DOI:
10.1039/D4RA07978G
(Paper)
RSC Adv., 2025,
15, 3273-3277
A facile synthesis of CF3-containing iminoisobenzofurans via copper-catalyzed oxygen trifluoromethylation–cyclization of o-vinyl-N-alkylamide†
Received
9th November 2024
, Accepted 13th December 2024
First published on 31st January 2025
Abstract
A copper-catalyzed oxygen trifluoromethylation of o-vinyl-N-alkylamide using Togni reagent as the trifluoromethyl precursor is demonstrated for the efficient synthesis of trifluoromethyl-containing iminoisobenzofurans. Preliminary mechanistic studies indicate that free radicals are involved in this process. The advantages of this approach include relatively mild reaction conditions and good functional group tolerance.
Introduction
Isobenzofurans are significant heterocyclic compounds that serve as a crucial reaction intermediate in the synthesis of numerous natural products and bioactive compounds.1–3 Notably, in recent years, iminoisobenzofurans, which are a crucial example of isobenzofurans,4,5 have emerged as highly attractive synthetic target compounds. The feasibility of traditional metal-catalyzed synthesis methods for these compounds, such as Pt- and Ag-/Au-catalyzed intramolecular cyclization of o-alkynylbenzamides and o-alkynylbenzohydroxamic acid derivatives, has been proven by many previous works.6,7 Based on these attempts, photo-induced cyclization and electrochemical reactions have also been realized for the synthesis of iminoisobenzofurans. For example, Lei et al. developed a mild and efficient electrochemical cyclization method for olefin amides, yielding benzamide, oxazoline, and iminoisobenzofuran compounds in high yields. Notably, the team discovered that this synthesis method operates effectively without the need for external oxidants or metal catalysts, thereby offering a novel approach for the preparation of iminoisobenzofurans. A similar synthetic approach was introduced for radical cascade cyclization of olefinic amides and diselenides, generating more than 40 examples of iminoisobenzofurans.8 Guo et al. exploited the regioselective and chemoselective O-cyclization or N-cyclization of olefin amides and diselenides to develop a new selenide imine isobenzofuran or isoindole via solar photocatalysis, facilitating the aerobic synthesis of indolones.9 Zhu et al. demonstrated a photo-induced construction approach to generate sulfonamidylated iminoisobenzofurans and benzoxazines via mild and metal-free synthesis by reacting 2-vinylbenzamides with N-(2-vinylphenyl) amides.10 Xiao et al. prepared diversely functionalized CF3-containing iminoisobenzofurans through a visible-light-induced photocatalytic radical addition/cyclization reaction, with satisfactory substrate scope and functional group tolerance.11 The aforementioned results demonstrate an advancement in the synthesis methods for iminoisobenzofurans. However, they also highlight several challenges associated with organic electrochemistry and photocatalytic synthesis, including slow reaction rates, high economic costs, and difficulties in controlling reaction conditions.12 Consequently, the development of novel catalysts for the synthesis of iminoisobenzofurans, building upon traditional synthesis methods, remains a valuable area for further investigation.
Trifluoromethyl groups (CF3) play an increasingly vital role in the fields of pharmaceuticals and agrochemicals owing to their unique structural motifs. They have a direct impact on the permeability, lipophilicity, and metabolic stability of the corresponding organic products.13–15 Consequently, in recent years, the incorporation of CF3 groups into organic molecules, particularly through the C-trifluoromethylation reaction, has become a prominent area of interest for researchers.16 Togni reagents, which are two trifluoromethyl-modified organic hypervalent iodine compounds, serve as effective electrophilic trifluoromethylation agents. They not only react with a variety of nucleophiles to facilitate efficient electrophilic trifluoromethylation but are also compatible with numerous sensitive functional groups, thereby broadening their applicability.17–19 Copper salts and their complexes serve as effective catalysts in the synthesis of a wide range of natural and synthetic compounds. Over the years, their efficacy has gained widespread recognition. In copper-catalyzed coupling reactions, particularly those that facilitate the formation of intramolecular oxy-trifluoromethylations, copper salts or complexes in various oxidation states are integral to the catalytic process.20–23 For instance, Buchwald et al. obtained cyclic lactones/ethers with satisfactory yields via Cu-catalyzed oxy-trifluoromethylations of unactivated alkenes.24 Building upon this foundation, the researchers further investigated the bifunctional trifluoromethylation of alkenes, specifically focusing on the amino-trifluoromethylation reaction, which simultaneously generates C–CF3 and C–N bonds. Notably, the intramolecular aminotrifluoromethylation reaction facilitates the construction of N-heterocycles through intramolecular cyclization, initiated by the trifluoromethylation of amine alkenes or alkynes. The resulting compounds have garnered significant interest from researchers as potential new agricultural chemicals.25,26 In 2016, Wang reported a copper-catalyzed aminotrifluoromethylation of o-vinyl-N alkoxyamide with the Togni reagent towards the synthesis of CF3-containing lactams.27 In addition, as for CF3-iminoisobenzofuran development, the exploration of efficient, green and mild synthesis methods is still challenging.
