DOI:
10.1039/C9RA01203F
(Paper)
RSC Adv., 2019,
9, 9187-9192
Synthesis of N-aryl β-amino acid derivatives via Cu(II)-catalyzed asymmetric 1,4-reduction in air†
Received
16th February 2019
, Accepted 11th March 2019
First published on 20th March 2019
Abstract
In the presence of the inexpensive and stable stoichiometric reductant polymethylhydrosiloxane (PMHS) as well as certain amounts of appropriate alcohol and base additives, the non-precious metal copper-catalyzed asymmetric 1,4-hydrosilylation of β-aryl or β-alkyl-substituted N-aryl β-enamino esters was well realized to afford a diverse range of N-aryl β-amino acid esters in high yields and excellent enantioselectivities (26 examples, 90–98% ee). This approach tolerated the handling of both catalyst and reactants in air without special precautions. The chiral products obtained have been successfully converted to the corresponding enantiomerically enriched β-lactam and unprotected β-amino acid ester, which highlighted the synthetic utility of the developed catalytic procedure.
Introduction
Enantiomerically pure N-aryl β-amino acids and their derivatives are very attractive targets for asymmetric synthesis in view of their usefulness as key structural backbones of many drug intermediates and natural products.1 For instance, they are important synthons in the synthesis of β-lactam, which have proven to be of interest as antibiotics,2 human leucocyte elastase inhibitors or β-lactamase inhibitors.3 One of the most facile methods toward enantiomerically enriched N-aryl β-amino acids and their derivatives is the catalytic enantioselective reduction of N-aryl β-dehydroamino acid derivatives.4 Ru,4,5 Rh,4,6 and Ir4e,7-catalyzed asymmetric hydrogenation of N-acyl protected β-dehydroamino acid esters have been intensively pursued and good to excellent enantioselectivities have been realized. With respect to the studies on the reduction of N-aryl β-dehydroamino acid derivatives, Zhang et al. presented the first Rh-catalyzed asymmetric hydrogenation of N-aryl β-enamino esters with good to high ee's in 2005.8 The enantioselective hydrogenation of exocyclic N-arylenamines mediated by Ir catalyst system was described by Zhou and co-workers in 2009.9 In 2014, Zhou et al. reported the non-noble metal nickel-catalyzed asymmetric transfer hydrogenation for the preparation of β-amino acid derivatives in good to excellent enantioselectivities while only 30% ee and 10% yield were obtained for the substrate ethyl β-phenyl β-(phenylamino)acrylate.10 In addition, organocatalytic asymmetric hydrosilylation of N-aryl β-enamino esters using HSiCl3 as the reducing reagent has also emerged as an efficient alternative to transition metal-catalyzed hydrogenation for the synthesis of chiral β-amino acids derivatives.11
Employing stoichiometric amounts of silane as reductant, copper hydride-catalyzed stereoselective conjugate reduction of β,β-disubstituted Michael acceptors represents a practical, efficient, and cost-effective method that generate enantioenriched carbonyl compounds possessing a tertiary stereocenter at the β-position.12 The first copper mediated asymmetric 1,4-hydrosilylation of various β-amino-substituted α,β-unsaturated esters to β-azaheterocyclic acid derivatives of excellent enantiopurities was disclosed by Buchwald et al. in 2004.13 Zheng and co-workers then successfully applied this catalyst system in the preparation of γ-amino butyric acid derivatives.14 By utilizing a Cu(II)/dipyridylphosphine (P-Phos)15/PMHS (polymethylhydrosiloxane) system, we described the highly enantioselective conjugate reduction of a variety of β-alkyl-substituted β-(acylamino)acrylates with up to 99% ee in 2011.16 Later on, we attempted to extend this catalyst system to the asymmetric 1,4-hydrosilylation of β-methyl β-(arylamino)acrylates, which rendered low-to-moderate yields and enantioselectivities (7 examples, 33–72% yield, 23–91% ee) in the presence of certain amounts of MeONa and tBuOH as additives.17 To the best of our knowledge, a highly stereoselective 1,4-reduction of β-substituted N-aryl β-enamino esters mediated by non-noble metal catalysts has not been realized at present. Herein, we report our systematical studies on the CuH-catalyzed asymmetric conjugate reduction in ambient atmosphere for constructing a broad assortment of chiral β-aryl or β-alkyl-substituted β-(arylamino) acid derivatives. Furtherly, the synthetic utility of the methodology was demonstrated by efficient conversion of representative enantiomerically enriched N-aryl β-amino acid esters to the corresponding unprotected β-amino acid ester and β-lactam.
