DOI:
10.1039/C9CC02210D
(Communication)
Chem. Commun., 2019,
55, 5938-5941
Pd-catalyzed synthesis of α,β-unsaturated ketones by carbonylation of vinyl triflates and nonaflates†
Received
20th March 2019
, Accepted 24th April 2019
First published on 24th April 2019
Abstract
A general and highly chemoselective Pd-catalyzed protocol for the synthesis of α,β-unsaturated ketones by carbonylation of vinyl triflates and nonaflates is presented. Applying the specific monophosphine ligand cataCXium® A, the synthesis of various vinyl ketones as well as carbonylated natural product derivatives proceeds in good yields.
α,β-Unsaturated ketones represent a class of highly valuable intermediates in organic synthesis, which continue to attract the interest of academic and industrial researchers for various applications.1 As illustrated in Scheme 1, a wide range of diverse compounds including pharmaceuticals,2 polymers,3 flavors,4 and biologically5 or optically6 important molecules can be conveniently synthesized from such starting materials.
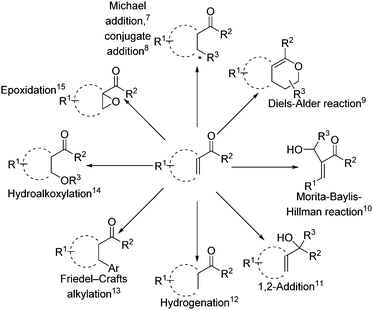 |
| Scheme 1 Selected transformation of α,β-unsaturated ketones. | |
Traditionally, α,β-unsaturated ketones are synthesized via multistep reactions, e.g. sulfide or sulfoxide dehydrogenation,16 enamine transformations,17 Aldol18 or Knoevenagel condensation,19 as well as the so-called Saegusa oxidation.20 More recently, protocols based on the dehydrogenation of ketones,21 alcohols22 and alkenes,23 or the carbonylation of alkynes24 and vinyl iodides,25etc.26 became popular, too. Despite all these achievements, the search for alternative procedures for this class of building blocks remains a challenging but rewarding task.
Based on our long standing interest in reductive carbonylations and alkoxycarbonylations,27 we envisioned the synthesis of α,β-unsaturated ketones via carbonylative coupling reactions of phenylboronic acids and CO with vinyl triflates or nonaflates easily derived from ketones. Herein, we report a general and selective palladium catalyst system which allows for such transformations.
In our initial experiments, we investigated the carbonylation of cyclohexenyl triflate (1) in the presence of phenylboronic acid using previously optimized carbonylative coupling reaction conditions (1 mol% Pd(OAc)2, 1.5 mol% ligand, 0.75 eq. TMEDA in 2 mL toluene with 5 bar CO).28 As shown in Scheme 2, standard mono- and bidentate phosphines, focusing especially on bulky ligands, were tested for this benchmark reaction. Ligands L1 and L2 with integrated basic sites (pyridine), which recently have been proven to be highly efficient in various carbonylation reactions,29 as well as commercially available ligands L3–L6 were not suitable for this transformation giving in general low or no yield of the desired product 3. Here, in most cases decomposition of the substrate was observed. In contrast, when applying cataCXium® A (BuPAd2, L7), the reaction proceeded extremely well to give the desired product in quantitative yield (99%). Based on this result of cataCXium® A, we also tested other sterically hindered adamantyl-substituted ligands (L8–L11), however, 3 was obtained in only 48% or lower yield.30
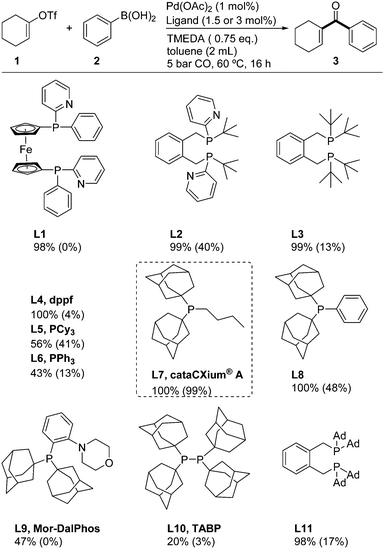 |
| Scheme 2 Pd-catalyzed synthesis of 1-cyclohexenyl phenyl ketone in the presence of different ligands. Reaction conditions: 0.5 mmol 1, 1.2 eq. of 2, 1.5 mol% for diphosphines, 3 mol% for monophophines, in argon. Yields and conversions were determined by GC with n-hexadecane as standard, the values given within parentheses refer to the yields of 3 while the other values refer to the conversions of 1. | |
Next, critical reaction parameters including [Pd] and ligand concentration, temperature, and solvent were investigated. As shown in Table 1, control experiments without Pd(OAc)2 and/or ligand revealed no formation of the desired product (Table 1, entries 2–4). Notably, the catalyst loading can be decreased to only 0.1 mol% resulting in a slight decrease of the yield from 99% to 82% (Table 1, entries 5–7), which indicated the efficiency of this catalytic system. Best results for the benchmark reaction at low catalyst loading were obtained at 60 °C and experiments at R.T. or 40 °C showed a significant decrease of the yield of 3 to 12 and 46%, respectively (Table 1, entries 9 and 10).
