DOI:
10.1039/D4RA07485H
(Review Article)
RSC Adv., 2024,
14, 39230-39241
Chemistry and properties of fluorescent pyrazole derivatives: an approach to bioimaging applications†
Received
19th October 2024
, Accepted 28th November 2024
First published on 11th December 2024
Abstract
Fluorescent bioimaging is a crucial technique for in vivo studies in real cell samples, providing vital information about the metabolism of ions or molecules of biological and pharmaceutical significance. This technique typically uses probes based on organic small-molecule fluorophores, with N-heteroaromatic scaffolds playing an essential role due to their exceptional electronic properties and biocompatibility. Among these, pyrazole derivatives stand out as particularly promising due to their high synthetic versatility and structural diversity. This review highlights prominent examples from the period 2020–2024, focusing on the chemistry, properties, and bioimaging applications of fluorescent pyrazole derivatives. By highlighting the latest advancements in this field, this manuscript aims to inspire and motivate researchers, emphasizing the potential impact of this work on the future of bioimaging.
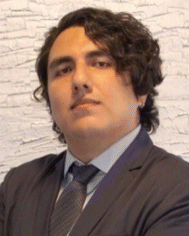 Santiago Melo-Hernández | Santiago Melo-Hernandez was born in Bogotá (Colombia) and received BSc degrees in Chemistry (2020) and Microbiology (2021), both at the Universidad de Los Andes (Bogotá). Currently, he works as a patent advisor at a law firm, being in close contact with the latest developments in different industries, especially in the pharmaceutical and biotechnology fields. His research interest focuses on synthesising compounds with potential biological activity and biological evaluation to develop new therapeutic and/or diagnostic leads. |
 María-Camila Ríos | Maria-Camila Rios was born in Medellin (Colombia); she received her BSc degree in 2021 and her MSc in 2024 at the Universidad de Los Andes (Bogotá). Currently, she works as a research assistant at the bioorganic compounds research group in the same institution under the supervision of Prof. Jaime Portilla. Her research interest focuses on synthesising heterocyclic compounds with biological or photophysical relevance. |
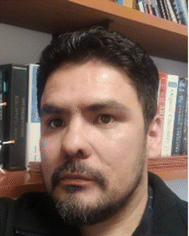 Jaime Portilla | Jaime Portilla was born in Cali (Colombia); he is a research professor at the Department of Chemistry of the Universidad de Los Andes in Bogotá, where he led the bioorganic compounds research group in 2008. He completed his PhD in Organic Synthesis under the supervision of Prof. J. Quiroga (2007) at the Universidad del Valle (Cali). His current research interests involve the areas of supramolecular chemistry and molecular recognition. His research group focuses on eco-compatible organic synthesis approaches, predominantly in 5 : 6 aza-fused heterocyclic compounds with biological or photophysical potential. |
1 Introduction
In recent years, non-invasive optical techniques, such as fluorescence-based bioimaging, have emerged as rapid and efficient tools for real-time monitoring of biological processes in live tissues and cells.1–3 These methods are crucial for both basic biological research and clinical applications, including therapeutic and diagnostic purposes, as they enable the testing of metabolic processes and the monitoring of the changes in biochemical indicators and biomarkers. The obtained results can be achieved using affordable and user-friendly devices such as fluorescence and confocal microscopes.4–6 Applications of these methods include general cell staining,7–9 labelling of subcellular structures,10–12 and the detection of ions or small molecules, such as Cu2+, reactive oxygen species, and reactive nitrogen species (ROS or RNS).13–17 These techniques also facilitate the assessment of intracellular conditions, including pH18–20 and hypoxia,21–23 which are important for sensing cancer,24–26 bacterial infections,27–29 ischemic injury,30–32 and even for theragnostics (a combination of therapy and diagnostics).33–35 When a bioimage is required for detection, the sensing can be achieved by monitoring changes in fluorescence, either a turn-on or turn-off response, resulting from the interaction of the probe with the target analyte or cells1,2,4,5 (Fig. 1a).
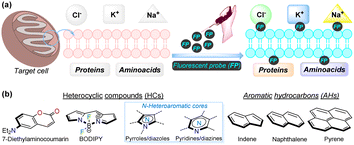 |
| Fig. 1 (a) Pictorial depiction of bioimaging acquisition by fluorescent probes. (b) Structural cores of several fluorophores used for bioimaging. | |
Considering the broad scope of applicability described above, there has been significant interest from both academia and industry in developing suitable systems, platforms, and methodologies for bioimaging applications.1–35 However, it remains challenging to identify novel, highly sensitive, and selective probes with good physiological compatibility.36–38 Many fluorescent scaffolds have been explored to develop new molecular probes tailored for bioimaging in this field. Various reported fluorophores include those containing heterocyclic rings, such as coumarin,39,40 boron complexes (e.g., BODIPY),41,42 and N-heteroaromatic cores (e.g., pyrroles, diazoles, pyridines, diazines, etc.).17,43,44 Additionally, probes based on aromatic hydrocarbons (e.g., indane, naphthalene, pyrene, tetraphenylethene, among others) have also been explored, despite their relatively lower biocompatibility34,45–47 (Fig. 1b).
Fluorophores based on N-heteroaromatic cores have been extensively explored for various biological and photophysical applications, as the heteroatom imparts important electronic properties to the probes. These compounds are usually stable17,43,44 and exhibit excellent synthetic versatility, particularly in terms of ring construction and subsequent functionalization reactions.43,44,48,49 Thus, exploration of fluorescent N-heterocyclic compounds remains an ongoing challenge, with continuous efforts aimed at improving their scope and applicability. Many organic compounds have been widely studied in the field of organic materials in recent years,43,50,51 leading to the successful application of porphyrins,52,53 poly-pyrroles,54 boron dipyrrolomethane (BODIPY),55,56 and certain 5
:
6 aza-fused diazoles with key dipolar motifs57–59 (Fig. 2a). Specifically, diverse pyrazole derivatives have shown fluorescent properties with high quantum yields and good photostability, thermostability, solvatofluorochromism, etc.;38,60–62 however, what stands out most in pyrazole derivatives is their high synthetic versatility, from the construction of the simple and fused ring to the functionalization reactions of the same43,63–65 (Fig. 2b).
 |
| Fig. 2 (a) Structure of some fluorescent N-heterocyclic dyes, (b) applications in detection chemistry, and (c) general syntheses of pyrazole derivatives. | |
Considering bioimaging applications, pyrazole derivatives have been reported to have good membrane permeability and biocompatibility, making them suitable as bioactive and biosensing compounds, and, due to their N-donor character,60–65 also ideal for cation detection in vivo.17,62,66 Many fluorescent pyrazoles for bioimaging purposes have been reported, with the pyrazoline ring being more frequent than the aromatic core; still, their fluorescent property is usually based on their substituents or fused rings (Fig. 2b). Therefore, and as a follow-up to the last review by our group in this regard,17 this review covers the chronological reports made in the previous five years (2020–2024) on pyrazole derivatives for use in bioimaging. The discussion focuses on analysing five works per year, covering the compounds' syntheses and their bioimaging properties.
To better understand the purpose of this review, Table 1 presents a summary with the most pertinent results for some of the probes analysed. Specifically, five examples matching each of the years explored are shown, in which the syntheses and applications date are detailed. Table 1 and Fig. 2c show how pyrazoline, pyrazole, and fused pyrazole rings are built by cyclization reactions of 1,3-biselectrophilic reagents with hydrazine derivatives; likewise, the appropriate functionalization or derivatization of pyrazoles for different applications is also shown. The primary aim of this work is to share the knowledge gained on the chemistry of pyrazole derivatives and their photophysical pertinency.17,43,44,62–65 Thus, we hope that this review manuscript will be a helpful contribution to further researchers aiming for novel fluorophores synthesis for bioimaging applications.
