Size-fractionation of trace elements in dusty snow from open pit bitumen mines and upgraders: collection, handling, preparation and analysis of samples from the Athabasca bituminous sands region of Alberta, Canada†
Received
4th May 2021
, Accepted 3rd February 2022
First published on 4th February 2022
Abstract
A robust sample collection, handling, processing and analytical method was developed for reliable quantification of trace elements (TEs) in dusty snow. Experiments were conducted by spiking the snow and ASTM Type I water, with liquid and solid multielemental standards under both acidified (pH < 2) and unacidified conditions (pH ∼6) for a time series analysis up to one month in the metal-free ultraclean SWAMP laboratory. For the “dissolved” concentration of TEs (<0.45 μm), except for Ag, all 39 TEs investigated were precisely quantified at low levels (10 to 30 ng L−1). Regarding particulate matter (>0.45 μm), there was a significant loss by entrapment to the walls of polypropylene bottles and syringes. Employing metal-free sampling protocols, snow was collected from five bogs in the Athabasca Bituminous Sands (ABS) region, one of the largest reservoirs of bitumen in the world, to determine the impact of airborne emissions from open pit mining and upgrading. The “dissolved” fraction of bitumen enriched TEs (V, Ni, and Mo) and potentially toxic TEs (Cd, Pb, Sb, and Tl) was low. For all the TEs, including the conservative lithophiles (La and Th), total concentrations in snow and in the dust extracted from snow were greater near mining activities, whereas microscopic analyses revealed a greater size, abundance, and variability of dust particles. The enrichment factors of TEs in dust showed insignificant enrichment near industrial activities (e.g., Pb EF = 1). Furthermore, the results show that most TEs in snow near industry occur almost exclusively in the particulate fraction.
Environmental significance
Open pit mining activities generate massive quantities of dust, which makes it very challenging to reliably determine the concentrations of trace elements (TEs) in the “dissolved” fraction (i.e., filterable through 0.45 μm membranes). Employing metal-free ultraclean lab protocols, multiple experiments were conducted to develop and validate a reliable method to clearly distinguish between “dissolved” and particulate TEs in dusty snow. Open pit mining and upgrading of the Athabasca Bituminous Sands (ABS) have been reported to be a significant source of potentially toxic TEs. Here, we report that the “dissolved” concentrations of TEs in snow from the ABS region were extremely low and that most TEs occur almost exclusively in the particulate fraction. Molybdenum may be a helpful tracer of atmospheric deposition from industrial activities in the region.
|
Introduction
Snowpack is an excellent archive of wintertime atmospheric deposition of dust and chemical contaminants. It has been used worldwide for decades to measure the deposition of trace elements (TEs).1–15 The atmospheric cycling of TEs including emission, transportation and deposition depends on numerous environmental factors including the physicochemical properties of TE species and particle size distribution.16–20 The residence and storage of TEs in snowpack are subject to dynamic processes affecting their influx and translocation during intermittent snowmelts.21,22 For example, TE release from snowpack includes elution when temperatures are above freezing, with the soluble forms of TEs i.e. those found in a <0.45 μm or “dissolved” fraction, eluting early during the melting process.16 It is well established that the accumulation, transformation and release of TEs in snowpack are relevant to aquatic and terrestrial environments.23–26
Although the average concentration of water-soluble TEs in a snowpack is generally low, the first fractions of meltwater runoff are enriched with soluble TEs, which are swept out with the first infiltrating liquid.16 The snow cover can store TEs at subfreezing temperatures and suddenly release the “dissolved” fraction of TEs when the temperature rises.27,28 The load of soluble TEs in a snowpack can be concentrated as much as five-fold in the first fractions of snowmelt runoff, and the melt–freeze cycles can further concentrate the “dissolved” fraction of TEs in a certain portion of the snow cover, preparing the concentrated pool of TEs for rapid release.16 Once a layer of snow is deposited, it undergoes metamorphism, and the individual snowflakes disappear as the snow grains change by increasing the grain size and surface area.16,22 The specific surface area of snow particles is continuously reduced, and their boundaries migrate, causing a segregation of TEs from snow particles as the snow/ice crystalline lattice melt and recrystallize.16 The snow grains are thus continuously purified, and therefore most of the TE load is carried where the liquid water moves through the snow. Clearly, the reliable determination of the “dissolved” fraction of TEs in snow has broad environmental and ecological significance, as this fraction is considered most bioavailable. The presence of particulate matter in snow makes it very challenging to determine the concentrations of TEs in the “dissolved” fraction, due to the risk of solid–solution interactions during sample handling including collection in the field, plus melting and acidification in the laboratory.
