DOI:
10.1039/D3RA06396H
(Review Article)
RSC Adv., 2023,
13, 32596-32626
Recent synthetic strategies for the construction of functionalized carbazoles and their heterocyclic motifs enabled by Lewis acids†
Received
20th September 2023
, Accepted 25th October 2023
First published on 6th November 2023
Abstract
This article demonstrates recent innovative cascade annulation methods for preparing functionalized carbazoles and their related polyaromatic heterocyclic compounds enabled by Lewis acid catalysts. Highly substituted carbazole scaffolds were synthesized via Lewis acid mediated Friedel–Crafts arylation, electrocyclization, intramolecular cyclization, cycloaddition, C–N bond-formations, aromatization and cascade domino reactions, metal-catalyzed, iodine catalyzed reactions and multi-component reactions. This review article mainly focuses on Lewis acid-mediated recent synthetic methods to access a variety of electron-rich and electron-poor functional groups substituted carbazole frameworks in one-pot reactions. Polyaromatic carbazole and their related nitrogen-based heterocyclic compounds were found in several synthetic applications in pharma industries, energy devices, and materials sciences. Moreover, the review paper briefly summarised new synthetic strategies of carbazole preparation approaches will assist academic and pharma industries in identifying innovative protocols for producing poly-functionalized carbazoles and related highly complex heterocyclic compounds and discovering active pharmaceutical drugs or carbazole-based alkaloids and natural products.
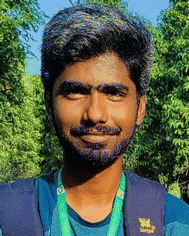 Marappan Pradeep Kumar | Marappan Pradeep Kumar, originally from Tiruppur, Tamil Nadu, holds a B.Sc in Chemistry from Bharathiar University (2018–2021) and an M.Sc in Chemical Sciences from Pondicherry University (2021–2023). He gained research experience through a summer research fellowship at IIT Madras under the guidance of Prof. G. Sekar. Currently, he serves as a Project Assistant in the OMC research group at the National Institute of Technology Karaikal, Puducherry, working under the supervision of Dr Vasudevan Dhayalan. His research interests encompass Organocatalysis and Green Chemistry, reflecting his commitment to sustainable and environmentally friendly chemical processes. |
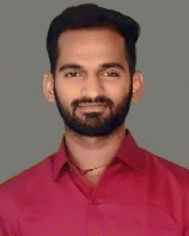 Mahantesh G. | Mahantesh was born in Kerehalli, Karnataka, India. He received his Bachelor of Science (BS) in Chemistry from Vijayanagara Sri Krishnadevaraya University in Bellari (2019–2022). At the National Institute of Technology (NITPY), Puducherry, India, he is working towards a Master's degree (MS) in Chemistry (2023–2025). He is presently working on master's studies in NHC organocatalysis with the OMC group of Dr Vasudevan Dhayalan and has an interest in synthetic organic chemistry. |
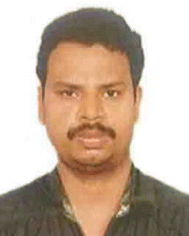 P. Amaladass | Dr P. Amaladass was born in Tamil Nadu state, India, in 1978. He was completed his schooling in the year of 1996 from Don Bosco Higher Secondary School in Tamil Nadu state. He received his under-graduation (B.Sc.,-Chemistry) and post-graduation (M.Sc.,-Chemistry) degree from Loyola College in Chennai which affiliated to University of Madras. After completion of his post-graduation, he worked as Junior Research Fellow in Spic Science foundation during ten months period. He obtained his Ph.D., in Organic Chemistry (Year: 2007) from the University of Madras, Department of Organic Chemistry under the supervision of Dr A. K. Mohanakrishnan (Prof. & Head of Organic Chemistry Department, University of Madras). His area of research is in synthetic organic chemistry. He is mainly focused on the synthesis of organic molecules which is highly useful in the area of “Light emitting diodes, solar cells, field effect transistors and hole transport materials”. Additionally, he did his post-doctoral research in Weizmann Institute of Science, Israel during the year of 2008–2009 under the supervision of Dr Michael Bendikov. In Israel, he did research on “Synthesis of oligoselenophenes (End free as well as End-capped Sexiselenophene analogues, End free fluorinated plus End capped fluorinated analogues) for organic field effect transistors (OFETs) applications”. Then he moved to next post-doctoral research in Nanyang Technological University, Singapore under the supervision of Dr Liu Xue-Wei during the year of 2009–2011. During his tenure in NTU, he synthesized organic dyes (aryl/heteroaryl ethyne bridged and selenophene based dyes) and also he has done basic fabrication part. After post-doc work in Singapore, he moved to Seoul National University from South Korea during the year of 2011 to 2015 under the supervision of Prof. Tae-Lim Choi. He has done research on synthesis of highly region-regular and high molecular weight polymers for organic photo-voltaic applications (OPVs). In addition, He also carried out research work on Nanostar and Nanonetwork crystals fabricated by in situ Nanoparticlizations of conjugated polymers. Additionally, he worked on synthesis of organic dyes based on boron analogues (BODPIY chemistry) for medicinal applications as sensors from South Korea in Dankook University during the period of 2015–2016. After completing his post-doctoral research, he moved as research professor at Korea University in South Korea (2017–2018). He has focused on “Synthesis of wide-bandgap conjugated copolymer and its application to non-fullerene polymer solar cells”. Currently, he is working as Assistant professor in the department of Chemistry from Madanapalle Institute of Technology & Science (MITS). Now, he is focussing on the synthesis of organic functional materials. |
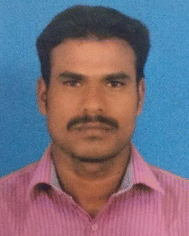 Chitrarasu Manikandan | Dr Chitrarasu Manikandan was born in Thozuvanthangal, Sankarapuram, Tamil Nadu, India. He received his Bachelor of Science (BS) in chemistry from Thiruvalluvar University (2007–2010). At the University of Madras, he completed his MS and PhD in Chemistry. He's presently working on projects Assistant in National Institute of Technology (NITPY) Puducherry now working NHC with the OMC group of Dr Vasudevan Dhayalan and was an interest in synthetic organic chemistry. |
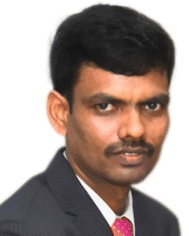 Vasudevan Dhayalan | Dr Vasudevan Dhayalan obtained his MSc in Organic Chemistry (2005) and his Ph.D. in Organic Chemistry (2011) at the University of Madras, India. He then joined the group of Prof. Masahiko Hayashi at Kobe University (Kobe, Japan) as a postdoctoral researcher (2011–2012). Later, he worked with Prof. Paul Knochel at the Ludwig-Maximilians-University Munich (Munich, Germany) for three years (2013–2015). In 2016, he joined the group of Prof. Anat Milo at the Ben-Gurion University of the Negev (Beer Sheva, Israel) with PBC outstanding postdoctoral research fellowship (2016–2020). Currently, he works as DST-SERB-Ramanujan Faculty at the National Institute of Technology Puducherry (Karaikal, India), supported by the Ramanujan fellowship (2020–2025). He was recently awarded a prestigious Ramanujan fellowship and Core-Research Grant (CRG) from Science and Engineering Research Board (SERB), India. His OMC research group projects are focused on the design and synthesis of drugs and natural products via organocatalysis and Li, Mg, Zn-organometallics. He has published 79 research articles in reputed international journals till now (September 2023). |
1. Introduction
Carbazoles are one of the most important nitrogen-based tricyclic aromatic heterocycles, as their aryl rings fused polycyclic carbazole scaffolds are present in many drugs and natural products (Fig. 1).1–19 In recent years, many research groups have focused on the difficult synthesis of annulated carbazole scaffolds using Lewis acid-mediated methods which have applications in bio-medical and pharmaceutical fields.20–27 However several catalytic and non-catalytic methods have been discovered for the formation of the annulated carbazoles.28–32 The first isolation of carbazole from coal tar was reported by Graebe and Glazer in 1872.33 Later, in 1965 murrayanine, a carbazole derivative, was isolated from Murraya koenigii spreng by Bose and his groups.34 The groups of Knölker and Reddy together extensively studied the preparation of biologically active carbazoles and their alkaloids.35,36 Carbazole and its derivatives have attracted unique attention in synthetic organic chemistry and material sciences because of their numerous applications.37–51 In recent years, various research groups have remarkably found that carbazole derivative have divergent biological applications such as affinity to bind with estrogen receptors,52 anti-tumor activity,53–56 antiplasmodial,57 antimalarial,58 antibiotic,59 antifungal,60,61 etc. Besides their biological applications, they also have bioimaging properties, applications in developing OLEDs,46–49 OFETs,41 solardyes,43 conducting polymers,37 photovoltaic devices,39 optoelectric properties,38,40 and organic semiconductors. Several benzo- and naphthocarbazole analogs have been investigated as potential anticancer drugs, according to a previous report.62,63 Recently, several groups explored Lewis acid-mediated Friedel–Crafts arylation of benzyl halides, benzyl alcohol/acetate, olefins etc.64–72 Dhayalan et al. recently discovered new synthetic strategies via a Lewis acid-mediated Friedel–Crafts types arylation and heteroarylation of benzylic indole system.73 In 2008, Mohanakrishnan and co-workers demonstrated innovative cascade techniques for hetero-annulated carbazoles, enabled by Lewis acids using electron-rich arenes and hetero-arenes.74–81
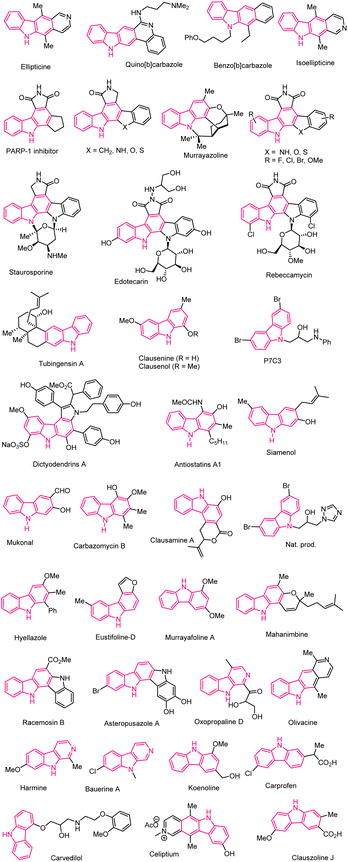 |
| Fig. 1 Carbazole-based biologically active natural products and drugs. | |
Over the past three decades, N-protected indole substrates have been widely used for the selective preparation of highly substituted carbazole derivatives used by various catalytic approaches. Due to their diverse applications, various synthetic methodologies have been developed for the synthesis of substituted carbazoles and annulated carbazole derivatives via cycloadditions,82–85 metal-catalyzed cross-coupling,86–89 metal-free cyclization,90–94 multi-component reactions,90,95 sigmatropic reactions74–76 and thermal electrocyclization,96–99 base-mediated cyclizations.100–103 Among these divergent methods, Lewis acid catalyzed methods are highly effective and attractive in academic and pharmaceutical industries due to their non-toxic, atom economy, high-efficiency conversions, and increased reproducibility. Over the past decade, various Lewis acid catalysis methods have been developed, resulting in the production of carbazole analogs because of their simplicity compared to traditional methods.74–81 Hence, in this review, we have summarised the various synthetic methods for constructing functionalized carbazoles and their heterocyclic motifs enabled by Lewis acids.
Based on the previous literature reports, various research groups described sustainable processes for the preparation of carbazole and their analogs via the Lewis acid mediated Friedel–Crafts types arylation and C–C cross-coupling reactions. In the initial test reaction, Dhayalan et al. planned for the preparation of N-protected bromomethyl indole,73–75 from corresponding indole methyl compounds via NBS-mediated radial bromination. The authors decided to prepare a synthetic precursor of N-SO2Ph, Ts, Boc groups protected-2-benzyl indoles 2a–c starting from the respective ester functional group substituted bromo methyl indoles 1a–c in the presence of 1–2 equivalent of ZnBr2 via Friedel–Crafts arylation approaches (Scheme 1).
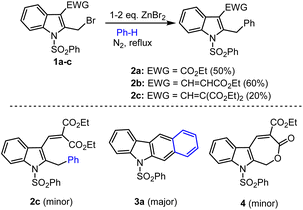 |
| Scheme 1 Arylation of 3-substituted bromomethyl indole used by ZnBr2. | |
2. Lewis acid catalysed synthesis of polyfunctional groups substituted carbazoles and their related heterocyclic scaffolds
2.1. Lewis acid mediated synthesis of annulated carbazoles derivatives from bromomethyl indoles
The direct arylation of respective indole-based benzylic bromo compound 1a/1b using benzene as an arene substrate and solvent in the presence of 2 eq. of ZnBr2 at reflux condition was found to be successful conversions and led to the formation of desired arylated indole products 2a and 2b in 50% and 60% yields respectively. However, under identical sustainable conditions, in the case of diethyl malonate containing benzylic bromo compound 1c, a similar Friedel–Crafts arylation protocol was found to produce a mixture of product as shown in Scheme 1.74 A careful column chromatographic separation of the crude reaction mixture led to the isolation of unexpected domino reaction product of benzo[b]carbazole 3a (25%) and byproduct lactone 4 (5%), in addition to the expected cross-coupling 2-benzylindole 2c (20%) as cited in Schemes 1 and 2. Authors observed the formation of stable cyclic lactone 4 that might be realized via the loss of ethyl bromide from the bromomethyl indole 1c in the presence of Lewis acid, which was confirmed by the formation of 4 (50–60%) under refluxing 1c with 2 equiv. of anhydrous ZnBr2 in DCE. The formation of fused benzo[b]carbazole product 3a might occur from arylmethylindole 2c. Hence, the N-phenylsulfonyl-2-benzylindole 2c was refluxed in a high boiling solvent, xylene for 1–2 h, which led to the formation of carbazole 3a in good yield (60%) and diethyl malonate (DEM) as a byproduct (Scheme 2).74 Obviously, the arylated compound 2 underwent ZnBr2 facilitated a thermally facile 1,5-hydrogen shift to form a triene species A, which on electrocyclization followed by subsequent elimination of diethyl malonate unit afforded the expected carbazole 3a in good yields. In addition, authors attempted several annulation reactions of benzyl indole 2b under reflux in xylenes but were unsuccessful in producing the desired carbazole. Hence, in the case of vinyl ester containing bromo compound 2b, the expected thermal 1,5-hydrogen shift is not as feasible as that of 2c.
