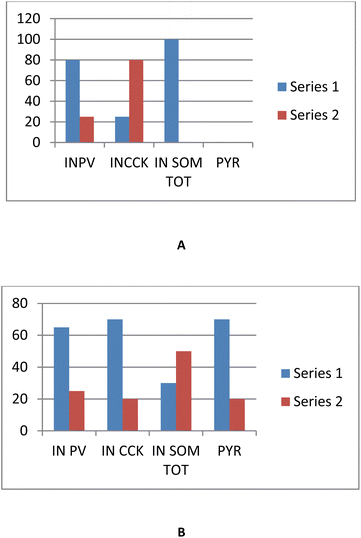 |
| Fig. 15 (A) Percentage of IN subtypes recoded in EC slices obtained from GAD65 (and GAD67 mice). (B) Percentage of IN subtypes and PYRs recorded from superficial EC layers 2–3 and deep EC layers 4 and 5. For statistical analysis, Fisher's exact test with Bonferroni's correction was used. | |
2.13 Anti-botulism
Botulism is a severe paralytic disorder caused by a neurotoxin produced by Clostridium botulinum.118 McClintic et al., described aminopyridines for treatments of botulism caused by botulinum neurotoxin (BoNT) that is an effective protein causing muscle paralysis and death by asphyxiation. When given consistently to mouse models of deadly botulism, the licensed medication 3,4-DAP (84) promptly reverses toxic indications of botulism and has antidote benefits. The effects of 3,4-DAP 84 and other AP on respiratory and ventilation during the later stages of botulism in mice were examined using unrestrained whole-body plethysmography (UWBP) and arterial blood gas measurements in combination (Fig. 16). A number of aminopyridines have therapeutic efficacies that were either superior to or equal to those of 3,4-DAP. These included aminopyridines that specifically increased tidal volume vs. respiratory level and vice versa (Fig. 17 and Table 12).119
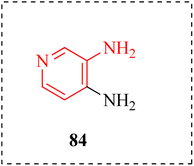 |
| Fig. 16 Structure of 3,4 diaminopyridine. | |
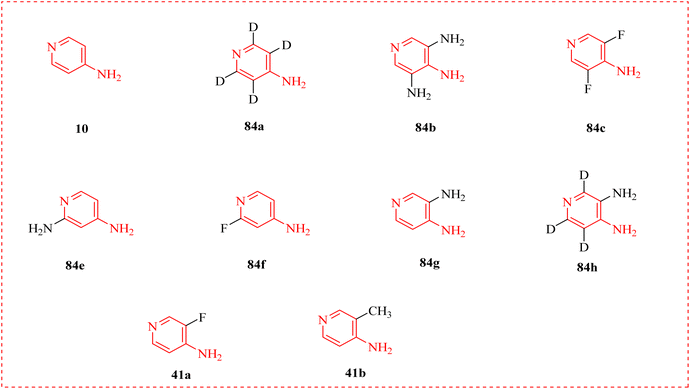 |
| Fig. 17 Evaluation of aminopyridine derivatives on ventilation. Structures of aminopyridines. 3,4-DAP is included for comparison. | |
Table 12 Summary of physicochemical properties120
Compounds |
MW |
log P |
pKa |
10 |
94.1 |
0.3 |
9.17 |
84a |
98.1 |
0.3 |
nd |
84b |
124.1 |
−1.2 |
9.08 |
84c |
130.1 |
0.4 |
5.12 |
84e |
109.1 |
−0.2 |
9.14 |
84f |
112.2 |
0.6 |
3.76 |
84g |
109.1 |
−0.5 |
9.17 |
41a |
112.1 |
0.3 |
7.19 |
41b |
112.2 |
0.5 |
9.43 |
3 Conclusion
Aminopyridines have emerged as a highly multifaceted and exceptional class of molecules with a wide array of biological activities and therapeutic potentials via targeting various enzyme receptors and ion channels. The recent advances in their synthesis have displayed innovative methodologies that enhance efficiency, selectivity, and yield, making these compounds more convenient for research and pharmaceutical applications. The biological activities of aminopyridines span a broad spectrum, including antibacterial, antifungal, anti-inflammatory effects, antiviral, anticancer, anti-botulism, anti-epileptic agents, anti-tuberculosis, anti-tranquilizing, antidepressants, anti-neurodegenerative, anti-multiple sclerosis, antitrypanosomal and anti-malarial agents. These activities underscore their potential as lead compounds in drug discovery and development. Structure–activity relationship (SAR) studies have been pivotal in understanding the mechanisms underlying these activities and have become significant for the rational design of more potent and selective aminopyridine derivatives. Key strategies, mainly transition metal-catalysed reactions, green chemistry approaches, and novel synthetic routes, have significantly contributed to the progress in this field. Despite the substantial progress, challenges remain, particularly in optimizing the pharmacokinetic and pharmacodynamics properties of aminopyridines to ensure their efficacy and safety in clinical profiles. Future research should focus on addressing these challenges, exploring novel synthetic methodologies, and expanding the scope of biological evaluations to fully harness the therapeutic potential of aminopyridines.