Open pit mining activities, whether these are limestone or aggregate quarries,29 or open air mines for base metals,30 iron ore,31,32 coal,33,34 uranium,35 diamonds36 or bitumen,37,38 all generate massive quantities of dust, further promoted by heavy vehicles.39 These mechanically generated dusts are dominated by comparatively large particles:40 once emitted to the air, they are locally deposited through sedimentation to the surrounding landscape, including vegetation, soils, and surface water. The scale of such mining activities is enormous: globally, the area affected by mining activities is more than 57
000 km2.41 The impact of these dusts is clearly seen in snow near mining activities, especially when compared to pristine snow from remote regions (Fig. 1).
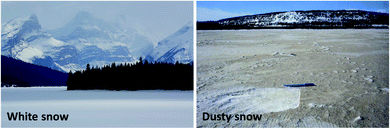 |
| Fig. 1 Snow with and without dust deposition. White snow, Jasper National Park (photo credit, William Shotyk). Dusty snow, Athabasca River, downwind from dry tailings (photo credit, Mark Donner). | |
Snowpack sampling is one of the approaches that has been used to identify the environmental impacts of open pit mining and upgrading activities of the Athabasca Bituminous Sands (ABS) in northeastern Alberta.42–44 Based on the recent studies of TEs in snow and water, it was reported that open pit mining and upgrading of the ABS is a significant source of Pb to the Athabasca River along with many other chalcophile elements including Ag, Cd, Sb and Tl.42 To assess potential impacts on aquatic and terrestrial ecosystems, and to understand the relevance to human health, it is essential to accurately quantify the fraction of TEs that can be released during snowmelt. To determine TEs in snow, particularly the “dissolved fraction”, snowpack sampling, handling and preparation are extremely critical to avoid any potential contamination and subsequently derive false statistical inferences i.e., Type I statistical error. On the one hand, metal-free ultraclean lab methods must be used to reliably quantify TEs in snow while avoiding sample contamination.45,46 On the other hand, when snow samples are melted in the lab, TEs can be lost from solution by adsorption to the walls of the containers.47,50 In the current study, employing metal-free ultraclean lab protocols, multiple laboratory experiments were conducted to optimize the sample handling, preparation and analysis of TEs in dusty snow.
The objectives of this study were: (1) to develop and validate a reliable and robust method to clearly distinguish between “dissolved” and particulate TEs in dusty snow, (2) to determine “dissolved” and total TE concentrations in snow from selected locations within the ABS region, and (3) to characterize the particulate matter which is clearly visible in snow samples collected near mining and upgrading activities. The elements included in the current study were: potentially toxic TEs of environmental concern (i.e., Ag, Cd, Pb, Sb and Tl); conservative, lithophile elements (e.g., La and Th), which reflect the abundance of mineral matter; the TEs which are known to be enriched in bitumen51 and may be emitted to the air from bitumen extraction, processing and upgrading (i.e., V, Ni and Mo).
