DOI:
10.1039/D4VA00293H
(Critical Review)
Environ. Sci.: Adv., 2025,
4, 571-583
A review of research progress on prevention and control technologies for arsenic and cadmium composite pollution in paddy soil†
Received
26th July 2024
, Accepted 7th December 2024
First published on 12th December 2024
Abstract
Arsenic (As) and cadmium (Cd) are among the most common potentially toxic elements (PTEs) found in paddy soil, where they can easily transfer from soil to rice grains. The widespread contamination of paddy soil with As and Cd, either individually or in combination, poses a significant threat to food security and human health in China. As and Cd exhibit different behaviours in soil, making the simultaneous management of As–Cd composite pollution a major technical challenge for safe rice production. This review summarizes several practical techniques for synchronously controlling uptake and translocation of As and Cd in rice plants, including water management, soil passivation, leaching technology, electrokinetic remediation, phytoremediation, selection of low-accumulation rice varieties, and application of foliar inhibitors. The treatment effects, mechanisms, and constraints of each technique are analyzed, and the development directions of the main control technologies are proposed. It emphasizes the importance of developing regionally adaptive, comprehensive technology models to manage paddy soil co-contaminated with Cd and As and ensure the safe production of rice.
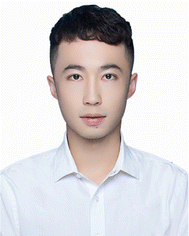 Hao Cui | Dr Hao Cui, a graduate of Southwest University, specializes in soil acidification and heavy metal remediation in karst farmland. He has published 12 papers in top international journals, including Science of the Total Environment and Journal of Analytical Atomic Spectrometry, with six as the first author (three in Q1 and three in Q2 journals). Dr Cui leads various research projects and participates in National Natural Science Foundation initiatives. He serves as a reviewer for Heliyon and the Chinese Journal of Analytical Chemistry and as a youth editorial board member for Atomic Spectroscopy. He has also presented at key conferences, including the Second National Soil Remediation Conference. |
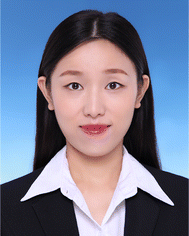 Lidan Lei | Li-Dan Lei obtained a PhD degree in Geochemistry from China University of Geosciences (Wuhan) and is currently employed full-time at Southwest University. Her major research interests lie in the field of environmental science, specifically the geochemical processes of environmental changes during geological periods and modern times. She has authored 20 academic papers and secured 2 invention patents. She serves as a reviewer for Heliyon. She has hosted and participated in 12 projects of various levels. |
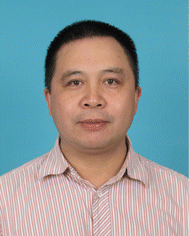 Songqing Liu | Dr Songqing Liu, a professor and master's supervisor, graduated from Sichuan Agricultural University and is recognized as an expert in the evaluation of undergraduate education and teaching by the Ministry of Education. He has long been engaged in research on farmland soil pollution remediation, horticulture, microbial resource development, and science popularization. He has led over 20 projects funded by the National Natural Science Foundation of China and the Sichuan Science and Technology Department and has published over 150 papers, including more than 40 SCI-indexed articles. |
Environmental significance
The remediation of potentially toxic elements (PTEs)in farmland is of paramount importance in environmental science. Firstly, employing methods such as low-accumulation rice varieties, phytoremediation, and passivation techniques effectively reduces the accumulation of PTEs in crops, thereby ensuring food safety and protecting human health. Secondly, these techniques decrease the mobility and bioavailability of PTEs in the soil, mitigating the risk of contamination to groundwater and surrounding ecosystems. Additionally, the application of PTEs remediation technologies restores and enhances soil health, promoting soil biodiversity and ecosystem stability. Innovations and systematic applications in the remediation process not only improve efficiency but also advance the fields of materials science, biotechnology, and other disciplines, fostering the sustainable development of environmental technologies. Overall, the remediation of PTEs in farmland is essential for achieving ecological environmental protection and sustainable agricultural development.
|
Rice (Oryza sativa L.) is China's most important crop, grown on about 25% of the cultivated area. The issue of excessive deposition of potentially toxic elements (PTEs) in rice occasionally poses a direct threat to food safety and human health. In several major grain-producing regions of China, cadmium (Cd) levels in rice exceed the standards in 10.3% of cases. Additionally, approximately 200
000 km2 of paddy fields is contaminated with PTEs such as cadmium (Cd) and arsenic (As).1 The presence of excessive PTEs in rice is closely related to soil pollution in paddy fields. In southern China, the rate of Cd contamination in agricultural land is 41.7%, while in northern China, it increases to 54.5%. Additionally, the overall rate of As contamination in farmland is 6.7%.2,3 In the major grain-producing area of the Pearl River Delta, more than 50.0% of farmland is threatened by toxic PTEs such as As and Cd. In the Yangtze River Delta, the rates of Cd and As contamination in paddy soil are 58.5% and 20.0%, respectively, and the main grain-producing area in western Chongqing is also threatened by As–Cd contamination.4,5 This shows that the As and Cd contamination is extensive and widespread across China. Moreover, rice has a strong ability to accumulate both As and Cd. Once the soil is polluted, the levels of As and Cd in rice grains can easily exceed the safety standard (0.2 mg kg−1).6 The situation regarding food safety is therefore concerning.
Toxic and hazardous PTEs in the environment inhibit the growth and development of rice. Cd, a toxic metal, is of particular concern as it is released into the environment and bioaccumulates in organisms through the food chain, leading to severe toxic effects.7 Numerous studies have demonstrated that excessive Cd accumulation in plants inhibits growth, damages cellular ultrastructure, and alters both primary and secondary metabolic processes. In soils, Cd exposure results in stunted rice root systems, leading to reduced plant height, lower yields, and in severe cases, total crop failure. Fan et al.8 reported that 0.5 M Cd reduced rice root growth by more than 40%, which was attributed to the degradation of the root cortex under Cd stress. High concentrations of cadmium (100 μM) are known to disrupt cellular membrane structures, including the nucleus, vacuoles, and mitochondria, and cause a reduction in nuclear size. Additionally, Cd-treated cells exhibit an increase in liposome formation, along with morphological deformation of nucleoli and chloroplasts. Studies also suggest that under flooded conditions, rice predominantly absorbs the inorganic arsenic species As(III) from the soil.9 Given that rice is typically cultivated under flooded and anaerobic conditions, prolonged waterlogging enhances the bioavailability of arsenic, making rice more susceptible to arsenic accumulation compared to other crops. When arsenic concentrations exceed 0.8 mg L−1, they significantly impact rice seed germination and root growth. In rice roots, 55–92% of the accumulated arsenic is sequestered in the cell wall rather than entering the vacuole and organelles, thereby inhibiting nutrient uptake. This disruption of nutrient absorption markedly impairs rice growth and development, potentially leading to desiccation and plant death.10 Thus, the safe management and remediation of paddy soil co-contaminated with As and Cd have become urgent priorities. This paper will focus on current major inhibition and control technologies, evaluating their effectiveness in simultaneously controlling As and Cd. It will specifically discuss the principles, application effects, and development trends of soil passivators and foliar inhibitors, which are the most frequently reported methods in this field. The aim is to provide technical references for managing soil co-contaminated with As and Cd in paddy fields.
1 Soil co-contaminated with As and Cd: control technologies
The control of PTEs in farmland soils primarily adheres to three principles:11 (1) altering the chemical forms of PTEs in the soil to reduce their bioavailability. (2) Using engineering techniques to lower the concentration or total amount of PTEs in the soil. (3) Changing the soil–crop system in severely polluted areas by growing non-food crops to reduce the transfer of PTEs into the food chain. The main types of technologies for this purpose can be categorized into physical, chemical, and biological methods.
1.1 Physical control technologies
1.1.1 Water management.
Water management is a common method for regulating the accumulation of PTEs in rice grains. The environmental behaviours of As and Cd in paddy soil during alternating wet and dry periods are almost completely opposite. During the flooding stage, dissimilatory sulfate reduction by sulfate-reducing bacteria converts SO42− to S2−, which then precipitates with Cd2+, thereby reducing the bioavailability of Cd2+.12 Additionally, under flooded and reducing conditions, especially when the redox potential is below −100 mV, Cd is released alongside the dissolution of iron and manganese oxides. These dissolved metals are absorbed by rice roots, entering the rice plants. During this process, Mn2+ and Cd2+ compete for transport proteins in the rice roots, resulting in a reduction in the absorption and transport of Cd2+ by rice root cells.13 During the drying stage, sulfur in CdS is oxidized to SO42−, releasing Cd2+ and increasing its bioavailability. Under these conditions, As(III) is oxidized to As(V), which is then extensively adsorbed by iron and manganese oxides, significantly reducing As-bioavailability; the basic mechanism is shown in Fig. 1. Besides their different responses to changes in soil redox potential, other factors also influence the activities of As and Cd. For example, Meng et al.14 found that modifying biochar to be rich in phosphorus to immobilize Cd2+ and Pb2+ actually activated As(III) in the soil. Similarly, Dong et al.15 developed Fe2O3-coated vermiculite to remediate soil contaminated with an As–Cd composite. This approach significantly reduced the available As content but only partially activated Cd. Studies have shown that alternating between flooding and drying conditions can reduce As content in brown rice by 41–45% compared to continuous flooding. However, this method can also increase Cd availability by more than 30%.16 Other research suggested that precise water management during the rice heading stage could effectively inhibit the accumulation of both As and Cd in rice grains.17 Precisely controlling soil Eh to −73 mV and pH to 6.2 through water management could achieve the simultaneous immobilization of As and Cd.18 However, achieving such precise control was often challenging in large-scale rice production. Therefore, applying specific passivators for As and Cd at different stages of water management in paddy fields could lead to more effective simultaneous treatment.