Table 1 Overview of some pyrazole derivatives for bioimaging application of 2020 to 2024a
Section, Probe |
General probe synthesis |
Application data |
Complete reaction conditions and yields are shown, which is not always common in article schematics. |
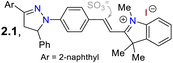 |
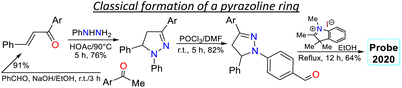 |
LOD/turn-on = 80 nM at 480 nm in 5% PBS/DMF, Ф = 13% at 640 nm.67 Mitochondrial sensing |
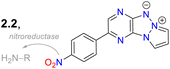 |
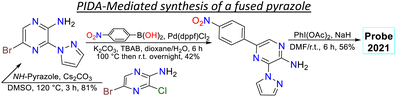 |
LOD/turn-on = 18.6 ng L−1, Ф = 76%/R-NH2 at 546 nm in DMSO, τ = 6.65 ns.68 Hypoxia sensing |
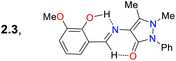 |
 |
LOD/turn-on = 720 nM at 518 nm in EtOH.69 Al+3 sensing in human colon living cells (HP-29) |
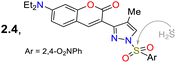 |
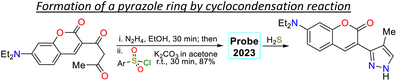 |
LOD/turn-on = 18.7 nM, Ф = 27% at 500 nm in MeCN.70 H2S sensing in MCF-7 cells |
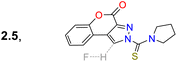 |
 |
LOD = 4.62 nM/turn-on at 492 nm in MeCN.71 F− sensing in T24 cells |
2 Articles analysed
2.1. Probes reported in 2020
First, Zhang et al.67 reported the synthesis and long-term bioimaging of the pyrazoline-BODIPY hybrid probe 4 and nanoparticles (NPs) 4-NPs for ultrafast cell staining. Probe 4 was obtained in a 22% yield by the Vilsmeier–Haack reaction of N-phenylpyrazoline 1, yielding the N-(4-formylphenyl)pyrazoline 2. Then, a one-pot reaction of 2 with 2,4-dimethylpyrrole (3) and BF3 offered 4. The probe exhibited an absorption band (λabs) at 499 nm and an emission band (λem) at 511 nm with a fluorescence quantum yield (ΦF) of 30%, attributed to an intramolecular charge transfer (ICT) from the pyrazoline ring to the BODIPY ring. The solvatofluorochromism of 4 showed a blue-shift with a decreased fluorescence intensity in polar solvents; however, an opposite result was observed in non-polar solvents. The rotational restriction around the BODIPY ring indicated a twisted ICT (TICT)-locally excited-state effect, which could explains their findings. This probe showed a high ΦF in aqueous medium due to an aggregation-induced emission (AIE) process, and it was applied to obtain NPs that were tested on HeLa and A549 cells, which revealed that the fluorescence intensity had a positive dependency on the time of incubation and concentration of 4-NPs. The in vivo fluorescence of the probe injected in the tumours of a mouse model was also explored, staining the tumour for up to 12 days, indicating this was probe suitable for long-term, non-invasive tumour progression monitoring (Scheme 1a).
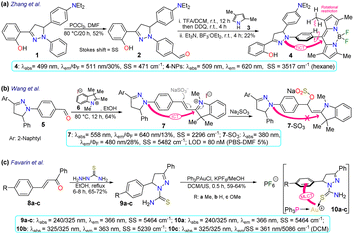 |
| Scheme 1 Synthesis and optical data of pyrazolines (a) 4, (b) 7, and (c) 10a–c. | |
Subsequently, Wang et al.68 employed probe 7 for in vivo sulfite detection in the mitochondria. This probe was synthesised in a 64% yield via the condensation of N-(4-formylphenyl)pyrazoline with the indolinium salt 6. The λabs at 558 nm and λem at 640 nm with ΦF of 13% for 6 showed visible changes in the presence of sulfite by interruption of the ICT process due to a break in the π-conjugation upon the anion addition to the salt, acting as a Michael acceptor. The observed changes showed up as a blueshift to 380 nm and 480 nm with a ΦF of 28%, with a reported limit of detection (LOD) of 80 nM for sulfite. This probe was designed to be located in the mitochondria due to the interaction of the negative charge of the mitochondrial membrane and the positive charge of the indolinium salt, which served as the guiding group. Green emission was observed in the presence of sulfite, indicating that it could efficiently sense exogenous and endogenous sulfite (Scheme 1b).
Favarin et al.69 obtained three gold-based pyrazoline dual probes 10a–c in 59–64% yields by reacting chloro(triphenylphosphine)gold(I) (Ph3PAuCl), potassium hexafluorophosphate (KPF6), and the pyrazoline-based ligands 9a–c. These ligands exhibited absorption bands around 240 and 325 nm and emission bands around 366 nm, and although they were fluorescent dyes, gold complexes enhanced the emission via charge-transfer (CT) processes governed by metal-to-ligand CT (MLCT). The MLCT favoured the intraligand CT (ILCT) and ligand-to-ligand CT (LLCT), as shown by DFT calculations in the ground state, leading the authors to assume that the emission of complexes 10a–c was due to the mentioned CT processes. However, the photophysical mechanism in the excited state still needs to be studied in more depth as this is less simple. The CT processes led to two λem, a blue one when excited at about 305 nm and a green one when excited at 405 nm. Cellular imaging was performed on two cancer cell lines (MDA-MD231 and MCF-7) and one non-cancerous healthy cell line (HUVEC). For the studied cell lines, 10a–c showed good internalisation and staining of the cytoplasm and they seemed to be suitable dyes for general staining or even for co-staining assays, as their dual emission may allow using further dyes that are compatible with at least one of the fluorescence modes of 10a–c, thereby increasing their span of utility. However, it must be noted that 10a–c were found to be highly cytotoxic for cancer and non-cancer cell lines, which would impair their application for in vivo imaging, as their use would hinder studying cellular processes and lead to the death of the cells (Scheme 1c).
Alizadeh et al.70 used the pyrazolopyridine–coumarin hybrid probes 13a–h for general cell staining, synthesised in 70–85% yields via a 1,3-dipolar cycloaddition reaction of hydrazonoyl chloride 11 and the enone 12. The reaction proceeded in the presence of ammonium acetate by nucleophilic substitution of the chloride on the coumarin moiety with the nitrogen source, forming an enamine that then reacted with the carbonyl to generate products after aromatization. These probes exhibited coplanar, rigid, and aromatic structures, which were responsible for their fluorescence, except for probes bearing a nitro group as this favoured internal conversion over radiative decay; likewise, the emission spectra were barely affected by the substituents. As a representative example, probe 13a exhibited λabs at 330 nm, λem at 465 nm, ΦF of 65%, good photostability, and pH sensitivity; as a result, this probe was applied for the cellular imaging of MG-63 cells. The probe showed good internalisation into the cell, apparently even into the nuclei, as there were no black spots on the images after 30 min of incubation (Scheme 2a).
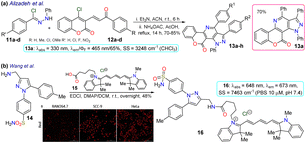 |
| Scheme 2 Synthesis and photophysical data of (a) the fused pyrazoles 13a–h and (b) the π-extended pyrazole 16. Bioimage of 16 in RAW264.7, SCC-9, and HeLa cell lines by ref. 71 with minor modifications licensed under Creative Commons CC BY 4.0. | |
As a final example in 2020, Wang et al.71 obtained the NIR fluorescent probe celecoxib-based 16 in a 48% yield by the amidation reaction of the 3-aminomethylpyrazole 14 with the carboxylic acid 15 bearing a cyanine moiety. This probe was used in cyclooxygenase sensing 2 (COX-2) in tumoural cells. The photophysical properties of probe 16 were recorded in PBS at pH 7.4 to achieve conditions as close as possible to the cellular environment, displaying λabs at 648 nm and λem at 673 nm. In addition, docking studies showed that 16 was a potent inhibitor of COX-2, whose binding site was the same as free celecoxib, i.e. inside the active side pocket. The COX-2 inhibiting activity of 16 was confirmed by in vitro tests using purified COX-2m, and its actual capability for imaging in living cells was investigated by imaging assays in a normal cell line (RAW264.7) and two cancer cell lines (HeLa and SCC-9). It's very low toxicity to the three cell lines with differential and scarce fluorescence for normal cells but strong fluorescence for cancer cells was evidenced; thus, 16 could discern normal and cancerous cells. As a result, the optical mechanism for 16 must be associated with its accumulation around COX-2, which is less expressed in normal cells, explaining why the RAW264.7 cells barely shined while the cancer cells strongly fluoresced (Scheme 2b).