Methodology
Sample containers, cleaning, and preparation
Wide mouth 1 L polypropylene bottles (PP, Fisher Scientific) were used to collect the snow samples. Before sampling, the bottles were thoroughly cleaned following the SWAMP Lab cleaning procedure. Briefly, the bottles were soaked in soapy water (5% v/v Fisher Versa Clean), rinsed with ASTM Type II water and then soaked in 5% w/w hydrochloric acid (HCl, Trace grade), rinsed and then soaked in 10% w/w nitric acid (HNO3, Trace grade), rinsed and then leached at 80 °C with fresh 10% w/w HNO3. After leaching, the bottles were rinsed 3 times with ASTM Type II water and 3 times with ASTM Type I water. The bottles were air dried in a metal-free PP Class 100, laminar flow clean air cabinet. Once the bottles were completely dried, the lids were put on and the bottles were packed in PE ziplock bags in preparation for work in the field to collect snow samples. The detailed bottle cleaning procedure is provided in the ESI.†
Sample collection
For practice, fresh snow samples were collected in triplicate from the campus of the University of Alberta, to optimize the method for the determination of TEs in snow. Snow samples were also collected in March 2015 from five ombrotrophic peat bogs, namely MIL, JPH4, McK, McM and ANZ from the Athabasca Bituminous Sands region (Table S1† and Fig. 2). These bogs were used to study contemporary atmospheric metal deposition using Sphagnum moss52 and age-dated peat cores had been collected for retrospective analyses.53 The sampling sites were selected based on the distance from the midpoint of the ABS mining and upgrading activities (Fig. 2). It should be noted that the snow samples collected from the Anzac (ANZ) bog are located 9.3 km from an upgrader that was operational at that time. This upgrader was later (January 2016) shut down (Long Lake Upgrader|Oil Sands Magazine). At each peat bog, triplicate snow samples were collected from 3 locations within each bog (n = 9 per bog). The details of the sampling from the ABS regions are provided in the ESI.†
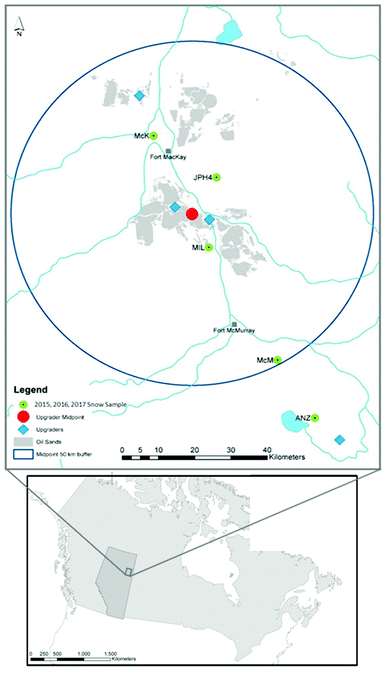 |
| Fig. 2 Location of the five peat bogs where snow samples were collected (MIL, JPH4, McK, McM and ANZ). The map also shows the industrial zone, tailings ponds (grey areas), locations of the bitumen upgraders (blue diamonds) and the midpoint (red circle) used to measure the distances between the sampling locations and the mid-point between two central upgraders. | |
Laboratory experiments for snow sample handling and processing
Losses of dissolved TEs by adsorption to container walls.
The snow samples collected from the University of Alberta were initially put in a fridge (4 °C) to slowly thaw the snow for ∼12 h. Afterwards, the thawed samples were transferred to metal free, laminar flow clean air cabinets (Class 100). The snowmelt was filtered through acid cleaned PTFE 0.45 μm filters into 125 mL acid cleaned PP bottles and spiked with 10, 20 and 30 ng L−1 of dissolved TEs using CLMS-1 and CLMS-2AN multielement standards as well as Mo, Re, and Sb single element standards (SPEX CertiPrep). Un-spiked snow samples were also included to determine background TE concentrations. Overall, 40 TEs were tested, and two sets of samples were prepared for comparison: one set was not acidified to mimic the actual snowmelt samples for dissolved TEs and the other set was acidified. The pH of the un-acidified snow samples was adjusted to ∼6, similar to the actual snowmelt, using a buffer made from ultrapure ammonium carbonate ((NH4)2CO3, >99.999% trace metals basis: Sigma Aldrich, St Louis, Missouri, USA). The pH of the acidified snow samples was adjusted to <2 using HNO3 (0.5%) that had been purified twice by sub-boiling distillation. To determine the optimum times for sample analysis and storage, all of the samples were analysed at selected time intervals: immediately after spiking (T0 h), after one hour (T1 h), after 1 day (T1 day, 24 h), after 1 week (T1 week, 168 h) and after 1 month (T1 month, 720 h). In addition to the snow samples, simulation samples prepared with ASTM Type I Milli-Q water (MQW) were also used to repeat the experiment. Furthermore, the same experiment for dissolved TEs was also conducted at higher TE concentrations (200 ng L−1). After the experiment, the empty bottles were cut open and studied for TE adsorption.