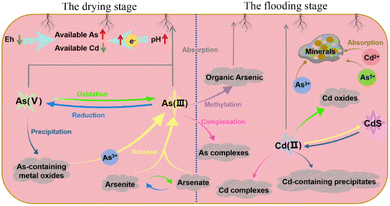 |
| Fig. 1 The different chemical behaviours of As and Cd during the flooding and drying stages in paddy soil. | |
1.1.2
Ex situ remediation technologies.
The ex situ soil replacement method involves replacing or diluting the contaminated in situ soil with uncontaminated soil. This can be achieved in three ways: first, by covering the contaminated soil with clean soil to create a new topsoil layer, thereby reducing the likelihood of crop roots coming into contact with the polluted soil; second, by thoroughly mixing clean soil with the original soil to dilute the contaminants to levels below the standard values; and third, by directly replacing the contaminated soil with clean soil.19 Additionally, the commonly used deep plowing method involves mixing uncontaminated subsoil with contaminated topsoil to reduce the concentration of PTEs in the plow layer. This method essentially follows the same principle as the ex situ soil replacement method. Deep plowing is particularly suitable for soils with mild pollution and significant surface accumulation of PTEs. The ex situ soil replacement method for heavy metal remediation has been applied both domestically and internationally for an extended period, demonstrating successful outcomes in regions such as the Zhangshi irrigation district in China and the Kanna River basin in Japan.20 Using the ex situ soil replacement method requires that the properties of the replacement soil are consistent with those of the original soil to support healthy crop growth. The thickness of the replacement soil should generally range from 15 to 30 cm, depending on the specific characteristics of the soil to address most needs. However, this method often faces limitations such as a shortage of clean soil sources and high costs. Additionally, it does not fundamentally remove PTEs from the soil, so it is most effective when combined with other chemical or biological technologies. For example, after electrokinetic remediation has concentrated PTEs in specific areas of the soil, the ex situ soil replacement method can be used to remove small portions of the contaminated soil. Alternatively, in situ excavation of contaminated soil can be followed by ex situ chemical remediation, with the remediated clean soil then refilled into the original site.21
1.2 Chemical treatment technologies
1.2.1 Soil leaching method.
Soil leaching, a physico-chemical remediation technique, involves using leaching agents or chemical additives to chelate, desorb, dissolve, or fix contaminants, thereby removing pollutants from the soil and achieving remediation. This technique is particularly effective for the rapid removal of total As and Cd PTEs from the soil.22 The commonly used soil leaching agents can be categorized as follows:23,24 (1) inorganic leaching agents, such as inorganic acids and bases; (2) heavy metal chelators, including ethylenediaminetetraacetic acid (EDTA), polyphosphate chelating agents, N-(2-hydroxyethyl)ethylenediamine triacetic acid (HEDTA), and dihydroxyethyl glycine (DEG); (3) surfactants, including inorganic surfactants such as Triton, and biosurfactants. Soil pH plays a crucial role in the leaching process of heavy metals, where an increase in the pH of the leaching agent typically results in a decrease in heavy metal concentration. Acidic pH conditions not only facilitate the mobilization of heavy metals but also inhibit the release of soil precipitates. However, the leaching process may have varying degrees of impact on soil enzyme activity, microbial diversity, and plant growth. EDTA is a widely used chelating agent, favoured for its ability to form more stable complexes with metals and its higher extraction efficiency.25 In field trials, following EDTA leaching treatment, the removal rates of As, Pb, Zn, and Cd reached 60%, 76%, 29%, and 53%, respectively.26,27 Additionally, combining EDTA with other agents can achieve the simultaneous removal of multiple heavy metals. For instance, compared to individual treatments (removal rates all exceeding 65%), the combined treatment of EDTA and tartaric acid improved the removal rates of Pb and Zn in contaminated farmland to 88.3% and 89.9%, respectively.28,29 Despite its effectiveness, EDTA's low biodegradability and prolonged retention in soils pose concerns, as long-term accumulation may lead to extensive dissolution of soil minerals and organic matter, potentially altering soil's physical and chemical properties and, in severe cases, even causing soil structure collapse, rendering it unsuitable for vegetation or construction. In contrast, naturally biodegradable chelating agents can effectively extract heavy metals under neutral or mildly alkaline conditions. For example, a mixture of dicalcium phosphate and cow manure significantly reduced the bioavailability of cadmium and zinc by 60% and 39%, respectively.30 Similarly, the use of dicalcium phosphate mixed with red soil has been reported for the remediation of Pb- and As-contaminated soils, with exogenous dicalcium phosphate promoting the fixation of Pb in red soil. The MPC binder demonstrated excellent immobilization efficiency for Pb (99.9%), though it was less effective for As. The addition of red mud enhanced the immobilization efficiency for As to 80.5%, due to strong complexation between AsO2− and Fe3+.31
1.2.2 Electrochemical treatment technology.
Electrochemical control involves inserting electrodes into polluted soil, applying direct current, and using soil pore water or added electrolytes to conduct electricity. During this process, pollutants migrate to designated areas through mechanisms such as electro-osmosis, electrophoresis, and electromigration.32 Currently, electrochemical control technology primarily focuses on two approaches: one uses conventional electrokinetic remediation principles to laterally migrate PTEs, thereby removing soil near the electrodes that is enriched with heavy metal ions; the other employs the principle of microbial fuel cells, where electrodes are placed vertically. This setup causes longitudinal migration of PTEs away from the rice root microenvironment, thereby reducing crop absorption of these target heavy metal ions.33 Electrochemical control technology offers notable advantages in terms of remediation effectiveness and operability, with removal rates for exchangeable PTEs reaching up to 95.0%.34 However, several limitations arise during the remediation process. On the one hand, hydrolysis can cause a “focusing effect” near the electrodes, leading to the crystallization and precipitation of metal ions.35 This reduces soil porosity and weakens the electric current intensity, ultimately impairing the migration of metal ions. On the other hand, electrodes are susceptible to corrosion under conditions of excessive acidity or alkalinity.36,37 To address these issues, researchers have proposed several optimization methods. For instance, using citric acid as a cathodic buffer solution can enhance the electrochemical effect. Compared to the non-strengthened group, the addition of citric acid neutralizes the OH− produced near the cathode, maintaining an acidic state throughout. This significantly improves the remediation effectiveness for Cd.38 Using nitric acid to control the pH of the cathode chamber allows for the recovery of 98.0% of Cd from kaolin, with a consumption of 13 liters of nitric acid and 30 kW h−1 of electricity per cubic meter of kaolin. This results in costs of 5.50 dollars per m3 for nitric acid and 2.31 dollars per m3 for electricity.39 Additionally, some researchers have employed the cathode proximity method (i.e., continuously approaching the fixed anode), which creates an alkaline environment that promotes the migration of As. Conversely, using the anode proximity method more effectively facilitates the migration of Cd.40 Electrochemical control technology has achieved relatively high levels of treatment for cations such as Cd2+, Pb2+, and Cu2+. However, the treatment effectiveness for variable-valence PTEs such as As and Hg remains unstable. Additionally, due to the opposite chemical properties of As and Cd, it is difficult to find an electrolyte that can simultaneously promote the migration of both PTEs. Some studies have shown that humic acids (HAs) can effectively enhance the efficiency of electrokinetic remediation for As. HAs can dissolve metal oxides that bind As or form ternary complexes with them, thereby releasing As. Furthermore, HAs can occupy adsorption sites on the solid phase of the soil through competitive adsorption, which also promotes the release of As.41,42 While HAs enhance the remediation effect, they do not alter other soil properties, thus reducing the risk of secondary pollution. Studies have also reported that low relative molecular weight fulvic acid (FA) can activate Cd in soil. Leveraging its ability to activate both PTEs, HAs may serve as an electrolyte enhancer for simultaneous electrokinetic remediation of As and Cd. As a new green technology, electrochemical control has been extensively researched at the laboratory scale and has shown successful cases in the remediation of site heavy metal-contaminated soil, sludge, and solid waste disposal. However, its application in large-scale farmland soil pollution remediation remains limited. Additionally, with the concept of carbon neutrality gaining traction, high-energy consumption methods may need improvement. For instance, using solar energy or bioelectricity for power supply can reduce energy consumption and costs, thereby increasing the market competitiveness of the method.
1.3 Biological control technologies
1.3.1 Phytoremediation technology.
Phytoremediation is also a current research direction for the remediation of soil co-contaminated with As and Cd. Plants have various mechanisms for dealing with pollutants, including absorption, detoxification or reduction of toxicity, immobilization of pollutants in soil, promotion of pollutant volatilization, degradation, and desalination.43 This technology has the advantages of low cost and environmental friendliness, making it a highly promising technique for soil remediation.44 In addition to relying on the inherent abilities of plants, various methods can be employed to enhance phytoremediation capabilities, further improving the efficiency and effectiveness of plant-based remediation.