Results and discussion
As almost no (E)-geometric isomers were obtained during the synthesis of substrates,18,19 we commenced our studies by examining the effects of various copper precursors on the conjugate reduction of the model substrate (Z)-methyl 3-phenyl-3-(phenylamino)acrylate 1a (Table 1). PMHS, which is a by-product of the organosilicon industry and has been well-known for its low-cost, non-toxicity and air stability, was selected as the hydride donor. As shown in entry 1, when 1a was submitted to a given set of conditions [10 mol% of CuF2, 4 mol% of L1a as the chiral ligand, 10 equiv. of PMHS as the reductant, 20 mol% of MeONa and 4 equiv. of tBuOH as the additives], the reaction proceeded in toluene at 60 °C under ambient atmosphere to 53% conversion after 60 h to furnish (−)-methyl 3-phenyl-3-(phenylamino)-propionate (2a) in 91% ee. Similar to previous findings,20 the extent of conversions varied considerably as function of the counterions of copper. Although promising enantioselectivities were achieved as well by applying Cu(OAc)2·H2O or Cu(OAc)2, lower activities exhibited (entries 3 and 4 vs. entry 1). Almost no reaction was observed by using CuCl2 or Cu(CH3COCH2COCF3)2 as the copper precursor (entries 2 and 6). With respect to CuTC, 45% conversion and 90% ee were reached (entry 5). In consideration of both activity and enantioselectivity, CuF2 appeared to be the preponderant choice.
Table 1 Effects of copper salts and ligands on the asymmetric 1,4-reduction of (Z)-methyl 3-phenyl-3-(phenylamino)acrylate 1aa
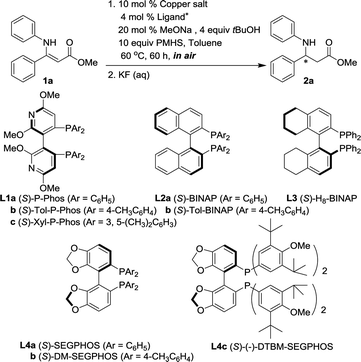
|
Entry |
Copper salt |
Ligand |
Conv.b (%) |
eec (%) |
Reaction conditions: 0.30 mmol substrate, substrate concentration = 0.30 M in toluene.
The conversions were determined by NMR and GC analysis.
The ee values were determined by chiral HPLC analysis (see the ESI).
n.d. = not determined.
|
1 |
CuF2 |
L1a
|
53 |
91 (−) |
2 |
CuCl2 |
L1a
|
<5 |
n.d.d |
3 |
Cu(OAc)2 |
L1a
|
31 |
90 (−) |
4 |
Cu(OAc)2·H2O |
L1a
|
10 |
90 (−) |
5 |
CuTC |
L1a
|
45 |
90 (−) |
6 |
Cu(CH3COCH2COCF3)2 |
L1a
|
<5 |
n.d.d |
7 |
CuF2 |
L1b
|
27 |
90 (−) |
8 |
CuF2 |
L1c
|
<5 |
85 (−) |
9 |
CuF2 |
L2a
|
<5 |
n.d.d |
10 |
CuF2 |
L2b
|
16 |
84 (−) |
11 |
CuF2 |
L3
|
<5 |
n.d.d |
12 |
CuF2 |
L4a
|
25 |
95 (−) |
13 |
CuF2 |
L4b
|
<5 |
84 (−) |
14 |
CuF2 |
L4c
|
<5 |
n.d.d |
Subsequently, the abilities of chiral ligands were investigated for the hydrosilylation of 1a (Table 1, entries 7–14). Among the chiral diphosphines screened, (S)-Tol-P-Phos (L1b) gave comparative ee with that of (S)-P-Phos under otherwise identical conditions (entry 7 vs. entry 1). Besides, a higher ee (95%) was achieved by employing (S)-SEGPHOS (L4a) as the chiral ligand while the reaction conversion was only 25% after 60 h (entry 12).