Table 1 Pd-catalyzed synthesis of 1-cyclohexenyl phenyl ketone under various conditions

|
Entry |
Pd(OAc)2/mol% |
cataCXium® A/mol% |
Solvent |
T/°C |
Conv. (yield)/% |
Reaction conditions: 0.5 mmol 1, argon atmosphere. Yields and conversions were determined by GC with n-hexadecane as standard. |
1 |
1 |
3 |
Toluene |
60 |
100 (99) |
2 |
1 |
0 |
Toluene |
60 |
20 (0) |
3 |
0 |
3 |
Toluene |
60 |
0 (0) |
4 |
0 |
0 |
Toluene |
60 |
11 (0) |
5 |
0.5 |
1.5 |
Toluene |
60 |
100 (99) |
6 |
0.1 |
0.3 |
Toluene |
60 |
98 (82) |
7 |
0.05 |
0.15 |
Toluene |
60 |
72 (40) |
8 |
0.1 |
0.3 |
THF |
60 |
96 (56) |
9 |
0.5 |
1.5 |
Toluene |
40 |
75 (46) |
10 |
0.5 |
1.5 |
Toluene |
25 |
29 (12) |
With the optimized reaction conditions in hand, carbonylations of 1 with CO and structurally diverse boronic acids were performed (Scheme 3). Besides phenylboronic acid, eleven other substrates were converted to the corresponding products 5a–5f in moderate to excellent yields (52–91%). Here, various arylboronic acids substituted by –F, –COOMe, –NO2, –COMe, –CH
CH2, and –CF3 in either ortho-, meta-, or para-position gave the desired ketones. In addition, heterocyclic substrates including furan, pyridine, and thiophene derivatives are converted smoothly to the corresponding vinyl heteroaryl ketones in 71–89% yields (5g–5i). Notably, vinyl boronic acid was tested too, yielding 98% of the desired divinyl ketone 5j, which provides possibilities for interesting cyclization reactions.31
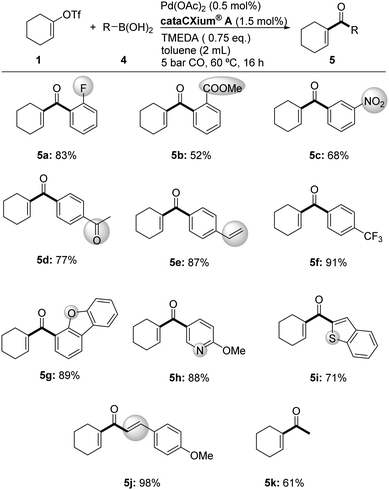 |
| Scheme 3 Pd-catalyzed synthesis of 1-cyclohexenyl aryl ketones. Reaction conditions: 0.5 mmol 1, 1.2 eq. of 4, in argon. Isolated yields. | |
Apart from aromatic and vinyl boronic acids, also methyl boronic acid can be employed in this transformation to access methyl vinyl ketones, e.g.5k, which constitute key synthetic intermediates.32
Next, we studied the carbonylation of structurally diverse triflates with CO and phenylboronic acid. As shown in Scheme 4, vinyl triflates with seven- and eight-membered rings were carbonylated successfully under optimized conditions to give 7a and 7b. Similarly, 7c was isolated in 75%. Obviously, this procedure is not limited to cyclic substrates. As an example, the linear vinyl ketone 7d was obtained in 72% yield. Furthermore, functionalization of naturally occurring terpenes can be easily achieved. Hence, derivatives of camphor, ketoisophorone, verbenone, and pulegone are smoothly converted to the corresponding vinyl ketones under standard conditions in good isolated yields (7e–7h).