The details of the other experiments to determine the losses of particulate TEs by entrapment in the pores of container walls and proton-promoted dissolution of particulate matter during sample acidification are provided in the ESI.†
Processing of snow collected from the ABS region
A schematic flowchart for the sample processing is provided in Fig. 3. The surface and subsurface snow samples were initially put in a fridge (4 °C) to slowly thaw the snow for ∼12 h, and after that the thawed samples were transferred to the metal free, laminar flow clean air cabinets (Class 100). Immediately after melting (within one hour), an aliquot of the unacidified surface snowmelt was filtered through acid-cleaned PTFE 0.45 μm filters using acid-cleaned PP syringes. After filtration, the surface snow sample filtrates were acidified (pH < 2) using 0.5% HNO3 to determine “dissolved”/filterable TE concentrations (<0.45 μm).
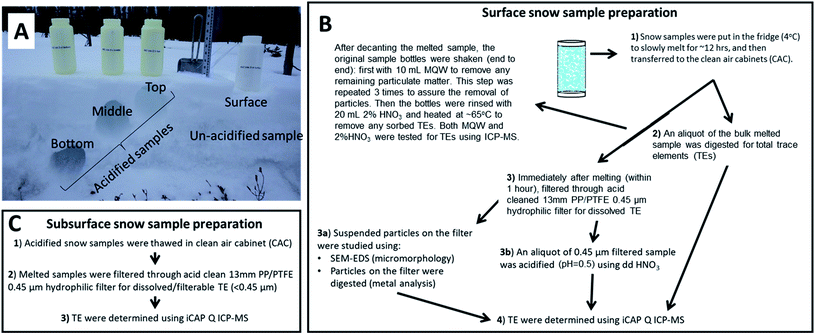 |
| Fig. 3 Illustration of (A) collected snow samples and sample preparation of (B) unacidified surface snow, and (C) acidified subsurface snow samples. | |
For total TE concentrations, an aliquot (2.00 mL) of the melted unfiltered, unacidified surface snow sample was digested in concentrated HNO3 (3.00 mL) using high-pressure microwave-assisted digestion (Ultraclave, MLS GmbH, Leutkirch, Germany). The dissolved TE concentrations were subtracted from the totals to determine the operationally defined particulate TE concentrations. To determine the concentration of TEs in dust/particulate matter (>0.45 μm) in surface snow, the 0.45 μm filters used to filter the snowmelt were taken to extract the particulate matter which was then digested in HNO3 (6 mL) and HBF4 (0.2 mL, Laboratory Grade). The details of the digestion program as well the procedure for extracting dust particles from snow are reported elsewhere.52,54
Analytical methods
ICP-QMS analysis.
Trace element concentrations were determined using a quadrupole ICP-MS (iCAP Qc, Thermo Fisher Scientific, Waltham, MA, USA) in kinetic energy discrimination mode (KED) as described in Shotyk et al. (2014).52 The autosampler of the ICP-MS is housed within a metal-free, Class 100, laminar flow clean air cabinet. Multielement stock solutions (1 and 2A, Spex CertiPrep Metuchen, NJ, USA) as well as single-element solutions (Mo, Re, and Sb) were diluted daily as appropriate to create the calibration curves. A linear regression was confirmed for all selected isotopes (R2 > 0.99) before sample analysis. Using indium (115In, 5 μg L−1) as the internal standard, introduced through a separate line along with the samples, all samples were analyzed in triplicate, with instrument parameters of 80 sweeps and 30 ms dwell time for all isotopes. The ICP-QMS tune settings and operating parameters are provided previously.55 The accuracy of the analyses of aqueous solutions was determined using certified, standard reference materials for freshwater (NIST 1640a and SPS-SW2). These results are summarized in Table S2,† along with the corresponding Limits of Detection (LODs) and Limits of Quantification (LOQs).
Scanning electron microscopy (SEM) of dust in surface snow.