Further studies demonstrated that the reaction outcomes also largely relied on the selection of both base and alcohol additives (Table 2), which was consistent with previous findings.12c,13,16,21 When MeONa was replaced with more bulky EtONa or tBuONa, the enantioselectivity remained almost unchanged using (S)-P-Phos as the chiral ligand whilst a lower reaction activity was rendered (Table 2, entries 1 and 2 vs.Table 1, entry 1). To our delight, the replacement of tBuOH with less sterically encumbered alcoholic additive MeOH led to dramatic enhancements in reaction activity [53% conv. to 98% conv. for (S)-P-Phos L1a, 25% conv. to >99% conv. for (S)-SEGPHOS L4a, Table 2, entries 3 and 6 vs.Table 1, entries 1 and 12]. Moreover, utilizing (S)-SEGPHOS as the ligand, in the presence of MeOH and tBuONa as the additives, the desirable product 2a was obtained quantitatively (>99% conversion, 92% isolated yield) with 96% ee (entry 7).
Table 2 Effects of additives on the asymmetric 1,4-reduction of (Z)-methyl 3-phenyl-3-(phenylamino) acrylate 1aa
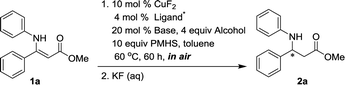
|
Entry |
Ligand |
Alcohol |
Base |
Conv.b (%) |
eec (%) |
Reaction conditions: 0.30 mmol substrate, substrate concentration = 0.30 M in toluene.
The conversions were determined by NMR and GC analysis.
The ee values were determined by chiral HPLC analysis.
The isolated yield was 92%.
|
1 |
L1a
|
tBuOH |
EtONa |
44 |
90 (−) |
2 |
L1a
|
tBuOH |
tBuONa |
44 |
90 (−) |
3 |
L1a
|
MeOH |
MeONa |
98 |
89 (−) |
4 |
L1a
|
MeOH |
EtONa |
98 |
90 (−) |
5 |
L1a
|
MeOH |
tBuONa |
>99% |
89 (−) |
6 |
L4a
|
MeOH |
MeONa |
>99% |
95 (−) |
7 |
L4a
|
MeOH |
tBuONa |
>99%
d
|
96 (−) |
With the aforementioned preferred conditions in hand, we set out to establish the general utility of this copper-catalyzed protocol for the asymmetric conjugate reduction of a vast array of N-aryl β-aryl β-enamino esters 1b–s in air. As the results summarized in Table 3 indicated, consistently high enantioselectivities were obtained in all cases (91–98% ee). Replacing the methyl ester of 1a with ethyl ester (1b) slightly diminished the enantiopurity of the product (entry 1 vs.Table 2, entry 7). The introduction of a para-MeO substituent to the N-arene ring of 1a resulted in distinct decreases in reaction activities (entries 4 and 6 vs.Table 2, entry 7). Similarly, the presence of an electron-donating group on the β-aryl group had a pronounced influence on the reactivities (entries 7, 8, 13 and 14 vs.Table 2, entry 7). For instance, when the ortho-position of β-phenyl on 1a was substituted by a methoxy group (1h), the isolated yield of chiral product dropped from 92% (Table 2, entry 7) to 22% (entry 7). Nonetheless, the existence of an electron-withdrawing group on the β-aryl group favored the conjugate reductions in terms of both activities and enantioselectivities (entries 3, 9–12 and 15–18).
Table 3 Copper-catalyzed asymmetric hydrosilylation of various N-aryl β-aryl β-enamino estersa
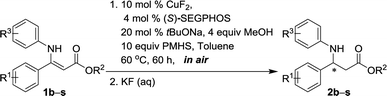
|
Entry |
Substrate |
R1 |
R2 |
R3 |
Yieldb (%) |
eec (%) |
Reaction conditions: 0.30 mmol substrate, substrate concentration = 0.30 M in toluene.
Isolated yield.
The ee values were determined by chiral HPLC analysis.