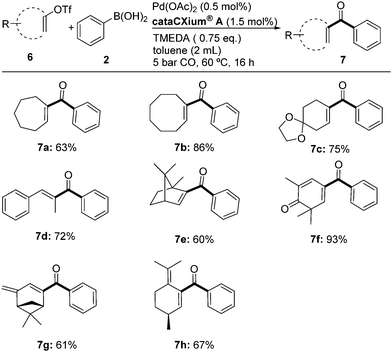 |
| Scheme 4 Pd-catalyzed synthesis of vinyl phenyl ketones. Reaction conditions: 0.5 mmol 6, 1.2 eq. of 2, in argon. Isolated yields. | |
From a synthetic point of view, the use of more stable triflate analogues is interesting due to the easier handling and the avoidance of unwanted side reactions. In this respect, the use of vinyl nonafluorobutanesulfonates (vinyl nonaflates) for the carbonylative synthesis of α,β-unsaturated ketones is appealing. As exemplified in Scheme 5, several nonaflates underwent smooth transformations in up to 89% yield of the desired products 3, 7e and 7f.
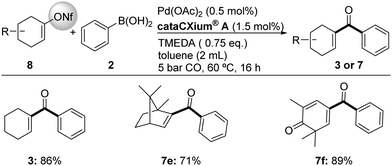 |
| Scheme 5 Pd-catalyzed synthesis of vinyl phenyl ketones from vinyl nonaflates. Reaction conditions: 0.5 mmol 8, 1.2 eq. of 2, in argon. GC yields with n-hexadecane as internal standard. | |
Furthermore, our catalytic system was tested on 1 g scale. As showed in Scheme 6, cyclohexenyl triflate 1 was successfully converted to the corresponding carbonylated product 3 in >99% yield.
 |
| Scheme 6 Gram scale synthesis of 1-cyclohexenyl phenyl ketone. Yield was determined by GC with n-hexadecane as standard. | |
In conclusion, we present a convenient and general procedure for the synthesis of α,β-unsaturated ketones under mild conditions. Using the palladium acetate in combination with the monophosphine ligand cataCXium® A a variety of such products including aliphatic, (hetero)aromatic and divinyl ketones can be efficiently accessed. For the first time, in this procedure we adapted vinyl triflates for the synthesis of α,β-unsaturated ketones with CO as carbonylation partner. The synthetic utility of the protocol is demonstrated in the carbonylation of vinyl triflates including derivatives of camphor, ketoisophorone, verbenone, and pulegone. Furthermore this catalyst system is applicable for the carbonylation of more stable nonaflates.
We are grateful for the financial support from the BMBF and the State of Mecklenburg-Western Pommerania. We thank the analytical department of Leibniz-Institute for Catalysis at the University of Rostock for their excellent service, and Mrs Sandra Leiminger for her kind help in the preparation of experimental materials.
Conflicts of interest
There are no conflicts to declare.
Notes and references
- For some reports in recent years on the chemistry of α,β-unsaturated ketones, see:
(a) T. Fukuda, H. Hashimoto and H. Tobita, J. Am. Chem. Soc., 2014, 136, 80–83 CrossRef CAS PubMed;
(b) Y. Hayashi and N. Umekubo, Angew. Chem., Int. Ed., 2018, 57, 1958–1962 CrossRef CAS PubMed;
(c) A. Lator, S. Gaillard, A. Poater and J. L. Renaud, Chem. – Eur. J., 2018, 24, 5770–5774 CrossRef CAS PubMed;
(d) R. Lonsdale and M. T. Reetz, J. Am. Chem. Soc., 2015, 137, 14733–14742 CrossRef CAS PubMed;
(e) V. Modrocká, E. Veverková, M. Mečiarová and R. Šebesta, J. Org. Chem., 2018, 83, 13111–13120 CrossRef PubMed;
(f) B. W. Turnbull, J. Chae, S. Oliver and P. A. Evans, Chem. Sci., 2017, 8, 4001–4005 RSC;
(g) J. Wen, H. Cheng, G. Raabe and C. Bolm, Org. Lett., 2017, 19, 6020–6023 CrossRef CAS PubMed;
(h) Y. Zhu, H. Qian, B. A. Drake and R. Jin, Angew. Chem., Int. Ed., 2010, 49, 1295–1298 CrossRef CAS PubMed.