SEM was used to obtain high-resolution (3 nm) and high magnification (250
000×) digital images of the pieces cut from the bottoms of the PP bottles and syringes and to study the surface texture and microstructures of particulate matter in snow. The samples were mounted on aluminum stubs using double-sided carbon tape and examined under variable pressure (VP) mode without any pre-treatment or coating of the particles. A high spatial resolution JEOL SEM (Field Emission) located in the Department of Earth Sciences, University of Alberta was used. The details of the SEM procedure were provided previously.56,57
Statistical analysis
To determine the statistical significance of the difference between the mean values of trace element concentrations between sampling sites and sampling years, analysis of variance (ANOVA) was employed using SAS 9.3 statistical software. After the significant difference from ANOVA, the least significant difference (LSD) was employed for pairwise comparison between two means.
Results and discussion
A robust analytical method was developed for reliable quantification of TEs at low levels in snow (10 to 30 ng L−1). Multiple time series laboratory experiments were conducted by spiking snow and ASTM Type I water, with liquid and solid multi-elemental standards in the metal-free ultraclean SWAMP laboratory for reliable quantification of TEs.
Optimization of snow sample handling and processing
Dissolved/filterable TEs (<0.45 μm).
The results of the experiments conducted to optimize the recovery of dissolved TEs are reported in Table S3.† In MQW, the spiked concentration (10 to 30 ng L−1) of all the dissolved TEs, except Ag, were successfully recovered at all the time intervals (90 to 110%, T0 to T1 month). Both acidified and unacidified samples yielded similar and reproducible results. Likewise, in snow, the recoveries of all the spiked TEs, except Ag, were acceptable in both acidified and unacidified samples (85 to 113%, spiked – background concentrations in snow). The recoveries of all the dissolved TEs, except Ag, spiked at 200 ng L−1 were also excellent (±10%), both in MQW and snow.
Silver – a challenging trace element.
Silver appeared to be a very challenging element to reliably recover in both MQW and snow. Immediately after spiking with 10 to 30 ng L−1, about 60 to 70% of the spiked Ag was lost from the un-acidified MQW samples (Fig. 4A). The Ag loss was even greater (∼80% at T0) in the un-acidified snowmelt sample (Fig. 4B). After T0, no further loss of Ag was found. For the experiment conducted at higher concentration (200 ng L−1 Ag spiked), the initial Ag loss from the unacidified MQW and snowmelt samples was low (8 to 9% of the spiked concentration, Fig. 4C). However, after a month of incubation, around 70% of the spiked Ag was lost from the unacidified snowmelt sample (Fig. 4C). Overall, the recovery of Ag was very low in unacidified samples (29 to 44% in MQW and 17 to 22% in snow). This Ag loss from the un-acidified samples was due to the sorption to the bottle walls, as it is well known that Ag can be adsorbed to container walls such as borosilicate glass, Pyrex and polyethylene.47–50
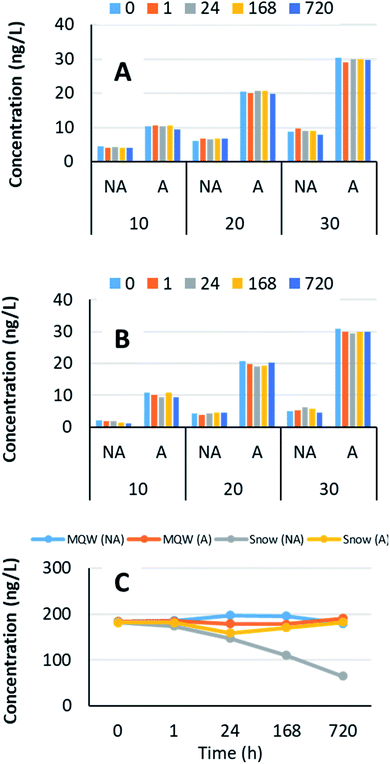 |
| Fig. 4 Concentrations of Ag in the acidified (A) and not acidified (NA) snow and Milli Q water (MQW) spiked with 10, 20, 30 and 200 ng L−1 multielemental standards (CLMS-1 and CLMS-2AN) as well as Mo, Re, and Sb. The experiments were designed to determine sorption to polypropylene (PP) bottle walls over time: immediately after spiking (T0), after one hour (1), after 1 day (24), after 1 week (168) and after 1 month (720). (A) Silver in MQW, (B) silver in snow and (C) silver in MQW and snow (200 ng L−1 spiked). | |
Concern about the emissions of Ag to the environment from bitumen mining and upgrading began with the paper by Kelly et al. (2010).42 In the intervening period, we have found no evidence of environmental Ag contamination of plants52,58,59 or surface water.53,57,60,61 Moreover, age-dated peat cores show that atmospheric Ag deposition in the region has been in decline for decades.62 Given the extremely low concentrations of Ag in all of the samples collected to date, the challenges associated with its reliable determination and the lack of evidence of environmental contamination, there is no real need for further studies of this element in the ABS region.