|
1 |
1b
|
H |
Et |
H |
90 |
93 (+) |
2 |
1c
|
4-MeO |
Et |
H |
65 |
92 (+) |
3 |
1d
|
4-Br |
Et |
H |
94 |
94 (+) |
4 |
1e
|
H |
Me |
4-MeO |
30 |
94 (+) |
5 |
1f
|
4-Me |
Me |
4-MeO |
90 |
93 (+) |
6 |
1g
|
4-Cl |
Me |
4-MeO |
30 |
95 (−) |
7 |
1h
|
2-MeO |
Me |
H |
22 |
92 (−) |
8 |
1i
|
3-MeO |
Me |
H |
62 |
95 (−) |
9 |
1j
|
3-F |
Me |
H |
95 |
94 (+) |
10 |
1k
|
3-Cl |
Me |
H |
94 |
94 (−) |
11 |
1l
|
3-Br |
Me |
H |
93 |
98 (+) |
12 |
1m
|
3-CF3 |
Me |
H |
95 |
96 (+) |
13 |
1n
|
4-Me |
Me |
H |
88 |
91 (+) |
14 |
1o
|
4-MeO |
Me |
H |
65 |
94 (−) |
15 |
1p
|
4-F |
Me |
H |
96 |
94 (+) |
16 |
1q
|
4-Cl |
Me |
H |
95 |
94 (−) |
17 |
1r
|
4-Br |
Me |
H |
94 |
95 (−) |
18 |
1s
|
4-CF3 |
Me |
H |
96 |
94 (+) |
Encouraged by the successful 1,4-hydrosilylation of N-aryl β-aryl β-enamino esters, we then applied the present catalyst system in the enantioselective conjugate reduction of a wide scope of β-alkyl, β-naphthyl or β-heteroaryl substituted N-phenyl β-enamino esters (3a–g). Gratifyingly, as illustrated in Scheme 1, the present protocol worked effectively for the productive access to a variety of desirable products (4a–g) of excellent enantiopurities (90–98% ee) under a given set of conditions. The sterically hindered β-alkyl substituent on the substrates was conductive to higher ee values (4gvs.4d–f).
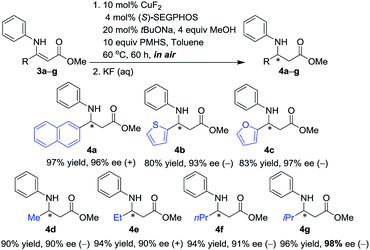 |
| Scheme 1 Copper-catalyzed asymmetric hydrosilylation of β-heteroaryl or β-alkyl-substituted N-phenyl β-enamino esters. | |
With the availability of an effective catalytic method for the asymmetric preparation of structurally diverse β-substituted β-(arylamino) acid esters, a range of other enantiomerically enriched molecules become accessible. For instance, as Scheme 2 outlined, treatment of (−)-methyl 3-phenyl-3-(phenylamino)-propionate (2a, 95% ee) with methylmagnesium bromide in ether at −40 °C furnished chiral β-lactam (R)-1,4-diphenylazetidin-2-one (5) in 70% yield with 94% ee after 1 h.22 The β-lactam derivatives possess the basic skeleton of monobactam antibiotics,2 β-lactamase inhibitors,3 and cholesterol absorption inhibitors.23 Moreover, N-(para-methoxyphenyl) group of 2f (93% ee) was readily deprotected by using ceric ammonium nitrate (CAN) at −10 °C for only 1 h to provide β-amino ester (R)-methyl 3-amino-3-(p-tolyl)propanoate 6 in 73% yield and 91% ee,24 which constitutes crucial structural elements of β-peptides and many other biologically active compounds.25
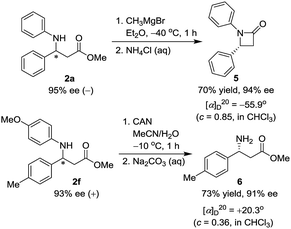 |
| Scheme 2 Conversion of N-aryl β-amino esters 2a and 2f to chiral β-lactam 5 and unprotected β-amino ester 6. | |
Conclusions
In conclusion, in the presence of certain amounts of appropriate additives tBuONa and MeOH, the combination of catalytic amounts of CuF2 and chiral ligand SEGPHOS as well as the stoichiometric hydride donor PMHS generated in situ an efficient catalyst system for the asymmetric conjugate reduction of a broad spectrum of β-aryl, β-heteroaryl, or β-alkyl-substituted N-aryl β-enamino esters with good activity and uniformly high ee values (26 examples, 90–98% ee). The present catalyst system features high air-stability, excellent stereocontrols, cost efficiency, and mild conditions and therefore offers a good opportunity for the practical preparation of N-aryl β-amino acid derivatives. The efficient transformation of enantiomerically enriched N-aryl β-amino esters to β-lactam and unprotected β-amino ester further evinced the good utility of this methodology.