-
(a) J. W. Choi, B. K. Jang, N. C. Cho, J. H. Park, S. K. Yeon, E. J. Ju, Y. S. Lee, G. Han, A. N. Pae, D. J. Kim and K. D. Park, Bioorg. Med. Chem., 2015, 23, 6486–6496 CrossRef CAS PubMed;
(b) R. S. Gouhar, E. F. Ewies, M. F. El-Shehry, M. N. F. Shaheen and E.-M. M. E. Ibrahim, J. Heterocycl. Chem., 2018, 55, 2368–2380 CrossRef CAS;
(c) X. Ma, L. Liu, J. Wang, X. Xi, X. Xie and H. Wang, J. Org. Chem., 2018, 83, 14518–14526 CrossRef CAS PubMed.
- O. Green, N. A. Smith, A. B. Ellis and J. N. Burstyn, J. Am. Chem. Soc., 2004, 126, 5952–5953 CrossRef CAS.
- A. Bianco, C. Cavarischia and M. Guiso, Eur. J. Org. Chem., 2004, 2894–2898 CrossRef CAS.
-
(a) W. Choi, I. Jung, S. Kwon, C. Ha and W. Cho, Polym. Degrad. Stab., 1998, 61, 15–20 CrossRef CAS;
(b) J. Park, J. G. Park, W. M. Choi, C. S. Ha and W. J. Cho, J. Appl. Polym. Sci., 1999, 74, 1432–1439 CrossRef CAS;
(c) C. Franceschini, C. Trapella, F. Sforza, R. Gavioli and M. Marastoni, J. Enzym. Inhib. Med. Chem., 2013, 28, 560–564 CrossRef CAS PubMed.
-
(a) R. Nazir, T. T. Meiling, P. J. Cywiński and D. T. Gryko, Asian J. Org. Chem., 2015, 4, 929–935 CrossRef CAS;
(b) S. Wu, N. Yang, Y. Liu, J. Cao, H. Hu, Y. Sun and J. Liu, J. Polym. Sci., Part A: Polym. Chem., 2011, 49, 293–299 CrossRef CAS.
-
(a) J.-S. Li, H.-F. Cui, K.-F. Zhang, J. Nie and J.-A. Ma, Eur. J. Org. Chem., 2017, 2545–2552 CrossRef CAS;
(b) H. Pellissier, Adv. Synth. Catal., 2015, 357, 2745–2780 CrossRef CAS.
- M. S. Taylor, D. N. Zalatan, A. M. Lerchner and E. N. Jacobsen, J. Am. Chem. Soc., 2005, 127, 1313–1317 CrossRef CAS PubMed.
-
(a) V. Eschenbrenner-Lux, K. Kumar and H. Waldmann, Angew. Chem., Int. Ed., 2014, 53, 11146–11157 CrossRef CAS PubMed;
(b) W. Lin, L. Yuan, Z. Cao, Y. Feng and L. Long, Chem. – Eur. J., 2009, 15, 5096–5103 CrossRef CAS PubMed;
(c) A. B. Northrup and D. W. MacMillan, J. Am. Chem. Soc., 2002, 124, 2458–2460 CrossRef CAS PubMed.
- Y. Wei and M. Shi, Chem. Rev., 2013, 113, 6659–6690 CrossRef CAS PubMed.
- S. Crotti, G. Belletti, N. Di Iorio, E. Marotta, A. Mazzanti, P. Righi and G. Bencivenni, RSC Adv., 2018, 8, 33451–33458 RSC.
- N. J. Martin and B. List, J. Am. Chem. Soc., 2006, 128, 13368–13369 CrossRef CAS PubMed.