Data availability
No primary research results, software or code have been included and no new data were generated or analysed as part of this review.
Author contributions
T. Khalid: writing – review & editing. A. Malik: supervision, validation, and investigation. N. Rasool: validation, and investigation. A. Kanwal & H. Nawaz: review & editing. I. Almas: writing – review & editing.
Conflicts of interest
There are no conflicts to declare.
Acknowledgements
We strongly acknowledge the Organic material synthesis lab of Government College University, Faisalabad.
References
- S. Pearce, Drug Discovery, 2017, 67, 1–5 Search PubMed.
- K. Schofield, Hetero-aromatic Nitrogen Compounds: Pyrroles and Pyridines, Springer, 2013 Search PubMed.
- M. B. Islam, M. I. Islam, N. Nath, T. B. Emran, M. R. Rahman, R. Sharma and M. M. Matin, BioMed Res. Int., 2023, 2023, 1–15 Search PubMed.
- F. Islam, M. A. Hossain, N. M. Shah, H. T. Barua, M. A. Kabir, M. J. Khan and R. Mullick, J. Chem., 2015, 2015, 1–8 CrossRef.
- S. De, A. K. SK, S. K. Shah, S. Kazi, N. Sarkar, S. Banerjee and S. Dey, RSC Adv., 2022, 12, 15385–15406 RSC.
- C. Verma, K. Y. Rhee, M. Quraishi and E. E. Ebenso, J. Taiwan Inst. Chem. Eng., 2020, 117, 265–277 CrossRef CAS.
- X. H. Makhoba, Recent Developments in the Synthesis and Applications of Pyridines, 2023, pp. 143–158 Search PubMed.
- S. R. Alizadeh and M. A. Ebrahimzadeh, Mini Rev. Med. Chem., 2021, 21, 2584–2611 CrossRef CAS PubMed.
- N. Siddiqui, W. Ahsan, M. S. Alam, B. Azad and M. J. Akhtar, Res. J. Pharm. Technol., 2011, 4, 1918–1932 Search PubMed.
- S. Cherukupalli, R. Karpoormath, B. Chandrasekaran, G. A. Hampannavar, N. Thapliyal and V. N. Palakollu, Eur. J. Med. Chem., 2017, 126, 298–352 CrossRef CAS PubMed.
- R. Sahu, R. Mishra, R. Kumar, C. Majee, A. Mazumder and A. Kumar, Mini Rev. Med. Chem., 2022, 22, 248–272 CrossRef CAS PubMed.
- M. Sankara and L. Sidde, Int. J. Pharmacogn. Chem., 2020, 12–18 Search PubMed.
- A. Riedel, R. Lang, B. Rohm, M. Rubach, T. Hofmann and V. Somoza, J. Nutr. Biochem., 2014, 25, 750–757 CrossRef CAS PubMed.
- N. Shafiq, N. Shahzad, F. Rida, Z. Ahmad, H. A. Nazir, U. Arshad, G. Zareen, N. Attiq, S. Parveen and M. Rashid, Future Med. Chem., 2023, 15, 1069–1089 CrossRef CAS PubMed.
- P. Narendar, J. Parthiban, N. Anbalagan, V. Gunasekaran and J. T. Leonard, Biol. Pharm. Bull., 2003, 26, 182–187 CrossRef CAS PubMed.