The most widely applied technique in phytoremediation involves the use of hyperaccumulating plants to extract or sequester PTEs such as Cd and Pb from soil. However, this method has limitations, including land occupation, restricted sources of remediation plants, poor growth adaptability, and remediation efficiency being affected by soil properties and the extractability of PTEs. These factors result in long remediation cycles and high costs. Significant progress has been made in selecting remediation plants for soils contaminated with a single heavy metal. In addition to early reports of Cd and As hyperaccumulating plants such as Sedum alfredii and Solanum nigrum, many high-biomass ornamental plants and bioenergy crops are now also used for remediating soil heavy metal pollution. For instance, dandelion (Taraxacum officinale Hand.-Mazz.) and black nightshade (Solanum nigrum L.) have demonstrated effective remediation of Cd-contaminated soil, with Cd concentration in the shoots of both plants exceeding 100 mg kg−1.45,46
Recently, Ephedra equisetina has been found to exhibit superior Cd accumulation compared to Sedum alfredii, with a biomass 59 times greater. Therefore, its total Cd accumulation surpasses that of Sedum alfredii-like hyperaccumulating plants.47 Moreover, Ephedra equisetina demonstrates strong adaptability and stress resistance, offering a promising solution for remediating heavy metal-polluted soils where other remediation plants may struggle to grow.48 Furthermore, studies have found that while plants such as okra and grain amaranth have much lower accumulation rates compared to Sedum alfredii, and they can potentially match its total accumulation due to their high biomass. For As-contaminated soil, alternative plants such as rice, chia sage, and sweet sorghum (Sorghum bicolor L.) achieve only 15.4%, 38.0%, and 18.6% of the total accumulation seen in Sedum alfredii, respectively, and thus cannot replace it. Given the differing transport mechanisms for As and Cd in plants, finding species that can simultaneously remediate soil contaminated with both As and Cd remains a significant challenge. To address the issue and enhance the accumulation rate of remediation plants, researchers are developing auxiliary phytoremediation technologies aimed at finding or engineering plants capable of hyperaccumulating both As and Cd simultaneously. One approach to enhancing absorption efficiency is through genetic engineering. Various genes involved in transportation, metal chelation, compartmentalization, antioxidation, and metabolic regulation in plants affect the absorption, transport, and tolerance of As and Cd. Research has shown that overexpressing the heterologous gene PvACE3 (derived from Sedum alfredii, an As efflux transporter) in transgenic Arabidopsis thaliana increases its tolerance to As and promotes the translocation of As from roots to shoots. This results in a 7.5-fold increase in the As content in the aboveground parts compared to wild-type Arabidopsis thaliana. Additionally, miR156 has been identified as a key regulatory factor in plant development and stress response.49 Under Cd stress, overexpression of miR156 in Arabidopsis thaliana significantly elongates the primary root length, increases biomass and chlorophyll content, enhances antioxidant enzyme activity, reduces the level of reactive oxygen species (ROS), and decreases Cd accumulation. In contrast, silenced lines exhibit the opposite phenotype.50 Therefore, reducing the expression of miR156 in Arabidopsis thaliana can significantly enhance its Cd enrichment. By regulating these genes through genetic engineering, Arabidopsis thaliana may be developed as a preferable plant for the remediation of soil co-contaminated with As and Cd.
Another approach is the synergistic interaction between microorganisms and plants to improve the efficiency of phytoremediation. Compared to single-biological remediation modes, the combined plant–microbe remediation approach has greater potential. This synergy allows both plants and microorganisms to leverage their respective advantages, thereby improving the overall efficiency of heavy metal remediation. Rhizosphere microorganisms can directly enhance the phytoremediation process by altering soil pH, releasing chelating agents (such as organic acids, iron carriers, etc.), and participating in redox reactions.51 Li et al.52 used Fusarium solani FS10-C to enhance the remediation of Cd-contaminated farmland by Sedum alfredii. After 15 days of growth in simulated soil with a Cd concentration of 5 mg kg−1, the aboveground dry weight of the plants increased by 21.0%, and the Cd removal rate from the soil reached 35.3%. The results demonstrated that Fusarium solani FS10-C primarily promoted the growth of Sedum alfredii, thereby increasing its biomass and enhancing the absorption of Cd.53 Other studies have shown that arbuscular mycorrhizal fungi (AMF) can enhance the absorption of PTEs by hyperaccumulating plants. AMF improve plant colonization ability, promote nutrient absorption, and facilitate healthy growth, thereby increasing crop yield.54 The mechanism by which the mycorrhizal symbiotic system affects the accumulation of As and Cd in plants is complex. This includes the ability of AMF to fix As and Cd using mycelium, influence the biosynthesis of plant root cell wall components, thereby affecting the distribution of As and Cd, and alter the expression of As and Cd transport proteins.55 Lu et al.56 inoculated Mortierella sp. on the hyperaccumulating plant Sphagneticola calendulacea, resulting in an enhanced ability to transport Cd from roots to aboveground parts. This treatment also assisted Sedum alfredii in transporting As, thereby promoting the distribution of As to the aboveground parts.57
Due to the difficulty in screening for remediation plants with the capability to hyperaccumulate both As and Cd, intercropping, rotation, or relay cropping of heavy metal remediation plants with cash crops may help mitigate the shortcomings of single remediation plants.58 Projects have already adopted the method of intercropping Cd-hyperaccumulating plants with medicinal herbs such as Radix Paeoniae Alba (white peony root), achieving simultaneous soil remediation, crop production, and increased income. The Cd content of white peony root meets the standard, and the soil Cd content has decreased by more than 15.0%. The annual income is approximately twice that of planting food crops. Currently, this method has been applied to an area of 0.667 km2 (about 1000 acres) and has shown good results in Cd pollution remediation. Additionally, employing planting methods such as intercropping remediation plants with crops can address the issue of land occupation to some extent, alleviating the current pressure on food production in China while ensuring safe cultivation practices.
1.3.2 Hyperaccumulator plants for extraction of PTEs.
In practical applications, phytoremediation technology primarily utilizes hyperaccumulator plants to extract PTEs such as Cd and Pb from the soil. However, this method faces several limitations, including the temporary unavailability of contaminated arable land for cultivation, limited sources of suitable remediation plants, poor adaptability of plants to varying growth conditions, variability in remediation efficiency influenced by soil properties and heavy metal extractability, as well as long remediation periods and high costs. Currently, significant progress has been made in selecting remediation plants for soils contaminated with a single heavy metal. In addition to early reported hyperaccumulator plants such as Pteris vittata and Sedum alfredii for As and Cd, many high-biomass landscape plants and bioenergy crops have successfully been applied in practical soil heavy metal remediation.59,60 These new remediation plants not only enhance the diversity of plant sources but also demonstrate significant advancements in growth adaptability and remediation efficiency. For instance, Sedum alfredii Hance, a hyperaccumulator plant, is utilized for the removal of PTEs such as zinc (Zn) and Cd from farmland.61 Du et al.62 demonstrated that the addition of the super-engineered bacterium Rhodococcus qingshengii increased the absorption rates of Zn, Cd, nickel (Ni), and Pb by Sedum alfredii Hance by 112%, 105%, 46%, and 49%, respectively. After inoculation with R. qingshengii, a significant increase in the expression of all genes related to Zn, Cd, and Pb was observed, thereby enhancing the phytoremediation efficiency of Sedum alfredii Hance for these metals. In practice, hyperaccumulator plants often require the incorporation of soil amendments (such as biochar and animal manure) along with phosphate minerals (such as hydroxyapatite). These amendments reduce the potential toxicity of heavy metals to crops while enhancing the plants' tolerance and growth capabilities in adverse environments, thereby promoting and improving their ability to absorb and accumulate heavy metals. Salinthip Chunwichit et al.63 applied a commercial Calendula variety combined with added earthworm compost for the remediation of soil contaminated with Zn and Cd. Among the varieties tested, the “Dragon Yellow” variety exhibited the highest accumulation of Zn and Cd, demonstrating not only its effectiveness in in situ remediation of soil heavy metal pollution but also its potential to provide significant income for farmers.
Due to the challenge of selecting remediation plants with hyperaccumulation abilities for both As and Cd, intercropping, rotation, or mixed cropping of heavy metal remediation plants with economic crops may help mitigate the limitations of using a single remediation plant. An ongoing project has implemented intercropping of Cd-hyperaccumulator plants with the medicinal herb Bletilla striata, achieving simultaneous soil remediation, crop production, and increased income.64 The Cd content in Bletilla striata met all standards, and the soil Cd content decreased by more than 15.0%. Annual income doubled compared to growing grain crops, and this method currently covers an area of 0.667 square kilometres (about 1000 mu), demonstrating effective Cd pollution remediation. Additionally, adopting planting methods such as crop rotation and mixed cropping with remediation plants and agricultural crops helps address the issue of land occupation. These methods, while ensuring safe planting practices, also contribute to gradually reducing heavy metal content in the soil.