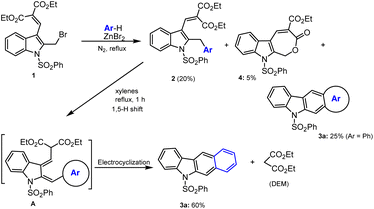 |
| Scheme 2 ZnBr2 mediated domino reaction of indole methyl bromides. | |
The domino reaction of bromomethyl indole 1c with arenes or heteroarenes in the presence of ZnBr2 under heating conditions in 1.2-DCE at 80 °C for 1–5 h produced the arylated indole A via Friedel–Crafts process. Next ZnBr2 prompted thermal 1,5-hydrogen shift to form a triene intermediate B, which occurs at high-temperature electrocyclization permit. The desired non-aromatic compound C formation followed by subsequent aromatization and elimination of diethyl malonate unit afforded the expected carbazole 3a in good yields as shown in the Scheme 3.74–76
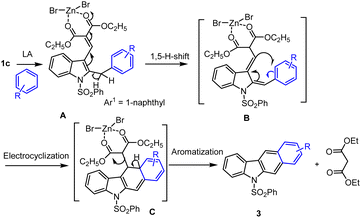 |
| Scheme 3 Proposed mechanism for domino reaction of bromomethyl indole enabled by ZnBr2. | |
Due to the high simplicity of the present domino reaction protocol reported by Dhayalan et al., they planned and tested this protocol by setting reaction between arenes/heteroarenes and bromomethyl heterocycles and benzylic bromides (1d–k) as cited in Scheme 4. Surprisingly, bromo compound 1d–k on heating with arenes in the presence of 1–2 equiv. of ZnBr2 or catalytic amounts of 10–20 mol% Lewis acid (InBr3, Yb(OTf)3, FeBr3, SnCl4, Sc(OTf)3) led to the formation of a variety of carbazole derivatives (Scheme 5) in the range of 25–65% yields.
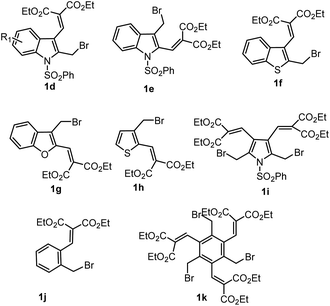 |
| Scheme 4 Appropriate aryl and heteroaryl methyl bromides for domino reactions catalysed by LAs. | |
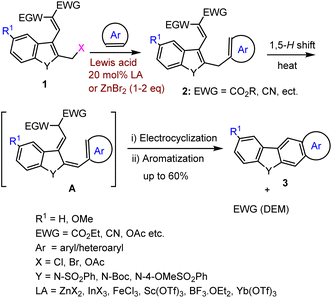 |
| Scheme 5 LA-mediated domino reaction of aryl/heteroaryl methyl bromides with various arenes and heteroarenes. | |
The bromo compounds substrate scope and product limitations of the domino reaction were further explored with 2-bromomethyl indole 1d, 3-bromomethyl indole 1e, benzo[b]thienyl 2-methylbromide 1f, 3-benzo[b]furan 3-methylbromide 1g, 3-bromomethylthiophene 1h. 2,5-di-bromo methyl pyrrole 1i, as well as mono and tri-benzyl bromide 1j–k, under optimized reaction conditions, obtained a broad range of results summarised in Schemes 5–7.74–76
Furthermore, using optimized domino reaction approaches various highly functionalized arenes and heteroarenes were tested under identical conditions in the presence of 2 eq. ZnBr2 or 10 mol% InBr3 in 1,2-DCE under refluxing condition 1–5 h. Electron-donating arenes and heteroarenes produced expected complex heterocyclic compounds in moderate to good yield as mentioned in the Schemes 5 and 6. This domino system tolerates various heteroarenes such as furan, thiophene, indole, benzofuran, and benzothiophene and arenes such as benzene, toluene, xylene, anisole, veratrole, mesityl, fullerene, naphthalene, and the list of obtained carbazole products and their derivatives have been shown in Scheme 7.
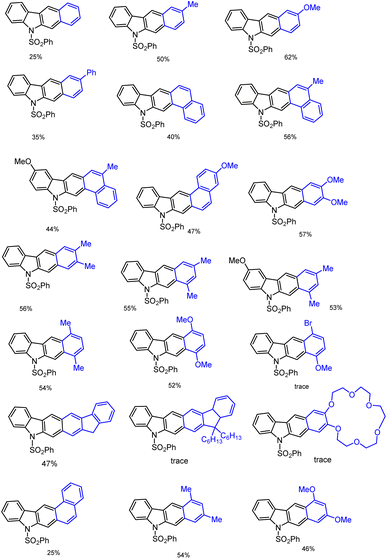 |
| Scheme 6 ZnBr2 mediated domino reaction of indole methyl bromides with various arenes. | |
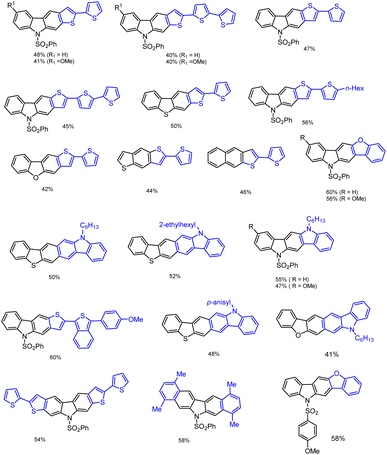 |
| Scheme 7 ZnBr2 mediated domino reaction of indole methyl bromides with various hetero-arenes. | |
Finally, the challenging bis-annulation of pyrrole bromo compound 1f was performed with bithiophene/p-xylene used by 4 equiv. of ZnBr2 to afford dithienocarbazole and dibenzocarbazole in 54% and 58% yields, respectively (Scheme 7). Unfortunately, the tribromo compound 1k failed to produce expected poly-aromatic products 3 under the same reaction conditions.
In 2009 Dhayalan and co-workers reported a direct and general method for the preparation of cyclo[b]-fused carbazoles scaffolds that have been established by starting from suitably N-SO2Ph protected 2/3-(bromomethyl)indoles and various arenes and electron-rich heteroarenes under mild conditions via Lewis acid catalysis.75 The attractive new feature of this efficient protocol is the fact that a broad variety of highly π-conjugated annulated carbazole derivatives can be readily accessed by the suitable choice of commercially available variety of electron-rich arenes and heteroarenes. The annulation method has been successfully extended to (bromomethyl)benzene as well as 1,3,5-tri(bromomethyl)benzene. The results of the Lewis acid-mediated cascade approach indicated that arenes were found to react less favourably with the indolyl-2-methylacetate substrate than with the comparable 2-(bromomethyl)indole. However, in the case of the simple benzylic acetate, the system was found to be more applicable compared to benzylic bromides.
Surprisingly, under identical conditions, in the presence of 20 mol%. ZnBr2 in 1,2-DEC under refluxing condition 1–5 h the domino reaction of the n-Boc protected bromo compound 1d′ with electron-rich heteroarenes such as furan, thiophene, and indole led to the formation of annulated N–H free carbazole 3′ in 55–66% yield. Fortunately, the reaction between bromo compound 1d′ and veratrole produced the desired annulated carbazoles in 62% of yield (Scheme 8)77 This system clearly indicates that in the case of 1,2-dimethoxybenzene or heteroarenes, the electron density of the veratryl/heteroaryl unit induces the formation of carbazoles. But solvents like benzene, toluene, xylene, and anisole under the same conditions led to the formation of corresponding 2-arylated indole 3-aldehydes 2′. The indole-2-arylated intermediate A upon intramolecular cyclization promoted by ZnBr2 may lead to the formation of the desired cyclized product C. The later elimination of diethyl malonate and aromatization process followed by simple cleavage of labile Boc unit might have produced N–H carbazole derivatives 3′ in good yields as shown in Scheme 9.77
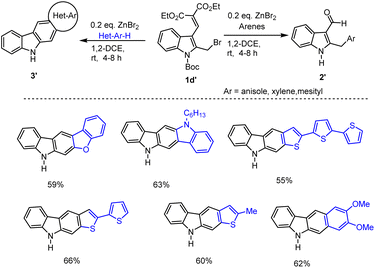 |
| Scheme 8 ZnBr2 mediated domino reaction of Boc-protected indole methyl bromides with various heteroarenes. | |
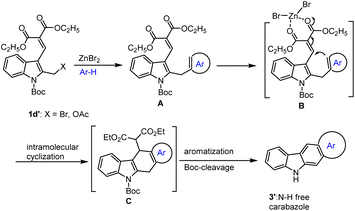 |
| Scheme 9 Proposed mechanism for domino reaction of N-Boc-bromomethyl indole enabled by ZnBr2. | |
Later, Dhayalan and co-workers developed another new method for the FeCl3-Lewis acid-mediated simple and practical cascade annulation sequence that has been developed in this project which lead to new synthetic applications in the field of synthesizing polycyclic aromatic and heterocyclic products. An attractive industrial applicable domino reaction procedure was established using N-SO2Ph-protected bromomethyl indole diacetate 5. The interesting results were obtained which are summarised in Scheme 10.76 The synthesized π-conjugated indole and thiophene-based annulated carbazoles derivatives can be readily be applied in various pharmaceutical and materials applications such as OLED, OFETS, organic semiconductor, organic photovoltaics, and conductive polymers.
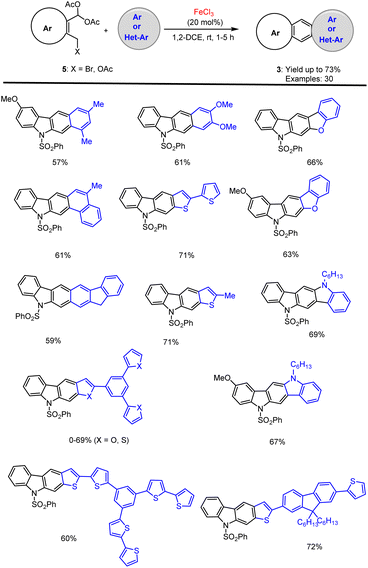 |
| Scheme 10 FeCl3 mediated cascade annulation of bromomethyl indole with various arenes and heteroarenes. | |
Recently in 2022, the groups of Mohanakrishnan and co-workers reported the straightforward protocol for the annulation of 2-bromomethylindoles 1c and 6 containing masked aldehyde with electron-rich heteroarenes in the presence of 20 mol% of Zn(OTf)2 in 1,2-DCE at room temperature for 1–3 h led to the formation of benzo[b]ring fused annulated carbazole derivatives in good yields in the range of 40–70%. This reaction condition led to the production of highly complex heterocyclic compounds, 3 in high yields as cited in Scheme 11.104
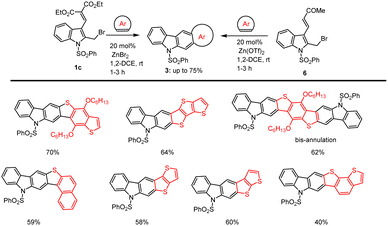 |
| Scheme 11 Zn(II)-catalyzed annulation of vinyl ester substituted 2/3-bromomethylindole with heteroarenes. | |
In 2021 Mohanakrishnan and his group reported the synthesis of annulated carbazole 8a 69% by reacting bromo-methylindole with veratrole by using 20 mol% FeCl3 as Lewis acid. They further studied substrate scope by having the best conditions in their hand. The desired annulated carbazoles were generated in 8b–e 64–70% yields by the cascade annulation reaction of 3-bromomethylindole provided by 2-vinyl ester with xylenes and benzodioxole in the presence of 20 mol% FeCl3 (Scheme 12).105 In the same way, napthocarbazole (8f–g) was prepared with yields of 74% and 76%. The proposed Lewis acid mediated annulation mechanism is cited in Schemes 12 and 13. Initially arylmethylindoles (A) is formed and undergo FeCl3-catalyzed intramolecular Friedel–Crafts alkylation at the beta position of methyl vinyl ketone (MVK) unit followed by aromatization to give adduct dihydrocarbazole (C) followed by aromatization through FeCl3 influenced elimination of acetone affords carbazoles 8.
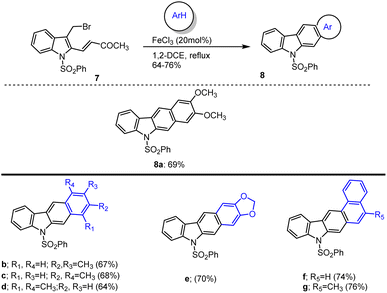 |
| Scheme 12 Preparation of annulated carbazoles. | |
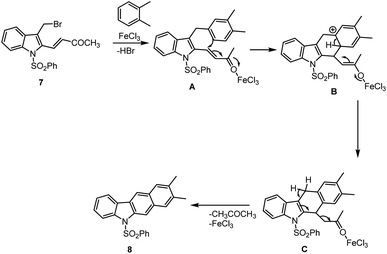 |
| Scheme 13 Plausible mechanism for FeCl3-catalyzed synthesis of carbazoles. | |
The same group additionally prepared hetero-annulated carbazoles by utilizing SnCl4 as a Lewis acid in 1,2-DCE at room temperature. Different hetero-annulated carbazoles were prepared by using the best conditions. The reaction of 3-bromo-methylindole 7 with methylthiophene afforded corresponding thienocarbazoles 8a–b accordingly. An indivisible mixture of carbazole 8c and 8c′ was formed (1
:
0.4 ratio) by the reaction of bromomethylindole with benzo(b)thiophene. 8d was formed on reaction with 2-bromomethylindole with benzo[b]furan. The desired heteroannulated carbazoles 8e–j were obtained by reaction of bromomethylindole with thiophene-, indole-, and pyrrole-based heteroarenes as mentioned in Scheme 14.105
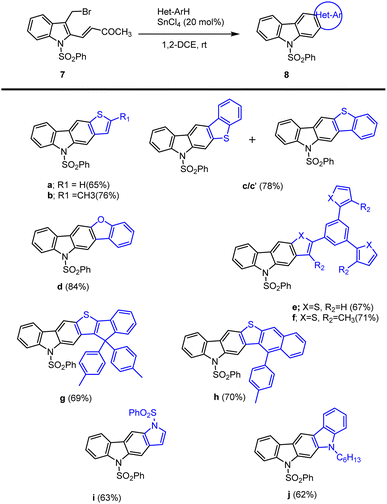 |
| Scheme 14 Synthesis of hetero-annulated carbazoles. | |
2.2. Synthesis of substituted carbazole analogs from acetoxymethyl-, pivaloyloxymethyl-and hydroxymethyl indoles enabled by Lewis acids
Mohanakrishnan and co-workers showed a new method for the preparation of 5-aryl substituted carbazole derivatives via BF3·OEt2 Lewis acid-mediated domino reaction of bis-diacetoxymethyl substituted aryl and heteroaryl compounds as shown in Scheme 15.106 In the presence of 40 mol% BF3OEt2 at rt, 4–6 h, they planned the annulation reactions of indole-derived tetra-acetate 9 with 2-hexylthiophene and bithiophene, which on cyclization followed by aromatization furnished the conjugated annulated heterocycles 10 in 49–58% yields. Similarly, under identical conditions, the annulation reaction with benzofuran afforded the anticipated carbazole product 10 in 53% yields.
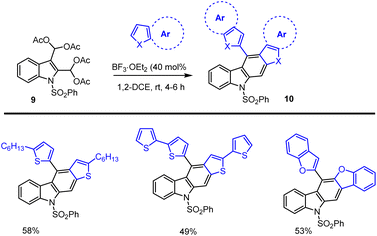 |
| Scheme 15 BF3·OEt2 Lewis-catalyzed construction of carbazole motifs. | |
Later, in 2016, the same group developed a straightforward method for preparing aryl and hetero-aryl ring fused annulated carbazoles was developed using SnCl4-mediated Friedel–Crafts arylation, cyclization, and aromatization reactions from readily accessible 3-acetyl or aryl groups substituted 2-pivaloyloxymethyl indoles 11 (Scheme 16).107 The initial step involved SnCl4 mediated Friedel–Crafts acylation giving the intermediate A followed by intramolecular cyclization led to the dihydrocarbazole B, and the final steps involved a simple aromatization process to offer the expected napthocarbazole 12 as shown in Scheme 17. The required indole starting material is easily prepared from commercially available 2-methylindole via Friedel–Crafts acylation method, followed by NBS-bromination and pivaloylation steps. Remarkably, various electron-poor and electron-rich aroyl units including indoles or different heterocyclic systems are well tolerated in the presence of stoichiometric amount of SnCl4. Moreover, this protocol could be extended to the successful synthesis of poly-heterocyclic compounds via bis-cascade annulation of 2,5-bis-(2-pivaloyloxymethyl)pyrrole under optimized conditions, which can offer good yield of complex carbazole.