- R. M. Mohareb, M. Y. Zaki and N. S. Abbas, Steroids, 2015, 98, 80–91 CrossRef CAS PubMed.
- L. M. Potikha and V. S. Brovarets, Chem. Heterocycl. Compd., 2020, 56, 1460–1464 CrossRef.
- Y. Ling, Z.-Y. Hao, D. Liang, C.-L. Zhang, Y.-F. Liu and Y. Wang, Drug Des. Dev. Ther., 2021, 4289–4338 CrossRef CAS PubMed.
- S. Malynych, I. Luzinov and G. Chumanov, J. Phys. Chem. B, 2002, 106, 1280–1285 CrossRef CAS.
- T. Chand and B. Savitri, Ind. Biotechnol. Vitam., Biopigm., Antioxid., 2016, 41–65 CAS.
- V. Gasperi, M. Sibilano, I. Savini and M. V. Catani, Int. J. Mol. Sci., 2019, 20, 974 CrossRef CAS PubMed.
- E. Anderson, Drugs, Labor, and Colonial Expansion, 2003, p. 159 Search PubMed.
- B. T. Green, S. T. Lee, K. E. Panter and D. R. Brown, Food Chem. Toxicol., 2012, 50, 2049–2055 CrossRef CAS PubMed.
- R. E. Dewey and J. Xie, Phytochemistry, 2013, 94, 10–27 Search PubMed.
- S. Worbs, K. Köhler, D. Pauly, M.-A. Avondet, M. Schaer, M. B. Dorner and B. G. Dorner, Toxins, 2011, 3, 1332–1372 CrossRef CAS PubMed.
- R. J. Bertz, A. Persson, E. Chung, L. Zhu, J. Zhang, D. McGrath and D. Grasela, Pharmacotherapy, 2013, 33, 284–294 CrossRef CAS PubMed.
- Y. Chen, X. Dong, Q. Wang, Z. Liu, X. Dong, S. Shi and H. Xiao, Front. Pharmacol, 2020, 11, 569843 CrossRef CAS PubMed.
- C. A. Calderón-Ospina and M. O. Nava-Mesa, CNS Neurosci. Ther., 2020, 26, 5–13 CrossRef PubMed.
- J. L. Griffin, S. A. Bonney, C. Mann, A. M. Hebbachi, G. F. Gibbons, J. K. Nicholson, C. C. Shoulders and J. Scott, Physiol. Genomics, 2004, 17, 140–149 CrossRef CAS PubMed.
- R. N. Rao and K. Chanda, Chem. Commun, 2022, 58, 343–382 RSC.
- M. J. Dias Pires, D. L. Poeira and M. M. B. Marque, Eur. J. Org Chem., 2015, 2015, 7197–7234 CrossRef CAS.
- S. Ekins, J. Mestres and B. Testa, Br. J. Pharmacol., 2007, 152, 21–37 CrossRef CAS PubMed.
- S. Momeni and R. Ghorbani-Vaghei, Sci. Rep., 2023, 13, 1–12 CrossRef PubMed.
- Q. Q. Lu, Y. M. Chen, H. R. Liu, J. Y. Yan, P. W. Cui, Q. F. Zhang, X. H. Gao, X. Feng and Y. Z. Liu, Drug Dev. Res., 2020, 81, 1037–1047 CrossRef CAS PubMed.
- L. Kang, X.-H. Gao, H.-R. Liu, X. Men, H.-N. Wu, P.-W. Cui, E. Oldfield and J.-Y. Yan, Mol. Diversity, 2018, 22, 893–906 CrossRef CAS PubMed.
- J. Chen, D. Yang, Y. Zhang, L. Yang, Q. Wang, M. Jiang and L. Pan, Int. J. Biol. Macromol., 2024, 259, 1–15 Search PubMed.
- F. Fastier and M. McDowall, Aust. J. Exp. Biol. Med. Sci., 1958, 36, 365–372 CrossRef CAS PubMed.
- J. L. Segal, J. F. Thompson and J. A. Tayek, Pharmacotherapy, 2007, 27, 789–792 CrossRef CAS PubMed.