2.2. Probes reported in 2021
He et al.72 synthesised the (pyrazolin-3-yl) phenyl acrylate 19a–b in a 50–60% yield by the esterification between 2-(pyrazolin-3-yl)phenol 17a–b and acryloyl chloride 18. These probes exhibited very weak emission at 490 nm, but this increased upon the nucleophilic addition of glutathione to the acrylate receptor fragments 19a–b. Utilising DFT studies, the authors reported that the highest occupied molecular orbital (HOMO) was focused on the N-phenylpyrazoline moiety and the lowest unoccupied molecular orbital (LUMO) on the acrylate, causing ICT with fluorescence turn-off; thus, the LUMO was disturbed upon the conjugated addition of the respective thiol group, raising the HOMO–LUMO energy gap and thereby preventing quenching. The probes offered low toxicity, and 19a was applied for imaging tests in cells cultured with and without glutathione. Incubation of the cells with 19a led to blue fluorescence inside the cells without any morphological artefacts, indicating that 19a could detect endogenous glutathione, and has potential as a new tool for studying glutathione's biological role in different processes (Scheme 3a).
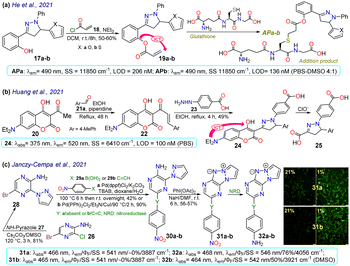 |
| Scheme 3 Synthesis and photophysical data of (a) 19a–b, (b) 24, and (c) 31/32a–b. Bioimaging of A2058 cells cultured under normoxic (21% pO2) and hypoxic (1% pO2) conditions for 24 h with 31a–b (prior 2 h at 8 μM); ref. 73 with minor changes licensed under Creative Commons CC BY 4.0. | |
Huang et al.74 obtained the coumarin-3-ylpyrazoline 24 for in vivo hypochlorite sensing by reacting 3-acetylcoumarin 20 with p-tolualdehyde (21a) to produce the enone 22, which then reacted with arylhydrazine 23 to afford 24 in a 49% yield. Probe 24 showed λabs at 375 nm, λem at 520 nm, and a donor–acceptor structure from an electron-donating group (EDG), such as diethylamino, to an electron-withdrawing group (EWG), such as hydrazone, in the pyrazoline fragment, favouring the emission of 24 by an ICT phenomenon. This probe was degraded to the dicarboxylic acid 25 through hypochlorite-mediated oxidation upon adding the analyte (on the coumarin ring), quenching its fluorescence. The fluorophore 24 showed slight cytotoxicity and good internalisation into the cytoplasm for cell imaging. When the cells or zebrafish were cultured without the analyte, the probe showed strong green fluorescence, which was quenched upon addition of the analyte, implying that 24 could detect exogenous ClO−. HeLa cells were also incubated with LPS to stimulate the endogenous production of ClO−, which was also detected (Scheme 3b).
Janczy-Cempa et al.73 used the nitrocompounds 31a–b as probes sensitive to nitroreductase, allowing in vivo hypoxia evaluation by imaging. The probes were synthesised by reacting the pyrazine 26 with NH-pyrazole (27) to form the N-hetarylpyrazole 28, which then reacted with the appropriate nitroaryl derivative 29a–b to yield the intermediates 30a–b, which were finally subjected to a PIDA-mediated cyclization reaction (Scheme 3c). Probes 31a–b showed λabs around 465 nm, λem around 541 nm, and very weak fluorescence as the nitro group quenched the process. The reduced fluorophores 32a–b showed good photostability and responses against human nitroreductase (NRD), even in the presence of interferents, and their fluorescence was not affected by molecular oxygen. To evaluate the bioimaging suitability of the probes, they were cultured with A2058 cells under normoxic and hypoxic conditions. Cells grown under normoxic conditions showed a slight fluorescence emission, possibly due to the activity of basal levels of NRD. However, when subjected to hypoxic conditions (less than 1% oxygen), the cells showed increased green emission, indicating a more significant reduction of probes 31a–b.
Krishnaveni et al.75 reported bisimine 35 as a probe for the in vivo detection of Zn2+ that was prepared by the condensation reaction of 5-bromosalicylaldehyde (21b) with hydrazine and then with 4-formylpyrazole 34 to produce the probe in a 98% yield. Probe 35 exhibited two λabs at 288 and 366 nm, a weak λem at 511 nm, a HOMO spread over the entire molecule, and a LUMO focused over the imine-bromophenol moiety. No fluorescence was observed for 35 due to the s-cis/s-trans isomerisation of the bisimine group (–CH
N–N
CH–). However, when 35 was complexed with Cd2+ or Zn2+, the HOMO was focused on the metal, while the LUMO remained similar; thus, the fluorescence in 35-M was attributed to a metal-to-ligand charge transfer (MLCT). This probe could detect Zn2+ in HeLa cells and zebrafish embryos, exhibiting little toxicity. Zebrafish did not show fluorescence with 35 and without the analyte, while an intense yellow emission was observed under the exposition and internalisation with Zn2+ (Scheme 4a).
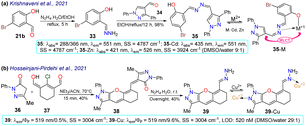 |
| Scheme 4 Synthesis and photophysical data of (a) 35/35-M and (b) 39/39-Cu. | |
In the last example of 2021, Hosseinjani-Pirdehi et al.76 obtained the tetrahydro-chromeno[2,3-c]pyrazole 39 by reacting 2 equivalents of pyrazolone 36 with formylated 1,3-biselectrophyle 37 to produce 38, which ultimately suffered hydrazinolysis at 39 in a 40% yield (Scheme 4b). This probe was used for in vivo Cu2+ sensing and both 39 and 39-Cu showed no displacements of λabs and λem, but the fluorescence was enhanced in 39-Cu. For 39, no fluorescence was observed due to the E/Z isomerisation of the hydrazone moiety, which was restricted once the metal had chelated, causing more rigidity in the molecule. This probe was used for bioimaging and the in vivo detection of Cu2+. Cells incubated solely with 39 showed no emission; however, the cells showed a greenish-yellow emission after Cu2+ was added to the culture. This result indicates that 39 had reasonable internalisation rates and could detect intracellular Cu2+. However, it is essential to note that 39 showed significant toxicity towards cells. Given this, 39 might not be the best tool for ion monitoring in living systems, but it could still be used for in vitro or ex vivo testing.
2.3. Probes reported in 2022
Liang et al.37 obtained the 1,4-disubstituted pyrazoles 44 and 48 for the in vivo detection of c-Met, a protein highly expressed in solid tumours that is a strategic target for cancer diagnosis and detection. The synthesis started from the arylaldehyde 21c or anhydride 45, and it continued by incorporating a bromo-alkyl moiety that was then stirred with the pyrazolyl–boronic acid 41 to form the boronic esters 42 and 47, which were subjected to a Suzuki–Miyaura reaction yielding 44 and 48. Both dyes showed a photoinduced electron transfer (PET) process with fluorescence turn-off, in which the excited coumarin moiety transferred an electron to the receptor quinoline moiety; however, this process was inhibited upon binding to c-Met, turning on the emission of 44 and 48 (Schemes 5a and 5b). As for the bioimaging assays, both probes exhibited slight fluorescence in normal cells, which was related to basal levels of c-Met as this is a protein linked to several metabolic processes for almost any cell. In contrast, when the fluorophore was used with a c-Met overexpressing cell line, there was an apparent increase in the fluorescence, indicating that both probes could be used for detecting changes in the levels of c-Met. However, it must be considered that both dyes were highly toxic to at least two cell lines; thus, their application spectrum might not be as broad as expected.
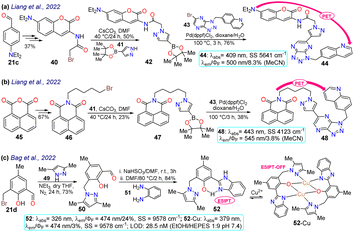 |
| Scheme 5 Synthesis and photophysical data of (a) 44, (b) 48, and (c) 52/52-Cu. | |
Later, Bag et al.77 synthesised the pyrazole derivative 52, an excited-state intramolecular proton-transfer (ESIPT) active sensor for sensing Cu2+ in cells and plants. Their synthesis started from the 3-bromomethylsalicylaldehyde 21d and the NH-pyrazole 49 to form the intermediate 50; which was then reacted with sodium bisulfite and later with o-phenylenediamine 51 to yield the benzimidazole derivative 52.77 Unlike other sensors for metal cations analysed above, 52 was a turn-off sensor whose emission was quenched by adding Cu2+. This dye exhibited a λabs at 326 nm and a λem at 474 nm with a ΦF of 24%, whereas 52-Cu showed a redshifted λabs at 379 nm with a λem that was not shifted but was dramatically decreased (ΦF = 3%) due to an ESIPT process. In the excited state, the OH group of 52 formed intramolecular hydrogen bonds with pyridine-like nitrogen in pyrazole and benzimidazole rings, giving a strong blue emission; still, the fluorescence was quenched upon the formation of 52-Cu as the heteroatoms were occupied. Next, 52 was used for in vivo Cu2+ sensing, with MCF-7 cells, chickpeas, and Mung beans tested. For the cells, 52 showed almost no toxicity; while samples treated with 52 without Cu2+ showed strong blue emission inside the cells and the sprout of the beans, revealing that 52 could be well absorbed in mammal and plant cells. In contrast, when the samples were treated with 52 and Cu2+, the fluorescence was quenched in cells and plants (Scheme 5c).