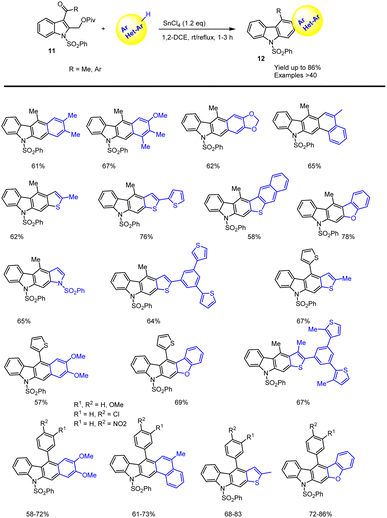 |
| Scheme 16 SnCl4-catalyzed cascade reaction of 2-pivaloyloxymethyl indoles with various arenes and heteroarenes. | |
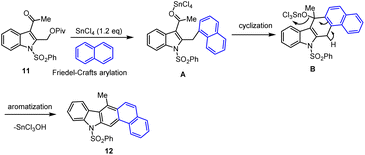 |
| Scheme 17 Proposed mechanism of SnCl4-catalyzed cascade reaction of 2-pivaloyloxymethyl indoles. | |
In order to synthesize highly substituted carbazole analogues 15, Banerjee and his colleagues established an efficient cascade protocol in 2019 for annulating DACs and indonyl methyl 3-alcohol using Lewis acids. The annulation of indole alcohol 14 was performed at room temperature, with cyclopropyl ester 13 using a 20 mol% InCl3 catalysed [3 + 3] annulation in DCM, and the desired highly substituted carbazoles 15 was obtained with moderate to good yield, up to 72%, as illustrated in Scheme 18.108
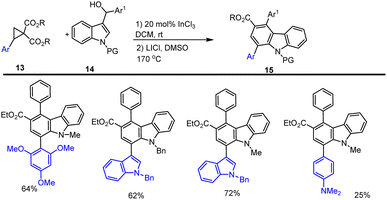 |
| Scheme 18 Lewis acid catalyzed [3 + 3] annulation with indole and DACs. | |
2.3. Synthesis of annulated carbazoles from propargylic alcohols and ester used by Lewis acids
Reddy et al. demonstrated the mild conditions for the synthesis of carbazole analogs 18 using a catalytic amount of Lewis acid (BF3·OEt2, 5 mol%) with the addition of propargylic alcohols 17 and indole ester 16 through Friedel–Crafts arylation and electrocyclization of allene B in DBU, CH3CN at room temperature for 1–5 h. Applying this method allows various polycyclic aromatic compounds to be synthesized efficiently as shown in Schemes 19 and 20,109 the reaction proceeds under sustainable conditions, yielding water as the byproduct.
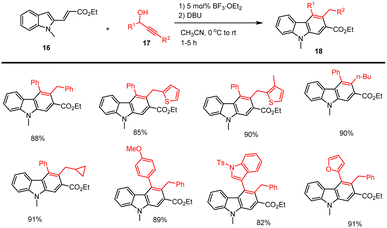 |
| Scheme 19 BF3·OEt2-catalyzed construction of carbazole. | |
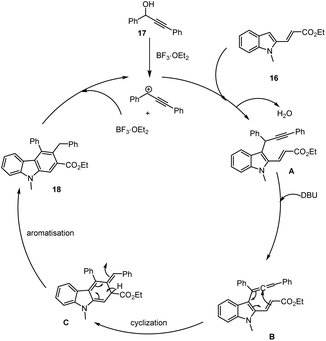 |
| Scheme 20 Proposed reaction mechanism for the construction of carbazoles. | |
In 2018 a new copper(II)-catalyzed intermolecular cascade annulation approach for the construction of a large variety of pentacyclic backbone having valuable carbazole derivatives 21 was developed from easily available propargylic alcohols as a substrate 19 reported by Liang and his group. This procedure, which involves a following sequences Meyer–Schuster rearrangement/-isomerization/cascade cyclization, permits facile and atom-economical access to various carbazole based heterocyclic compounds with wide-range of functional-group tolerance in good to moderate yields in toluene at 120 °C under mild conditions as shown in Scheme 21.110
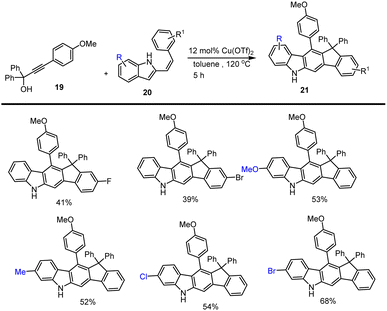 |
| Scheme 21 Lewis acid-catalysed synthesis of highly substituted carbazole from propargylic alcohols. | |
In 2014 Ma and his groups developed a simple and more efficient [i-PrAuCl]/AgSbF6-catalyzed cascade reaction of indole alkynols 22, for which starting materials are readily available and prepared from the 1,2-addition of indole-carbaldehydes with the related terminal alkynes in presence of 4 Å MS, offering various functional groups substituted carbazoles 23 with good yields under mild conditions. This cascade reaction tolerated various functional groups including electron-rich and electron-poor substituents in the presence of 5 mol% Au catalyst in 1,2-DCE at 25 °C for 15 h and obtained results are summarised in Scheme 22.111
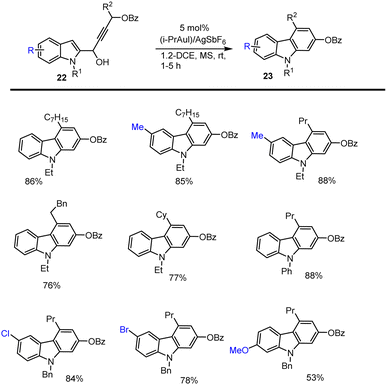 |
| Scheme 22 Au-catalysed preparation of carbazoles. | |
BF3 Lewis acid promoted annulated cascade reactions carried out between indole alcohol 24 and propargylic alcohols 25 furnished carbazoles with excellent selectivity and good yields of carbazoles 26 up to 72% based on the substrate structure reported by Huang et al. This cascade reaction tolerated various functional groups including electron-rich and poor substituents in the presence of 1.5 eq. of Lewis acid in CH3CN at 60 °C for 1 h as cited in Scheme 23.112
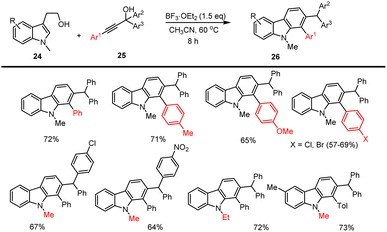 |
| Scheme 23 BF3·OEt2 Lewis acid catalyzed preparation of carbazole. | |
Through Lewis acid catalysed dehydrative [3 + 3]-annulation of easily available indole methyl alcohols 27 and substituted propargylic alcohols 28, Wang et al. investigated a new technique for producing nitrogen-based heterocycles. It was established to produce the required poly functional groups substituted carbazole derivatives 29 with moderate to good yields and under sustainable conditions, however it simply produced water as a green byproduct. The proposed cascade annulation reaction and the mechanism was described in the Schemes 24 and 25 involving the following sequences such as a cascade domino process involving Friedel–Crafts-type allenylation, 1,5-H shift, 6π-eletrocyclization, and Wagner–Meerwein rearrangement, aromatisation process.113
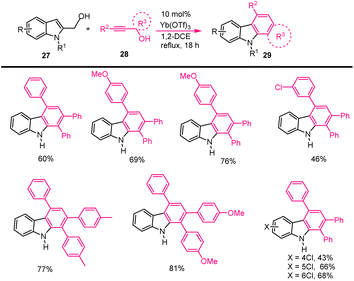 |
| Scheme 24 Synthesis of carbazole via Lewis acid catalyzed cascade reaction. | |
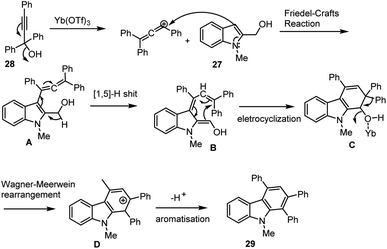 |
| Scheme 25 Proposed mechanism for Lewis acid catalyzed cascade reaction. | |
Tsuchimoto, Shirakawa and their groups have established a new annulation method that sustainable system permits the assembly of readily available indole building blocks 30 convert into diverse aryl- and heteroaryl ring fused annulated[a]carbazoles 31 enabled by an In(ONf)3 Lewis acid catalyst in 2005. The primary characteristics of this novel approach are the direct application of aromatic C–H bond activation and the evident absence of extra-directing functional groups on the aryl substrates. Various aryl and heteroaryl systems tolerate this annulation protocol in indole building blocks and the list of obtained conjugated carbazole products as summarised in Scheme 26 in high yield.114
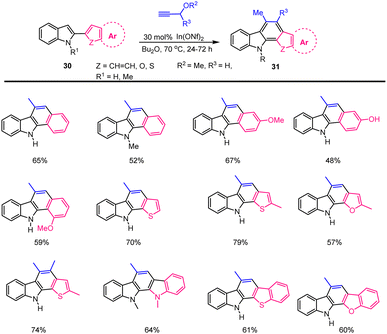 |
| Scheme 26 In(ONf)3-catalyzed annulation of 2-aryl and heteroaryl indoles with propargyl ethers. | |
2.4. Synthesis of annulated carbazoles via intramolecular C–H amination approaches
Jiang et al. in 2008 developed a novel method for the preparation of unsymmetrical carbazole derivatives 33 enabled by 5 mol% Pd(OAc)2. The intramolecular cyclization approach involves the selective functionalization of an aromatic C–H bond and the construction of an intramolecular new aromatic C–N bond. This coupling method is well-suited to a variety of electron-rich and poor functional groups. The efficacy of the new method was established by the concise preparation of various carbazole-based natural products 33 from commercially available starting materials 32 in good yield as cited in Scheme 27.115
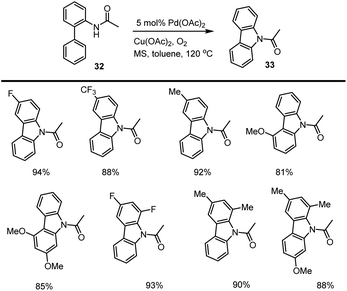 |
| Scheme 27 Synthesis of carbazoles via C–H activation and C–N bond formation. | |
Chang and his groups demonstrated the synthesis of carbazoles under mild conditions through intramolecular oxidative C–N bond-forming reaction of N-protected 2-amido biphenyls 34. As expected, the intramolecular cyclization took place in the presence Cu(OTf)2 5 mol%. Under optimized conditions, various substrates are afforded moderate to high yields of substituted carbazoles up to 91%. This intramolecular oxidative C–N bond formation reaction works well with other Lewis acids such as Zn(OTf)2, Zn(OAc)2, Sc(OTf)3, Fe(OAc)2, BF3·OEt2 has a lower yield compared to copper catalyst as cited in Scheme 28.116
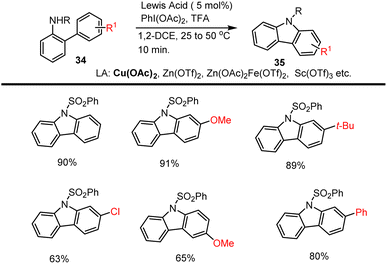 |
| Scheme 28 Synthesis of carbazoles via intramolecular oxidative C–N bond formation reaction. | |
Yin and his group showed a Pd(II)/Sc(OTf)3 catalyzed intramolecular C–H functionalization of biphenyl amide 34′ to formation of carbazole derivatives 36 in the use of O2 as the sole oxidant under mild conditions. This C–H activation reaction tolerated various functional groups including electron-rich and electron-poor substituents in the presence of 40 mol% Lewis acid in DMF at 120 °C for 24 h and obtained results are summarised in Scheme 29.117
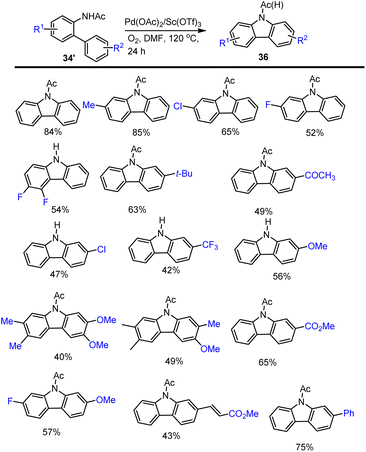 |
| Scheme 29 Pd(II)/Sc(III)-catalyzed intramolecular oxidative C–H amination. | |
Hashmi and his groups reported a mild synthesis of carbazole derivatives and related building blocks 39 through an intramolecular C–H amination approach. A prominent advantage of this new protocol is highly reactive aryl sulfilimines scaffolds 38 working well under photo condition as well as Lewis acids. To find the best-optimized reaction conditions, authors tested different Lewis acid catalysts, and various light sources. In the presence of LEDs, light itself produced the best yield with a wide-ranging range of substrate scope and more significant numbers of functional group tolerances, as shown in Scheme 30.118
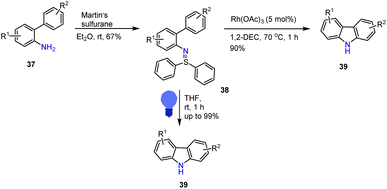 |
| Scheme 30 Synthesis of carbazoles from sulfilimines by intramolecular C–H aminations. | |
In 2011 Ren and co-workers examined a BF3·OEt2 Lewis acid-mediated nucleophilic aromatic substitution process for the regioselective preparation of highly functionalized carbazole scaffolds 42 in good yields. These amination reactions are carried out between Ar-Br 40 and Ar-(OH)2 41 in the presence of 5 mol% Pd cat. and 2 eq. of BF3·OEt2 under mild conditions. This C–C and C–N coupling system tolerates various classes of functional groups such as CHO, CO2R, CN, NO2, F, Cl, etc. Furthermore, this novel procedure was effectively applied to prepare carbazole-based natural alkaloids, such as clausine A–C analogs. The obtained interesting results are summarised in Scheme 31.119
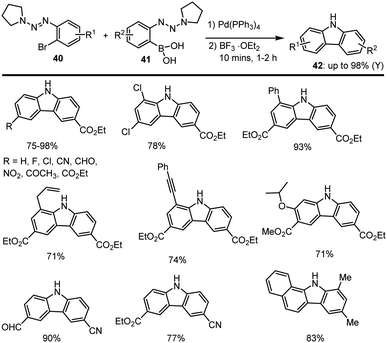 |
| Scheme 31 Lewis acid-promoted synthesis of functionalized carbazoles. | |
Mohanakrishnan and his groups developed a new method for the preparation of indolocarbazole via ZnBr2 mediated intramolecular cyclization of nitro compound 43 in the presence of triethyl phosphite at 90–95 °C for 12–15 h led to the construction of the respective indolocarbazole derivatives 44 in excellent yields in the range of 85–91%. This system tolerates a wide range of electron-donating groups as shown in Scheme 32.120
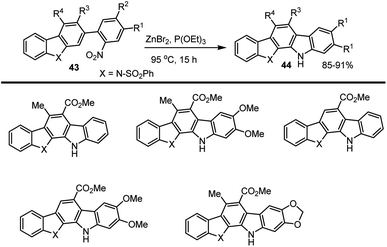 |
| Scheme 32 ZnBr2-mediated synthesis of indolocarbazole. | |
2.5. Synthesis of annulated carbazoles via multi component coupling reactions