- X. H. Gao, J. J. Tang, H. R. Liu, L. B. Liu and Y. Z. Liu, Drug Dev. Res., 2019, 80, 438–445 CrossRef CAS PubMed.
- A. M. King, N. B. Menke, K. D. Katz and A. F. Pizon, J. Med. Toxicol., 2012, 8, 314–321 CrossRef PubMed.
- V. I. Leussink, X. Montalban and H.-P. Hartung, CNS Drugs, 2018, 32, 637–651 CrossRef CAS PubMed.
- M. Malhotra, P. Ghai, B. Narasimhan and A. Deep, Arab. J. Chem., 2016, 9, S1443–S1449 CrossRef CAS.
- D. Moro-Sibilot, N. Cozic, M. Pérol, J. Mazières, J. Otto, P. Souquet, R. Bahleda, M. Wislez, G. Zalcman and S. Guibert, Ann. Oncol., 2019, 30, 1985–1991 CrossRef CAS PubMed.
- D. F. Heigener and M. Reck, Small Molecules in Oncology, 2018, pp. 57–65 Search PubMed.
- S. Lindquist and M. Stangel, Neuropsychiatr. Dis. Treat., 2011, 341–349 CAS.
- H. G. Piskorik, Analytical profiles of drug substances, Elsevier, 1985, 14, 107–133 CAS.
- C. P. Fitzsimons, F. Monczor, N. Fernández, C. Shayo and C. Davio, J. Biol. Chem., 2004, 279, 34431–34439 CrossRef CAS PubMed.
- K. K. Gaines, Urol. Nurs., 2004, 24, 207–209 Search PubMed.
- J. Hao, L. Brosse, C. Bonnet, M. Ducrocq, F. Padilla, V. Penalba, A. Desplat, J. Ruel and P. Delmas, Faseb. J., 2021, 35, e22025 CrossRef CAS PubMed.
- H. C. de Kraker, The University of Texas at San Antonio, 2022 Search PubMed.
- H. Li, C. Zhang, R. Fan, H. Sun, H. Xie, J. Luo, Y. Wang, H. Lv and T. Tang, J. Ethnopharmacol., 2016, 193, 117–124 CrossRef CAS PubMed.
- K. Jones, Five- and six-membered fused systems with bridgehead (ring junction) heteroatoms concluded : 6-6 bicyclic with one or two N or other heteroatoms, Polycyclic, Spirocyclic, Elsevier, 2008 Search PubMed.
- P. Bing, W. Zhou and S. Tan, Front. Pharmacol, 2022, 13, 1–10 Search PubMed.
- L. E. Kass and J. Nguyen, Wiley Interdiscip. Rev.:Nanomed. Nanobiotechnol., 2022, 14, e1756 CAS.
- L. Strękowski, Five- and six-membered fused systems with bridgehead (ring junction) heteroatoms including 6-6 bicyclic with one or two Nitrogen or other heteroatoms, Polycyclic, Spirocyclic, Elsevier, 2022 Search PubMed.
- M. Fioranelli, M. G. Roccia, D. Flavin and L. Cota, Int. J. Mol. Sci., 2021, 22, 5277 CrossRef CAS PubMed.
- E. R. Rayburn, S. J. Ezell and R. Zhang, Mol. Cell. Pharmacol., 2009, 1, 29 CrossRef CAS PubMed.
- M. Philip, D. A. Rowley and H. Schreiber, Semin. Cancer Biol., 2004, 433–439 CrossRef CAS PubMed.
- N. Hfidhi, I. Bkhairia, D. Atoui, J. Boonmak, M. Nasri, R. Ben Salem, S. Youngme and H. Naïli, Appl. Organomet. Chem., 2019, 33, e4793 CrossRef.
- J. Williams-Nguyen, J. B. Sallach, S. Bartelt-Hunt, A. B. Boxall, L. M. Durso, J. E. McLain, R. S. Singer, D. D. Snow and J. L. Zilles, J. Environ. Qual., 2016, 45, 394–406 CrossRef CAS PubMed.
- M. A. Salam, M. Y. Al-Amin, M. T. Salam, J. S. Pawar, N. Akhter, A. A. Rabaan and M. A. Alqumber, Healthcare, 2023, 1946 CrossRef PubMed.