2 Synergistic passivation control materials
2.1
In situ soil passivants
Soil immobilization technology involves adding agents that immobilize PTEs in the soil, thereby altering conditions such as soil pH, redox potential, and rhizosphere microbial reactions. This process aims to reduce the bioavailability of PTEs, thereby decreasing their uptake by plants. Due to its convenience and ease of operation, this technique has become a standard practice in various heavy metal remediation scenarios, including the remediation of composite pollution involving As and Cd. The passivation mechanism of common soil passivators for PTEs in farmland is shown in Fig. 2.
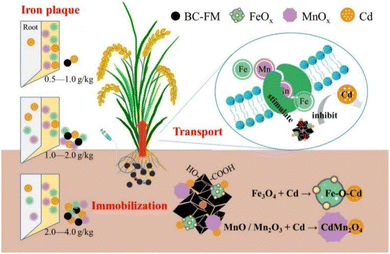 |
| Fig. 2 Schematic diagram illustrating the passivation mechanism of Fe–Mn-based soil passivators for Cd.65 | |
2.1.1 Inorganic passivation materials.
Inorganic composite passivators primarily include calcium (Ca), magnesium (Mg), sulfur (S), phosphorus (P), silicon (Si), selenium (Se), and iron (Fe). Due to the opposite chemical behaviors of As and Cd in the soil, it is challenging for a single inorganic soil immobilizer to effectively immobilize both As and Cd simultaneously.66 Common Cd immobilizers typically function by adjusting pH levels, such as lime and natural minerals like hydrotalcite. By raising the pH, they generate OH− or CO32− which precipitates with Cd, thereby reducing its bioavailability.67 Additionally, hydrotalcite not only alters soil pH but also utilizes Mg2+ to exchange with Cd2+ through homogeneous exchange, further reducing Cd bioavailability in the soil. Inorganic phosphorus-based soil immobilizers immobilize Cd through ion exchange, coordination bonds, and chemical co-precipitation.68 Studies have shown that using silicon-containing soil immobilizers can increase plant tolerance to Cd. The application of silicon not only reduces Cd transport in the apoplast and symplast of crops but also decreases the movement of Cd within plant cells through adsorption by polysilicic acid and silica gel.69 Additionally, monosilicic acid can directly precipitate Cd ions in soil solutions and plant tissue fluids. Huang et al.70 reported that the application of Se-enriched soil immobilizers could effectively reduce Cd content in rice grains by up to 65%. The results indicated that Se can mitigate the absorption and transport of Cd in rice plants.71 Se competes with Cd at the root level, reducing Cd uptake by rice plants. In paddy fields, the chemical behaviors of As and Cd are opposite; thus, traditional strategies to reduce Cd by altering pH may inadvertently activate As.
Currently, common methods for immobilizing As focus on elements such as Fe and Mn.72 These elements can reduce the bioavailability of As through the positive charges on the surface of Fe and Mn particles, thereby reducing the bioavailability of As and other anions. Additionally, the formed Fe–Mn nodules can adsorb As from the soil, reducing its mobility. The typical mechanism of immobilization is illustrated in Fig. 3. Wang et al.73 reported that oxidation can convert As(III) to As(V), thereby decreasing the mobility and bioavailability of As. Silicon-containing materials have a specific adsorption capacity for As and can regulate gene expression related to As transport, thus reducing the uptake and translocation of As in crops.74 The enhanced immobilization effect of modified biochar on As is mainly due to the introduction of elements such as iron and calcium. Mengyu Ma et al.75 reported that phosphogypsum, when used as a soil immobilizer, can remediate soil contaminated with As and Cd. It reduces the concentration of As in leachate from 25.75 μg L−1 to 5.88 μg L−1 and decreases the bioavailable content of Cd by 42%. This material decreases the activity of PTEs in the soil by increasing the specific surface area, introducing numerous hydroxyl groups, and enhancing electrostatic adsorption and co-precipitation. It exhibits different immobilization rates for various PTEs, with rates of Cu > As > Zn > Ni > Cd. Similarly, Pengle Cao et al.76 utilized nano-scale silica as a soil heavy metal immobilizer, modifying it with a silane coupling agent containing mercapto groups and ferrous (Fe(II)) salts. This modification resulted in a surface rich in S–Fe–S functional groups. Experimental results showed that this material effectively immobilizes PTEs in the soil, reducing the bioavailability of Pb, Cd, and As by 97.1%, 85.0%, and 80.1%, respectively.
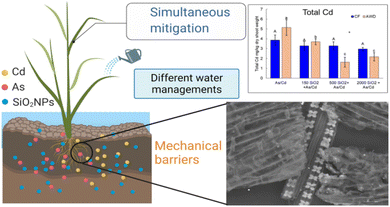 |
| Fig. 3 Simultaneous mitigation of arsenic and cadmium accumulation in rice seedlings by silicon oxide nanoparticles.73 | |
2.1.2 Organic soil passivators.
In recent years, organic materials have been widely used in the field of remediation of soils contaminated with cationic PTEs. Organic matter exhibits a strong spatial clustering characteristic with cationic PTEs such as Cd.77 Through a series of reactions, including ion exchange, adsorption, chelation, flocculation, and precipitation, it forms insoluble flocculated substances with Cd. Humic acid (HA), a natural organic soil passivator, primarily fixes heavy metal ions in the soil through the adsorption of coordination compounds formed between humic substances and heavy metal ions by clay minerals.78 Extensive experiments conducted by our research group have demonstrated that the molecular weight of humic acid directly affects the activity of PTEs in the soil. When the molecular weight of exogenously added humic acid exceeds 1000, it enhances the adsorption of PTEs by soil aggregates, thereby passivating the PTEs in the soil. However, when the molecular weight of exogenously added humic acid is less than 1000, the small molecules of humic acid can actually activate PTEs in the soil.79 Studies have indicated that the ratio of humic acid to fulvic acid (H/F) is a crucial indicator determining the activation effect of the active components of exogenously added humic acid on PTEs.79 With a constant content of exogenously added humic acid, when H/F ≥ 7/3, cationic PTEs in the soil preferentially convert to organically bound states with relatively lower activity, whereas when H/F ≤ 5/5, cationic PTEs in the soil convert to exchangeable and carbonate-bound states.
However, the effects of HA on As are multifaceted. Research by Duo-Rui Zhang et al.80 has shown that HA can promote the bio-oxidation of arsenopyrite surfaces and the immobilization of As. HA mainly facilitates the formation of flocculated porous structures composed of microbial cells and jarosite on the arsenopyrite surface, which can adsorb large amounts of As, inhibiting its release and activation. Jie Ma et al.81 demonstrated that HA can affect the adsorption of As by ferrous hydroxide gel in soil. Using a colloid and solute transport model to elucidate the adsorption mechanism of HA–ferrous hydroxide gel on As in porous media, they found that under alkaline conditions, HA forms a chain-like structure that couples with ferrous hydroxide gel, promoting its adsorption of As. Under neutral conditions, HA occupies the adsorption sites on the ferrous hydroxide gel for As, reducing the gel's adsorption capacity for As. Acidic conditions can disrupt the structure of the ferrous hydroxide gel itself, making it difficult to adsorb As.
The essence of soil passivation technology lies in adjusting the state of PTEs such as As and Cd in the soil through various mechanisms to reduce their bioavailability.82 However, it is important to note that this technology cannot fundamentally reduce the total amount of PTEs in the soil. Moreover, with changes in soil properties, there is a risk of PTEs being re-released. Bashir et al.83 mentioned that rice fields, as highly complex systems, often have additional fertilizers applied besides the passivators. In this process, passivated As and Cd are prone to secondary release. Furthermore, the market offers a wide variety of soil passivators, and China's soil types are extremely complex. There is still a lack of long-term effectiveness assessments and systematic evaluations of soil passivators, which severely restricts the choice and use by agricultural technicians. Therefore, it is necessary to propose scientifically reasonable and appropriate soil passivators tailored to specific conditions.
2.2 Leaf resistance and control of PTEs (As and Cd)
Foliar inhibitor technology involves applying physiological antagonistic elements or physiologically active substances, such as humic acid, directly to crop leaves. This method aims to alter the transport and distribution of PTEs within the plant, thereby preventing their translocation to the edible parts and improving the safety and quality of agricultural products. Not only is this approach easy to implement and suitable for large-scale field application, but it also demonstrates significant blocking effects.84 When applied appropriately, soil immobilization technology can also increase crop yield, making it a promising method for the safe utilization of farmland contaminated with PTEs. With the rapid development of drone technology, the cost of foliar application has decreased, making it a popular technique in field applications.85 In the national key research project undertaken by our research group (Demonstration of Comprehensive Prevention and Remediation Technologies for PTEs and Agricultural Non-Point Source Pollution in Southwestern Grain-Producing Areas, 2018YFD0800600), we combined foliar blocking agents with low-accumulation rice varieties, reducing Cd content in brown rice by 27–89%. The field demonstration maintained costs between 20 and 2000 yuan per mu, with a demonstration area in Chongqing covering 1200 mu and an extension area of 90
000 mu.