A green synthetic method for producing carbazole and its derivatives 48 from basic chemical molecules such as hydroxy acetophenone 45 and -keto-ester 47 was disclosed by Yanlong Gu and co-workers. The annulation reaction was performed in a bio-based green solvent, glycerol using 15 mol% Sc(OTf)3 as a Lewis acids catalyst. The important aspect of this cascade reaction is that solvent and catalyst can be recovered and reused. The reasonable mechanism of [4 + 2] annulation reaction was proposed via the nucleophilic attack of C2 carbon at the carbonyl carbon of α-hydroxy acetophenone generating the intermediate A. The activation of intermediate A with the use of Sc(OTf)3 enhances the formation of intermediate B by pinacol-type rearrangement reaction. The intermediate B reacts with another molecule of β-keto-ester to form the intermediate C. Another nucleophilic addition of the C3 carbon of indole onto the keto group takes place, leading to the formation of species D which bears a six-membered ring system. Finally, the carbazole analogs 48 were generated by the dehydration of water molecules (Schemes 33 and 34).121
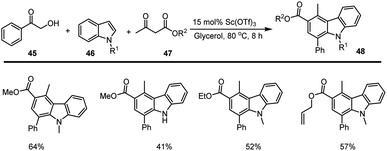 |
| Scheme 33 Sc(OTf)3-catalyzed preparation of carbazoles via [4 + 2] annulation reaction. | |
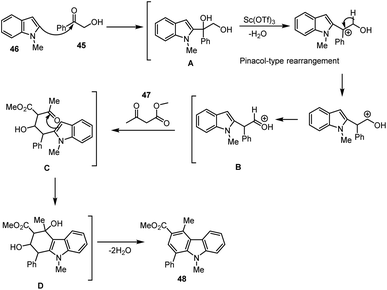 |
| Scheme 34 Plausible mechanism for Sc(OTf)3-catalyzed synthesis of carbazoles. | |
Gu et al. have discovered a unique three-component coupling process using the Lewis acid Bi(OTf)3 and indoles 46, bromoacetaldehyde acetals 49, and aryl ketones 50 to synthesise structurally varied carbazoles 51 in good yields. With the aid of 10 mol% bismuth(III) triflate, the multi-component indole reaction takes place with ketone and bromo compounds in CH3CN for 16 h at 80 °C. Given the abundance of readily accessible simple indoles and the wide range of commercially available aryl ketones 50, the suggested catalytic technique is a potential way for the synthesis of carbazole libraries with a high degree of diversity (Scheme 35).122,123
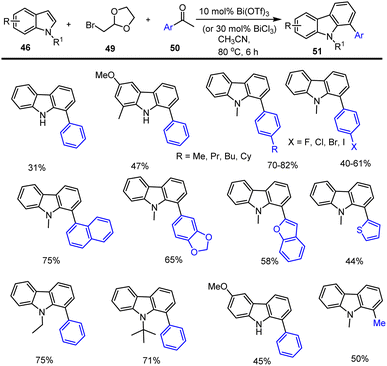 |
| Scheme 35 Bi(OTf)3-catalyzed three-component coupling reactions. | |
2.6. Synthesis of annulated carbazoles scaffolds from aromatic aldehydes or ketones enabled by Lewis acids
Indolyl ring-linked benzo[b]carbazoles 53 were synthesized by the efficient one-pot FeCl2-catalyzed cycloaddition of the indole moiety 46 and simple phenyl dialdehyde (o-phthalaldehyde) 52, as reported by Xu and his colleagues in 2014. This process involved sequential carbon–carbon bond-forming addition, intramolecular alkylation, and aromatization. The proposed Lewis acid-catalyzed cycloaddition reaction and mechanism were discussed in Schemes 36 and 37. The addition of indole 46 and dialdehyde 52 to generate species A and B after intramolecular cyclization and elimination of water to furnish the carbazoles 53 in good yields. This process tolerates various electron-rich and electron-poor functional groups in MeOH at rt for 12 h.124
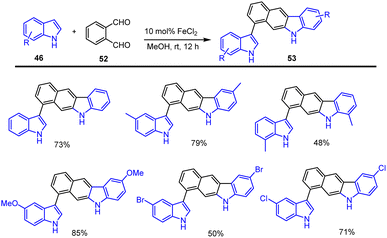 |
| Scheme 36 Fe-catalyzed cycloaddition of indoles and o-phthalaldehyde. | |
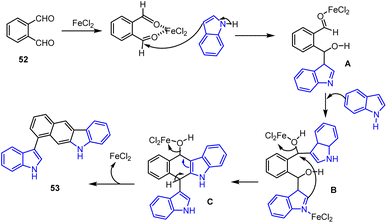 |
| Scheme 37 Proposed mechanism for Fe-catalyzed cycloaddition reaction. | |
By using Fe-catalyzed domino reaction sequences from substituted indole methyl benzaldehyde derivatives 55, Jana and groups established a unique and effective methodology for producing substituted benzo[b]carbazole derivatives 54. Notably, this sustainable system produced substituted carbazole derivatives in good to high yields as mentioned in Scheme 38.125
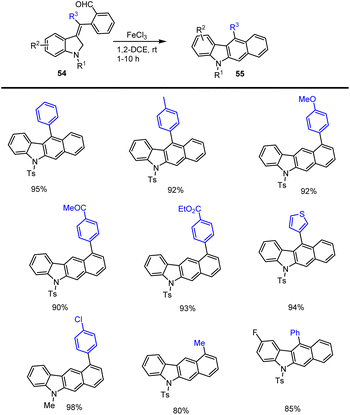 |
| Scheme 38 Synthesis of substituted benzo[b]carbazoles enabled by Fe-catalysis. | |
Recently Ruijter and co-workers reported a strategy for the synthesis of carbazole analogs 58 using a stoichiometric amount of Lewis acid Sc(OTf)3 through condensation between indole aldehyde 56 and phosphonate ester 57 in Cs2CO3, 1,4-dioxane at 100 °C for 24 h. Using this applicable method, various functional group substituted carbazole compounds can also be synthesized efficiently under sustainable conditions. The obtained list of carbazole derivatives is cited in Scheme 39.126
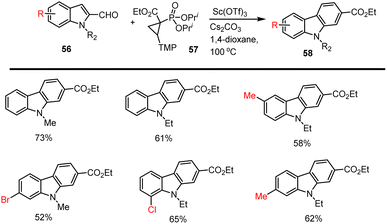 |
| Scheme 39 Synthesis of carbazoles used by Sc(OTf)3. | |
In 2002, Ila and co-workers developed SnCl4-induced domino carbocationic rearrangement for the synthesis of carbazole analogs 60 from N-protected indolyl cyclopropyl ketones 59 leading to the formation of 2,3-substituted cyclopentyl ring fused carbazoles (Scheme 40).127 This typical annulation system allows us to form synthetically useful carbazole products with good yields. Several unexpected interesting paths are involved in the presence of Lewis acid-mediated intramolecular enolate cyclization, indole position-2 C–H carbon cyclization, and the elimination of indole moiety. This protocol tolerates various functional groups on aryl rings, and the proposed possible domino reaction mechanism has been discussed in Scheme 41.
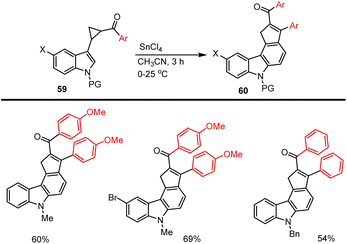 |
| Scheme 40 SnCl4-induced rearrangement of the synthesis of carbazole. | |
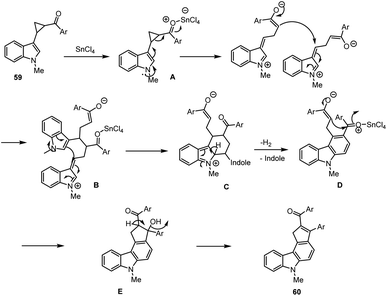 |
| Scheme 41 Proposed mechanism of domino carbocationic rearrangement. | |
Wang groups in 2015 significantly described a simple and direct protocol for the preparation of benzo[b] carbazole derivatives 62 enabled by Fe-catalysed 5-exo-dig intramolecular cyclization and a subsequent 6π-electrocyclization and aromatization. With the help of the Fe-catalyst, the annulation reaction went through an unusual [1,4]-Ts group migration from nitrogen to oxygen. As illustrated in Scheme 42, this straightforward, environmentally friendly, non-toxic iron catalytic system has key advantages for the moderate to good yield synthesis of annulated carbazole derivatives, including tolerating functional groups like F, Cl, Me, CN, NO2, etc. (Scheme 43) follows Iron assisted keto–enol tautomerization, cyclization, electrocyclization, aromatization, and tosyl group migration led to afford the unexpected fused carbazole motifs.128
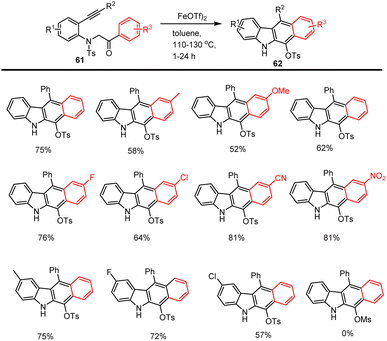 |
| Scheme 42 Fe-catalyzed method to benzo[b]carbazoles. | |
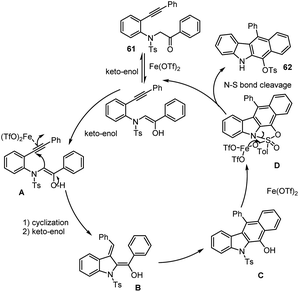 |
| Scheme 43 Proposed pathway for preparation of benzo[b]carbazoles via Fe-catalysis. | |
A unique technique for the synthesis of hydroxyl group substituted N–H free carbazoles 65 was published in 2018 by the team of Chen and co-workers. It involves the sequential cross-coupling of the C–C bond of electron-rich indoles and pyrrole with Cu. This report represents an atom economical procedure for the synthesis of both symmetric and unsymmetric functional groups substituted carbazoles achieved from easily accessible indole starting materials without the need for any expensive transition metal catalyst, ligands and harsh reaction conditions (Scheme 44). Possible mechanism involves the following sequences Lewis acid mediated Michael addition to furnish species A followed by intramolecular cyclization produce intermediate B and them simple aromatization offer substituted carbazole 65 as mentioned in Scheme 45. This simple Lewis acid system provides important features for the synthesis of annulated carbazole derivatives in good to high yields, including toleration of functional groups such as F, Cl, Br, I, Me, OMe, COMe, NO2, etc., under sustainable conditions.129
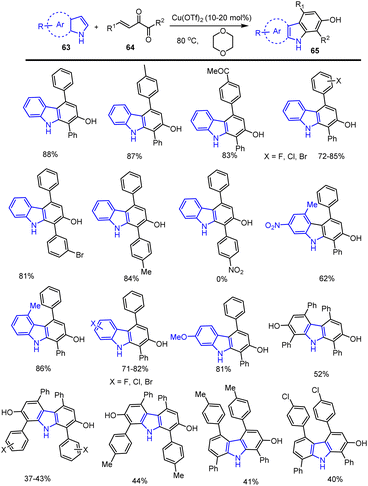 |
| Scheme 44 Preparation of oxygenated carbazole scaffolds. | |
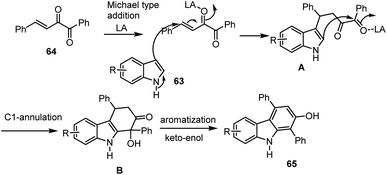 |
| Scheme 45 Proposed strategy for the construction of carbazole. | |
The group of Mohanakrishnan and colleagues in 2013 examined the Lewis acid-mediated thermal cyclization of the enamine 66 was then carried out using different Lewis acids, such as ZnBr2, CuBr2, InBr3, CeCl3, and FeCl3, and the obtained results are cited in Scheme 46. In general, the preparation of carbazole 67 was found to be successful with various Lewis acids.130
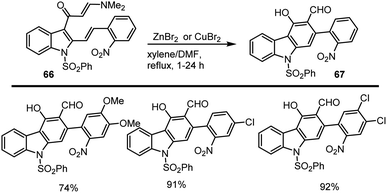 |
| Scheme 46 Lewis acid mediated synthesis of tri-substituted carbazole. | |
Rossi and co-workers reported the synthesis of highly substituted amino carbazole derivatives were obtained by using InCl3 as a Lewis acid and another LAs such as GaCl3 and TiCl4 also work well; under optimized condition, the desired carbazole 69 was obtained in 71–83% yield. Next, the authors decided to evaluate the scope and limitations of the suitable indole substrates catalysed by InCl3, which is an air-insensitive Lewis acid and is used as a carbon–carbon triple bond activator and can be used in catalytic amounts as LA even in producing the water from the first condensation process. Under the optimized domino reaction conditions, 10 mol% of InCl3, pyrrolidine (1.2 equiv.), dry CH3CN at 75 °C led to the expected amino carbazoles 69 in good yields as shown in Scheme 47.131
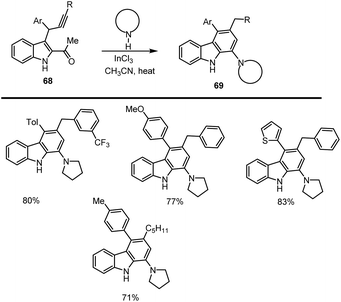 |
| Scheme 47 InCl3-Lewis acid mediated aminobenzannulation approach. | |
Chinmay Chowdhury and co-workers reported an atom-economical way of preparing carbazoles containing diverse functional groups 71 through Pd(II) catalyzed cascade reaction using 1-(indol-2-yl)but-3-yn-1-ols as starting material 70. The catalyst Pd(OAc)2bpy acting as lewis acid activates the triple bond of the substrate to generate intermediate A. The triple bond of the intermediate may undergo intramolecular nucleophilic attack (6-endo-dig) to form palladated intermediate B. Subsequent deprotonation at the indole ring followed by reprotonation of the hydroxyl group followed by dehydration and protonolysis leads to the formation of carbazole 71 and the catalyst is regenerated as mentioned in the Schemes 48 and 49.132
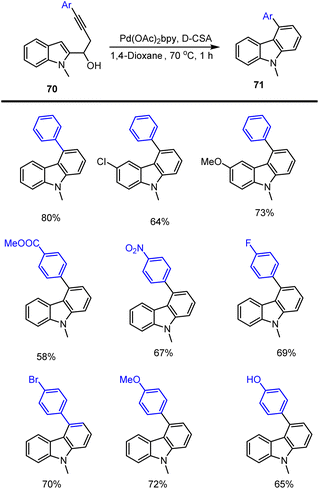 |
| Scheme 48 Synthesis of carbazoles using Pd(OAc)2bpy catalyst. | |
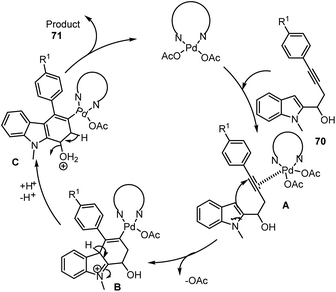 |
| Scheme 49 Plausible mechanism for Pd(OAc)2bpy-catalyzed synthesis of carbazoles. | |