Based on the free radical addition/cyclization mechanism of alkenes, we designed an efficient synthetic method for CF3-containing iminoisobenzofurans, utilizing the Togni reagent as a CF3 source and a copper salt as the catalyst. This approach offers the advantage of employing a non-noble metal catalyst, while its reaction conditions are characterized by their simplicity, mildness, and efficiency.
Results and discussion
Optimization for the synthesis of iminoisobenzofurans
Initially, Togni's reagent (2a) was chosen as a CF3 radical precursor to verify the feasibility of the reaction, as shown in Table 1. Our investigation began by reacting CuI, 1,10-phenanthroline (phen), and CH3CN at 50 °C under a nitrogen atmosphere (Table 1, entry 1), resulting in the successful synthesis of the target product 3a with a yield of 52%.
Table 1 Optimization of the reaction conditionsa
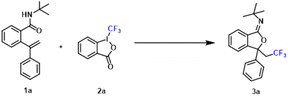
|
Entry |
[Cu] |
Ligand |
Solvent |
T (°C) |
Yieldb (%) |
Reaction conditions: N-(tert-butyl)-2-(1-phenylvinyl)benzamide (1a) (0.2 mmol), Togni's reagent (2a) (0.22 mmol), CuBr·Me2S (0.02 mmol), CH3CN (1 mL), 50 °C, N2, and 16 h. Isolated yields. THF, tetrahydrofuran; DCE, and 1,2-dichloroethane. |
1 |
CuI |
Phen |
CH3CN |
50 |
52 |
2 |
CuI |
— |
CH3CN |
50 |
56 |
3 |
— |
Phen |
CH3CN |
50 |
0 |
4 |
— |
— |
CH3CN |
50 |
0 |
5 |
CuBr |
— |
CH3CN |
50 |
48 |
6 |
Cu2O |
— |
CH3CN |
50 |
83 |
7 |
CuBr2 |
— |
CH3CN |
50 |
68 |
8 |
[(CH3CN)4Cu]PF6 |
— |
CH3CN |
50 |
57 |
9 |
C5H3CuO2S |
— |
CH3CN |
50 |
45 |
10 |
CuBr·Me2S |
— |
CH3CN |
50 |
91 |
11 |
CuOAc |
— |
CH3CN |
50 |
53 |
12 |
CuCl |
— |
CH3CN |
50 |
56 |
13 |
CuBr·Me2S |
— |
CH3CN |
r. t. |
40 |
14 |
CuBr·Me2S |
— |
CH3CN |
40 |
80 |
15 |
CuBr·Me2S |
— |
CH3CN |
60 |
88 |
16 |
CuBr·Me2S |
— |
THF |
50 |
42 |
17 |
CuBr·Me2S |
— |
DCE |
50 |
36 |
18 |
CuBr·Me2S |
— |
MeOH |
50 |
51 |
19 |
CuBr·Me2S |
— |
1,4-Dioxane |
50 |
41 |
For the proposed reaction conditions, parameters such as the metal catalyst, ligand, solvent, and temperature were systematically examined (Table 1). In entry 2, a higher yield of 3a (56%) was achieved without the addition of phen as the ligand reagent, indicating that the ligand was not essential for this reaction. Other copper metal catalysts were tested under the same conditions, with CuBr·Me2S demonstrating the highest effectiveness (entries 2–12). Adjusting the temperature, by increasing and decreasing it, did not yield satisfactory results (entries 13–15). Among the solvents screened, CH3CN provided the most favorable yield (entries 16–19). Additionally, it was confirmed that the inert atmosphere (N2) was crucial for achieving high reaction efficiencies. Encouraged by these findings, a reaction was conducted using N-(tert-butyl)-2-(1-phenylvinyl)benzamide (1a), Togni's reagent (2a), and CuBr·Me2S in CH3CN under a nitrogen atmosphere at 50 °C (oil bath), resulting in a high yield of 91% for the target product 3a (entry 10).