- Z. Kibou, N. Aissaoui, I. Daoud, J. A. Seijas, M. P. Vázquez-Tato, N. Klouche Khelil and N. Choukchou-Braham, Molecules, 2022, 27, 3439 CrossRef CAS PubMed.
- U. S. F. Arrozi and S. Sugiarto, Indones. J. Chem., 2023, 23, 1108–1119 CrossRef.
- M. S. Al-Fakeh, M. A. Alsikhan and J. S. Alnawmasi, Molecules, 2023, 28, 2555 CrossRef CAS PubMed.
- K. J. Orie, P. J. Nna and R. U. Duru, Res. J. Pure Sci. Technol., 2024, 7, 96–107 Search PubMed.
- H. İlkimen and A. GÜLBANDILAR, Pamukkale UJ Eng. Sci., 2024, 1000, 1–7 Search PubMed.
- M. De Rycker, S. Wyllie, D. Horn, K. D. Read and I. H. Gilbert, Nat. Rev. Microbiol., 2023, 21, 35–50 CrossRef CAS PubMed.
- P. Büscher, G. Cecchi, V. Jamonneau and G. Priotto, Lancet, 2017, 390, 2397–2409 CrossRef PubMed.
- C. G. Veale, D. Laming, T. Swart, K. Chibale and H. C. Hoppe, ChemMedChem, 2019, 14, 2034–2041 CrossRef CAS PubMed.
- R. Silva-Oliveira, L. S. Sangenito, A. Reddy, T. Velasco-Torrijos, A. L. Santos and M. H. Branquinha, Trop. Med. Infect. Dis., 2023, 8, 1–15 Search PubMed.
- A. J. Thompson, B. L. Banwell, F. Barkhof, W. M. Carroll, T. Coetzee, G. Comi, J. Correale, F. Fazekas, M. Filippi and M. S. Freedman, Lancet Neurol., 2018, 17, 162–173 CrossRef PubMed.
- C. Walton, R. King, L. Rechtman, W. Kaye, E. Leray, R. A. Marrie, N. Robertson, N. La Rocca, B. Uitdehaag and I. van Der Mei, Mult. Scler. J., 2020, 26, 1816–1821 CrossRef PubMed.
- C. Bevan and J. M. Gelfand, Curr. Treat. Options Neurol., 2015, 17, 1–14 CrossRef PubMed.
- S. Rodríguez-Rangel, A. D. Bravin, K. M. Ramos-Torres, P. Brugarolas and J. E. Sánchez-Rodríguez, Sci. Rep., 2020, 10, 52 CrossRef PubMed.
- E. C. Aydoğmuş and G. I. Garip, Cancer Rep., 2024, 7, e70072 CrossRef PubMed.
- R. Abbas, H. Inayah, H. Hassan and F. F. Al-Kazzaz, Medico-Legal Update, 2021, 21 Search PubMed.
- M. Dietrich, H.-P. Hartung and P. Albrecht, Neurol. Neuroimmunol. Neuroinflamm., 2021, 8, e976 CrossRef PubMed.
- F. Türker, S. A. A. Noma, A. Aktaş, K. Al-Khafaji, T. Taşkın Tok, B. Ateş and Y. Gök, Monatsh. fur Chem., 2020, 151, 1557–1567 CrossRef.
- A. Shakeel, A. A. Altaf, A. M. Qureshi and A. Badshah, J. Drug Des. Med. Chem., 2016, 2, 1–12 Search PubMed.
- F. Rizvi, R. Ahmed, M. A. Bashir, S. Ullah, H. Zafar, A. tu-Wahab, H. Siddiqui and M. I. Choudhary, Future Med. Chem., 2023, 15, 1757–1772 CrossRef CAS PubMed.
- D. R. Niri, M. H. Sayahi, S. Behrouz, A. Moazzam, F. Rasekh, N. Tanideh, C. Irajie, M. S. Nezhad, B. Larijani and A. Iraji, Heliyon, 2023, 9, 1–11 Search PubMed.
- M. Sopjani, R. Morina, V. Uka, N. T. Xuan and M. Dërmaku-Sopjani, Curr. Mol. Med., 2021, 21, 417–425 CrossRef CAS PubMed.