2.7. Synthesis of annulated carbazoles via cascade domino reactions
Ma and co-workers in 2011 reported AuCl3-catalyzed cyclization of 1-(indol-2-yl)-3-alkyn-1-ols 72 in toluene at room temperature for 3–15 h to form a fused benzene ring leading to a series of carbazole analogs 73 in good yields up to 90%. A possible intramolecular cyclization mechanism has been examined for the formation of expected carbazole derivatives 73 as shown in Scheme 50.133
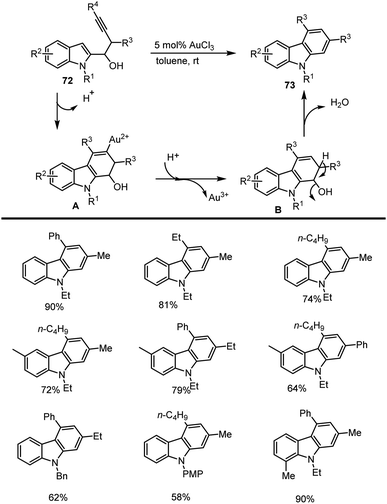 |
| Scheme 50 AuCl3-catalyzed synthesis of carbazole. | |
In 2007 Yang and his groups demonstrated Zn(OTf)2-Lewis acid catalyzed tandem annulation of aryl isonitriles 75 and indole-based allenic esters 74 which is an efficient and simple route for synthesising a broad range of structurally essential and biologically active carbazoles analogs 76. This reaction substrate scope is employed with the use of readily available starting materials under mild reaction conditions. Selectively generated heterocyclic compounds are found to have considerable value in drug discovery and natural product synthesis. In Schemes 51 and 52, the proposed reaction path is explored, and a number of carbazole derivatives 76 is cited.134
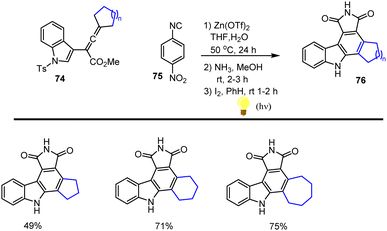 |
| Scheme 51 Lewis acid-catalyzed tandem annulation reaction. | |
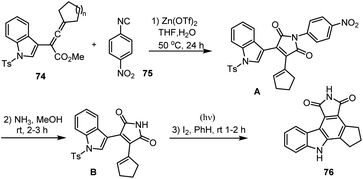 |
| Scheme 52 Zn(OTf)2-catalyzed cascade annulations for synthesis of carbazole. | |
In 2014 Wan and his groups showed a convenient procedure to access benzo[b]carbazole motifs 78 via a successive Cu-catalyzed arylation of electron-rich indole derivatives with Michael acceptor 77 and I2-promoted electrophilic cyclization followed by nucleophilic substitution and aromatization. The scope of the reaction is shown in Scheme 53.135
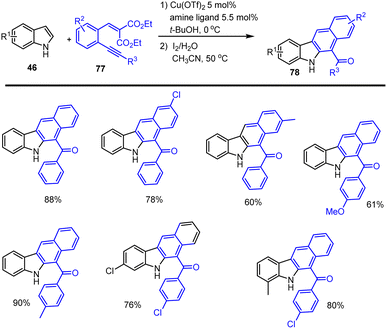 |
| Scheme 53 Cu-catalysed alkylation and I2-promoted cyclization of indoles. | |
In 2016, Mohanakrishnan and his teams established a straightforward, easy-to-follow approach for the one-pot electrocyclization and aromatization reaction procedure utilised to create heteroaryl ring fused annulated carbazoles 80. The annulation reaction was carried out with 3-indolylpyrroles 79 and 50 mol% of FeCl3 Lewis acid in DCM at 0 °C for 10 min. Which led to the formation of the respective pyrrolocarbazoles 80 in excellent yields up to 85% (Scheme 54).136
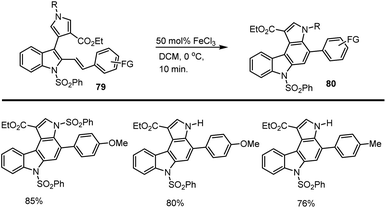 |
| Scheme 54 Synthesis of pyrrolo[2,3-c]carbazoles catalysed by FeCl3. | |
Pelkey and his groups reported a simple and flexible method to access 3-pyrrolin-2-one fused carbazoles. The critical essential step involves the BF3-mediated cyclization in DCM at −40 °C, providing access to aryl group-substituted carbazole derivatives 82 in good yields. This method represents the first example of the preparation of the benzo[a]pyrrolo[c]carbazole core system. In the literature, it was found that indolo[a]pyrrolo[c]carbazol-5-one is a significant biologically active compound (Scheme 55).137
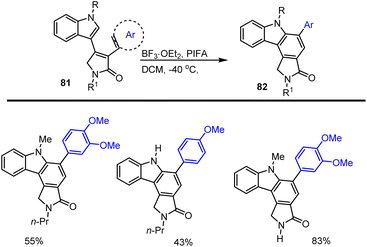 |
| Scheme 55 Synthesis of benzo[a]carbazoles enabled by LA. | |
Recently Balamurugan and his groups developed an efficient method for preparation highly functionalized hydroxy carbazole building blocks 84. The mild synthesis involves domino catalysis by 1 mol% of Sc(OTf)3 and 2 mol% of Rh2(OAc)4. The critical role of Sc(OTf)3 is to facilitate both the initial intermolecular Michael reaction of the indole derivatives 83 and the subsequent Rh(II)-catalyzed intramolecular annulation in DCM at rt with the addition of TFA. This protocol offers good yields of annulated carbazoles with various functional groups, as shown in Scheme 56.138
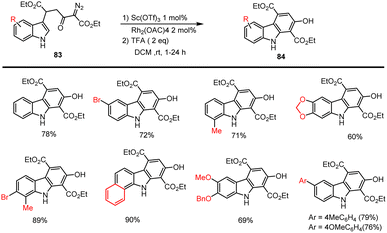 |
| Scheme 56 LA catalysed annulation of indolyl α-diazo acetate. | |
France and co-workers reported a new method of synthesizing 1-hydroxy carbazoles 86 ad 87 via acetal ring opening benzannulations of compound 85 by using Lewis acid as a catalyst. During the Lewis acid screening for ring-opening benzannulation, researchers found that Yb(OTf)3 acts as an excellent catalyst leading to maximum yield compared to other Lewis acids. When they explored benzannulation reactions with synthesized substrates of substituted dihydrofuran acetals, it was found that trans-dihydrofuran isomers react faster than the corresponding cis-dihydrofuran. The contradiction is illustrated by the fact that the trans-3-phenyl modified dihydrofuran substrate generated an epimer of the same substrate rather than a hydroxy carbazole. It is reasonable that the phenyl substituent provides anchimeric assistance resulting in the stabilization of the dihydrofuran and reversible ring opening. It is also found that when one drop of water is added to Al(OTf)3, the desired carbazole derivatives are formed with a high yield. This rapid shift in the reactivity with added water is due to the formation of TfOH which enables the formation of dihydrofuran hemiacetal intermediate that undergoes ring opening. When the effects of changing N-methyl substituent to a N-benzyl was explored, it was found that procedure A gives maximum yield for N-benzyl substituents. It is noteworthy, that the reaction did not work when 3-indolyl dihydrofuran was explored (Scheme 57).139
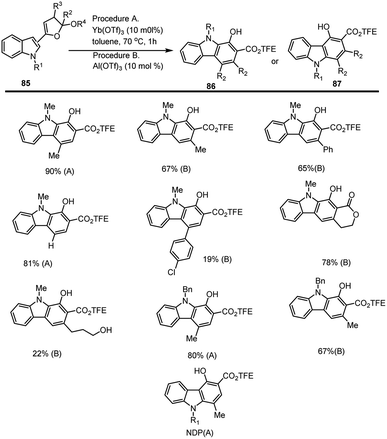 |
| Scheme 57 Synthesis of carbazoles via acetal ring opening benzannulations by using lewis acid. | |
Wang and his groups showed a series of new carbazolequinones 90 prepared by a B(C6F5)3-catalyzed [4 + 2] cyclization reaction. This method involved a simple operation, a broad range of substrate variety, and high atomic economy, 1 mol% Lewis acid catalyst loading and avoided using toxic metal catalysts. The carbazole-fused derivatives was found a significant impact on the fluorescence properties (Scheme 58).140
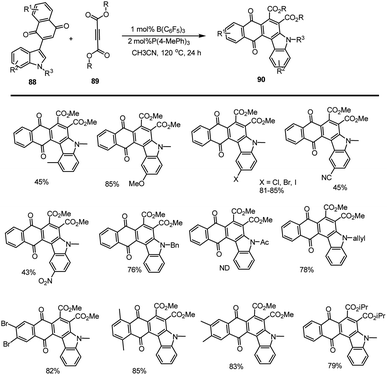 |
| Scheme 58 [4 + 2] Cyclization strategy catalysed by B(C6F5)3. | |
Given the expected carbazole 92 in lower yields ranging from 6% to 39% at 50 °C for after stirring for 1–10 h as shown in Scheme 59, Liu and groups investigated the annulation reaction of ynamide 91 bearing two aryl groups at the alkyne terminus using various Lewis acids such as Zn(OTf)2, ZnCl2, FeCl3, and AgSbF6 as the catalyst. Gratifyingly, JohnphosAu(MeCN)SbF6 was one of the best catalysts for this protocol, leading to the production of 1-aryl substituted carbazole 92 in 93% yield. The resulting high-yield formation of carbazole indicates that the nature of the phosphine ligands played a significant role in increasing catalytic activity.141
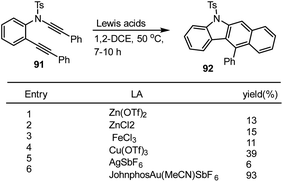 |
| Scheme 59 Synthesis of functionalized benzo[b]carbazoles enabled by Lewis acids. | |
3. Selective examples of biologically active carbazole derivatives and their applications
Carbazoles and their analogs are one of the most essential heteroaromatic compounds, where many of them are isolated from various natural sources. Over the last two decades, several research groups and pharma companies have focused on the design and synthesis of annulated carbazoles and related polyaromatic heterocycles via catalytic and non-catalytic methods. In recent years, many research reports shows that carbazole scaffolds have found to have significant applications in medicinal and pharmaceutical fields such as antitumor, anticancer, antiviral, antibacterial, Kinesin Spindle Protein Inhibitors, neuroprotective agents, XO inhibitors, CDK5/p25 kinase inhibition, Pim kinase inhibitors, antiplasmodial, antimalarial, antioxidant, antiproliferative agents, telomerase inhibitors etc. Moreover, carbazole backbone is present in many pharma drugs and natural products. The selectively collected list of carbazoles and annulated carbazoles as well as their bio-applications are detailed and summarised in the Table 1.142–179
Table 1 Application of biologically active carbazole scaffolds
S. no. |
Compound structure |
Activity/properties |
Ref. |
1 |
 |
Antitumor |
142 |
2 |
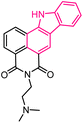 |
Antitumor |
143 |
3 |
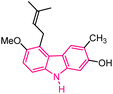 |
Antitumor |
144 |
4 |
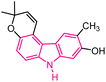 |
Antitumor |
144 |
5 |
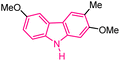 |
Antitumor |
144 |
6 |
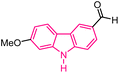 |
Antitumor |
145 |
7 |
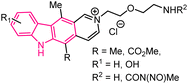 |
Antitumor |
146 |
8 |
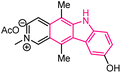 |
Antitumor |
142 |
9 |
 |
Antitumor |
147 |
10 |
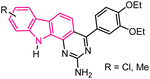 |
Antitumor |
148 |
11 |
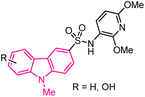 |
Anticancer |
149 |
12 |
 |
Anticancer |
150 |
13 |
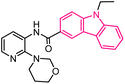 |
Anticancer |
149 |
14 |
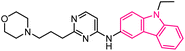 |
Anticancer |
149 |
15 |
 |
Anticancer |
151 |
16 |
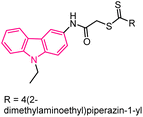 |
Anticancer |
152 |
17 |
 |
Anticancer |
153 |
18 |
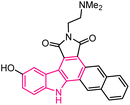 |
Anticancer |
153 |
19 |
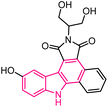 |
Anticancer |
153 |
20 |
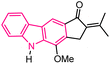 |
Anticancer |
154 |
21 |
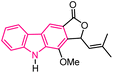 |
Anticancer |
154 |
22 |
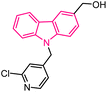 |
Anticancer |
149 |
23 |
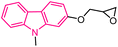 |
Anticancer |
149 |
24 |
 |
Anticancer |
155 |
25 |
 |
Anticancer |
155 |
26 |
 |
Breast cancer |
155 |
27 |
 |
Anticancer |
155 |
28 |
 |
Antibacterial |
156 |
29 |
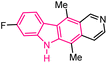 |
Antibacterial |
156 |
30 |
 |
Antibacterial |
156 |
31 |
 |
Antibacterial |
157 |
32 |
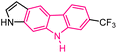 |
Kinesin spindle protein inhibitors |
158 |
33 |
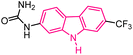 |
Kinesin spindle protein inhibitors |
158 |
34 |
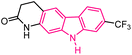 |
Kinesin spindle protein inhibitors |
158 |
35 |
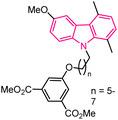 |
STAT3 inhibitors |
159 |
36 |
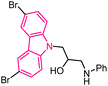 |
Neuroprotective agents |