Roy et al.78 obtained the pyrazolone 54 by reacting 3-methoxy-salicylaldehyde (21e) with the aminopyrazolone 53. This probe showed three λabs at 230, 268, and 326 nm but was not fluorescent due to PET and ESIPT processes. However, complex 54-Al showed a redshift to 404 nm. Upon the excitation of 54, its aryloxyimine group transferred one electron to the adjacent enone moiety, and the molecular conformation allowed the intramolecular hydrogen bond formation. Notably, the PET and ESIPT processes were not viable after forming 54-Al as the heteroatom donors were occupied in chelating the Al3+ (Scheme 6a). Likewise, 54 was used for intracellular Al3+ sensing by bioimaging, with an absence of emission observed for cells treated with Al3+, but once the dye was added, a strong green fluorescence was seen. Nevertheless, care must be taken when using this probe as it was confirmed through utilising an acridine orange/ethidium bromide co-staining assay that the 54-Al complex may interact with single-stranded DNA, which could affect the cell cycle and lead to cell death via apoptosis.
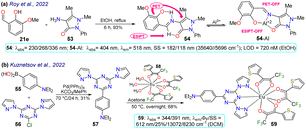 |
| Scheme 6 Synthesis and photophysical data of probes (a) 54/54-Al and (b) 59. | |
Finally, Kuznetsov et al.79 prepared the phosphorescent probe 59 by a Suzuki coupling of the arylboronic acid 55 with the heteroaryl chloride 56, yielding the tridentate ligand 57, which then reacted with the Eu(III) complex 58, delivering 59. This probe showed two λabs at 344 and 391 nm with a λem at 612 nm, typical for Eu(III) complexes (Scheme 6b). As NIR radiation is minor phototoxic and more penetrating than visible radiation, these results suggest the probe would not cause cellular photodamage and would offer deep tissue penetration. By testing the temperature effect on the luminescence of 59, it was found that raising the temperature reduced the lifetime of its emission, indicating its high sensitivity to temperature changes. Nanoparticles 59-NPs with a superficial positive charge were also prepared as their target is the mitochondrion, whose membrane is anionic. Still, 59-NPs were found in endosomes, lysosomes, or similar parts, not in the mitochondria. Moreover, phosphorescence lifetime imaging microscopy (PLIM) assays were used with uncharged 59-NPs for sensing cellular temperature. The images showed a general typical temperature in cells, although some colder spots could also be seen. However, these results were good enough to indicate that the probe is suitable and viable for intracellular temperature review. The same behaviour was found for modified 59-NPs, indicating that the cationic surface did not affect its sensing properties.
2.4. Probes reported in 2023
Krishnan et al.80 synthesised pyrazolo[4,3-b]pyridine 62 in an 85% yield through a Povarouv reaction of 5-aminopyrazole 60 with the arylaldehyde 61 and then used this probe for the detection of BF3 in E. coli and HeLa cells. Probe 62 displayed an absorption band at 336 nm and an emission band at 440 nm, which were then redshifted in the presence of BF3 – at 368 and 473 nm, respectively. Upon gradual BF3 addition, the absorption band of probe 62 decreased while the fluorescence band increased, increasing the quantum yield from 35% to 65% due to ICT from the alkoxy group to the boron atom. Probe 62 was tested in the presence of E. coli cells, with blue fluorescence observed once the bacterial culture was incubated with BF3. Also, 3-(4,5-dimethylthiazol-2-yl)-2,5-diphenyltetrazolium bromide (MTT) assays were performed, offering green fluorescence, which indicates that the intracellular uptake of BF3 resulted in the complexation in the intracellular region (Scheme 7a).
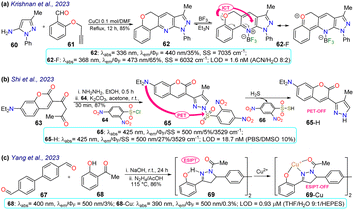 |
| Scheme 7 Synthesis and optical data of probes based on (a) pyrazolopyridine 62/62-F, (b) 3-(coumarin-3-yl)pyrazole 65/65-H, and (c) pyrazoline 69/69-Cu. | |
In another work, Shi et al.81 prepared 3-(coumarin-3-yl)pyrazole 65 by two steps in an 87% yield, implying a cyclocondensation reaction of the β-diketone 63 with hydrazine, followed by a sulfonylation reaction with 2,4-dinitrobenzenesulfonyl chloride (64). This probe exhibited a λabs at 425 nm and a λem at 500 nm without a shift in these wavelengths in the presence of H2S. Nevertheless, upon analyte addition, the solution changed its colour, and the ΦF was enhanced from 5% to 27% as a PET-OFF process was activated once the sulfonyl group was cleaved in 64 by H2S. Competitive assays were performed with varied cations, anions, and some amino acids, and it was found that the reaction with H2S was unperturbed by the presence of other analytes and lasted 3 min. Still, with other analytes like cysteine, the reaction took 30 min or longer. Ultimately, the authors investigated the recognition of exogenous and endogenous H2S in MCF-7 cells, which were incubated with the analyte and a fluorescence turn-on of the cells was observed with an LOD of 18.7 nM (Scheme 7b).
Yang et al.82 synthesised the bispyrazoline 69 in an 86% yield through a condensation reaction of 4,4′-diformylbiphenyl (67) with 2-hydroxyacetophenone 68 followed by a cyclisation reaction with hydrazine. Probe 69 exhibited a λabs at 400 nm, shifted to 390 nm in the presence of Cu2+, and a λem at 500 nm. In the presence of the analyte, the fluorescence decreased, with ΦF going from 3% to 0.3% due to an interrupted ESIPT process. Biological assays were performed on HeLa cells with 69 and 69-Cu, evidencing that these cells had blue emission with good permeability in the presence of 69, and then by adding Cu2+ to the system, the fluorescence signal weakened, making it suitable for the detection of intracellular copper ions (Scheme 7c).
Two examples of pyrazoles were reported in 2023 that do not present an approach to bioimaging but focus on the design of fluorophores, as discussed below. First, Wei et al.83 obtained 72 by condensing 3-pyrazolylhydrazide 70 with salicylaldehyde 71. This probe showed two λabs at 309 and 374 nm, which were shifted to 281 and 406 nm upon the addition of Ga3+ ions. Likewise, weak fluorescence was observed (ΦF = 0.2%), which was increased (ΦF = 57%) in the presence of the analyte, obtaining an LOD of 12.1 mM. The almost no fluorescence of 72 was due to a PET-OFF process in the complex 72-Ga. The authors studied the effect of the diethylamino group in 72, evidencing the necessity of this group for the photophysical properties (Scheme 8a). Second, Patil et al.84 synthesised the imine 74 in an 80% yield using the reaction of 4-aminoantipyrine (72) with 2,5-dihydroxyacetophenone (73). This dye exhibits two λabs at 326 and 364 nm, but in the presence of Cu2+, the band at 364 nm was shifted to 425 nm (Scheme 8b). Upon increasing the concentration of Cu2+, a progressive rise and decrease in the peaks at 425 and 364 nm, respectively, was observed, which evidenced the formation of the complex 74-Cu, which favoured a ligand-to-metal charge transfer (LMCT). An emission peak at 465 nm was evidenced for 74 and 74-Cu, with fluorescence enhancement in the presence of Cu2+ due to a chelation-enhanced fluorescence process (CHEF).
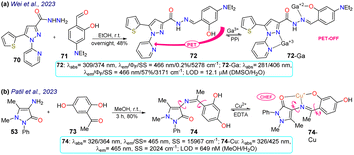 |
| Scheme 8 Synthesis and optical data of (a) 72/72-Ga and (b) 74/74-Cu. | |
2.5. Probes reported in 2024
Rasin et al.85 synthesised the fused pyrazole 78 by the cyclisation reaction of the 1,3-biselectrophyle 76 with the thiosemicarbazide 77. Probe 78 exhibited two absorption bands at 325 nm and 372 nm, and upon the addition of fluoride ions, a new band emerged around 430 nm, indicating the formation of 78-F; 78 displayed a λem at 476 nm that was shifted to 492 nm for 78-F with an increase in the ΦF from 38% to 64%. Complex 78-F demonstrated a fluorescence enhancement compared to 78, which linked the relaxation from the stimulated state to the PET process. The authors investigated the detection of the anion on T24 living cells, and blue fluorescence was observed once the cells were treated with the sensor and fluoride ions, indicating that 78 could detect the anion on the cell's cytoplasm (Scheme 9a).