Experimental
General procedure of asymmetric hydrosilylation in air [Table 2, entry 7, (Z)-methyl 3-phenyl-3-(phenylamino)acrylate, 1a]
CuF2 (3.0 mg, 3.0 × 10−2 mmol), (S)-SEGPHOS (L4a, 7.3 mg, 1.2 × 10−2 mmol) and sodium tert-butoxide (5.8 mg, 6.0 × 10−2 mmol) were weighed under air and placed in a 25 mL round-bottomed flask equipped with a magnetic stirring bar. Toluene (0.5 mL) was added and the mixture was stirred at room temperature for 30 min. Then PMHS (200 μL, 3.0 mmol) was added, and the solution was allowed to stir for further 10 min. Finally, a solution of (Z)-methyl 3-phenyl-3-(phenylamino)acrylate 1a (76 mg, 0.3 mmol) and MeOH (49 μL, 1.2 mmol) in toluene (0.5 mL) was added under vigorous stirring and the flask was stoppered. The reaction was carried out at 60 °C and monitored by TLC. Upon completion, the reaction mixture was treated with saturated KF solution (2 mL) and 2.0 mL diethyl ether. The mixture was stirred vigorously for 1 h. The aqueous layer was extracted with diethyl ether (3 × 3 mL). The combined organic layer was washed with water, dried over anhydrous Na2SO4, filtered through a plug of silica gel and concentrated in vacuo to provide the crude product. The conversion was determined by NMR and GC (column, HP-5; 25 m × 0.25 mm, carrier gas, N2). The enantiomeric excess of the product (−)-methyl 3-phenyl-3-(phenylamino)propanoate 2a was determined by chiral HPLC (column, Daicel Chiralcel OD-H, 25 cm × 4.6 mm) analysis. The pure product was isolated by column chromatography (ethyl acetate
:
petroleum ether = 1
:
10).
Procedure for the synthesis of β-lactam (R)-1,4-diphenylazetidin-2-one (5)22
To a solution of compound (−)-2a (95% ee, 50 mg, 0.20 mmol) in anhydrous Et2O (5 mL) was added dropwise a solution of 1 M CH3MgBr in Et2O (0.4 mL, 0.40 mmol) at −40 °C under nitrogen atmosphere. After stirring at −40 °C for 1 h, the reaction was quenched by adding an excess amount of saturated aqueous NH4Cl solution, followed by extracting with Et2O (2 × 10 mL). The organic phase was washed with brine and then dried over anhydrous Na2SO4, filtered, and concentrated under vacuum. The residue was purified by column chromatography on silica gel (ethyl acetate
:
petroleum ether = 1
:
15) to afford the chiral β-lactam 5 (31 mg, 70% yield, 94% ee) as a white solid. The ee value was determined by chiral HPLC analysis with a 25 cm × 4.6 mm Daicel Chiralcel OD-H column (eluent, 2-propanol/hexane 4
:
96; flow rate: 1.0 mL min−1; detection: 254 nm light).
Procedure for the synthesis of (R)-methyl 3-amino-3-(p-tolyl)propanoate (6)24a
A solution of ceric ammonium nitrate (280 mg, 0.51 mmol) in water (5 mL) was added dropwise to a solution of compound (+)-2f (93% ee, 50 mg, 0.17 mmol) in acetonitrile (5 mL) at −10 °C over 10 min. After the mixture was stirred for 1 h, water (5 mL) was added and MeCN was evaporated under vacuum. The residue was washed with Et2O (2 × 10 mL) and then added 10% aqueous Na2CO3 solution until pH = 6. The mixture was further washed with Et2O (2 × 10 mL). After the pH of the aqueous solution was tuned to be 8 by further adding 10% aqueous Na2CO3 solution, the mixture was extracted with EtOAc (3 × 10 mL). The combined organic layers were dried over anhydrous Na2SO4, filtered, and concentrated under vacuum. The residue was purified by column chromatography on silica gel (ethyl acetate: petroleum ether = 1
:
1) to give 6 (24 mg, 73% yield, 91% ee) as a brown oil. The ee value was determined by chiral HPLC analysis with a 25 cm × 4.6 mm Daicel Chiralcel OD-H column (eluent, 2-propanol/hexane 1
:
99; flow rate: 1.0 mL min−1; detection: 215 nm light).
Conflicts of interest
There are no conflicts to declare.
Acknowledgements
We thank the National Natural Science Foundation of China (21172049, 91127010, 21032003, and 21773052), the Program for Changjiang Scholars and Innovative Research Team in Chinese University (IRT 1231), the Natural Science Foundation of Zhejiang Province (LZ13B030001 and LZ16B020002), and the Program for Social Development of Hangzhou (20170533B10) for generous financial support.
Notes and references
-
(a) M. F. Pozza, K. Zimmermann, S. Bischoff and K. Lingenhohl, Prog. Neuro-Psychopharmacol. Biol. Psychiatry, 2000, 24, 647–670 CrossRef CAS
;
(b) L. Zhi, C. M. Tegley, K. B. Marschke and T. K. Jones, Bioorg. Med. Chem. Lett., 1999, 1009–1012 CrossRef CAS
.