On the basis of determining the optimal reaction conditions, the practicability of this method was discussed. We first explored amides 1 with different substituents on the N atom, as shown in Table 2. Reactions involving amide substrates with alkyl groups (tert-butyl, isopropyl, n-butyl and n-hexyl) were investigated with accepted yields (Table 2, 3a–3da), and it was revealed that steric hindrance could affect the yield. Moreover, amide substrates bearing large steric hindrance groups (cyclopropanyl, cyclopentanyl and cyclohexanyl) could be obtained with good yields (Table 2, 3ea–3ga). Next, substituents with phenyl afforded better yields than alkynyl (Table 2, 3ha and 3ia). The good electron supply and conjugation properties of phenyl may have a positive effect on the yield of the product 3. Therefore, we varied the substituents on phenyl to demonstrate this effect. The position of the methyl substituent on the phenyl group has little effect on the yield of the product (Table 2, 3ma and 3oa), but the yield of the product will decrease if the electron-withdrawing group is used as the phenyl-substituted group (Table 2, 3pa and 3sa). In general, irrespective of whether aliphatic substituents or electron-absorbing substituents were used, the yields of the above products are all acceptable. Even when heterocycles (furan and thiophene) are used as substituents (Table 2, 3ta and 3ua), the method is still applicable.
Table 2 Synthesis of 3a–3ua a b
Reaction conditions: N-2-(1-phenylvinyl)benzamide 1 (0.2 mmol), Togni's reagent 2a (0.22 mmol), CuBr·Me2S (0.02 mmol), CH3CN (1 mL), 50 °C, N2, 16 h. Isolated yields. |
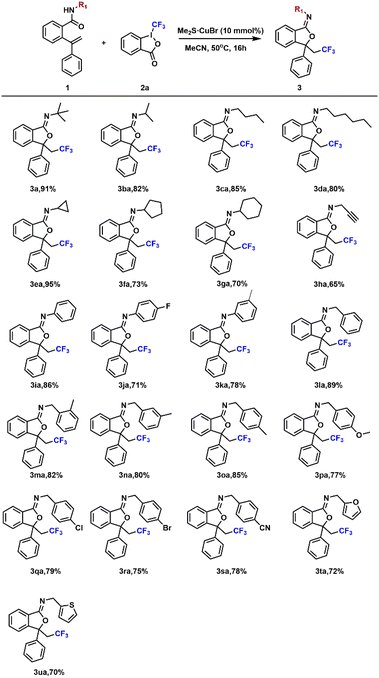 |
The types and positions of the substituted iminoisobenzofurans were discussed in Table 3, and all the compounds provide acceptable yields. Reactions of cyclopropanyl-substituted amides proceeded smoothly (Table 3, 3ab–3af), providing products in satisfactory yields, and the introduction of an electron-donating group (Me) could increase the yields of the products (Table 3, 3ab and 3ac). When an electron-drawing group such as –CN and –Br was present on the phenyl, the yields of the products could be improved, and the position of the electron-withdrawing group has little effect on the yield (Table 3, 3ad–3af). It is worth mentioning that the introduction of large steric groups (benzyl, tertiary butyl, phenmethyl and chlorobenzene) can offer corresponding products with higher yields (Table 3, 3ag and 3aj), demonstrating the universality of this reaction.
Table 3 Synthesis of 3ab–3aj a b
Reaction conditions: N-2-(1-phenylvinyl)benzamide (1) (0.2 mmol), Togni's reagent (2a) (0.22 mmol), CuBr·Me2S (0.02 mmol), CH3CN (1 mL), 50 °C, N2, and 16 h. Isolated yields. |
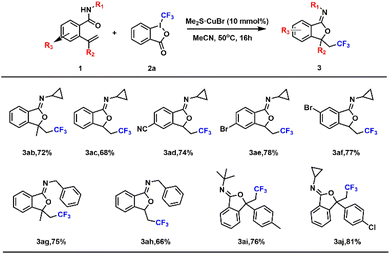 |
Proposed mechanism for the synthesis of iminoisobenzofuran
To gain insights into the potential pathway of copper-catalyzed oxytrifluoromethylation, we conducted a radical inhibition experiment (Scheme 1). In this standard reaction, the presence of 2.0 equivalents of radical scavengers, such as 2,2,6,6-tetramethylpiperidine 1-oxyl (TEMPO) and 2,6-di-tert-butyl-4-methylphenol (BHT), led to the complete absence of CF3-containing iminoisobenzofuran formation. These findings suggest that the reaction proceeds through radical species.