2.2.1 Traditional solution-based foliar spraying agents.
At present, the main foliar spraying agents are inorganic solutions, such as Si, Se, P, S, Mg, and Zn. Foliar spray agents are typically applied during the seedling stage, tillering stage, and flowering stage of rice, though there are reports indicating that they can also be applied during the grain-filling stage.86 These agents enhance the negative charge of plant cell walls, thereby increasing the plant's ability to fix PTEs. This process inhibits the translocation of PTEs between different tissues of the plant or forms compounds with PTEs in specific parts (such as roots, leaves, or stems) to co-precipitate.87 Additionally, it activates the plant's antioxidant defense mechanisms against PTEs. This mitigates the damage caused by PTEs to plant cells, improves photosynthesis, and enhances the absorption and transport of nutrients. Numerous reports have documented the remediation of farmlands contaminated with both As and Cd. For instance, Cui et al.37 reported that foliar spraying with a blocking agent containing Zn and Ge not only alleviated the toxicity of As and Cd to rice but also reduced their accumulation in rice grains. David Alexander et al.88 planted low-accumulation rice varieties in fields contaminated with Cd, Cu, Pb, and Zn. They sprayed the crops with a foliar blocking agent containing Si during the seedling, tillering, and flowering stages. After three applications, the results showed a significant reduction in the levels of Cd, Cu, Pb, and Zn in the rice grains, with Cd levels decreasing by 62%, effectively alleviating the stress of PTEs on the rice plants.
Additionally, the foliar application of trace elements such as boron (B), molybdenum (Mo), and selenium (Se) can not only effectively supplement the essential trace elements needed by crops and improve the quality of agricultural products but also exert antagonistic effects on PTEs, thereby mitigating their stress effects and reducing their accumulation in crops. For example, an appropriate concentration of selenium foliar fertilizer can inhibit the levels of Cd, Pb, and mercury (Hg) in strawberry and persimmon varieties. The mechanism involves effectively isolating heavy metal elements within the leaf cell walls through the foliar application of blocking agents, enhancing the resistance of rice to PTEs, and reducing or even blocking the transfer of PTEs into the food chain, as illustrated in Fig. 4. This method not only supplements trace elements but also provides a beneficial strategy for alleviating the problem of heavy metal pollution.89
Despite the simplicity, environmental friendliness, and relatively low cost of directly spraying foliar blocking agents, there are some limitations. First, plant leaves have a waxy cuticle, and when using ordinary inorganic solutions, over 95% of the foliar fertilizer does not enter the plant and is wasted.90 Additionally, high concentrations of inorganic solution-based foliar blocking agents can cause leaf burn, negatively impacting the plant's photosynthetic efficiency. Conversely, low concentrations of foliar fertilizers fail to achieve effective blocking, severely restricting the promotion and use of inorganic foliar blocking agents.91
2.2.2 Nanometer-scale solid foliar blocking agents.
With the continuous development and advancement of nanotechnology, nanometer-scale solid foliar blocking agents can be absorbed by plants and slowly released within the plant, achieving a slow-release effect. This approach aims to enhance rice's resistance to PTEs while reducing the frequency of spray applications, as depicted in Fig. 4,84 which illustrates the migration and transformation process of solid particulate foliar blocking agents within plant leaves. Nanometer-scale foliar blocking agents currently reported include ZnO-NPs, CuO-NPs, AgO-NPs, and CeO2-NPs. Marta Marmiroli et al.92 reported the use of CuO-NPs as a foliar blocking agent sprayed on zucchini leaves. CuO-NPs were detectable in the leaves from germination to flowering (within 60 days). This indicates that, compared to solution-based foliar blocking agents, solid nanometer-scale foliar blocking agents are more effectively retained in the leaves and can continuously release target elements, thereby affecting the plant throughout its lifecycle. Peng Zhang et al.93 demonstrated that CeO2-NPs help alleviate the stress of PTEs on rice. The continuous release of Ce3+ within the plant effectively enhances rice's resistance to extreme environments, such as low pH, prolonged flooding, or drought. Thea L. Read et al.91 compared the absorption and transport of ZnCl2 solution, Zn-EDTA, and ZnO-NPs as foliar blocking agents sprayed on wheat leaves. The results showed that inorganic ionic ZnCl2 is most easily absorbed and transported by the plant but can cause leaf burn, thereby reducing the photosynthetic capacity of the wheat leaves. Zn-EDTA adheres well to the leaf surface but lasts only 3–5 days, falling off to the ground after 7 days. ZnO-NPs can be absorbed by wheat leaves and stored in the mesophyll cells, continuously releasing Zn ions that are absorbed and transported by the wheat plant during growth. Currently, the physiological mechanisms by which foliar blocking agents alleviate the absorption and transport of PTEs in plants are not well understood, and their effectiveness is unstable. They are significantly influenced by plant species and weather conditions, and there is still a lack of feasibility reports for large-scale promotion.
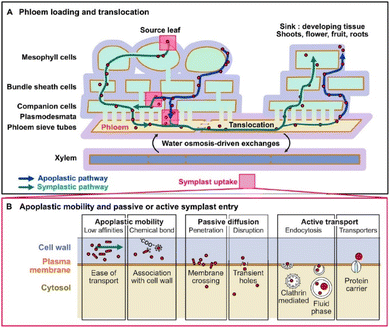 |
| Fig. 4 The migration and transformation process of solid granular foliar control agents in plant leaves.84 | |
In summary, multiple practical techniques have been explored for mitigating As and Cd contamination in rice, each with distinct mechanisms and efficacies. Table S1† summarizes the primary mechanisms and characteristics of these control methods.
3 Outlook
The effectiveness of different technologies varies based on their mechanisms, adaptability to soil–crop–climate conditions, and their impact on cost, implementation difficulty, and environmental friendliness. Overcoming these differences and maximizing the strengths of various technologies while addressing their limitations is crucial for the concurrent management of As and Cd in rice plants. For low-accumulation rice varieties, the focus lies in innovating selection methods and applying them systematically. This involves integrating modern molecular biology techniques with traditional botany and cultivation practices to rapidly screen for low-accumulation rice varieties while maintaining genetic stability. In plant remediation technology, efforts are directed towards enhancing efficiency, integrating remediation with utilization, reducing costs, and standardizing the entire process from seedling cultivation to post-harvest disposal and resource utilization. This includes developing comprehensive management techniques, reinforcing remediation methods, combining Cd and As remediation plant technologies, and implementing crop rotation and intercropping techniques involving remediation plants and rice. The development goal of immobilization technology is to achieve widespread application, high efficiency, low cost, environmental friendliness, long-term efficacy, and synergistic immobilization of multiple targets. Material modification, composite materials, and the development of new nano-materials are the primary means to achieve this goal. The efficacy of immobilization agents is closely related to soil properties. Therefore, in-depth research on the mechanisms of immobilization materials and the main soil property factors influencing their efficacy is crucial for selecting and applying these materials effectively. The application of foliar inhibitor materials is convenient and has seen rapid development in recent years. Key areas of focus include elucidating the physiological mechanisms of different types of materials, enhancing their adhesion and sustained release capabilities of active substances, and evaluating their safety.
4 Conclusion
(1) In summary, there are numerous technologies and methods available for the control and remediation of soil As and Cd, as well as their combined pollution. Among these, the main methods suitable for field-scale application include phytoremediation, water management, immobilization technology, plant remediation technology, screening and application of low-accumulation rice varieties, and foliar inhibitor technology. Techniques such as soil washing and electrokinetic remediation show potential for application but are mostly at the laboratory stage, with several feasibility issues to address before practical field-scale application.
(2) It is worth noting that soil PTEs pollution exhibits significant regional characteristics, influenced by variations in soil types, properties, pollution levels, climatic conditions, cropping systems, and cultivation practices across different regions. Therefore, directly applying highly effective technologies or models from one region to another is generally challenging. Additionally, different control technologies vary in mechanisms and efficiencies. A single technology may not fully achieve control objectives, especially in cases of severe or combined soil pollution, where multiple technologies are often required for synergistic benefits. Hence, the trend in comprehensive management of regional heavy metal pollution is to develop targeted technology models with high adaptability, based on regional habitat conditions and pollution characteristics.
Data availability
The data underlying this review paper, titled “A Review of Research Progress on Prevention and Control Technologies for Arsenic and Cadmium Composite Pollution in Paddy Soil” consist of previously published studies and publicly available datasets. All relevant data sources and references are cited within the manuscript. No new experimental data were generated for this review. Readers seeking additional information are encouraged to consult the original publications and datasets referenced throughout the paper.
Author contributions
Lidan Lei and Songqing Liu provided ideas and designed the review. Hao Cui, Rui Zeng, Fan Yang, Tao Han, and Bingbing Chen wrote the manuscript. All authors discussed the results and approved the final manuscript.
Conflicts of interest
There are no conflicts to declare.
Acknowledgements
This work was supported by the National Nature Science Foundation of Chongqing (CSTB2022NSCQ-MSX1453) and Sichuan Philosophy and Social Key Laboratory of Monitoring and Assessing for Rural Land Utilization (NDZDS202404).
Notes and references
- Y. Gao, Z. Duan, L. Zhang, D. Sun and X. Li, The Status and Research Progress of Cadmium Pollution in Rice- (Oryza sativa L.) and Wheat- (Triticum aestivum L.) Cropping Systems in China: A Critical Review, Toxics, 2022, 10, 794 CrossRef CAS.
- R. Li, J. Wang, Y. Zhou, W. Zhang, D. Feng and X. Su, Heavy metal contamination in Shanghai agricultural soil, Heliyon, 2023, 9, 22824 CrossRef.