Contribution of particulate matter for dissolved TEs.
All the TEs were more abundant in the filterable fraction (<0.45 μm) of the samples spiked with coal fly ash, soil, sediments and road dust compared to the un-spiked samples (Table S4†). Coal fly ash and soil released much higher TE concentrations than the sediments and road dust, both in MQW and snow. As expected, TE release was much higher in the acidified samples compared to the un-acidified samples. Overall, these results suggest that the snow samples must be kept un-acidified and processed as quickly as possible to accurately determine the dissolved fraction of TEs in snowpack.
Losses of particulate matter.
The results of the SEM-EDX study of the entrapment of particulate matter in the pores of the bottle walls are shown in Appendix A.† Particle adsorption was found for all the spiked reference materials i.e., soil, sediment, road dust and coal fly ash. However, a greater abundance of particles was adsorbed from the road dust. The size of the adsorbed particles ranged from ∼1 μm to ∼10 μm. In addition to the bottle walls, particles were also retained on the walls of syringes used to filter the bulk snow samples. The loss of particulate matter makes it challenging to perform the mass balance calculations for TEs. In addition to PP, Teflon bottles were also examined using SEM to illustrate the morphology and surface structure. Teflon surfaces are much smoother and non-porous compared to PP (Fig. 5) and are therefore better suited for sampling snow and water samples rich in particulate matter.
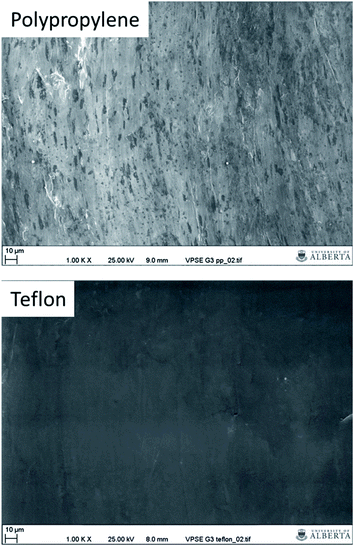 |
| Fig. 5 Scanning electron microscopy images of unused polypropylene (PP) and Teflon. Black spots on the PP represent a porous surface. | |
Dissolved/filterable TEs (<0.45 μm) in snow from the ABS region
TEs enriched in bitumen (V, Ni and Mo).
Overall, the “dissolved” concentrations of the TEs in the snow were low (Fig. 6). The concentrations of V and Ni, the two most abundant TEs in bitumen,63 were not significantly different among the different sites (Fig. 6). For example, MIL and JPH4 are 11 and 12 km, respectively, from the midpoint between the two central bitumen upgraders, whereas ANZ is 68 km from the midpoint. The maximum V concentration was found in snow from McK (25 km from the midpoint), whereas the maximum Ni was found at ANZ. In contrast to V and Ni, the greatest concentrations of dissolved Mo were found near mining and upgrading activities (186.7 ± 34.0 ng L−1 at MIL).