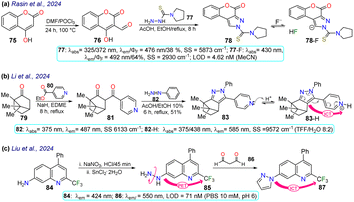 |
| Scheme 9 Synthesis and photophysical data of fluorophores (a) 78/78-F, (b) 83/83-H, and (c) 85/87. | |
Li et al.86 designed probe 83 to detect aqueous acid, which was obtained using the condensation reaction of the bicyclic ketone 79 with ethyl isonicotinate 80 to form the β-diketone 81, which finally cyclocondensed with phenylhydrazine (82). Probe 83 exhibited an absorption band at 375 nm, which was gradually diminished with the increase in the medium's acidity, and a new peak emerged at 438 nm. Likewise, an emission band at 487 nm was observed that decreased as the acidity increased, while a new peak at 585 nm emerged. These spectral changes were caused by protonation of the pyridine ring generating an ICT effect that led to the redshift of the spectrum. The authors conducted fluorescent bioimage studies, which indicated that 83 exhibited low cytotoxicity, making the probe well suited for monitoring pH alterations of zebrafishes as they show a strong fluorescence in acidic media (Scheme 9b).
Liu et al.87 obtained the pyrazole 87 by the cyclocondensation reaction of malonaldehyde (86) with the hetarylhydrazine 85, which was obtained via diazonium salts from the hetarylamine 84 (Scheme 9c). The 4-trifluoromethylquinoline group of 85 was the Golgi-target, and the hydrazine group was the recognition site. This probe exhibited an emission band at 424 nm but showed no fluorescence, due to the PET process generated by the presence of the amino group. However, once the probe reacted with 86, forming 87, the electron-donating capacity of the amino group was affected, offering both PET-OFF and ICT phenomena with a redshift in the emission spectra and an enhanced fluorescence. The MTT method was used with 87, and low cytotoxicity was found. Then, the ability of 85 to Golgi apparatus was proved by labelling HeLa cells and red staining the Golgi apparatus, with blue emission detected, indicating the excellent targeting of the probe with the desired target, with an LOD of 71 nM.
Deng and colleagues prepared the probe 89 using the reaction of tetrazine 88 with phenyl-propanenitrile, a type of [4 + 1] cycloaddition that is an alternative to the inverse electron-demanding Diels–Alder reaction that has gained attention in protein labelling, and drug delivery and thanks to its biocompatibility, rapid kinetics, and effective payload release (Scheme 10a).88 Probe 89 exhibited absorption and emission bands at 394 and 551 nm, respectively, with a ΦF of 42% in PBS buffer, probably giving an ICT-OFF process that initially occurs from the dialkylamino group to the π-deficient tetrazine ring. This probe was studied with live 193T-cell labelling on intracellular targets (nucleus proteins and mitochondria). Vivid mitochondria stains and orthogonal labelling were observed in the membrane, suggesting probe 89 is suitable for labelling bioimages.
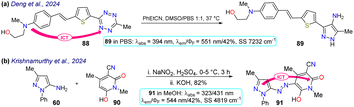 |
| Scheme 10 Synthesis and photophysical data of the probes (a) 89 and (b) 91. | |
Finally, Krishnamurthy et al.89 synthesised probe 91 in an 82% yield starting from the 5-aminopyrazole 60, which underwent diazotization followed by a coupling reaction with the cyanomethyl-pyridone 90. Probe 91 exhibited two absorption peaks at 323 nm and 431 nm and an emission peak at 544 nm with a ΦF of 42% in methanol, which helped stabilise the hydrazo tautomer 91. In solvents such as MeOH, DMSO, and DMF, the hydrazo form was stabilised generating a redshift on the absorption peaks, while in MeCN, DCM, and chloroform the azo form was stabilised generating a blue-shift on the spectra. An ICT process was responsible for the fluorescence of probe 91. This property was used for bioimaging in HeLa cells, with a strong green fluorescence observed, and was studied for the electrochemical detection of dopamine, demonstrating an LOD of 0.81 mM (Scheme 10b).
3 Conclusions
In summary, diverse synthetic transformations implying fluorescent pyrazoles have been reported, some by ring construction and others using functionalisation or derivatisation strategies, usually by classical reactions and reaction conditions that are easily reproducible. In this manner, novel functional pyrazoles with relevant applications have been obtained, with some examples finding use in bioimaging applications. These fluorescent dyes have proven to offer exceptional results in general cell staining and the detection of in-cell conditions, like temperature or hypoxia. The compounds herein discussed include further structural motifs, from additional heterocyclic, pyrazolines, or fluorescent groups to complexes with metal ions, for which different synthetic approaches are needed. For the evaluated systems, ICT, PET, and MLCT are the most prevalent photophysical phenomena that govern the fluorescence of the probes, which, upon reacting with the target (like metal ions, small molecules, or enzymes), is either enhanced or ‘turned-off’, leading to observable changes in the fluorescence that allow the sensing of the different targets, which in turn is what leads to pyrazole derivatives having so many applications. Various molecular architectures of pyrazoles-based compounds have been used in bioimaging applications, in which the incorporation of EDGs or highly conjugated substituents is the main constant in the probe design. Also, the probes must be functionalised with appropriate receptors (e.g. ion receptors, lipophilic fragments, or hydrogen bond mediators) so that they can perform their work in different environments. Notably, although there are many published articles on fluorescent probes for bioimaging applications based on small molecules, several of these works did not carry out an appropriate photophysical study for the respective development; as a result, a good look at this review could pointedly improve this issue.
Data availability
This review manuscript presents original work submitted for publication only in RSC Advances. The authors have no conflicts of interest to report with this submission, and they have all seen, revised, and approved this paper, which details the chemistry and properties of fluorescent probes based on pyrazole/pyrazoline derivatives with a focus on bioimaging applications.
Author contributions
The individuals listed as authors have contributed to developing this manuscript, and no other person was involved. The authors' contributions included M.-C. R. and S. M.-H. ran the article's research and analyses and the original draft composition, and J. P. conducted the composition of the original draft, edition, conceptualization, supervision, and sources. All authors have read and agreed to the published version of this manuscript.
Conflicts of interest
The authors declare no competing financial interest.
Acknowledgements
The authors thank the Chemistry Department and Vicerrectoría de Investigaciones from Universidad de los Andes for financial support. JP acknowledged support from the science faculty (project INV-2023-162-2810). JP also wishes to credit all Bioorganic Compounds Research Group members for their valuable collaboration in syntheses and properties of pyrazole derivatives.
Notes and references
- Z. Guo, S. Park, J. Yoon and I. Shin, Recent progress in the development of near-infrared fluorescent probes for bioimaging applications, Chem. Soc. Rev., 2014, 43, 16–29 RSC.
- M. Won, M. Li, H. S. Kim, P. Liu, S. Koo, S. Son, J. H. Seo and J. S. Kim, Visible to mid IR: A library of multispectral diagnostic imaging, Coord. Chem. Rev., 2021, 426, 213608 CrossRef CAS.
- T. Terai and T. Nagano, Fluorescent probes for bioimaging applications, Curr. Opin. Cell Biol., 2008, 12, 515–521 CrossRef CAS PubMed.
- T. Terai and T. Nagano, Small-molecule fluorophores and fluorescent probes for bioimaging, Pflügers Arch., 2013, 465, 347–359 CrossRef CAS.
- B. A. D. Neto, J. E. P. Sorto, A. A. M. Lapis and F. Machado, Functional chromophores synthesized via multicomponent Reactions: A review on their use as cell-imaging probes, Methods, 2023, 220, 142–157 CrossRef CAS.
- J. Milczarek, R. Pawlowska, R. Zurawinski, B. Lukasik, L. E. Garner and A. Chworos, Fluorescence and confocal imaging of mammalian cells using conjugated oligoelectrolytes with phenylenevinylene core, J. Photochem. Photobiol., B, 2017, 170, 40–48 CrossRef CAS PubMed.