- W. Wang, Y. Diao, W. Li, Y. Luo, T. Yang, Y. Zhao, T. Qi, F. Xu, X. Ma and H. Ge, Bioorg. Med. Chem. Lett., 2019, 29, 1507–1513 CrossRef CAS PubMed.
- N. Munir, T. A. Chohan, A. Qayyum, T. A. Chohan, F. Batool, M. W. Mustafa, S. Anwar, F. Alheibshy, W. Hussein and A. Alafnan, J. Biomol. Struct. Dyn., 2024, 1–16 CrossRef PubMed.
- H. Fu, J. Hardy and K. E. Duff, Nat. Neurosci., 2018, 21, 1350–1358 CrossRef CAS PubMed.
- M. Noble, K.-C. Tseng, H. Li and J. C. Elfar, Mil. Med., 2019, 184, 379–385 CrossRef PubMed.
- L. Yue, M. Talukder, A. Gurjar, J. I. Lee, M. Noble, R. T. Dirksen, J. Chakkalakal and J. C. Elfar, Muscle Nerve, 2019, 60, 192–201 CrossRef CAS PubMed.
- D. Vasu, H. T. Do, H. Li, C. D. Hardy, A. Awasthi, T. L. Poulos and R. B. Silverman, J. Med. Chem., 2023, 66, 9934–9953 CrossRef CAS PubMed.
- P. C. Gøtzsche and O. Dinnage, Int. J. Risk Saf. Med., 2020, 31, 157–163 Search PubMed.
- S. Kloiber and G. Konstantinou, in NeuroPsychopharmacotherapy, Springer, 2022, pp. 2037–2051 Search PubMed.
- B. Costall, B. Jones, M. Kelly, R. Naylor and D. Tomkins, Pharmacol. Biochem. Behav., 1989, 32, 777–785 CrossRef CAS PubMed.
- I. V. Palamarchuk, Z. T. Shulgau, M. A. Kharitonova and I. V. Kulakov, Chem. Pap., 2021, 75, 4729–4739 CrossRef CAS.
- G. A. C. Blood, Transfus. Med. Hemotherapy, 2016, 43, 203 CrossRef PubMed.
- I. Palamarchuk, Z. Shulgau, S. D. Sergazy, A. Zhulikeeva, T. Seilkhanov and I. Kulakov, Russ. J. Gen. Chem., 2022, 92, 1692–1705 CrossRef CAS.
- P. K. Govindappa, M. Jagadeeshaprasad, M. Talukder and J. Elfar, FASEB J., 2021, 35, 1–14 Search PubMed.
- T. Parmentier, F. M. James, E. Hewitson, C. Bailey, N. Werry, S. D. Sheridan, R. H. Perlis, M. L. Perreault, L. Gaitero and J. Lalonde, Sci. Rep., 2022, 12, 9143 CrossRef PubMed.
- L. Shi, L. Jia, Y. Wang, M. Xiu and J. Xie, Neurochem. Res., 2023, 48, 1707–1715 CrossRef CAS PubMed.
- Y. Sun, N. J. Guehl, Y.-P. Zhou, K. Takahashi, V. Belov, M. Dhaynaut, S.-H. Moon, G. El Fakhri, M. D. Normandin and P. Brugarolas, ACS Chem. Neurosci., 2022, 13, 3342–3351 CrossRef CAS PubMed.
- D. Paul, J. Cancer Metastasis Treat., 2020, 6, 1–31 Search PubMed.
- C.-H. Jiang, T.-L. Sun, D.-X. Xiang, S.-S. Wei and W.-Q. Li, Front. Pharmacol., 2018, 9, 1–13 CrossRef PubMed.
- F. Bray, M. Laversanne, E. Weiderpass and I. Soerjomataram, Cancer, 2021, 127, 3029–3030 CrossRef PubMed.
- Z. Guo, K. Guan, M. Bao, B. He and J. Lu, Pathol., Res. Pract., 2024, 260, 155460 CrossRef CAS PubMed.
- M. Sadia, J. Khan, R. Naz, M. Zahoor, S. W. A. Shah, R. Ullah, S. Naz, A. Bari, H. M. Mahmood and S. S. Ali, J. King Saud Univ., Sci., 2021, 33, 101331 CrossRef.