-
(a) S. Hata, T. Iwasawa, M. Iguchi, K. Yamada and K. Tomioka, Synthesis, 2004, 1471–1475 CAS
;
(b) C. C. Silveira, A. S. Vieira, A. L. Braga and D. Russowsky, Tetrahedron, 2005, 61, 9312–9318 CrossRef CAS
;
(c) X.-R. Li, C.-F. Lu, Z.-X. Chen, Y. Li and G.-C. Yang, Tetrahedron: Asymmetry, 2012, 23, 1380–1384 CrossRef CAS
.
-
(a) R. Joyeau, A. Felk, S. Guilaume, M. Wakselman, I. Vergely, C. Doucet, N. Roggetto and M. Reboud-Ravaux, J. Pharm. Pharmacol., 1996, 48, 1218–1230 CrossRef CAS
;
(b) M. Bordeau, F. Frébault, M. Gobet and J.-P. Picard, Eur. J. Org. Chem., 2006, 4147–4154 CrossRef CAS
.
- For some representative reviews, see:
(a) M. Liu and M. P. Sibi, Tetrahedron, 2002, 58, 7991–8035 CrossRef CAS
;
(b) J.-A. Ma, Angew. Chem., Int. Ed., 2003, 42, 4290–4299 CrossRef CAS
;
(c) C. Bruneau, J.-L. Renaud and T. Jerphagnon, Coord. Chem. Rev., 2008, 252, 532–544 CrossRef CAS
;
(d) B. Weiner, W. Szymański, D. B. Janssen, A. J. Minnaard and B. L. Feringa, Chem. Soc. Rev., 2010, 39, 1656–1691 RSC
;
(e) J.-H. Xie, S.-F. Zhu and Q.-L. Zhou, Chem. Rev., 2011, 111, 1713–1760 CrossRef CAS
;
(f) Y.-H. Ma, Y.-J. Zhang and W.-B. Zhang, Chin. J. Org. Chem., 2007, 27, 289–297 CAS
;
(g) P. S. Bhadury, S. Yang and B.-A. Song, Curr. Org. Synth., 2012, 9, 695–726 CrossRef CAS
and references cited therein.
- Examples include:
(a) W. D. Lubell, M. Kitamura and R. Noyori, Tetrahedron: Asymmetry, 1991, 2, 543–554 CrossRef CAS
;
(b) Y.-G. Zhou, W. Tang, W.-B. Wang, W. Li and X. Zhang, J. Am. Chem. Soc., 2002, 124, 4952–4953 CrossRef CAS
;
(c) J. Wu, X. Chen, R. Guo, C.-H. Yeung and A. S. C. Chan, J. Org. Chem., 2003, 68, 2490–2493 CrossRef CAS
;
(d) L. Qiu, J. Wu, S. Chan, T. T.-L. Au-Yeung, J.-X. Ji, R. Guo, C.-C. Pai, Z. Zhou, X. Li, Q.-H. Fan and A. S. C. Chan, Proc. Natl. Acad. Sci. U. S. A., 2004, 101, 5815–5820 CrossRef CAS
;
(e) L. Qiu, F. Y. Kwong, J. Wu, W. H. Lam, S. Chan, W.-Y. Yu, Y.-M. Li, R. Guo, Z. Zhou and A. S. C. Chan, J. Am. Chem. Soc., 2006, 128, 5955–5965 CrossRef CAS
.
- Examples include:
(a) G. Zhu, Z. Chen and X. Zhang, J. Org. Chem., 1999, 64, 6907–6910 CrossRef CAS
;
(b) M. Yasutake, I. D. Gridnev, N. Higashi and T. Imamoto, Org. Lett., 2001, 3, 1701–1704 CrossRef CAS
;
(c) D. Peña, A. J. Minnaard, J. G. de Vries and B. L. Feringa, J. Am. Chem. Soc., 2002, 124, 14552–14553 CrossRef
;
(d) W. Tang, W. Wang, Y. Chi and X. Zhang, Angew. Chem., Int. Ed., 2003, 42, 3509–3511 CrossRef CAS
;
(e) J. You, H.-J. Drexler, S. Zhang, C. Fischer and D. Heller, Angew. Chem., Int. Ed., 2003, 42, 913–916 CrossRef CAS
;
(f) M. T. Reetz and X. Li, Angew. Chem., Int. Ed., 2005, 44, 2959–2962 CrossRef CAS
;
(g) W. Tang, A. G. Capacci, A. White, S. Ma, S. Rodriguez, B. Qu, J. Savoie, N. D. Patel, X. Wei, N. Haddad, N. Grinberg, N. K. Yee, D. Krishnamurthy and C. H. Senanayake, Org. Lett., 2010, 12, 1104–1107 CrossRef CAS
;
(h) X. Zhang, K. Huang, G. Hou, B. Cao and X. Zhang, Angew. Chem., Int. Ed., 2010, 49, 6421–6424 CrossRef CAS
.