160 |
37 |
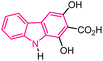 |
Neuroprotective agents |
161 |
38 |
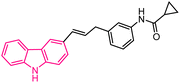 |
XO inhibitors |
162 |
39 |
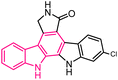 |
CDK5/p25 kinase inhibition |
163 |
40 |
 |
Pim kinase inhibitors |
164 |
41 |
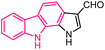 |
Pim kinase inhibitors |
164 |
42 |
 |
Pim kinase inhibitors |
165 |
43 |
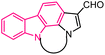 |
Pim kinase inhibitors |
166 |
44 |
 |
Antiplasmodial |
167 |
45 |
 |
Antiplasmodial |
167 |
46 |
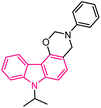 |
Antiplasmodial |
167 |
47 |
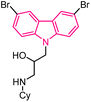 |
Antimalarial |
168 |
48 |
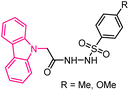 |
Antioxidant |
169 |
49 |
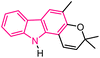 |
Antioxidant |
170 |
50 |
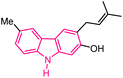 |
Anti-HIV |
171 |
51 |
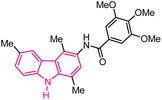 |
Antiproliferative agents |
172 |
52 |
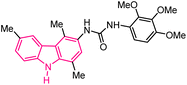 |
Antiproliferative agents |
172 |
53 |
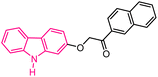 |
Antiproliferative agents |
173 |
54 |
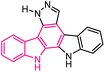 |
Antiproliferative agents |
174 |
55 |
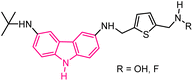 |
Telomerase inhibitors |
175 |
56 |
 |
PARP-1 inhibitor |
176 |
57 |
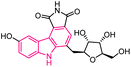 |
Chk-1 inhibitor |
177 |
58 |
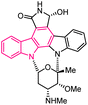 |
Chk-1 inhibitor |
177 |
59 |
 |
Anti-yeast |
178 |
60 |
 |
Antifungal |
179 |
4. Selective examples for carbazole-based material applications
In recent days, many material science research groups have been very much interested in the design and preparation of highly π-conjugated sulfur and nitrogen-based annulated carbazoles derivatives that could be readily applied in various materials applications such as organic light-emitting diodes (OLEDs), thermally activated delayed fluorescence (TADF), Mitochondria-targeted fluorescent (MTF), fluorescent properties, conductive polymers, etc. The selectively collected list of carbazoles and annulated carbazoles and their materials applications or properties are detailed and summarised in the Fig. 2–5.180–208
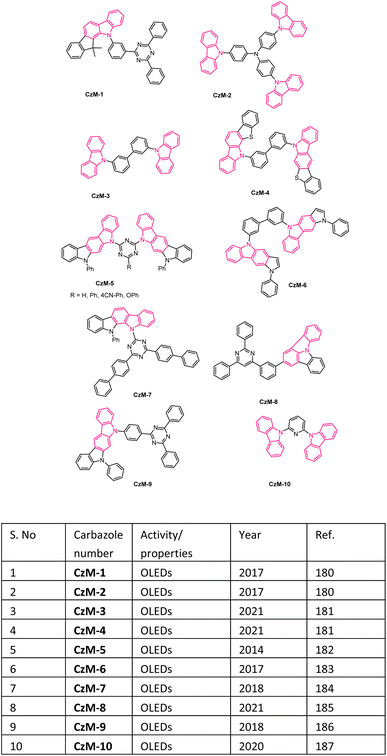 |
| Fig. 2 Organic light-emitting diodes (OLEDs) of carbazole analogs. | |
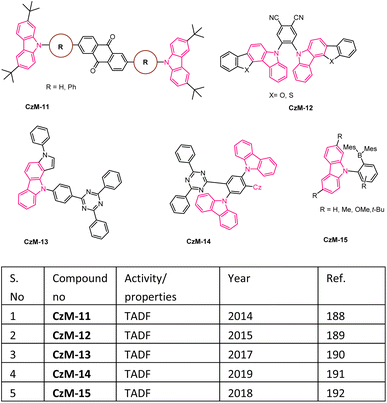 |
| Fig. 3 Thermally activated delayed fluorescence (TADF) of carbazole derivatives. | |
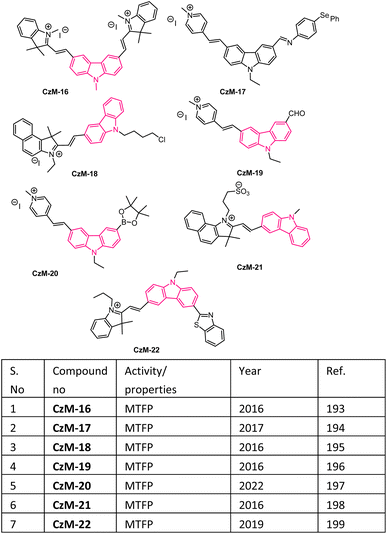 |
| Fig. 4 Mitochondria-targeted fluorescent probe (MTFP) of carbazole scaffolds. | |
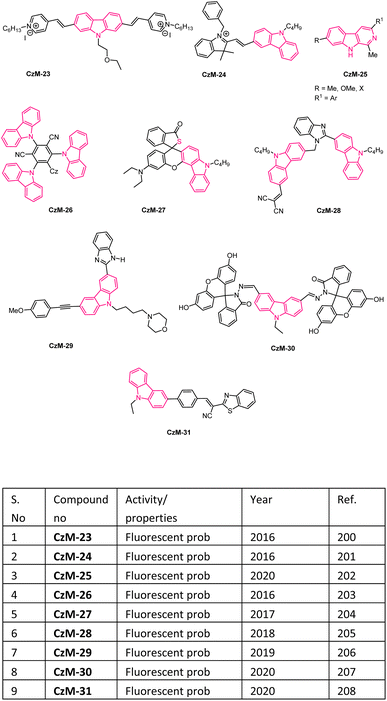 |
| Fig. 5 Fluorescent properties of carbazole scaffolds. | |
5. Conclusions
Over the past two decades, Lewis acid-mediated synthetic methods are remarkably considered as one of the significant approaches and an appropriate tool in modern organic synthesis which plays a vital role in discovering carbazole-based pharmaceutical drugs or carbazole-alkaloids and natural products. This review article mainly proves that the recent innovative cascade annulation strategy for synthesizing polyfunctionalized carbazoles and their related nitrogen-based complex heterocyclic compounds with poly aromatic compounds enabled by Lewis acid. Highly conjugated carbazole-based oligothiophenes and poly aromatic nitrogen heterocycles were synthesized via Lewis acid-mediated organic synthesis, including Friedel–Crafts arylation, electrocyclization, intramolecular cyclization, intramolecular oxidative C–N bond-formations, aromatization and cascade domino reactions under sustainable conditions. The highly substituted carbazole and their related heterocyclic carbazole compounds were found in several synthetic applications in medicinal chemistry, chemical biology, energy storage devices, and materials applications. Moreover, the review paper briefly summarised new synthetic strategies for producing carbazole derivatives which will assist academics and industries in identifying innovative, sustainable protocols for constructing poly-functionalized carbazoles and related highly complex heterocyclic compounds and discovering active pharmaceutical drugs or carbazole-based alkaloids and natural products.
Author contributions
VD wrote the major part of the paper. MPK is responsible for a list of reference paper collections; a few schemes and applications are described. GM, PA and CM partially wrote a few sections and supported manuscript corrections.
Conflicts of interest
There are no conflicts to declare.
Acknowledgements
Vasudevan Dhayalan expresses his gratitude to the DST-SERB for the Ramanujan Fellowship (Grant No. RJF/2020/000038), Core research grant (CRG/2022/001855) and the National Institute of Technology Puducherry, Karaikal, India for their assistance with research.
Notes and references
- J. M. Pedersen, W. R. Bowman, M. R. Elsegood, A. J. Fletcher and P. J. Lovell, J. Org. Chem., 2005, 70, 10615–10618 CrossRef CAS PubMed
. - M. W. Saif and R. B. Diasio, Clin. Colorectal Cancer, 2005, 5, 27–36 CrossRef CAS PubMed
. - M. R. Naffziger, B. O. Ashburn, J. R. Perkins and R. G. Carter, J. Org. Chem., 2007, 72, 9857–9865 CrossRef CAS PubMed
. - A. Ueno, T. Kitawaki and N. Chida, Org. Lett., 2008, 10, 1999–2002 CrossRef CAS PubMed
. - K. E. Knott, S. Auschill, A. Jäger and H.-J. Knölker, Chem. Commun., 2009, 1467–1469 RSC
. - G. G. Rajeshwaran and A. K. Mohanakrishnan, Org. Lett., 2011, 13, 1418–1421 CrossRef CAS PubMed
. - A. Caron, A. C. Hernandez-Perez and S. K. Collins, Org. Process Res. Dev., 2014, 18, 1571–1574 CrossRef CAS
. - A. E. Goetz, A. L. Silberstein, M. A. Corsello and N. K. Garg, J. Am. Chem. Soc., 2014, 136, 3036–3039 CrossRef CAS PubMed
. - P. Raju, G. Gobi Rajeshwaran and A. K. Mohanakrishnan, Eur. J. Org Chem., 2015, 2015, 7131–7145 CrossRef CAS
. - S. Wu, S. Harada, T. Morikawa and A. Nishida, Chem. Pharm. Bull., 2018, 66, 178–183 CrossRef CAS PubMed
. - H. Li, Q. Chen, Z. Lu and A. Li, J. Am. Chem. Soc., 2016, 138, 15555–15558 CrossRef CAS PubMed
. - O. m. Dilek, S. l. Patir, T. Tilki and E. Ertürk, J. Org. Chem., 2019, 84, 7901–7916 CrossRef CAS PubMed
. - V. Y. Shuvalov, V. A. Elisheva, A. S. Belousova, E. V. Arshinov, L. V. Glyzdinskaya, M. A. Vorontsova, S. A. Chernenko, A. S. Fisyuk and G. P. Sagitullina, Chem. Heterocycl. Compd., 2020, 56, 73–83 CrossRef CAS
. - O. Dilek, S. l. Patir, T. Tilki and E. Ertürk, J. Org. Chem., 2019, 84, 7901–7916 CrossRef CAS PubMed
. - T. Nishiyama, A. Matsuoka, R. Honda, T. Kitamura, N. Hatae and T. Choshi, Tetrahedron, 2020, 76, 131110 CrossRef CAS
. - J. Matsuoka, S. Inuki, Y. Matsuda, Y. Miyamoto, M. Otani, M. Oka, S. Oishi and H. Ohno, Chem.–Eur. J., 2020, 26, 11150–11157 CrossRef CAS PubMed
. - C. Alayrac, D. Schollmeyer and B. Witulski, Chem. Commun., 2009, 1464–1466 RSC
. - B. R. Rosen, E. W. Werner, A. G. O'Brien and P. S. Baran, J. Am. Chem. Soc., 2014, 136, 5571–5574 CrossRef CAS PubMed
. - S. B. Markad and N. P. Argade, Org. Lett., 2014, 16, 5470–5473 CrossRef CAS PubMed
. - W. M. O'Brien and G. F. Bagby, Pharmacotherapy, 1987, 7, 16–24 CrossRef PubMed
. - C. Ito, M. Itoigawa, A. Sato, C. M. Hasan, M. A. Rashid, H. Tokuda, T. Mukainaka, H. Nishino and H. Furukawa, J. Nat. Prod., 2004, 67, 1488–1491 CrossRef CAS PubMed
. - B. Vehar, M. Hrast, A. Kovač, J. Konc, K. Mariner, I. Chopra, A. O'Neill, D. Janežič and S. Gobec, Bioorg. Med. Chem., 2011, 19, 5137–5146 CrossRef CAS PubMed
. - T. Takeuchi, S. Oishi, T. Watanabe, H. Ohno, J.-i. Sawada, K. Matsuno, A. Asai, N. Asada, K. Kitaura and N. Fujii, J. Med. Chem., 2011, 54, 4839–4846 CrossRef CAS PubMed
. - A. A. Pieper, S. L. McKnight and J. M. Ready, Chem. Soc. Rev., 2014, 43, 6716–6726 RSC
. - Y. Feng, D. Li, Q. Wang, S. Wang, X. Meng, Z. Shao, M. Zhu and X. Wang, Sens. Actuators, B, 2016, 225, 572–578 CrossRef CAS
. - Z. Meng, H. Yu, L. Li, W. Tao, H. Chen, M. Wan, P. Yang, D. J. Edmonds, J. Zhong and A. Li, Nat. Commun., 2015, 6, 6096 CrossRef CAS PubMed
. - X. Wu, J. Kosaraju, W. Zhou and K. Y. Tam, ACS Chem. Neurosci., 2017, 8, 676–685 CrossRef CAS PubMed
. - T. Janosik, A. Rannug, U. Rannug, N. Wahlström, J. Slätt and J. Bergman, Chem. Rev., 2018, 118, 9058–9128 CrossRef CAS PubMed
. - F. Giraud, E. Pereira, F. Anizon and P. Moreau, Eur. J. Org Chem., 2019, 2019, 5025–5042 CrossRef CAS
. - T. Aggarwal and A. K. Verma, Org. Biomol. Chem., 2019, 17, 8330–8342 RSC
. - A. Banerjee, S. Kundu, A. Bhattacharyya, S. Sahu and M. S. Maji, Org. Chem. Front., 2021, 8, 2710–2771 RSC
. - J. Roy, A. K. Jana and D. Mal, Tetrahedron, 2012, 68, 6099–6121 CrossRef CAS
. - C. Graebe and C. Glaser, Adv. Cycloaddit., 1872, 163, 343–360 Search PubMed
. - D. Chakraborty, B. Barman and P. Bose, Tetrahedron, 1965, 21, 681–685 CrossRef CAS
. - H.-J. Knölker and K. R. Reddy, Chem. Rev., 2002, 102, 4303–4428 CrossRef PubMed
. - A. W. Schmidt, K. R. Reddy and H.-J. Knölker, Chem. Rev., 2012, 112, 3193–3328 CrossRef CAS PubMed
. - G. Zotti, G. Schiavon, S. Zecchin, J.-F. Morin and M. Leclerc, Macromolecules, 2002, 35, 2122–2128 CrossRef CAS
. - P. Gao, D. Cho, X. Yang, V. Enkelmann, M. Baumgarten and K. Müllen, Chem.–Eur. J., 2010, 16, 5119–5128 CrossRef CAS PubMed
. - J. Li and A. C. Grimsdale, Chem. Soc. Rev., 2010, 39, 2399–2410 RSC
. - N. Blouin and M. Leclerc, Acc. Chem. Res., 2008, 41, 1110–1119 CrossRef CAS PubMed
. - C. Wang, H. Dong, W. Hu, Y. Liu and D. Zhu, Chem. Rev., 2012, 112, 2208–2267 CrossRef CAS PubMed
. - X. Liu, Y. Xu and D. Jiang, J. Am. Chem. Soc., 2012, 134, 8738–8741 CrossRef CAS PubMed
. - J.-Y. Su, C.-Y. Lo, C.-H. Tsai, C.-H. Chen, S.-H. Chou, S.-H. Liu, P.-T. Chou and K.-T. Wong, Org. Lett., 2014, 16, 3176–3179 CrossRef CAS PubMed
. - F. Chen, Y. S. Hong, S. Shimizu, D. Kim, T. Tanaka and A. Osuka, Angew. Chem., Int. Ed., 2023, 62, e202302761 CrossRef PubMed
. - J. Zhang, W. Chen, S. Kalytchuk, K. F. Li, R. Chen, C. Adachi, Z. Chen, A. L. Rogach, G. Zhu and P. K. Yu, ACS Appl. Mater. Interfaces, 2016, 8, 11355–11365 CrossRef CAS PubMed