- S. Naz, F. A. Shah, H. Nadeem, S. Sarwar, Z. Tan, M. Imran, T. Ali, J. B. Li and S. Li, Drug Des., Dev. Ther., 2021, 1459–1476 CrossRef PubMed.
- Y. Y. Yan, X. X. Zhang, Y. Xiao, X. B. Shen, Y. J. Jian, Y. M. Wang, Z. H. She, M. M. Liu and X. H. Liu, J. Med. Chem., 2022, 65, 13216–13239 CrossRef CAS PubMed.
- K. Hchicha, M. Korb, R. Badraoui and H. Naïli, New J. Chem., 2021, 45, 13775–13784 RSC.
- R. Teran, R. Guevara, J. Mora, L. Dobronski, O. Barreiro-Costa, T. Beske, J. Pérez-Barrera, R. Araya-Maturana, P. Rojas-Silva and A. Poveda, Molecules, 2019, 24, 1–20 CrossRef PubMed.
- N. Mhadhbi, N. Issaoui, W. S. Hamadou, J. M. Alam, A. S. Elhadi, M. Adnan, H. Naïli and R. Badraoui, ChemistrySelect, 2022, 7, 1–9 CrossRef.
- W. Luan, M. Zheng, Y. Yang, Y. Chen, X. Zhang, L. Zhu and C. Lin, Gold Bull., 2023, 1–12 Search PubMed.
- S. Liu, C. Huang, C. Huang, Y. Huang, Y. Yu, G. Wu, F. Guo, Y. Jiang, S. Wan and Z. Zhu, J. Enzyme Inhib. Med. Chem., 2023, 38, 2227779 CrossRef PubMed.
- H. Li, Y. Wang, R. Fan, H. Lv, H. Sun, H. Xie, T. Tang, J. Luo and Z. Xia, Drug Des., Dev. Ther., 2016, 2173–2180 CAS.
- S. B. Lagu, R. P. Yejella, S. Nissankararao, R. R. Bhandare, V. S. Golla, B. V. Subrahmanya Lokesh, M. M. Rahman and A. B. Shaik, PLoS One, 2022, 17, 1–12 CrossRef PubMed.
- S. Balestrini, A. Arzimanoglou, I. Blümcke, I. E. Scheffer, S. Wiebe, J. Zelano and M. C. Walker, Epileptic Disord., 2021, 23, 1–16 CrossRef PubMed.
- B. Villasana-Salazar, R. Hernández-Soto, M. E. Guerrero-Gómez, B. Ordaz, G. Manrique-Maldonado, K. Salgado-Puga and F. Peña-Ortega, Epilepsy Res., 2020, 166, 106375 CrossRef CAS PubMed.
- U. B. Hedrich, S. Lauxmann, M. Wolff, M. Synofzik, T. Bast, A. Binelli, J. M. Serratosa, P. Martínez-Ulloa, N. M. Allen and M. D. King, Sci. Transl. Med., 2021, 13, eaaz4957 CrossRef PubMed.
- Y. Wang, V. Tsytsarev and L.-D. Liao, APL Bioeng., 2023, 7, 1–14 Search PubMed.
- P. Scalmani, R. Paterra, M. Mantegazza, M. Avoli and M. de Curtis, J. Neurosci., 2023, 43, 1987–2001 CrossRef CAS PubMed.
- Z. F. Dembek, L. A. Smith and J. M. Rusnak, Disaster Med. Public Health Prep., 2007, 1, 122–134 CrossRef PubMed.
- W. T. McClintic, Z. D. Chandler, L. M. Karchalla, C. A. Ondeck, S. W. O'Brien, C. J. Campbell, A. R. Jacobson and P. M. McNutt, J. Pharmacol. Exp. Ther., 2024, 388, 637–646 CrossRef CAS PubMed.
- W. T. McClintic, Z. D. Chandler, S. W. O'Brien, A. Jacobson and P. M. McNutt, Toxicon, 2024, 237, 107456 CrossRef.
|
This journal is © The Royal Society of Chemistry 2025 |
Click here to see how this site uses Cookies. View our privacy policy here.