- M. Afzal, S. Muhammad, D. Tan, S. Kaleem, A. A. Khattak, X. Wang, X. Chen, L. Ma, J. Mo, N. Muhammad, M. Jan and Z. Tan, The Effects of Heavy Metal Pollution on Soil Nitrogen Transformation and Rice Volatile Organic Compounds under Different Water Management Practices, Plants, 2024, 13, 871–921 CrossRef CAS PubMed.
- X. Wu and H. Zhang, Evaluation of ecological environmental quality and factor explanatory power analysis in western Chongqing, China, Ecol. Indic., 2021, 132, 108311 CrossRef.
- L. Ao, R. Chang, Y. Tang and S. Zhang, Ecological risk assessment and source tracing of heavy metals in surface sediments of a hilly riverine reservoir in Chongqing, China, Environ. Sci. Eur., 2024, 36, 69 CrossRef CAS.
- C. Peng, H. Song, Z. Zhao, X. Kuang, Y. Wang, S. Chen, Y. Chai, M. Bai and L. Peng, Foliar spraying with a mixture of transpiration inhibitor-rhamnolipid reduces the Cd content in rice grains, Sci. Total Environ., 2023, 885, 163844 CrossRef PubMed.
- S. P. McGrath, Keeping toxic cadmium out of the food chain, Nat. Food, 2022, 3, 569–570 CrossRef CAS PubMed.
- X. Fan, X. Wen, F. Huang, Y. Cai and K. Cai, Effects of silicon on morphology, ultrastructure and exudates of rice root under heavy metal stress, Acta Physiol. Plant., 2016, 38, 197 CrossRef.
- E. Byeon, H.-M. Kang, C. Yoon and J.-S. Lee, Toxicity mechanisms of arsenic compounds in aquatic organisms, Aquat. Toxicol., 2021, 237, 105901 CrossRef CAS PubMed.
- X. Wang, L. Liu, D. Liang, Y. Liu, Q. Zhao, P. Huang, X. Li and W. Fan, Accumulation, transformation and subcellular distribution of arsenite associated with five carbon nanomaterials in freshwater zebrafish specific-tissues, J. Hazard. Mater., 2021, 415, 125579 CrossRef CAS.
- Y. Wan, J. Liu, Z. Zhuang, Q. Wang and H. Li, Heavy Metals in Agricultural Soils: Sources, Influencing Factors, and Remediation Strategies, Toxics, 2024, 12, 63 CrossRef CAS.
- M. Lin, S. Ma, J. Liu, X. Jiang and D. Dai, Remediation of Arsenic and Cadmium Co-Contaminated Soil: A Review, Sustainability, 2024, 16, 687 CrossRef CAS.
- J.-D. Chang, S. Huang, N. Konishi, P. Wang, J. Chen, X.-Y. Huang, J. F. Ma and F.-J. Zhao, Overexpression of the manganese/cadmium transporter OsNRAMP5 reduces cadmium accumulation in rice grain, J. Exp. Bot., 2020, 71, 5705–5715 CrossRef CAS.
- X. Meng and R. Hu, Nitrogen/phosphorus enriched biochar with enhanced porosity activated by guanidine phosphate for efficient passivation of Pb(II), Cu(II) and Cd(II), J. Mol. Liq., 2021, 323, 115071 CrossRef CAS.
- Y. Dong, W. Zeng, H. Lin and Y. Yang, Preparation of Fe(2)O(3)-coated vermiculite composite by hydrophobic agglomeration and its application in As/Cd co-contaminated soil, Environ. Technol., 2022, 43, 83–94 CrossRef CAS.
- A. S. M. H. M. Talukder, C. A. Meisner, M. A. R. Sarkar, M. S. Islam, K. D. Sayre, J. M. Duxbury and J. G. Lauren, Effect of water management, arsenic and phosphorus levels on rice in a high-arsenic soil–water system: II. Arsenic uptake, Ecotoxicol. Environ. Saf., 2012, 80, 145–151 CrossRef CAS PubMed.
- P. Hu, J. Huang, Y. Ouyang, L. Wu, J. Song, S. Wang, Z. Li, C. Han, L. Zhou, Y. Huang, Y. Luo and P. Christie, Water management affects arsenic and cadmium accumulation in different rice cultivars, Environ. Geochem. Health, 2013, 35, 767–778 CrossRef CAS PubMed.
- T. Honma, H. Ohba, A. Kaneko-Kadokura, T. Makino, K. Nakamura and H. Katou, Optimal Soil Eh, pH, and Water Management for Simultaneously Minimizing Arsenic and Cadmium Concentrations in Rice Grains, Environ. Sci. Technol., 2016, 50, 4178–4185 CrossRef CAS PubMed.
- P. Song, D. Xu, J. Yue, Y. Ma, S. Dong and J. Feng, Recent advances in soil remediation technology for heavy metal contaminated sites: a critical review, Sci. Total Environ., 2022, 838, 156417 CrossRef CAS PubMed.
- P. Ji, Y. Song, T. Sun, Y. Liu, X. Cao, D. Xu, X. Yang and T. McRae, In-Situ Cadmium Phytoremediation using Solanum Nigrum L.: the Bio-Accumulation Characteristics Trail, Int. J. Phytorem., 2011, 13, 1014–1023 CrossRef CAS PubMed.
- X. Liao, Y. Li, R. Miranda-Avilés, X. Zha, J. H. H. Anguiano, C. D. Moncada Sánchez, M. J. Puy-Alquiza, V. P. González and L. F. R. Garzon, In situ remediation and ex situ treatment practices of arsenic-contaminated soil: an overview on recent advances, J. Hazard. Mater. Adv., 2022, 8, 100157 CAS.
- J. D. Aparicio, E. E. Raimondo, J. M. Saez, S. B. Costa-Gutierrez, A. Álvarez, C. S. Benimeli and M. A. Polti, The current approach to soil remediation: a review of physicochemical and biological technologies, and the potential of their strategic combination, J. Environ. Chem. Eng., 2022, 10, 107141 CrossRef CAS.
- H. Lin, Z. Wang, C. Liu and Y. Dong, Technologies for removing heavy metal from contaminated soils on farmland: a review, Chemosphere, 2022, 305, 135457 CrossRef CAS.
- P. Saengwilai, W. Meeinkuirt, T. Phusantisampan and J. Pichtel, Immobilization of Cadmium in Contaminated Soil Using Organic Amendments and Its Effects on Rice Growth Performance, Exposure Health, 2020, 12, 295–306 CrossRef CAS.
- T. Kameda, R. Suto, M. Tamzid Rahman, S. Kumagai, Y. Saito, Y. Nomura, D. Kawamura and T. Yoshioka, Extraction and enrichment of lead from contaminated soil using chelation reactions, Results Chem., 2024, 7, 101473 CrossRef CAS.
- J. F. Morales Arteaga, S. Gluhar, A. Kaurin and D. Lestan, Simultaneous removal of arsenic and toxic metals from contaminated soil: laboratory development and pilot scale demonstration, Environ. Pollut., 2022, 294, 118656 CrossRef CAS PubMed.
- G. Thalassinos, E. Levizou, J. Rinklebe, S. M. Shaheen and A. Vasileios, Enhancing the Phytoextraction of Cd, Cu, Pb, and Zn by Portulaca oleracea in a Heavily Contaminated Soil Using Low Molecular Weight Organic Substances: Is Phytoremediation Viable?, Earth Syst. Environ., 2024, 8, 923–936 CrossRef.
- S. Cheng, Q. Lin, Y. Wang, H. Luo, Z. Huang, H. Fu, H. Chen and R. Xiao, The removal of Cu, Ni, and Zn in industrial soil by washing with EDTA-organic acids, Arabian J. Chem., 2020, 13, 5160–5170 CrossRef CAS.
- J. Wang, J. Jiang, D. Li, T. Li, K. Li and S. Tian, Removal of Pb and Zn from contaminated soil by different washing methods: the influence of reagents and ultrasound, Environ. Sci. Pollut. Res. Int., 2015, 22, 20084–20091 CrossRef CAS.
- P. Saengwilai and W. Meeinkuirt, Cadmium (Cd) and zinc (Zn) accumulation by Thai rice varieties and health risk assessment in a Cd–Zn co-contaminated paddy field: effect of soil amendments, Environ. Geochem. Health, 2021, 43, 3659–3674 CrossRef CAS.
- L. Wang, L. Chen, B. Guo, D. C. W. Tsang, L. Huang, Y. S. Ok and V. Mechtcherine, Red mud-enhanced magnesium phosphate cement for remediation of Pb and As contaminated soil, J. Hazard. Mater., 2020, 400, 123317 CrossRef CAS PubMed.
- Z. Sun, M. Zhao, L. Chen, Z. Gong, J. Hu and D. Ma, Electrokinetic remediation for the removal of heavy metals in soil: limitations, solutions and prospection, Sci. Total Environ., 2023, 903, 165970 CrossRef CAS.
- W. Gustave, Z. Yuan, F. Liu and Z. Chen, Mechanisms and challenges of microbial fuel cells for soil heavy metal(loid)s remediation, Sci. Total Environ., 2021, 756, 143865 CrossRef CAS.
- J. Xu, C. Liu, P.-C. Hsu, J. Zhao, T. Wu, J. Tang, K. Liu and Y. Cui, Remediation of heavy metal contaminated soil by asymmetrical alternating current electrochemistry, Nat. Commun., 2019, 10, 2440 CrossRef PubMed.