- S. Enthaler, G. Erre, K. Junge, K. Schröder, D. Addis, D. Michalik, M. Hapke, D. Redkin and M. Beller, Eur. J. Org. Chem., 2008, 3352–3355 CrossRef CAS
.
- Q. Dai, W. Yang and X. Zhang, Org. Lett., 2005, 7, 5343–5345 CrossRef CAS
.
- X.-B. Wang, D.-W. Wang, S.-M. Lu, C.-B. Yu and Y.-G. Zhou, Tetrahedron: Asymmetry, 2009, 20, 1040–1045 CrossRef CAS
.
- P. Yang, H. Xu and J. Zhou, Angew. Chem., Int. Ed., 2014, 53, 12210–12213 CrossRef CAS
.
- Examples for enantioselective organocatalytic reduction of N-aryl β-enamino esters include:
(a) A. V. Malkov, S. Stončius, K. Vranková, M. Arndt and P. Kočovský, Chem.–Eur. J., 2008, 14, 8082–8085 CrossRef CAS
;
(b) H. J. Zheng, W. B. Chen, Z. J. Wu, J. G. Deng, W. Q. Lin, W. C. Yuan and X. M. Zhang, Chem.–Eur. J., 2008, 14, 9864–9867 CrossRef CAS
;
(c) A. V. Malkov, K. Vranková, S. Stončius and P. Kočovský, J. Org. Chem., 2009, 74, 5839–5849 CrossRef CAS
;
(d) Y. Jiang, X. Chen, Y. Zheng, Z. Xue, C. Shu, W. Yuan and X. Zhang, Angew. Chem., Int. Ed., 2011, 50, 7304–7307 CrossRef CAS
;
(e) S. Jones and X. Li, Tetrahedron, 2012, 68, 5522–5532 CrossRef CAS
;
(f) P. Zhang, C. Wang, L. Zhou and J. Sun, Chin. J. Chem., 2012, 30, 2636–2640 CrossRef CAS
;
(g) X. Chen, X.-Y. Hu, C. Shu, Y.-H. Zhang, Y.-S. Zheng, Y. Jiang, W.-C. Yuan, B. Liu and X.-M. Zhang, Org. Biomol. Chem., 2013, 11, 3089–3093 RSC
;
(h) Y. Jiang, X. Chen, X.-Y. Hu, C. Shu, Y.-H. Zhang, Y.-S. Zheng, C.-X. Lian, W.-C. Yuang and X.-M. Zhang, Adv. Synth. Catal., 2013, 355, 1931–1936 CrossRef CAS
;
(i) J. Ye, C. Wang, L. Chen, X. Wu, L. Zhou and J. Sun, Adv. Synth. Catal., 2016, 358, 1042–1047 CrossRef CAS
;
(j) D. Brenna, R. Porta, E. Massolo, L. Raimondi and M. Benaglia, ChemCatChem, 2017, 9, 941–945 CrossRef CAS
;
(k) X. Dai, G. Weng, S. Yu, H. Chen, J. Zhang, S. Cheng, X. Xu, W. Yuan, Z. Wang and X. Zhang, Org. Chem. Front., 2018, 5, 2787–2793 RSC
.
- For representative reviews, see:
(a) O. Riant, N. Mostefai and J. Courmarcel, Synthesis, 2004, 2943–2958 CrossRef CAS
;
(b) S. Rendler and M. Oestreich, Angew. Chem., Int. Ed., 2007, 46, 498–504 CrossRef CAS PubMed
;
(c) C. Deutsch, N. Krause and B. H. Lipshutz, Chem. Rev., 2008, 108, 2916–2927 CrossRef CAS PubMed
;
(d) B. H. Lipshutz, Synlett, 2009, 509–524 CrossRef CAS
and references cited therein; ;
(e) J. Chen and Z. Lu, Org. Chem. Front., 2018, 5, 260–272 RSC
.
- M. P. Rainka, Y. Aye and S. L. Buchwald, Proc. Natl. Acad. Sci. U. S. A., 2004, 101, 5821–5823 CrossRef CAS PubMed
.