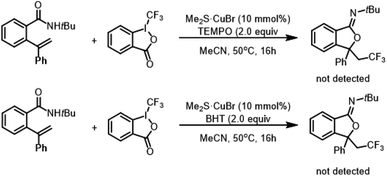 |
| Scheme 1 Radical inhibition experiments. | |
Based on the observations and literature reports, a plausible mechanism for this copper-catalyzed oxytrifluoromethylation was illustrated in Scheme 2. A single electron transfer (SET) between CuI and Togni's reagent generated the reactive CF3 radical. Subsequently, the CF3 radical attacked the C
C bond of substrate 1a, resulting in the formation of intermediate A, which was then oxidized by CuII to yield the benzylic carbocation B. Finally, an intramolecular nucleophilic attack followed by deprotonation led to the formation of the desired product 3a.
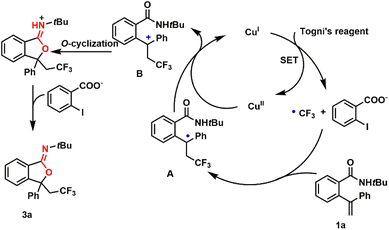 |
| Scheme 2 Proposed mechanism. | |
Experimental
In a typical synthesis of CF3-containing iminoisobenzofurans, N-(tert-butyl)-2-(1-phenylvinyl)benzamide (1a) (0.2 mmol, 1.0 equiv.), 2a (0.22 mmol, 1.1 equiv.) and 0.02 mmol of CuBr·Me2S (10 mmol%.) were dissolved in MeCN (1 mL). Thereafter, the mixture was stirred at 50 °C under an N2 atmosphere in the oil bath for 16 hours. Upon completion of the reaction, as indicated by thin-layer chromatography, the reaction mixture was concentrated using rotary evaporation. The resulting products were then purified via silica column chromatography, generally employing a solvent mixture of 50–70% petroleum ether in dichloromethane.
Conclusions
In summary, we developed a new method to directly synthesize various CF3-containing iminoisobenzofurans using Cu-catalyzed oxygen trifluoromethylation of N-alkylamide under mild and green reaction conditions. By further expanding the substrate range, we obtained 30 substrates and conducted mechanistic studies. The reported method opens up a new path for the green and mild preparation of iminoisobenzofurans.
Data availability
The data that support the findings of this study are available from the corresponding author, upon reasonable request.
Author contributions
Shuo Gao: investigation, data curation, and methodology. Qiwang Zhou: investigation and data curation. Zilin Liu: data curation. Kaiyuan Zhang: writing – original draft. Di Yang: supervision and writing – review & editing. Zhenhua Zhang: writing – review & editing, supervision, funding acquisition, and conceptualization.
Conflicts of interest
There are no conflicts to declare.
Acknowledgements
The authors thank the Natural Science Foundation of Shandong Province (ZR2022QB182, ZR2022QB254).
Notes and references
- Y.-J. Kwon, M.-J. Sohn, C.-J. Kim, H. Koshino and W.-G. Kim, J. Nat. Prod., 2012, 75, 271–274 CrossRef CAS PubMed.
- M. S. Abdelfattah, M. A. Arai and M. Ishibashi, Chem. Pharm. Bull., 2016, 64, 668–675 CrossRef CAS PubMed.
- M. K. Peters and R. Herges, Beilstein J. Org. Chem., 2017, 13, 2659–2662 CrossRef CAS PubMed.
- F. Wang, Y.-D. Wang, L.-C. Cai, Z.-W. Miao and R.-Y. Chen, Chin. J. Org. Chem., 2008, 28, 1523–1533 CAS.
- C. Pan, S. Luo, Y. Wu, J.-T. Yu and C. Zhu, Org. Chem. Front., 2023, 10, 3479–3484 RSC.
-
(a) B. Yao, C. Jaccoud, Q. Wang and J. Zhu, Chem.–Eur. J., 2012, 18, 5864 CrossRef CAS;
(b) Y. Madich, R. Alvarez and J. M. Aurrecoechea, Eur. J. Org Chem., 2014, 28, 6263–6271 CrossRef.
-
(a) D. Ding, T. Mou, J. Xue and X. Jiang, Chem. Commun., 2017, 53, 5279 RSC;
(b) X. Bantreil, A. Bourderioux, P. Mateo, C. E. Hagerman, M. Selkti, E. Brachet and P. Belmont, Org. Lett., 2016, 18, 4814 CrossRef CAS PubMed.