- T. Sakamoto, J. Itoh, K. Mori and T. Akiyama, Org. Biomol. Chem., 2010, 8, 5448–5454 RSC.
- J.-J. Yun, M.-L. Zhi, W.-X. Shi, X.-Q. Chu, Z.-L. Shen and T.-P. Loh, Adv. Synth. Catal., 2018, 360, 2632–2637 CrossRef CAS.
- M. Bougauchi, S. Watanabe, T. Arai, H. Sasai and M. Shibasaki, J. Am. Chem. Soc., 1997, 119, 2329–2330 CrossRef CAS.
-
(a) B. M. Trost, Chem. Rev., 1978, 78, 363–382 CrossRef CAS;
(b) P. Tuchinda, V. Prapansiri, W. Naengchomnong and V. Reutrakul, Chem. Lett., 1984, 1427–1430 CrossRef CAS.
- P. Zhang and L.-C. Li, Synth. Commun., 1986, 16, 957–965 CrossRef CAS.
-
(a) L. Kollár and P. Pongrácz, J. Organomet. Chem., 2018, 866, 184–188 CrossRef;
(b) G. Wittig and H. D. Frommeld, Chem. Ber., 1964, 97, 3548–3559 CrossRef CAS.
-
(a) E. Balducci, E. Attolino and M. Taddei, Eur. J. Org. Chem., 2011, 311–318 CrossRef CAS;
(b) L. A. Paquette, B. E. Kern and J. Méndez-Andino, Tetrahedron Lett., 1999, 40, 4129–4132 CrossRef CAS.
- For the first Saegusa oxidation, see:
(a) Y. Ito, T. Hirao and T. Saegusa, J. Org. Chem., 1978, 43, 1011–1013 CrossRef CAS . For a recent review on Saegusa oxidation, see: ;
(b) T. Hirao, J. Org. Chem., 2019, 84, 1687–1692 CrossRef CAS PubMed.
-
(a) J. Choi, A. H. MacArthur, M. Brookhart and A. S. Goldman, Chem. Rev., 2011, 111, 1761–1779 CrossRef CAS PubMed;
(b) T. Diao and S. S. Stahl, J. Am. Chem. Soc., 2011, 133, 14566–14569 CrossRef CAS PubMed;
(c) J. Muzart and J. Pete, J. Mol. Catal., 1982, 15, 373–376 CrossRef CAS;
(d) K. C. Nicolaou, T. Montagnon, P. Baran and Y.-L. Zhong, J. Am. Chem. Soc., 2002, 124, 2245–2258 CrossRef CAS;
(e) M. Tokunaga, S. Harada, T. Iwasawa, Y. Obora and Y. Tsuji, Tetrahedron Lett., 2007, 48, 6860–6862 CrossRef CAS.
-
(a) G. E. Dobereiner and R. H. Crabtree, Chem. Rev., 2010, 110, 681–703 CrossRef CAS PubMed;
(b) K. C. Nicolaou, Y.-L. Zhong and P. Baran, J. Am. Chem. Soc., 2000, 122, 7596–7597 CrossRef CAS;
(c) M. Uyanik, M. Akakura and K. Ishihara, J. Am. Chem. Soc., 2009, 131, 251–262 CrossRef CAS.
- M. A. Bigi and M. C. White, J. Am. Chem. Soc., 2013, 135, 7831–7834 CrossRef CAS.
-
(a) R. J. Cox and A. S. Evitt, Org. Biomol. Chem., 2007, 5, 229–232 RSC;
(b) K. Miura, K. Yamamoto, A. Yamanobe, K. Ito, H. Kinoshita, J. Ichikawa and A. Hosomi, Chem. Lett., 2010, 39, 766–767 CrossRef CAS;
(c) F. Zeng and H. Alper, Org. Lett., 2013, 15, 2034–2037 CrossRef CAS PubMed;
(d) P. DeShong, D. R. Sidler and G. A. Slough, Tetrahedron Lett., 1987, 28, 2233–2236 CrossRef CAS.