- J. Deng, X.-P. Hu, J.-D. Huang, S.-B. Yu, D.-Y. Wang, Z.-C. Duan and Z. Zheng, J. Org. Chem., 2008, 73, 6022–6024 CrossRef CAS PubMed
.
-
(a) J. Wu and A. S. C. Chan, Acc. Chem. Res., 2006, 39, 711–720 CrossRef CAS PubMed
;
(b) C.-C. Pai, C.-W. Lin, C.-C. Lin, C.-C. Chen, A. S. C. Chan and W. T. Wong, J. Am. Chem. Soc., 2000, 122, 11513–11514 CrossRef CAS
.
- Y. Wu, S.-B. Qi, F.-F. Wu, X.-C. Zhang, M. Li, J. Wu and A. S. C. Chan, Org. Lett., 2011, 13, 1754–1757 CrossRef CAS PubMed
.
- Y. Sui, Q. Fang, M. Li, Y. Hu, H. Xia, S. Li and J. Wu, Chin. J. Chem., 2012, 30, 2611–2614 CAS
.
- L. Zhang and J. Herndon, Organometallics, 2004, 23, 1231–1235 CrossRef CAS
.
- A. Gossauer, F. Nydegger, T. Kiss, R. Sleziak and S.-E. Helen, J. Am. Chem. Soc., 2004, 126, 1772–1780 CrossRef CAS PubMed
.
-
(a) S. Sirol, J. Courmarcel, N. Mostefaï and O. Riant, Org. Lett., 2001, 3, 4111–4113 CrossRef CAS PubMed
;
(b) J. Wu, J. X. Ji and A. S. C. Chan, Proc. Natl. Acad. Sci. U. S. A., 2005, 102, 3570–3575 CrossRef CAS PubMed
;
(c) F. Yu, J.-N. Zhou, X.-C. Zhang, Y.-Z. Sui, F.-F. Wu, L.-J. Xie, A. S. C. Chan and J. Wu, Chem.–Eur. J., 2011, 17, 14234–14240 CrossRef CAS PubMed
.
-
(a) J. Yun and S. L. Buchwald, J. Am. Chem. Soc., 1999, 121, 5640–5644 CrossRef CAS
;
(b) D. S. Hays and G. C. Fu, Tetrahedron, 1999, 55, 8815–8832 CrossRef CAS
;
(c) G. Hughes, M. Kimura and S. L. Buchwald, J. Am. Chem. Soc., 2003, 125, 11253–11258 CrossRef CAS PubMed
;
(d) B. H. Lipshutz, J. M. Servesko and B. R. Taft, J. Am. Chem. Soc., 2004, 126, 8352–8353 CrossRef CAS PubMed
.
-
(a) V. Michaut, F. Metz, J.-M. Paris and J.-C. Plaquevent, J. Fluorine Chem., 2007, 128, 889–895 CrossRef CAS
;
(b) S. Tang, J. He, Y. Sun, L. He and X. She, J. Org. Chem., 2010, 75, 1961–1966 CrossRef CAS PubMed
.
- C. P. Cannon, N. Engl. J. Med., 2015, 372, 2387–2397 CrossRef CAS PubMed
.
-
(a) D. R. Kronenthal, C. Y. Han and M. K. Taylor, J. Org. Chem., 1982, 47, 2765–2768 CrossRef CAS
;
(b) M. Rodríguez-Mata, E. García-Urdiales, V. Gotor-Fernández and V. Gotor, Adv. Synth. Catal., 2010, 352, 395–406 CrossRef
.
-
(a) B. Geueke, T. Heck, M. Limbach, V. Nesatyy, D. Seebach and H.-P. Kohler, FEBS J., 2006, 273, 5261–5272 CrossRef CAS PubMed
;
(b) Y. Zhang, L. Li, W. Yuan and X. Zhang, Chem. Res. Chin. Univ., 2015, 31, 381–387 CrossRef CAS
;
(c) A. Onoda, H. Harada, T. Uematsu, S. Kuwabata, R. Yamanaka, S. Sakurai and T. Hayashi, RSC Adv., 2017, 7, 1089–1092 RSC
.
Footnotes |
† Electronic supplementary information (ESI) available: Spectral, analytical data for all substrates and chiral products. See DOI: 10.1039/c9ra01203f |
‡ These authors contributed equally to this work. |
|
This journal is © The Royal Society of Chemistry 2019 |
Click here to see how this site uses Cookies. View our privacy policy here.