- V. P. De Souza, V. Vendrusculo, A. M. Morás, L. Steffens, F. S. Santos, D. J. Moura, F. S. Rodembusch and D. Russowsky, Synthesis and photophysical study of new fluorescent proton transfer dihydropyrimidinone hybrids as potential candidates for molecular probes, New J. Chem., 2017, 41, 15305–15311 RSC.
- Y. Li, Q. Chen, X. Pan, W. Lu and J. Zhang, Development and Challenge of Fluorescent Probes for Bioimaging Applications: From Visualization to Diagnosis, Top. Curr. Chem., 2022, 380, 22 CrossRef CAS PubMed.
- D. Pirone, J. Lim, F. Merola, L. Miccio, M. Mugnano, V. Bianco, F. Cimmino, F. Visconte, A. Montella, M. Capasso, A. Iolascon, P. Memmolo, D. Psaltis and P. Ferraro, Stain-free identification of cell nuclei using tomographic phase microscopy in flow cytometry, Nat. Photonics, 2022, 16, 851–859 CrossRef CAS PubMed.
- R. R. Mayank, A. Singh, N. Garg, N. Kaur and N. Singh, Mitochondria- and nucleolus-targeted copper(i) complexes with pyrazole-linked triphenylphosphine moieties for live cell imaging, Analyst, 2020, 145, 83–90 RSC.
- M. Gao, H. Su, S. Li, Y. Lin, X. Ling, A. Qin and B. Z. Tang, An easily accessible aggregation-induced emission probe for lipid droplet-specific imaging and movement tracking, Chem. Commun., 2017, 53, 921–924 RSC.
- A. Reicher, J. Reiniš, M. Ciobanu, P. Růžička, M. Malik, M. Siklos, V. Kartysh, T. Tomek, A. Koren, A. F. Rendeiro and S. Kubicek, Pooled multicolour tagging for visualizing subcellular protein dynamics, Nat. Cell Biol., 2024, 26, 745–756 CrossRef PubMed.
- M. O. Rodrigues, M. N. Eberlin and B. A. D. Neto, How and Why to Investigate Multicomponent Reactions Mechanisms? A Critical Review, Chem. Rec., 2021, 21, 2762–2781 CrossRef CAS.
- S. Das, H. K. Indurthi, P. Asati and D. K. Sharma, Small Molecule Fluorescent Probes for Sensing and Bioimaging of Nitroreductase, ChemistrySelect, 2022, 7, e202102895 CrossRef CAS.
- J. T. Hou, K. K. Yu, K. Sunwoo, W. Y. Kim, S. Koo, J. Wang, W. X. Ren, S. Wang, X. Q. Yu and J. S. Kim, Fluorescent Imaging of Reactive Oxygen and Nitrogen Species Associated with Pathophysiological Processes, Chem, 2020, 6, 832–866 CAS.
- L. C. Murfin, M. Weber, S. J. Park, W. T. Kim, C. M. Lopez-Alled, C. L. McMullin, F. Pradaux-Caggiano, C. L. Lyall, G. Kociok-Köhn, J. Wenk, S. D. Bull, J. Yoon, H. M. Kim, T. D. James and S. E. Lewis, Azulene-Derived Fluorescent Probe for Bioimaging: Detection of Reactive Oxygen and Nitrogen Species by Two-Photon Microscopy, J. Am. Chem. Soc., 2019, 141, 19389–19396 CrossRef CAS PubMed.
- A. Tigreros and J. Portilla, Fluorescent Pyrazole Derivatives: An Attractive Scaffold for Biological Imaging Applications, Curr. Chin. Sci., 2021, 1, 197–206 CrossRef CAS.
- H. Chen, F. Ding, Z. Zhou, X. He and J. Shen, FRET-based sensor for visualizing pH variation with colorimetric/ratiometric strategy and application for bioimaging in living cells, bacteria and zebrafish, Analyst, 2020, 145, 4283–4294 RSC.
- S. Gond, P. Yadav, B. S. Chauhan, S. Srikrishna and V. P. Singh, Development of an ‘OFF-ON-OFF’ colorimetric and fluorometric pH sensor for the study of physiological pH and its bioimaging application, J. Mol. Struct., 2022, 1252, 132147 CrossRef CAS.
- X.-L. Xue, Y. Wang, S. Chen, K.-P. Wang, S.-Y. Niu, Q.-S. Zong, Y. Jiang and Z.-Q. Hu, Monitoring intracellular pH using a hemicyanine-based ratiometric fluorescent probe, Spectrochim. Acta, Part A, 2023, 284, 121778 CrossRef CAS.
- N. Bauer, I. Maisuls, A. Pereira da Graça, D. Reinhardt, R. Erapaneedi, N. Kirschnick, M. Schäfers, C. Grashoff, K. Landfester, D. Vestweber, C. A. Strassert and F. Kiefer, Genetically encoded dual fluorophore reporters for graded oxygen-sensing in light microscopy, Biosens. Bioelectron., 2023, 221, 114917 CrossRef CAS PubMed.
- V. Mirabello, F. Cortezon-Tamarit and S. I. Pascu, Oxygen Sensing, Hypoxia Tracing and in Vivo Imaging with Functional Metalloprobes for the Early Detection of Non-communicable Diseases, Front. Chem., 2018, 6, 00027 CrossRef.
- D. Chen, W. Wang, Q. Zhu, Q. Wang, D. Quan, Y. Zeng, K. Li, Y. Zhou, C. Liu, W. Zhan and Y. Zhan, In vivo real-time monitoring of the development of hypoxia and angiogenesis in cervical cancer, J. Chem. Eng, 2023, 473, 145498 CrossRef CAS.
- P. Feng, H. Zhang, Q. Deng, W. Liu, L. Yang, G. Li, G. Chen, L. Du, B. Ke and M. Li, Real-Time Bioluminescence Imaging of Nitroreductase in Mouse Model, Anal. Chem., 2016, 88, 5610–5614 CrossRef CAS.
- Q. Yang, S. Wang, D. Li, J. Yuan, J. Xu and S. Shao, A mitochondria-targeting nitroreductase fluorescent probe with large Stokes shift and long-wavelength emission for imaging hypoxic status in tumor cells, Anal. Chim. Acta, 2020, 1103, 202–211 CrossRef CAS.
- W. Wanas, S. A. Abd El-Kaream, S. Ebrahim, M. Soliman and M. Karim, Cancer bioimaging using dual mode luminescence of graphene/FA-ZnO nanocomposite based on novel green technique, Sci. Rep., 2023, 13, 27 Search PubMed.
- B. Brennecke, Q. Wang, Q. Zhang, H. Y. Hu and M. Nazaré, An Activatable Lanthanide Luminescent Probe for Time-Gated Detection of Nitroreductase in Live Bacteria, Angew. Chem., Int. Ed., 2020, 59, 8512–8516 CrossRef CAS PubMed.
- Q. Yang, Y. Wen, A. Zhong, J. Xu and S. Shao, An HBT-based fluorescent probe for nitroreductase determination and its application in: Escherichia coli cell imaging, New J. Chem., 2020, 44, 16265–16268 RSC.
- H. Jiang, Z. Cao, Y. Liu, R. Liu, Y. Zhou and J. Liu, Bacteria-Based Living Probes: Preparation and the Applications in Bioimaging and Diagnosis, Adv. Sci., 2024, 11, 2306480 CrossRef CAS.
- Y. Fan, M. Lu, X. A. Yu, M. He, Y. Zhang, X. N. Ma, J. Kou, B. Y. Yu and J. Tian, Targeted Myocardial Hypoxia Imaging Using a Nitroreductase-Activatable Near-Infrared Fluorescent Nanoprobe, Anal. Chem., 2019, 91, 6585–6592 CrossRef CAS PubMed.
- J. Zheng, Y. Shen, Z. Xu, Z. Yuan, Y. He, C. Wei, M. Er, J. Yin and H. Chen, Near-infrared off-on fluorescence probe activated by NTR for in vivo hypoxia imaging, Biosens. Bioelectron., 2018, 119, 141–148 CrossRef CAS PubMed.
- X. Han, R. Wang, X. Song, F. Yu, C. Lv and L. Chen, A mitochondrial-targeting near-infrared fluorescent probe for bioimaging and evaluating endogenous superoxide anion changes during ischemia/reperfusion injury, Biomaterials, 2018, 156, 134–146 CrossRef CAS PubMed.
- J. Qian and B. Z. Tang, AIE Luminogens for Bioimaging and Theranostics: From Organelles to Animals, Chem, 2017, 3, 56–91 CAS.