-
(a) F. Lu, J. Xu, H. Li, K. Wang, D. Ouyang, L. Sun, M. Huang, J. Jiang, J. Hu, H. Alhumade, L. Lu and A. Lei, Green Chem., 2021, 23, 7982–7986 RSC;
(b) H. Li, F. Lu, J. Xu, H. Alhumade, L. Lu and A. Lei, Org. Chem. Front., 2022, 9, 2786–2791 RSC.
- J. Hua, M. Bian, T. Ma, M. Yang, W. He, Z. Yang, C. Liu, Z. Fang and K. Guo, Catal. Sci. Technol., 2021, 11, 2299–2305 RSC.
- C. Pan, S. Luo, Y. Wu, J.-T. Yu and C. Zhu, Org. Chem. Front., 2023, 10, 3479–3484 RSC.
- Z.-C. Liu, Q.-Q. Zhao, J. Chen, Q. Tang, J.-R. Chen and W.-J. Xiao, Adv. Synth. Catal., 2018, 360, 2087–2092 CrossRef CAS.
-
(a) Y. Yuan and A. Lei, Nat. Commun., 2020, 11, 802 CrossRef CAS PubMed;
(b) B. Ma, D. Wang, J.-K. Jin, Z. Li, Q.-J. Liang, M.-Y. Sun, D. Liu, L.-J. Liu, H.-X. Shu, J. Yang, D. Li and J. He, Angew. Chem., Int. Ed., 2023, 62, e202300233 CrossRef CAS.
-
(a) L. Zhu, Y. Fang and C. Li, Chin. J. Chem., 2020, 38, 787 CrossRef CAS;
(b) A. Studer, Angew. Chem., Int. Ed., 2012, 51, 8950–8958 CrossRef CAS PubMed;
(c) D. J. P. Kornfilt and D. W. C. Macmilan, J. Am. Chem. Soc., 2019, 141, 6853 CrossRef CAS PubMed.
- M. Inoue, Y. Sumii and N. Shibata, ACS Omega, 2020, 5, 10633–10640 CrossRef CAS PubMed.
- Y. Ogawa, E. Tokayashi, K. Hirai and N. Shibata, iScience, 2020, 23, 104167 CrossRef.
-
(a) S. Guo, D. LabuSalim and S. P. Cook, Angew. Chem., Int. Ed., 2019, 58, 704 Search PubMed;
(b) P. Zhang, H. Shen, L. Zhu, W. Cao and C. Li, Org. Lett., 2018, 20, 7062 CrossRef CAS.
- J. Charpentier, N. Fruh and A. Togni, Chem. Rev., 2015, 115, 650–682 CrossRef CAS.
- C. R. Pitts, D. Bornemann, P. Liebing, N. Santschi and A. Togni, Angew. Chem., Int. Ed., 2019, 58, 1950–1954 CrossRef CAS PubMed.
- F. Bruning, C. R. Pitts, J. Kalim, D. Bornemann, C. Ghiazza, J. Montmollin, N. Trapp, T. Billard and A. Togni, Angew. Chem., Int. Ed., 2019, 58, 18937–18941 CrossRef.
- H. Egami, R. Shimizu and M. Sodeoka, Tetrahedron Lett., 2012, 53, 5503 CrossRef CAS.
- P. G. Janson, I. Ghoneim, N. O. Ilchenko and K. J. Szabó, Org. Lett., 2012, 14, 2882 CrossRef CAS PubMed.
- Y. Yasu, T. Koike and M. Akita, Angew. Chem., Int. Ed., 2012, 51, 9567 CrossRef CAS.
- Y. Li and A. Studer, Angew. Chem., Int. Ed., 2012, 51, 8221 CrossRef CAS.
- R. Zhu and S. L. Buchwald, J. Am. Chem. Soc., 2012, 134, 12462–12465 CrossRef CAS PubMed.
- H. Mei, J. Han, K. D. Klika, K. Izawa, T. Sato, N. A. Meanwell and V. A. Soloshonok, Eur. J. Med. Chem., 2020, 186, 111826 CrossRef CAS PubMed.
- H. Mei, J. Han, S. White, D. J. Graham, K. Izawa, T. Sato, S. Fustero, N. A. Meanwell and V. A. Soloshonok, Chem.–Eur. J., 2020, 26, 11349 CrossRef CAS PubMed.
- K. Shen and Q. Wang, Org. Chem. Front., 2016, 3, 222–226 RSC.
|
This journal is © The Royal Society of Chemistry 2025 |
Click here to see how this site uses Cookies. View our privacy policy here.