. - K. Ivaniuk, V. Cherpak, P. Stakhira, Z. Hotra, B. Minaev, G. Baryshnikov, E. Stromylo, D. Volyniuk, J. V. Grazulevicius and A. Lazauskas, J. Phys. Chem. C, 2016, 120, 6206–6217 CrossRef CAS
. - K. Albrecht, K. Matsuoka, D. Yokoyama, Y. Sakai, A. Nakayama, K. Fujita and K. Yamamoto, Chem. Commun., 2017, 53, 2439–2442 RSC
. - G. Li, J. Zheng, K. Klimes, Z.-Q. Zhu, J. Wu, H. Zhu and J. Li, ACS Appl. Mater. Interfaces, 2019, 11, 40320–40331 CrossRef CAS PubMed
. - Z.-J. Gao, T.-H. Yeh, J.-J. Xu, C.-C. Lee, A. Chowdhury, B.-C. Wang, S.-W. Liu and C.-H. Chen, ACS Omega, 2020, 5, 10553–10561 CrossRef CAS PubMed
. - P. Xu, X. Liu, L. Liu, W. Zhu, C. Li and M. Fang, J. Chin. Chem. Soc., 2021, 68, 106–113 CrossRef CAS
. - C. M. Hendrich, L. M. Bongartz, M. T. Hoffmann, U. Zschieschang, J. W. Borchert, D. Sauter, P. Krämer, F. Rominger, F. F. Mulks and M. Rudolph, Adv. Synth. Catal., 2021, 363, 549–557 CrossRef
. - E. Von Angerer and J. Prekajac, J. Med. Chem., 1986, 29, 380–386 CrossRef CAS PubMed
. - F. Song, D. Liu, X. Huo and D. Qiu, Arch. Pharm., 2022, 355, 2100277 CrossRef CAS PubMed
. - Y.-Q. Wang, X.-H. Li, Q. He, Y. Chen, Y.-Y. Xie, J. Ding, Z.-H. Miao and C.-H. Yang, Eur. J. Med. Chem., 2011, 46, 5878–5884 CrossRef CAS PubMed
. - D. Nettleton, T. Doyle, B. Krishnan, G. Matsumoto and J. Clardy, Tetrahedron Lett., 1985, 26, 4011–4014 CrossRef CAS
. - K. F. Kinzer and J. H. Cardellina, Tetrahedron Lett., 1987, 28, 925–926 CrossRef CAS
. - G. Lin and A. Zhang, Tetrahedron, 2000, 56, 7163–7171 CrossRef CAS
. - L. B. Kardono, C. K. Angerhofer, S. Tsauri, K. Padmawinata, J. M. Pezzuto and A. D. Kinghorn, J. Nat. Prod., 1991, 54, 1360–1367 CrossRef CAS PubMed
. - H. Cardellina II, M. P. Kirkup, R. E. Moore, J. S. Mynderse, K. Seff and C. J. Simmons, Tetrahedron Lett., 1979, 20, 4915–4916 CrossRef
. - L. M. Browne, K. L. Conn, W. Ayer and J. P. Tewari, Tetrahedron, 1991, 47, 3909–3914 CrossRef CAS
. - W. A. Ayer, P. A. Craw, Y.-t. Ma and S. Miao, Tetrahedron, 1992, 48, 2919–2924 CrossRef CAS
. - S. Routier, P. Peixoto, J.-Y. Mérour, G. Coudert, N. Dias, C. Bailly, A. Pierré, S. Léonce and D.-H. Caignard, J. Med. Chem., 2005, 48, 1401–1413 CrossRef CAS PubMed
. - S. Routier, J.-Y. Mérour, N. Dias, A. Lansiaux, C. Bailly, O. Lozach and L. Meijer, J. Med. Chem., 2006, 49, 789–799 CrossRef CAS PubMed
. - I. Iovel, K. Mertins, J. Kischel, A. Zapf and M. Beller, Angew. Chem., Int. Ed., 2005, 44, 3913–3917 CrossRef CAS PubMed
. - M. Angeli, M. Bandini, A. Garelli, F. Piccinelli, S. Tommasi and A. Umani-Ronchi, Org. Biomol. Chem., 2006, 4, 3291–3296 RSC
. - J. Kischel, I. Jovel, K. Mertins, A. Zapf and M. Beller, Org. Lett., 2006, 8, 19–22 CrossRef CAS PubMed
. - M. Sonntag and P. Strohriegl, Tetrahedron, 2006, 62, 8103–8108 CrossRef CAS
. - M. Rueping, B. J. Nachtsheim and W. Ieawsuwan, Adv. Synth. Catal., 2006, 348, 1033–1037 CrossRef CAS
. - J. Yadav, B. S. Reddy, S. Aravind, G. N. Kumar and A. S. Reddy, Tetrahedron Lett., 2007, 48, 6117–6120 CrossRef CAS
. - C. Unaleroglu and A. Yazici, Tetrahedron, 2007, 63, 5608–5613 CrossRef CAS
. - S.-J. Hong, S.-D. Jeong, J. Yoo, J. S. Kim, J. Yoon and C.-H. Lee, Tetrahedron Lett., 2008, 49, 4138–4141 CrossRef CAS
. - Z. Tu, B. R. Raju, T.-R. Liou, V. Kavala, C.-W. Kuo, Y. Jang, Y.-H. Shih, C.-C. Wang and C.-F. Yao, Tetrahedron, 2009, 65, 2436–2442 CrossRef CAS
. - V. Dhayalan, N. Ramesh and A. K. Mohanakrishnan, Synth. Commun., 2009, 39, 1241–1256 CrossRef CAS
. - A. K. Mohanakrishnan, V. Dhayalan, J. A. Clement, R. B. R. Sureshbabu and N. S. Kumar, Tetrahedron Lett., 2008, 49, 5850–5854 CrossRef CAS
. - V. Dhayalan, J. A. Clement, R. Jagan and A. K. Mohanakrishnan, Eur. J. Org Chem., 2009, 2009, 531–546 CrossRef
. - R. Sureshbabu, V. Saravanan, V. Dhayalan and A. K. Mohanakrishnan, Eur. J. Org Chem., 2011, 2011, 922–935 CrossRef
. - V. Dhayalan, R. Sureshbabu and A. K. Mohanakrishnan, Indian J. Chem., Sect. B: Org. Chem. Incl. Med. Chem., 2011, 50, 843 Search PubMed
. - V. Dhayalan and A. K. Mohanakrishnan, Synth. Commun., 2012, 42, 2149–2160 CrossRef CAS
. - V. Saravanan, T. Mageshwaran and A. K. Mohanakrishnan, J. Org. Chem., 2016, 81, 8633–8646 CrossRef CAS PubMed
. - P. Raju, T. Mageshwaran, B. M. Ramalingam and A. K. Mohanakrishnan, SynOpen, 2018, 02, 0246–0250 CrossRef CAS
. - B. Muthu Ramalingam and A. K. Mohanakrishnan, et al., J. Med. Chem., 2018, 61, 1285–1315 CrossRef CAS PubMed
. - C.-B. Chen, X.-F. Wang, Y.-J. Cao, H.-G. Cheng and W.-J. Xiao, J. Org. Chem., 2009, 74, 3532–3535 CrossRef CAS PubMed
. - M. Tiano and P. Belmont, J. Org. Chem., 2008, 73, 4101–4109 CrossRef CAS PubMed
. - T. G. Back, A. Pandyra and J. E. Wulff, J. Org. Chem., 2003, 68, 3299–3302 CrossRef CAS PubMed
. - D. Alonso, E. Caballero, M. Medarde and F. Tomé, Tetrahedron Lett., 2005, 46, 4839–4841 CrossRef CAS
. - J. Y. Lee, H. Ha, S. Bae, I. Han and J. M. Joo, Adv. Synth. Catal., 2016, 358, 3458–3470 CrossRef CAS
. - J.-Q. Wu, Z. Yang, S.-S. Zhang, C.-Y. Jiang, Q. Li, Z.-S. Huang and H. Wang, ACS Catal., 2015, 5, 6453–6457 CrossRef CAS
. - Y. Zhou and J. G. Verkade, Adv. Synth. Catal., 2010, 352, 616–620 CrossRef CAS
. - F. Jafarpour and H. Hazrati, Adv. Synth. Catal., 2010, 352, 363–367 CrossRef CAS
. - S. Chen, Y. Li, P. Ni, H. Huang and G.-J. Deng, Org. Lett., 2016, 18, 5384–5387 CrossRef CAS PubMed
. - J. Wang, H.-T. Zhu, Y.-F. Qiu, Y. Niu, S. Chen, Y.-X. Li, X.-Y. Liu and Y.-M. Liang, Org. Lett., 2015, 17, 3186–3189 CrossRef CAS PubMed
. - R. Gu, A. Hameurlaine and W. Dehaen, J. Org. Chem., 2007, 72, 7207–7213 CrossRef CAS PubMed
. - R. Gu, S. Van Snick, K. Robeyns, L. Van Meervelt and W. Dehaen, Org. Biomol. Chem., 2009, 7, 380–385 RSC
. - C. Ding, S. Tu, Q. Yao, F. Li, Y. Wang, W. Hu and A. Zhang, Adv. Synth. Catal., 2010, 352, 847–853 CrossRef CAS
. - M. L. Deb, S. Mazumder, B. Baruah and P. J. Bhuyan, Synthesis, 2010, 2010, 929–932 CrossRef
. - S. Tohyama, T. Choshi, K. Matsumoto, A. Yamabuki, K. Ikegata, J. Nobuhiro and S. Hibino, Tetrahedron Lett., 2005, 46, 5263–5264 CrossRef CAS
. - M. F. Martínez-Esperón, D. Rodríguez, L. Castedo and C. Saá, Org. Lett., 2005, 7, 2213–2216 CrossRef PubMed
. - A. R. Katritzky and L. Xie, J. Org. Chem., 1995, 60, 3707–3710 CrossRef CAS
. - A. K. Mohanakrishnan and P. C. Srinivasan, J. Org. Chem., 1995, 60, 1939–1946 CrossRef CAS
. - K. Liu and S. Zhang, ACS Med. Chem. Lett., 2015, 6, 894–897 CrossRef CAS PubMed
. - A. R. Katritzky, G. Zhang, L. Xie and I. Ghiviriga, J. Org. Chem., 1996, 61, 7558–7563 CrossRef CAS PubMed
. - Y. Miki, Y. Aoki, H. Miyatake, T. Minematsu and H. Hibino, Tetrahedron Lett., 2006, 47, 5215–5218 CrossRef CAS
. - C. O. Salas, F. J. Reboredo, J. C. Estévez, R. A. Tapia and R. J. Estévez, Synlett, 2009, 2009, 3107–3110 CrossRef
. - P. Manikandan, E. Sankar and A. K. Mohanakrishnan, Synth. Commun., 2022, 52, 1389–1396 CrossRef CAS
. - V. Saravanan and A. K. Mohanakrishnan, Synthesis, 2021, 53, 2304–2318 CrossRef CAS
. - J. A. Clement, R. Sivasakthikumaran, A. K. Mohanakrishnan, S. Sundaramoorthy and D. Velmurugan, Eur. J. Org Chem., 2011, 2011, 569–577 CrossRef
. - V. Saravanan, T. Mageshwaran and A. K. Mohanakrishnan, J. Org. Chem., 2016, 81, 8633–8646 CrossRef CAS PubMed
. - R. K. Varshnaya and P. Banerjee, J. Org. Chem., 2019, 84, 1614–1623 CrossRef CAS PubMed
. - C. Raji Reddy, R. Rani Valleti and U. Dilipkumar, Chem.–Eur. J., 2016, 22, 2501–2506 CrossRef CAS PubMed
. - X.-S. Li, Y.-P. Han, X.-Y. Zhu, Y. Xia, W.-X. Wei, M. Li and Y.-M. Liang, Adv. Synth. Catal., 2018, 360, 4441–4445 CrossRef CAS
. - Y. Qiu, J. Zhou, C. Fu and S. Ma, Chem.–Eur. J., 2014, 20, 14589–14593 CrossRef CAS PubMed
. - K. Huang, G. Sheng, P. Lu and Y. Wang, Org. Lett., 2017, 19, 4114–4117 CrossRef CAS PubMed
. - S. Wang, Z. Chai, Y. Wei, X. Zhu, S. Zhou and S. Wang, Org. Lett., 2014, 16, 3592–3595 CrossRef CAS PubMed
. - T. Tsuchimoto, H. Matsubayashi, M. Kaneko, E. Shirakawa and Y. Kawakami, Angew. Chem., Int. Ed., 2005, 44, 1336–1340 CrossRef CAS PubMed
. - H. Jiang, K. Li, S. Dong, Z. Chen and G. Yin, Eur. J. Org Chem., 2023, e202300598 CrossRef CAS
. - S. H. Cho, J. Yoon and S. Chang, J. Am. Chem. Soc., 2011, 133, 5996–6005 CrossRef CAS PubMed
. - H. Jiang, K. Li, S. Dong, Z. Chen and G. Yin, Eur. J. Org Chem., 2023, 26, e202300598 CrossRef CAS
. - X. Tian, L. Song and A. S. K. Hashmi, Angew. Chem., Int. Ed., 2020, 59, 12342–12346 CrossRef CAS
. - W. Yang, J. Zhou, B. Wang and H. Ren, Chem.–Eur. J., 2011, 17, 13665–13669 CrossRef CAS PubMed
. - G. G. Rajeshwaran and A. K. Mohanakrishnan, Org. Lett., 2011, 13, 1418–1421 CrossRef CAS PubMed
. - Z. Chen, W. Huang, L. Yi, X. Dong, K. Sheng, M. Li, R. Bai, A. Y. Sidorenko, J. Huang and Y. Gu, Green Chem., 2022, 24, 2919–2926 RSC
. - F. Wu, W. Huang, Yiliqi, J. Yang and Y. Gu, Adv. Synth. Catal., 2018, 360, 3318–3330 CrossRef CAS
. - Y. Gu, W. Huang, S. Chen and X. Wang, Org. Lett., 2018, 20, 4285–4289 CrossRef CAS PubMed
. - J.-F. Zou, H. Wang, L. Li, Z. Xu, K.-F. Yang and L.-W. Xu, RSC Adv., 2014, 4, 47272–47277 RSC
. - K. Paul, K. Bera, S. Jalal, S. Sarkar and U. Jana, Org. Lett., 2014, 16, 2166–2169 CrossRef CAS
. - M. Faltracco, M. Damian and E. Ruijter, Org. Lett., 2021, 23, 7592–7596 CrossRef CAS
. - C. Venkatesh, H. Ila, H. Junjappa, S. Mathur and V. Huch, J. Org. Chem., 2002, 67, 9477–9480 CrossRef CAS PubMed
. - S. S. K. Boominathan, G. C. Senadi, J. K. Vandavasi, J. Y.-F. Chen and J.-J. Wang, Chem.–Eur. J., 2015, 21, 3193–3197 CrossRef CAS
. - Y. Qiao, X. X. Wu, Y. Zhao, Y. Sun, B. Li and S. Chen, Adv. Synth. Catal., 2018, 360, 2138–2143 CrossRef CAS
. - B. M. Ramalingam, V. Saravanan and A. K. Mohanakrishnan, Org. Lett., 2013, 15, 3726–3729 CrossRef CAS
. - D. Facoetti, G. Abbiati and E. Rossi, Eur. J. Org Chem., 2009, 2009, 2872–2882 CrossRef
. - S. Pramanik, S. Chatterjee, R. Banerjee and C. Chowdhury, Org. Lett., 2022, 24, 1895–1900 CrossRef CAS
. - Y. Qiu, W. Kong, C. Fu and S. Ma, Org. Lett., 2012, 14, 6198–6201 CrossRef CAS
. - Y. Li, H. Zou, J. Gong, J. Xiang, T. Luo, J. Quan, G. Wang and Z. Yang, Org. Lett., 2007, 9, 4057–4060 CrossRef CAS PubMed