- C. Pigot, D. Brunel and F. Dumur, Indane-1,3-Dione: From Synthetic Strategies to Applications, Molecules, 2022, 27, 5976 CrossRef CAS PubMed.
- S. M. Bentzen, Theragnostic imaging for radiation oncology: dose-painting by numbers, Lancet Oncol., 2005, 6, 112–117 CrossRef PubMed.
- M. J. Mantilla, C. E. Cabrera Díaz, G. Ariza-Aranguren, H. de Cock, J. B. Helms, S. Restrepo, E. Jiménez and A. M. Celis Ramírez, Back to the Basics: Two Approaches for the Identification and Extraction of Lipid Droplets from Malassezia pachydermatis CBS1879 and Malassezia globosa CBS7966, Curr. Protoc., 2021, 1, e122 CrossRef CAS.
- D. Liang, C. Yu, X. Qin, X. Yang, X. Dong, M. Hu, L. Du and M. Li, Discovery of small-molecule fluorescent probes for C-Met, Eur. J. Med. Chem., 2022, 230, 114114 CrossRef CAS PubMed.
- A. Tigreros, M. Macías and J. Portilla, Structural, Photophysical, and Water Sensing Properties of Pyrazolo[1,5-a]pyrimidine-Triphenylamine Hybrid Systems, ChemPhotoChem, 2022, 2022, e202200133 CrossRef.
- D. Cao, Z. Liu, P. Verwilst, S. Koo, P. Jangjili, J. S. Kim and W. Lin, Coumarin-Based Small-Molecule Fluorescent Chemosensors, Chem. Rev., 2019, 119, 10403–10519 CrossRef CAS PubMed.
- J. Xie, L. Wang, X. Su and J. Rodrigues, Coumarin-based Fluorescent Probes for Bioimaging: Recent Applications and Developments, Curr. Org. Chem., 2021, 25, 2142–2154 CrossRef CAS.
- R. J. Grams, W. L. Santos, I. R. Scorei, A. Abad-García, C. A. Rosenblum, A. Bita, H. Cerecetto, C. Viñas and M. A. Soriano-Ursúa, The Rise of Boron-Containing Compounds: Advancements in Synthesis, Medicinal Chemistry, and Emerging Pharmacology, Chem. Rev., 2024, 124, 2441–2511 CrossRef CAS.
- S. Das, S. Dey, S. Patra, A. Bera, T. Ghosh, B. Prasad, K. D. Sayala, K. Maji, A. Bedi and S. Debnath, BODIPY-Based Molecules for Biomedical Applications, Biomolecules, 2023, 13, 1723 CrossRef CAS.
- M. C. Rios, N. F. Bravo, C. C. Sanchez and J. Portilla, Chemosensors based on N-heterocyclic dyes: Advances in sensing highly toxic ions such as CN- and Hg2+, RSC Adv., 2021, 11, 34206–34234 RSC.
- J. T. Sarmiento and J. Portilla, Current Advances in Diazoles-based Chemosensors for CN- and FDetection, Curr. Org. Synth., 2023, 20, 77–95 CrossRef CAS.
- J. Zhang, X. Chai, X.-P. He, H.-J. Kim, J. Yoon and H. Tian, Fluorogenic probes for disease-relevant enzymes, Chem. Soc. Rev., 2019, 48, 683–722 RSC.
- Y. Yang, Q. Zhao, W. Feng and F. Li, Luminescent Chemodosimeters for Bioimaging, Chem. Rev., 2013, 113, 192–270 CrossRef CAS PubMed.
- C. L. Fleming, P. A. Sandoz, T. Inghardt, B. Önfelt, M. Grøtli and J. Andréasson, A Fluorescent Kinase Inhibitor that Exhibits Diagnostic Changes in Emission upon Binding, Angew. Chem., Int. Ed., 2019, 58, 15000–15004 CrossRef CAS.
- L. Türker and S. Variş, A review of polycyclic aromatic energetic materials, Polycyclic Aromat. Compd., 2009, 29, 228–266 CrossRef.
- M. Stępień, E. Gońka, M. Żyła and N. Sprutta, Heterocyclic Nanographenes and Other Polycyclic Heteroaromatic Compounds: Synthetic Routes, Properties, and Applications, Chem. Rev., 2017, 117, 3479–3716 CrossRef.
- H. M. Kim and B. R. Cho, Small-Molecule Two-Photon Probes for Bioimaging Applications, Chem. Rev., 2015, 115, 5014–5055 CrossRef CAS.
- A. Loudet and K. Burgess, BODIPY Dyes and Their Derivatives: Syntheses and Spectroscopic Properties, Chem. Rev., 2007, 107, 4891–4932 CrossRef CAS PubMed.
- M. O. Senge, N. N. Sergeeva and K. J. Hale, Classic highlights in porphyrin and porphyrinoid total synthesis and biosynthesis, Chem. Soc. Rev., 2021, 50, 4730–4789 RSC.
- J. Hwang, T. S. Reddy, H. Moon, H. D. Lee and M.-S. Choi, Cyanide detecting porphyrin fluorescent sensors: Effects of electron-donating/withdrawing substituents, Dyes Pigm., 2023, 215, 111243 CrossRef CAS.
- A. L. Pang, A. Arsad and M. Ahmadipour, Synthesis and factor affecting on the conductivity of polypyrrole: a short review, Polym. Adv. Technol., 2021, 32, 1428–1454 CrossRef CAS.
- F.-Z. Li, J.-F. Yin and G.-C. Kuang, BODIPY-based supramolecules: Construction, properties and functions, Coord. Chem. Rev., 2021, 448, 214157 CrossRef CAS.
- D. Kim, D. Ma, M. Kim, Y. Jung, N. H. Kim, C. Lee, S. W. Cho, S. Park, Y. Huh, J. Jung and K. H. Ahn, Fluorescent Labeling of Protein Using Blue-Emitting 8-Amino-BODIPY Derivatives, J. Fluoresc., 2017, 27, 2231–2238 CrossRef CAS.
- M. L. S. O. Lima, C. B. Braga, T. B. Becher, M. Odriozola-Gimeno, M. Torrent-Sucarrat, I. Rivilla, F. P. Cossío, A. J. Marsaioli and C. Ornelas, Fluorescent Imidazo[1,2-a]pyrimidine Compounds as Biocompatible Organic Photosensitizers that Generate Singlet Oxygen: A Potential Tool for Phototheranostics, Chem.–Eur. J., 2021, 27, 6213–6222 CrossRef CAS PubMed.
- A. Kolbus, T. Uchacz, A. Danel, K. Gałczyńska, P. Moskwa and P. Kolek, Fluorescent Sensor Based on 1H-Pyrazolo[3,4-b]quinoline Derivative for Detecting Zn2+ Cations, Molecules, 2024, 29, 823 CrossRef CAS PubMed.
- J. Orrego-Hernández, C. Lizarazo, J. Cobo and J. Portilla, Pyrazolo-fused 4-azafluorenones as key reagents for the synthesis of fluorescent dicyanovinylidene-substituted derivatives, RSC Adv., 2019, 9, 27318–27323 RSC.
- K. Saravana Mani, R. Rajamanikandan, G. Ravikumar, B. Vijaya Pandiyan, P. Kolandaivel, M. Ilanchelian and S. P. Rajendran, Highly Sensitive Coumarin–Pyrazolone Probe for the Detection of Cr 3+ and the Application in Living Cells, ACS Omega, 2018, 3, 17212–17219 CrossRef CAS.
- S. B. Subramaniyan, S. B. Annes, M. Yuvasri, K. Nivedha, S. Ramesh and V. Anbazhagan, 1,3,5-Triphenylpyrazoline Based Fluorescent Probe for Selective Sensing and Imaging of Glutathione in Live Cell under Oxidative Stress, ChemistrySelect, 2020, 5, 515–521 CrossRef CAS.
- A. Tigreros and J. Portilla, Recent progress in chemosensors based on pyrazole derivatives, RSC Adv., 2020, 10, 19693–19712 RSC.
- M.-C. Ríos and J. Portilla, Recent Advances in Synthesis and Properties of Pyrazoles, Chemistry, 2022, 4, 940–968 CrossRef.
- J. Portilla, Current Advances in Synthesis of Pyrazole Derivatives: An Approach Toward Energetic Materials, J. Heterocycl. Chem., 2024, 61(12), 2026–2039 CrossRef CAS.
- J. Orrego-Hernández, J. Cobo and J. Portilla, Synthesis, Photophysical Properties, and Metal-Ion Recognition Studies of Fluoroionophores Based on 1-(2-Pyridyl)-4-Styrylpyrazoles, ACS Omega, 2019, 4, 16689–16700 CrossRef.