- Y.-S. Ng, B. Sen Gupta and M. A. Hashim, Remediation of Pb/Cr co-contaminated soil using electrokinetic process and approaching electrode technique, Environ. Sci. Pollut. Res., 2016, 23, 546–555 CrossRef CAS.
- H. Cui, W. Guo, L. Jin, Y. e. Peng and S. Hu, Elemental screening of plant-based foods by slurry nebulization ICP-MS, J. Anal. At. Spectrom., 2020, 35, 592–599 RSC.
- Z. Z. Hao Cui, T. Wang, J. Hong, L. Lei and S. Wei, Uptake and Translocation of ZnO Nanoparticles in Rice Tissues Studied by Single Particle-ICP-MS, At. Spectrosc., 2023, 44, 343–353 CrossRef.
- A. Figueroa, C. Cameselle, S. Gouveia and H. K. Hansen, Electrokinetic treatment of an agricultural soil contaminated with heavy metals, J. Environ. Sci. Health, Part A: Toxic/Hazard. Subst. Environ. Eng., 2016, 51, 691–700 CrossRef CAS PubMed.
- J. O. Almeira, C.-S. Peng and A. Abou-Shady, Simultaneous removal of cadmium from kaolin and catholyte during soil electrokinetic remediation, Desalination, 2012, 300, 1–11 CrossRef.
- W. Yao, Z. Cai, S. Sun, M. Romantschuk, A. Sinkkonen, Y. Sun and Q. Wang, Electrokinetic-enhanced remediation of actual arsenic-contaminated soils with approaching cathode and Fe0 permeable reactive barrier, J. Soils Sediments, 2020, 20, 1526–1533 CrossRef CAS.
- J. Li, Y. Ding, K. Wang, N. Li, G. Qian, Y. Xu and J. Zhang, Comparison of humic and fulvic acid on remediation of arsenic contaminated soil by electrokinetic technology, Chemosphere, 2020, 241, 125038 CrossRef CAS PubMed.
- Y. Xu, Q. Lu, J. Li, L. Wan, S. Chen and Y. Lu, Effect of humus on the remediation of arsenic-contaminated soil by electrokinetic technology, Environ. Technol. Innovation, 2021, 21, 101297 CrossRef CAS.
- N. Sarwar, M. Imran, M. R. Shaheen, W. Ishaque, M. A. Kamran, A. Matloob, A. Rehim and S. Hussain, Phytoremediation strategies for soils contaminated with heavy metals: modifications and future perspectives, Chemosphere, 2017, 171, 710–721 CrossRef CAS.
- M. A. Ismael, A. M. Elyamine, M. G. Moussa, M. Cai, X. Zhao and C. Hu, Cadmium in plants: uptake, toxicity, and its interactions with selenium fertilizers, Metallomics, 2019, 11, 255–277 CrossRef CAS PubMed.
- H. Hammami, M. Parsa, M. H. Mohassel, S. Rahimi and S. Mijani, Weeds ability to phytoremediate cadmium-contaminated soil, Int. J. Phytorem., 2016, 18, 48–53 CrossRef CAS PubMed.
- S. Wei, Q. Zhou and U. K. Saha, Hyperaccumulative Characteristics of Weed Species to Heavy Metals, Water, Air, Soil Pollut., 2008, 192, 173–181 CrossRef CAS.
- P. B. Angon, M. S. Islam, S. Kc, A. Das, N. Anjum, A. Poudel and S. A. Suchi, Sources, effects and present perspectives of heavy metals contamination: soil, plants and human food chain, Heliyon, 2024, 10, e28357 CrossRef CAS PubMed.
- D. Zhang, M. Dyck, L. Filipović, V. Filipović, J. Lv and H. He, Hyperaccumulators for Potentially Toxic Elements: A Scientometric Analysis, Agronomy, 2021, 11, 1729 CrossRef CAS.
- Y. Chen, W. Xu, H. Shen, H. Yan, W. Xu, Z. He and M. Ma, Engineering Arsenic Tolerance and Hyperaccumulation in Plants for Phytoremediation by a PvACR3 Transgenic Approach, Environ. Sci. Technol., 2013, 47, 9355–9362 CrossRef CAS PubMed.
- L. Zhang, H. Ding, H. Jiang, H. Wang, K. Chen, J. Duan, S. Feng and G. Wu, Regulation of cadmium tolerance and accumulation by miR156 in Arabidopsis, Chemosphere, 2020, 242, 125168 CrossRef CAS PubMed.
- M. Rajkumar, S. Sandhya, M. N. Prasad and H. Freitas, Perspectives of plant-associated microbes in heavy metal phytoremediation, Biotechnol. Adv., 2012, 30, 1562–1574 CrossRef CAS.
- M. Li, H. Zhou, Y. Wangjin, M. Ye, X. Xu and X. Li, Remediation of Cd-contaminated soil by electrokinetics coupled with the permeable reactive barrier from immobilized yeast, Sci. Total Environ., 2023, 882, 163451 CrossRef CAS.
- A. Shi, J. Xu, Y. Shao, H. Alwathnani, C. Rensing, J. Zhang, S. Xing, W. Ni, L. Zhang and W. Yang, Salicylic Acid's impact on Sedum alfredii growth and cadmium tolerance: comparative physiological, transcriptomic, and metabolomic study, Environ. Res., 2024, 252, 119092 CrossRef CAS PubMed.
- M. Riaz, M. Kamran, Y. Fang, Q. Wang, H. Cao, G. Yang, L. Deng, Y. Wang, Y. Zhou, I. Anastopoulos and X. Wang, Arbuscular mycorrhizal fungi-induced mitigation of heavy metal phytotoxicity in metal contaminated soils: a critical review, J. Hazard. Mater., 2021, 402, 123919 CrossRef CAS PubMed.
- H. Li, M. Y. Gao, C. H. Mo, M. H. Wong, X. W. Chen and J.-J. Wang, Potential use of arbuscular mycorrhizal fungi for simultaneous mitigation of arsenic and cadmium accumulation in rice, J. Exp. Bot., 2021, 73, 50–67 CrossRef PubMed.
- R.-R. Lu, Z.-H. Hu, Q.-L. Zhang, Y.-Q. Li, M. Lin, X.-L. Wang, X.-N. Wu, J.-T. Yang, L.-Q. Zhang, Y.-X. Jing and C.-L. Peng, The effect of Funneliformis mosseae on the plant growth, Cd translocation and accumulation in the new Cd-hyperaccumulator Sphagneticola calendulacea, Ecotoxicol. Environ. Saf., 2020, 203, 110988 CrossRef CAS PubMed.
- E. Bona, F. Marsano, N. Massa, C. Cattaneo, P. Cesaro, E. Argese, L. S. di Toppi, M. Cavaletto and G. Berta, Proteomic analysis as a tool for investigating arsenic stress in Pteris vittata roots colonized or not by arbuscular mycorrhizal symbiosis, J. Proteomics, 2011, 74, 1338–1350 CrossRef CAS PubMed.
- S. Wu, C. Shen, Z. Yang, B. Lin and J. Yuan, Tolerance of Ricinus communis L. to Cd and screening of high Cd accumulation varieties for remediation of Cd contaminated soils, Int. J. Phytorem., 2016, 18, 1148–1154 CrossRef CAS.
- J. Ge, S. Tian, H. Yu, J. Zhao, J. Chen, L. Pan, R. Xie and L. Lu, Exogenous application of Mn significantly increased Cd accumulation in the Cd/Zn hyperaccumulator Sedum alfredii, Environ. Pollut., 2021, 278, 116837 CrossRef CAS PubMed.
- W. Xu, P. Xiang, X. Liu and L. Q. Ma, Closely-related species of hyperaccumulating plants and their ability in accumulation of As, Cd, Cu, Mn, Ni, Pb and Zn, Chemosphere, 2020, 251, 126334 CrossRef CAS PubMed.
- Q. Yang, X. Ma, S. Luo, J. Gao, X. Yang and Y. Feng, SaZIP4, an uptake transporter of Zn/Cd hyperaccumulator Sedum alfredii Hance, Environ. Exp. Bot., 2018, 155, 107–117 CrossRef CAS.
- S. Du, Q. Lu, L. Liu, Y. Wang and J. Li, Rhodococcus qingshengii facilitates the phytoextraction of Zn, Cd, Ni, and Pb from soils by Sedum alfredii Hance, J. Hazard. Mater., 2022, 424, 127638 CrossRef CAS PubMed.
- S. Chunwichit, T. Phusantisampan, A. Thongchai, P. Taeprayoon, N. Pechampai, J. Kubola, J. Pichtel and W. Meeinkuirt, Influence of soil amendments on phytostabilization, localization and distribution of zinc and cadmium by marigold varieties, Sci. Total Environ., 2024, 919, 170791 CrossRef CAS PubMed.
- X. He, X. Wang, J. Fang, Z. Zhao, L. Huang, H. Guo and X. Zheng, Bletilla striata: medicinal uses, phytochemistry and pharmacological activities, J. Ethnopharmacol., 2017, 195, 20–38 CrossRef CAS PubMed.