-
(a) W. Goure, M. E. Wright, P. Davis, S. S. Labadie and J. Stille, J. Am. Chem. Soc., 1984, 106, 6417–6422 CrossRef CAS;
(b) A. Petz, G. Péczely, Z. Pintér and L. Kollár, J. Mol. Catal. A: Chem., 2006, 255, 97–102 CrossRef CAS;
(c) A. Petz, Z. Pintér and L. Kollár, J. Biochem. Biophys. Methods, 2004, 61, 241–245 CrossRef CAS PubMed.
- For the other protocols for the synthesis of α,β-unsaturated ketones, see: α-methylenation,
(a) Y.-M. Li, S.-J. Lou, Q.-H. Zhou, L.-W. Zhu, L.-F. Zhu and L. Li, Eur. J. Org. Chem., 2015, 3044–3047 CrossRef CAS;
(b) N. L. Carreño, H. V. Fajardo, A. P. Maciel, A. Valentini, F. M. Pontes, L. F. Probst, E. R. Leite and E. Longo, J. Mol. Catal. A: Chem., 2004, 207, 91–96 CrossRef ; Carbonylative-Heck reactions,;
(c) X. F. Wu, H. Jiao, H. Neumann and M. Beller, ChemCatChem, 2011, 3, 726–733 CrossRef CAS ; Isomerization of secondary propargylic alcohols,;
(d) K. Tanaka, T. Shoji and M. Hirano, Eur. J. Org. Chem., 2007, 2687–2699 CrossRef CAS.
-
(a) A. Brennführer, H. Neumann and M. Beller, Synlett, 2007, 2537–2540 Search PubMed;
(b) A. Brennführer, H. Neumann, S. Klaus, T. Riermeier, J. Almena and M. Beller, Tetrahedron, 2007, 63, 6252–6258 CrossRef;
(c) S. Klaus, H. Neumann, A. Zapf, D. Strübing, S. Hübner, J. Almena, T. Riermeier, P. Groß, M. Sarich, W. R. Krahnert, K. Rossen and M. Beller, Angew. Chem., Int. Ed., 2006, 45, 154–158 CrossRef CAS.
-
(a) K. M. Driller, S. Prateeptongkum, R. Jackstell and M. Beller, Angew. Chem., 2011, 123, 558–562 CrossRef;
(b) H. Neumann, A. Sergeev and M. Beller, Angew. Chem., Int. Ed., 2008, 47, 4887–4891 CrossRef CAS;
(c) J. Schranck, X. F. Wu, H. Neumann and M. Beller, Chem. – Eur. J., 2012, 18, 4827–4831 CrossRef CAS PubMed.
-
(a) J. Liu, K. Dong, R. Franke, H. Neumann, R. Jackstell and M. Beller, J. Am. Chem. Soc., 2018, 140, 10282–10288 CrossRef CAS PubMed;
(b) L. Wang, H. Neumann and M. Beller, Angew. Chem., Int. Ed., 2018, 57, 6910–6914 CrossRef CAS PubMed;
(c) S. Zhang, H. Neumann and M. Beller, Org. Lett., 2019 DOI:10.1021/acs.orglett.9b00765.
- For the application of L10 in catalysis, see:
(a) S. Zhang, H. Neumann and M. Beller, Chem. – Eur. J., 2018, 24, 67–70 CrossRef CAS PubMed ; For reviews demonstrating the improved performance of bulky monodentate ligands in Pd-catalyzed coupling reactions, see: ;
(b) R. B. Bedford, C. S. J. Cazin and D. Holder, Coord. Chem. Rev., 2004, 248, 2283–2321 CrossRef CAS;
(c) F. Bellina, A. Carpita and R. Rossi, Synthesis, 2004, 2419–2440 CAS.
- For an example of using divinyl ketones for cyclization reactions, see: A. J. Frontier and C. Collison, Tetrahedron, 2005, 61, 7577–7606 CrossRef CAS.
- Methyl vinyl ketones have been a class of key synthetic intermediates for decades, for details, see: N. C. Ross and R. Levine, J. Org. Chem., 1964, 29, 2341–2346 CrossRef CAS.
Footnote |
† Electronic supplementary information (ESI) available. See DOI: 10.1039/c9cc02210d |
|
This journal is © The Royal Society of Chemistry 2019 |
Click here to see how this site uses Cookies. View our privacy policy here.