- M. U. Sarveswari S., A review on pyrazole moieties as organic chemosensors in the detection of cations and anions, Inorg. Chim. Acta, 2024, 569, 122118 CrossRef.
- Y. Zhang, X. Zheng, L. Zhang, Z. Yang, L. Chen, L. Wang, S. Liu and Z. Xie, Red fluorescent pyrazoline-BODIPY nanoparticles for ultrafast and long-term bioimaging, Org. Biomol. Chem., 2020, 18, 707–714 RSC.
- X. B. Wang, H. J. Li, Z. Chi, X. Zeng, L. J. Wang, Y. F. Cheng and Y. C. Wu, A novel mitochondrial targeting fluorescent probe for ratiometric imaging SO2 derivatives in living cells, J. Photochem. Photobiol., A, 2020, 390, 112339 CrossRef CAS.
- L. R. V. Favarin, G. B. Laranjeira, C. F. A. Teixeira, H. Silva, A. C. Micheletti, L. Pizzuti, A. Machulek Júnior, A. R. L. Caires, V. M. Deflon, R. B. P. Pesci, C. N. L. Rocha, J. R. Correa, L. M. C. Pinto and G. A. Casagrande, Harvesting greenish blue luminescence in gold(i) complexes and their application as promising bioactive molecules and cellular bioimaging agents, New J. Chem., 2020, 44, 6862–6871 RSC.
- A. Alizadeh, B. Farajpour, S. S. Mohammadi, M. Sedghi, H. Naderi-Manesh, C. Janiak and T. Knedel, Design and Synthesis of Coumarin-Based Pyrazolopyridines as Biocompatible Fluorescence Dyes for Live-Cell Imaging, ChemistrySelect, 2020, 5, 9362–9369 CrossRef CAS.
- X. Wang, L. Wang, L. Xie, Z. Xie, L. Li, D. Bui, T. Yin, S. Gao and M. Hu, Design and Synthesis of a Novel NIR Celecoxib-Based Fluorescent Probe for Cyclooxygenase-2 Targeted Bioimaging in Tumor Cells, Molecules, 2020, 25, 4037 CrossRef CAS.
- X. He, X. Cao, X. Tian and Y. Bai, A simple fluorescent probe for glutathione detection and its bioimaging application in living cells, Microchem. J., 2021, 166, 106135 CrossRef CAS.
- E. Janczy-Cempa, O. Mazuryk, D. Sirbu, N. Chopin, M. Żarnik, M. Zastawna, C. Colas, M. A. Hiebel, F. Suzenet and M. Brindell, Nitro-Pyrazinotriazapentalene scaffolds– nitroreductase quantification and in vitro fluorescence imaging of hypoxia, Sens. Actuators, B, 2021, 346, 130504 CrossRef CAS.
- Y. Huang, Y. Zhang, F. Huo and C. Yin, An innovative hypochlorite-sensing scaffold and its imaging application in vivo, Dyes Pigm., 2021, 191, 109378 CrossRef CAS.
- K. Krishnaveni, S. Murugesan and A. Siva, Fluorimetric and colorimetric detection of multianalytes Zn2+/Cd2+/F− ions via 5-bromosalicyl hydrazone appended pyrazole receptor; live cell imaging analysis in HeLa cells and zebra fish embryos, Inorg. Chem. Commun., 2021, 132, 108843 CrossRef CAS.
- H. Hosseinjani-Pirdehi, N. O. A. Mahmoodi and A. Taheri, Selective Cu2+ detection by a novel fluorescence hydrazone – Base probe in aqueous media, J. Photochem. Photobiol., A, 2021, 421, 113524 CrossRef CAS.
- R. Bag, Y. Sikdar, S. Sahu, C. Das Mukhopadhyay, M. G. B. Drew and S. Goswami, Benzimidazole based ESIPT active chemosensors enable nano–molar detection of Cu2+ in 90% aqueous solution, MCF–7 cells, and plants, J. Photochem. Photobiol., A, 2022, 431, 114006 CrossRef CAS.
- S. Roy, S. Kundu, S. Saha, K. Muddukrishnaiah, R. Pramanik and B. Biswas, Visible light-triggered pyrazole-functionalized reversible ionophore for selective monitoring of aluminium (III) ion, Appl. Organomet. Chem., 2022, 36, e6865 CrossRef CAS.
- K. M. Kuznetsov, V. A. Baigildin, A. I. Solomatina, E. E. Galenko, A. F. Khlebnikov, V. V. Sokolov, S. P. Tunik and J. R. Shakirova, Polymeric Nanoparticles with Embedded Eu(III) Complexes as Molecular Probes for Temperature Sensing, Molecules, 2022, 27, 8813 CrossRef CAS.
- U. Krishnan, S. Manickam and S. K. Iyer, BF3 detection by pyrazolo-pyridine based fluorescent probe and applications in bioimaging and paper strip analysis, J. Mol. Liq., 2023, 385, 122413 CrossRef CAS.
- G. J. Shi, Y. D. Wang, Z. X. Yu, Q. Zhang, S. Chen, L. Z. Xu, K. P. Wang and Z. Q. Hu, The coumarin-pyrazole dye for detection of hydrogen sulfide in cells, Spectrochim. Acta, Part A, 2023, 285, 121898 CrossRef CAS PubMed.
- Y. S. Yang, F. N. Wang, Y. P. Zhang, F. Yang and J. J. Xue, Novel Bis-pyrazoline Fluorescent Probe for Cu2+ and Fe3+ Detection and Application in Cell Imaging, J. Fluoresc., 2024, 34, 159–167 CrossRef CAS PubMed.
- K. Wei, B. Zhang, Y. Liu, M. Kang, P. Liu, X. Yang, M. Pei and G. Zhang, Comparison of two pyrazole derived “turn on” fluorescent probes for the recognition of Ga3+, J. Photochem. Photobiol., A, 2023, 440, 114656 CrossRef CAS.
- N. Patil, R. Dhake, R. Phalak, U. Fegade, C. Ramalingan, V. Saravanan, Inamuddin and T. Altalhi, A Colorimetric Distinct Color Change Cu(II) 4-{[1-(2,5-dihydroxyphenyl)ethylidene]amino}-1,5-dimethyl-2-phenyl-1,2-dihydro-3H-pyrazol-3-one Chemosensor and its Application as a Paper Test Kit, J. Fluoresc., 2023, 33, 1089–1099 CrossRef CAS PubMed.
- P. Rasin, S. M. Basheer, J. Haribabu, K. N. Aneesrahman, V. Manakkadan, V. N. Vadakkedathu Palakkeezhillam, N. Bhuvanesh, C. Echeverria, J. F. Santibanez and A. Sreekanth, Host–guest interactions of coumarin-based 1,2-pyrazole using analytical and computational methods: Paper strip-based detection, live cell imaging, logic gates and keypad lock applications, Heliyon, 2024, 10, e24077 CrossRef.
- X. Li, Z. Meng, S. Gong, Y. Liang, Y. Zhang, Y. Yang, X. Xu, Z. Wang and S. Wang, Development of a novel ratiometric fluorescent probe for real-time monitoring of acidic pH and its applications in food samples and biosystem, Microchem. J., 2024, 204, 111169 CrossRef CAS.
- X. Liu, K. Wang, L. Wei, Y. Wang, C. Liu, X. Rong, T. Yan, W. Shu and B. Zhu, A highly sensitive Golgi-targeted fluorescent probe for the simultaneous detection of malondialdehyde and formaldehyde in living systems and foods, Talanta, 2024, 278, 126427 CrossRef CAS.
- Y. Deng, T. Shen, X. Yu, J. Li, P. Zou, Q. Gong, Y. Zheng, H. Sun, X. Liu and H. Wu, Tetrazine-Isonitrile Bioorthogonal Fluorogenic Reactions Enable Multiplex Labeling and Wash-Free Bioimaging of Live Cells, Angew. Chem., Int. Ed., 2024, 63, e2023198 Search PubMed.
- C. Krishnamurthy, K. Jathi, K. M. Pallavi and C. Yesudhasan, Hydrazo Pyrazole-Pyridone Fluorescent tag for NLO, Live cell imaging, LFPs visualization, Photophysical probing, and Electrochemical sensor for Dopamine detection, Luminescence, 2024, 39, e4760 CrossRef CAS PubMed.
|
This journal is © The Royal Society of Chemistry 2024 |
Click here to see how this site uses Cookies. View our privacy policy here.