. - J. Wu, D. Wang, H. Wang, F. Wu, X. Li and B. Wan, Org. Biomol. Chem., 2014, 12, 6806–6811 RSC
. - P. Raju and A. K. Mohanakrishnan, Eur. J. Org Chem., 2016, 2016, 4361–4371 CrossRef CAS
. - N. J. Truax, F. Banales Mejia, D. O. Kwansare, M. M. Lafferty, M. H. Kean and E. T. Pelkey, J. Org. Chem., 2016, 81, 6808–6815 CrossRef CAS PubMed
. - S. Sakthivel and R. Balamurugan, J. Org. Chem., 2018, 83, 12171–12183 CrossRef CAS PubMed
. - S. Yuan, G. Guerra Faura, H. E. Areheart, N. E. Peulen and S. France, Molecules, 2022, 27, 8344 CrossRef CAS PubMed
. - H. Xu, B. Wang, F.-Y. Li and J.-Y. Wang, J. Org. Chem., 2023, 88, 2703–2713 CrossRef CAS PubMed
. - W. Xu, G. Wang, X. Xie and Y. Liu, Org. Lett., 2018, 20, 3273–3277 CrossRef CAS PubMed
. -
(a) C. Paoletti, J. B. Le Pecq, N. Dat-Xuong, P. Juret, H. Garnier, J. L. Amiel and J. Rouesse, Cancer Chemo- and Immunopharmacology, 1980, pp. 107–123 Search PubMed
;
(b) T. Indumathi, A. Muthusankar, P. Shanmughavel and K. J. R. Prasad, MedChemComm, 2013, 4, 450–455 RSC
. - A. Rozovsky, E. Regozin, M. Oron-Herman, A. Albeck and G. Gellerman, Eur. J. Org. Chem., 2015, 2015, 1811–1818 CrossRef CAS
. - C. Ito, M. Itoigawa, A. Sato, C. M. Hasan, M. A. Rashid, H. Tokuda, T. Mukainaka, H. Nishino and H. Furukawa, J. Nat. Prod., 2004, 67, 1488–1491 CrossRef CAS PubMed
. - W. Lin, Y. Wang, S. Lin, C. Li, C. Zhou, S. Wang, H. Huang, P. Liu, G. Ye and X. Shen, Eur. J. Med. Chem., 2012, 47, 214–220 CrossRef CAS PubMed
. - R. Mori, A. Kato, K. Komenoi, H. Kurasaki, T. Iijima, M. Kawagoshi, Y. B. Kiran, S. Takeda, N. Sakai and T. Konakahara, Eur. J. Med. Chem., 2014, 82, 16–35 CrossRef CAS PubMed
. - C. S. Francisco, L. R. Rodrigues, N. M. F. S. A. Cerqueira, A. M. F. Oliveira-Campos and A. P. Esteves, Eur. J. Med. Chem., 2014, 87, 298–305 CrossRef CAS PubMed
. - L. Vairavelu, M. Zeller and K. J. Rajendra Prasad, Bioorg. Chem., 2014, 54, 12–20 CrossRef CAS
. - Y. Liu, Y. Wu, l. Sun, Y. Gu and L. Hu, Eur. J. Med. Chem., 2020, 191, 112181 CrossRef CAS PubMed
. - B. Tylińska and B. Wiatrak, Biology, 2021, 10, 564 CrossRef PubMed
. - C. Ito, M. Itoigawa, K. Nakao, T. Murata, N. Kaneda and H. Furukawa, J. Nat. Med., 2012, 66, 357–361 CrossRef CAS PubMed
. - G. A. Çiftçi, H. E. Temel, Ş. U. Yıldırım, Z. A. Kaplancıklı, M. D. Altıntop and L. Genç, Med. Chem. Res., 2013, 22, 3751–3759 CrossRef
. -
(a) S. Routier, P. Peixoto, J.-Y. Mérour, G. Coudert, N. Dias, C. Bailly, A. Pierré, S. Léonce and D.-H. Caignard, J. Med. Chem., 2005, 48, 1401–1413 CrossRef CAS PubMed
;
(b) S. Routier, J.-Y. Mérour, N. Dias, A. Lansiaux, C. Bailly, O. Lozach and L. Meijer, J. Med. Chem., 2006, 49, 789–799 CrossRef CAS PubMed
. - W. Maneerat, T. Ritthiwigrom, S. Cheenpracha and S. Laphookhieo, Phytochem. Lett., 2012, 5, 26–28 CrossRef CAS
. - C. Ito, M. Itoigawa, K. Nakao, T. Murata, N. Kaneda and H. Furukawa, J. Nat. Med., 2012, 66, 357–361 CrossRef CAS PubMed
. - B. Vehar, M. Hrast, A. Kovač, J. Konc, K. Mariner, I. Chopra, A. O'Neill, D. Janežič and S. Gobec, Bioorg. Med. Chem., 2011, 19, 5137–5146 CrossRef CAS PubMed
. - R. Eswaramoorthy, H. Hailekiros, F. Kedir and M. Endale, Adv. Appl. Bioinf. Chem., 2021, 14, 13–24 Search PubMed
. - T. Takeuchi, S. Oishi, T. Watanabe, H. Ohno, J.-i. Sawada, K. Matsuno, A. Asai, N. Asada, K. Kitaura and N. Fujii, J. Med. Chem., 2011, 54, 4839–4846 CrossRef CAS PubMed
. - C. Saturnino, C. Palladino, M. Napoli, M. S. Sinicropi, A. Botta, M. Sala, A. Carcereri de Prati, E. Novellino and H. Suzuki, Eur. J. Med. Chem., 2013, 60, 112–119 CrossRef CAS PubMed
. - A. A. Pieper, S. L. McKnight and J. M. Ready, Chem. Soc. Rev., 2014, 43, 6716–6726 RSC
. - K. Liu and S. Zhang, ACS Med. Chem. Lett., 2015, 6, 894–897 CrossRef CAS PubMed
. - B. P. Bandgar, L. K. Adsul, H. V. Chavan, S. N. Shringare, B. L. Korbad, S. S. Jalde, S. V. Lonikar, S. H. Nile and A. L. Shirfule, Bioorg. Med. Chem., 2012, 20, 5649–5657 CrossRef CAS PubMed
. - P. Raju, G. Gobi Rajeshwaran and A. K. Mohanakrishnan, Eur. J. Org Chem., 2015, 2015, 7131–7145 CrossRef CAS
. - F. Giraud, R. Akué-Gédu, L. Nauton, N. Candelon, E. Debiton, V. Théry, F. Anizon and P. Moreau, Eur. J. Med. Chem., 2012, 56, 225–236 CrossRef CAS PubMed
. - V. Suchaud, L. Gavara, E. Saugues, L. Nauton, V. Théry, F. Anizon and P. Moreau, Bioorg. Med. Chem., 2013, 21, 4102–4111 CrossRef CAS PubMed
. - F. Giraud, M. Bourhis, L. Nauton, V. Théry, L. Herfindal, S. O. Døskeland, F. Anizon and P. Moreau, Bioorg. Chem., 2014, 57, 108–115 CrossRef CAS PubMed
. - Z. Bouaziz, S. Issa, J. Gentili, A. Gratz, A. Bollacke, M. Kassack, J. Jose, L. Herfindal, G. Gausdal, S. O. Døskeland, C. Mullié, P. Sonnet, C. Desgrouas, N. Taudon, G. Valdameri, A. Di Pietro, M. Baitiche and M. Le Borgne, J. Enzyme Inhib. Med. Chem., 2015, 30, 180–188 CrossRef CAS PubMed
. - J. Molette, J. Routier, N. Abla, D. Besson, A. Bombrun, R. Brun, H. Burt, K. Georgi, M. Kaiser, S. Nwaka, M. Muzerelle and A. Scheer, ACS Med. Chem. Lett., 2013, 4, 1037–1041 CrossRef CAS PubMed
. - İ. Çapan, M. Hawash, N. Jaradat, Y. Sert, R. Servi and İ. Koca, BMC Chem., 2023, 17, 60 CrossRef PubMed
. - Y. Y. Kok, L. Y. Mooi, K. Ahmad, M. A. Sukari, N. Mat, M. Rahmani and A. M. Ali, Molecules, 2012, 17, 4651–4660 CrossRef CAS PubMed
. - K. M. Meragelman, T. C. McKee and M. R. Boyd, J. Nat. Prod., 2000, 63, 427–428 CrossRef CAS PubMed
. - A. Panno, M. S. Sinicropi, A. Caruso, H. El-Kashef, J.-C. Lancelot, G. Aubert, A. Lesnard, T. Cresteil and S. Rault, J. Heterocycl. Chem., 2014, 51, E294–E302 CrossRef CAS
. - L.-C. Chen, S.-H. Juang, K.-M. Chang, C.-C. Tzeng, J.-J. Chen, I.-L. Chen and T.-C. Wang, Chem. Pharm. Bull., 2014, 62, 106–111 CrossRef CAS PubMed
. - B. Douara, Y. J. Esvan, E. Pereira, F. Giraud, Y. L. Volodina, D. N. Kaluzhny, A. A. Shtil, F. Anizon and P. Moreau, Tetrahedron, 2018, 74, 892–901 CrossRef CAS
. - C. Uvarani, N. Jaivel, M. Sankaran, K. Chandraprakash, A. Ata and P. S. Mohan, Fitoterapia, 2014, 94, 10–20 CrossRef CAS PubMed
. - D. Dunn, J. Husten, M. A. Ator and S. Chatterjee, Bioorg. Med. Chem. Lett., 2007, 17, 542–545 CrossRef CAS PubMed
. - S. Ichikawa, N. Tatebayashi and A. Matsuda, J. Org. Chem., 2013, 78, 12065–12075 CrossRef CAS PubMed
. - K. Sakano, K. Ishimaru and S. Nakamura, J. Antibiot., 1980, 33, 683–689 CrossRef CAS PubMed
. - N. M. Cuong, H. Wilhelm, A. Porzel, N. Arnold and L. Wessjohann, Nat. Prod. Res., 2008, 22, 950–954 CrossRef PubMed
. - K. Guo, H. Wang, Z. Wang, C. Si, C. Peng, G. Chen, J. Zhang, G. Wang and B. Wei, Chem. Sci., 2017, 8, 1259–1268 RSC
. - A. Arai, H. Sasabe, K. Nakao, Y. Masuda and J. Kido, Chem.–Eur. J., 2021, 27, 4971–4976 CrossRef CAS PubMed
. - D. Zhang, L. Duan, Y. Li, H. Li, Z. Bin, D. Zhang, J. Qiao, G. Dong, L. Wang and Y. Qiu, Adv. Funct. Mater., 2014, 24, 3551–3561 CrossRef CAS
. - J. M. Choi, J. H. Kim, Y. J. Kang and J. Y. Lee, Org. Electron., 2017, 49, 393–399 CrossRef CAS
. - Y. Li, D. Zhang and L. Duan, Org. Electron., 2018, 57, 53–59 CrossRef CAS
. - Y. Hiraga, R. Kuwahara and T. Hatta, Tetrahedron, 2021, 94, 132317 CrossRef CAS
. - D. Zhang, X. Song, M. Cai, H. Kaji and L. Duan, Adv. Mater., 2018, 30, 1705406 CrossRef PubMed
. - X. Zheng, F. Cao, C. Wang, T. Tsuboi, Y. Zhu, Q. Ai, C. Deng, D. Wang, L. Su, Z. Liu and Q. Zhang, J. Mater. Chem. C, 2020, 8, 10021–10030 RSC
. - Q. Zhang, H. Kuwabara, W. J. Potscavage Jr, S. Huang, Y. Hatae, T. Shibata and C. Adachi, J. Am. Chem. Soc., 2014, 136, 18070–18081 CrossRef CAS PubMed
. - D. R. Lee, S.-H. Hwang, S. K. Jeon, C. W. Lee and J. Y. Lee, Chem. Commun., 2015, 51, 8105–8107 RSC
. - J. H. Kim, M. Eum, T. H. Kim and J. Y. Lee, Dyes Pigm., 2017, 136, 529–534 CrossRef CAS
. - C. S. Oh, H. L. Lee, S. H. Han and J. Y. Lee, Chem.–Eur. J., 2019, 25, 642–648 CrossRef CAS PubMed
. - Y. H. Lee, S. Park, J. Oh, S.-J. Woo, A. Kumar, J.-J. Kim, J. Jung, S. Yoo and M. H. Lee, Adv. Opt. Mater., 2018, 6, 1800385 CrossRef
. - G. Wang, H. Chen, X. Chen and Y. Xie, RSC Adv., 2016, 6, 18662–18666 RSC
. - C. Sun, W. Du, P. Wang, Y. Wu, B. Wang, J. Wang and W. Xie, Biochem. Biophys. Res. Commun., 2017, 494, 518–525 CrossRef CAS PubMed
. - J. Xu, J. Pan, X. Jiang, C. Qin, L. Zeng, H. Zhang and J. F. Zhang, Biosens. Bioelectron., 2016, 77, 725–732 CrossRef CAS PubMed
. - Y. Feng, D. Li, Q. Wang, S. Wang, X. Meng, Z. Shao, M. Zhu and X. Wang, Sens. Actuators, B, 2016, 225, 572–578 CrossRef CAS
. - L. Xu, J. Yu, Y. Wang, Y. Chen, X. Zhang, R. Han, J. Jing, R. Zhang and X. Zhang, Dyes Pigm., 2022, 207, 110622 CrossRef CAS
. - Y. Liu, K. Li, K.-X. Xie, L.-L. Li, K.-K. Yu, X. Wang and X.-Q. Yu, Chem. Commun., 2016, 52, 3430–3433 RSC
. - W. Gao, Y. Ma and W. Lin, Analyst, 2019, 144, 4972–4977 RSC
. - Y. Liu, F. Meng, L. He, X. Yu and W. Lin, Chem. Commun., 2016, 52, 8838–8841 RSC
. - J.-Y. Wang, Z.-R. Liu, M. Ren, X. Kong, K. Liu, B. Deng and W. Lin, Sens. Actuators, B, 2016, 236, 60–66 CrossRef CAS
. - V. Y. Shuvalov, V. A. Elisheva, A. S. Belousova, E. V. Arshinov, L. V. Glyzdinskaya, M. A. Vorontsova, S. A. Chernenko, A. S. Fisyuk and G. P. Sagitullina, Chem. Heterocycl. Compd., 2020, 56, 73–83 CrossRef CAS
. - J. Zhang, W. Chen, S. Kalytchuk, K. F. Li, R. Chen, C. Adachi, Z. Chen, A. L. Rogach, G. Zhu, P. K. N. Yu, W. Zhang, K. W. Cheah, X. Zhang and C.-S. Lee, ACS Appl. Mater. Interfaces, 2016, 8, 11355–11365 CrossRef CAS PubMed
. - R. Guo, Q. Wang and W. Lin, J. Fluoresc., 2017, 27, 1969–1974 CrossRef CAS PubMed
. - L. Kong, L. Yang, G.-b. Zhang, Q.-y. Chen, H. Wang, X.-p. Gan, H. Li, H.-p. Zhou, J.-x. Yang and Y.-p. Tian, J. Mater. Sci., 2018, 53, 921–936 CAS
. - P. Ning, L. Hou, Y. Feng, G. Xu, Y. Bai, H. Yu and X. Meng, Chem. Commun., 2019, 55, 1782–1785 RSC
. - N. Wang, W. Xu, D. Song and P. Ma, Spectrochim. Acta, Part A, 2020, 227, 117692 CrossRef CAS PubMed
. - M. Peng, J. Yin and W. Lin, Spectrochim. Acta, Part A, 2020, 224, 117310 CrossRef CAS PubMed
.
Footnote |
† Dedicated to Prof. A. K. Mohanakrishnan. |
|
This journal is © The Royal Society of Chemistry 2023 |
Click here to see how this site uses Cookies. View our privacy policy here.