- W.-T. Tan, H. Zhou, S.-F. Tang, Q. Chen, X. Zhou, X.-H. Liu, P. Zeng, J.-F. Gu and B.-H. Liao, Simultaneous alleviation of Cd availability in contaminated soil and accumulation in rice (Oryza sativa L.) by Fe-Mn oxide-modified biochar, Sci. Total Environ., 2023, 858, 159730 CrossRef CAS PubMed.
- K. Wang, N. Zhang, F. Tan, J. He, J. Li and L. Bao, The influence of passivating agent on soil pollution, MethodsX, 2021, 8, 101321 CrossRef CAS PubMed.
- L. Sun, G. Zhou, R. Yang, Y. Li, S. Teng, L. Zhang and P. Yu, Synthesis of Novel Magnesium-Doped Hydroxyapatite/Chitosan Nanomaterial and Mechanisms for Enhanced Stabilization of Heavy Metals in Soil, J. Inorg. Organomet. Polym. Mater., 2022, 32, 3601–3620 CrossRef CAS.
- C. Peng, K. Gong, Q. Li, W. Liang, H. Song, F. Liu, J. Yang and W. Zhang, Simultaneous immobilization of arsenic, lead, and cadmium in soil by magnesium-aluminum modified biochar: influences of organic acids, aging, and rainfall, Chemosphere, 2023, 313, 137453 CrossRef CAS PubMed.
- M. Riaz, M. Kamran, M. Rizwan, S. Ali, A. Parveen, Z. Malik and X. Wang, Cadmium uptake and translocation: selenium and silicon roles in Cd detoxification for the production of low Cd crops: a critical review, Chemosphere, 2021, 273, 129690 CrossRef CAS PubMed.
- B. Huang, J. Xin, H. Dai and W. Zhou, Effects of Interaction between Cadmium (Cd) and Selenium (Se) on Grain Yield and Cd and Se Accumulation in a Hybrid Rice (Oryza sativa) System, J. Agric. Food Chem., 2017, 65, 9537–9546 CrossRef CAS PubMed.
- N. Liu, Z. Jiang, X. Li, H. Liu, N. Li and S. Wei, Mitigation of rice cadmium (Cd) accumulation by joint application of organic amendments and selenium (Se) in high-Cd-contaminated soils, Chemosphere, 2020, 241, 125106 CrossRef CAS PubMed.
- N. Chen, X. Wang, Y. Wan, Y. Luo, Y. Huang and L. Zhang, Simulated solar light driven Fe(III)/Fe(II) redox cycle for roxarsone degradation and simultaneous arsenate immobilization, J. Hazard. Mater., 2020, 394, 121635 CrossRef CAS PubMed.
- X. Wang, J. Jiang, F. Dou, W. Sun and X. Ma, Simultaneous mitigation of arsenic and cadmium accumulation in rice (Oryza sativa L.) seedlings by silicon oxide nanoparticles under different water management schemes, Paddy Water Environ., 2021, 19, 569–584 CrossRef.
- Y. Wang, K. Zhang, L. Lu, X. Xiao and B. Chen, Novel insights into effects of silicon-rich biochar (Sichar) amendment on cadmium uptake, translocation and accumulation in rice plants, Environ. Pollut., 2020, 265, 114772 CrossRef CAS PubMed.
- M. Ma, X. Xu, Z. Ha, Q. Su, C. Lv, J. Li, D. Du and R. Chi, Deep insight on mechanism and contribution of arsenic removal and heavy metals remediation by mechanical activation phosphogypsum, Environ. Pollut., 2023, 336, 122258 CrossRef CAS PubMed.
- P. Cao, K. Qiu, X. Zou, M. Lian, P. Liu, L. Niu, L. Yu, X. Li and Z. Zhang, Mercapto propyltrimethoxysilane- and ferrous sulfate-modified nano-silica for immobilization of lead and cadmium as well as arsenic in heavy metal-contaminated soil, Environ. Pollut., 2020, 266, 115152 CrossRef CAS PubMed.
- Z. Wang, R. Han, A. Muhammad, D.-X. Guan, E. Zama and G. Li, Correlative distribution of DOM and heavy metals in the soils of the Zhangxi watershed in Ningbo city, East of China, Environ. Pollut., 2022, 299, 118811 CrossRef CAS PubMed.
- M. Nikishina, L. Perelomov, Y. Atroshchenko, E. Ivanova, L. Mukhtorov and P. Tolstoy, Sorption of Fulvic Acids and Their Compounds with Heavy Metal Ions on Clay Minerals, Soil Syst., 2022, 6, 2 CrossRef CAS.
- H. XiuZhi, S. Yi, W. Tianyu, J. Zhenmao and W. Shiqiang, Regulation Effects of Humus Active Components on Soil Cadmium Availability and Critical Threshold for Rice Safety, J. Environ. Sci., 2024, 45, 439–449 Search PubMed.
- D.-r. Zhang, H.-r. Chen, J.-l. Xia, Z.-y. Nie, X.-l. Fan, H.-c. Liu, L. Zheng, L.-j. Zhang and H.-y. Yang, Humic acid promotes arsenopyrite bio-oxidation and arsenic immobilization, J. Hazard. Mater., 2020, 384, 121359 CrossRef CAS PubMed.
- J. Ma, H. Guo, L. Weng, Y. Li, M. Lei and Y. Chen, Distinct effect of humic acid on ferrihydrite colloid-facilitated transport of arsenic in saturated media at different pH, Chemosphere, 2018, 212, 794–801 CrossRef CAS PubMed.
- S. Bae, R. N. Collins, T. D. Waite and K. Hanna, Advances in Surface Passivation of Nanoscale Zerovalent Iron: A Critical Review, Environ. Sci. Technol., 2018, 52, 12010–12025 CrossRef CAS PubMed.
- S. Bashir, M. Adeel, A. B. Gulshan, J. Iqbal, S. Khan, M. Rehman and M. Azeem, Effects of Organic and Inorganic Passivators on the Immobilization of Cadmium in Contaminated Soils: A Review, Environ. Eng. Sci., 2019, 36, 986–998 CrossRef CAS.
- A. Avellan, J. Yun, B. P. Morais, E. T. Clement, S. M. Rodrigues and G. V. Lowry, Critical Review: Role of Inorganic Nanoparticle Properties on Their Foliar Uptake and in Planta Translocation, Environ. Sci. Technol., 2021, 55, 13417–13431 CrossRef CAS PubMed.
- P. Phuphong, I. Cakmak, A. Yazici, B. Rerkasem and C. Prom-u-Thai, Shoot and root growth of rice seedlings as affected by soil and foliar zinc applications, J. Plant Nutr., 2020, 43, 1259–1267 CrossRef CAS.
- J. Niu, C. Liu, M. Huang, K. Liu and D. Yan, Effects of Foliar Fertilization: A Review of Current Status and Future Perspectives, J. Soil Sci. Plant Nutr., 2021, 21, 104–118 CrossRef.
- R. Chen, C. Zhang, Y. Zhao, Y. Huang and Z. Liu, Foliar application with nano-silicon reduced cadmium accumulation in grains by inhibiting cadmium translocation in rice plants, Environ. Sci. Pollut. Res., 2018, 25, 2361–2368 CrossRef CAS PubMed.
- D. Alexander, R. Ellerby, A. Hernandez, F. Wu and D. Amarasiriwardena, Investigation of simultaneous adsorption properties of Cd, Cu, Pb and Zn by pristine rice husks using ICP-AES and LA-ICP-MS analysis, Microchem. J., 2017, 135, 129–139 CrossRef CAS.
- M. F. Salem, A. A. Tayel, F. M. Alzuaibr and R. A. Bakr, Innovative Approach for Controlling Black Rot of Persimmon Fruits by Means of Nanobiotechnology from Nanochitosan and Rosmarinic Acid-Mediated Selenium Nanoparticles, Polymers, 2022, 14, 2116 CrossRef CAS PubMed.
- D. Pimentel and M. Burgess, Small amounts of
pesticides reaching target insects, Environ. Dev. Sustainability, 2012, 14, 1–2 CrossRef.
- T. L. Read, C. L. Doolette, N. R. Howell, P. M. Kopittke, T. Cresswell and E. Lombi, Zinc Accumulates in the Nodes of Wheat Following the Foliar Application of 65Zn Oxide Nano- and Microparticles, Environ. Sci. Technol., 2021, 55, 13523–13531 CrossRef CAS PubMed.
- M. Marmiroli, L. Pagano, R. Rossi, R. De La Torre-Roche, G. O. Lepore, R. Ruotolo, G. Gariani, V. Bonanni, S. Pollastri, A. Puri, A. Gianoncelli, G. Aquilanti, F. d'Acapito, J. C. White and N. Marmiroli, Copper Oxide Nanomaterial Fate in Plant Tissue: Nanoscale Impacts on Reproductive Tissues, Environ. Sci. Technol., 2021, 55, 10769–10783 CrossRef CAS PubMed.
- P. Zhang, Z. Guo, F. A. Monikh, I. Lynch, E. Valsami-Jones and Z. Zhang, Growing Rice (Oryza sativa) Aerobically Reduces Phytotoxicity, Uptake, and Transformation of CeO2 Nanoparticles, Environ. Sci. Technol., 2021, 55, 8654–8664 CrossRef CAS PubMed.
|
This journal is © The Royal Society of Chemistry 2025 |
Click here to see how this site uses Cookies. View our privacy policy here.