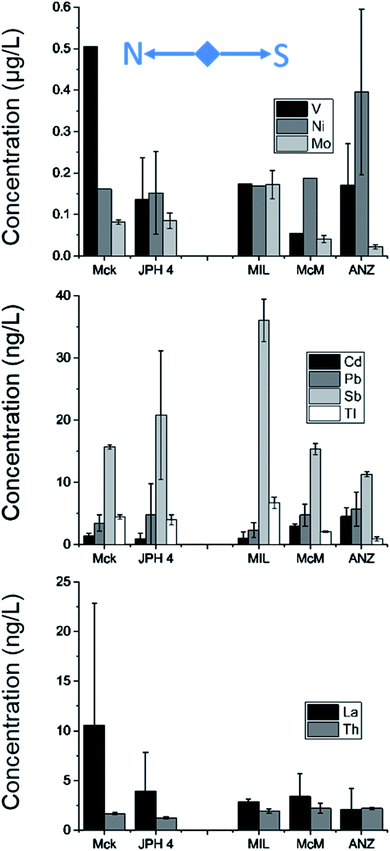 |
| Fig. 6 Dissolved trace element (TE) concentrations in surface snow samples collected in 2015 from five ombrotrophic peat bogs: MIL, JPH4, McK, McM and ANZ. The bars in the graphs show the average TE concentrations of triplicate samples and error bars represent 1 standard deviation. The blue diamond represents the mid-point between the two central bitumen upgraders. | |
Potentially toxic TEs (Cd, Pb, Sb, and Tl).
The “dissolved” concentrations of Cd, Pb, Sb and Tl were also low in all the snow samples (Fig. 6). In fact, the concentrations of Cd and Pb in the snow were similar in all the sampling sites in the region. The concentrations of Sb and Tl in the snow from MIL and JPH4 were greater (2–3 times for Sb and 4–7 times for Tl) compared to ANZ. The results of conservative lithophile elements showed ∼2 times more La at JPH4 compared to ANZ (Fig. 6). For perspective, the concentrations of “dissolved” TEs in the snow samples of the ABS region are comparable to or lower than the concentrations found in the same fraction of the Athabasca River (AR),53 suggesting that snowmelt is not a significant source of these elements to receiving water.
Total and particulate TEs
The total concentrations of all the TEs were greater near mining operations (Fig. 7). The concentrations of TEs found in bitumen (V, Ni, and Mo) were 2 to 6 times greater in the snow from JPH4 and MIL compared to ANZ. For the potentially toxic TEs, the concentrations were up to 5 times greater near mining activities. However, conservative lithophile elements such as La and Th were also greater at MIL and JPH4 compared to ANZ. Comparing all these elements, the total concentrations of Cd, Mo, Ni, Pb, Sb, Tl and V showed strong, positive correlations with La (r = 0.7–1.0) and Th (r = 0.5–1.0), illustrating the importance of mineral matter as hosts for the TEs in snow. Furthermore, by calculating the particulate fraction of TEs (total – dissolved concentrations), the greatest proportion by far (77 to 100%) of almost all the TEs was found in the particulate fraction (Table S5†).
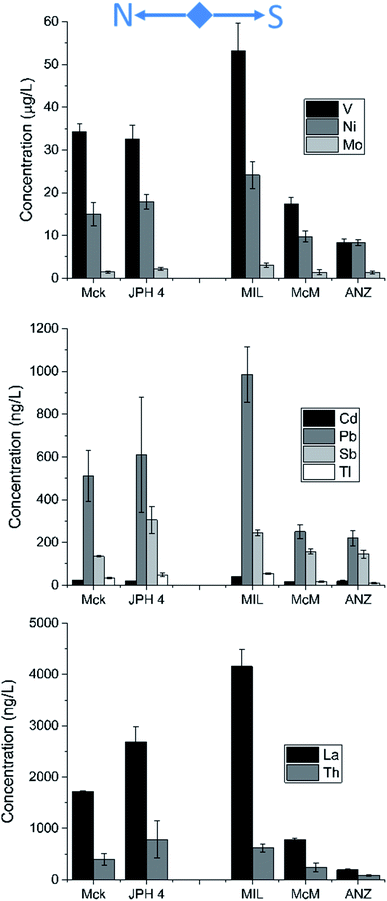 |
| Fig. 7 Total TE concentrations in snow samples collected in 2015 from five ombrotrophic peat bogs: MIL, JPH4, McK, McM and ANZ. The blue diamond represents the mid-point between the two central bitumen upgraders. | |
The concentrations of all the TEs in dust particles extracted from snow were higher near mining activities (Fig. 8). Specifically, V, Ni and Mo were 11, 3 and 3 times, respectively, greater in the dust from MIL compared to ANZ. At JPH4, the concentrations of these three elements were 2 to 6 times greater. For potentially toxic TEs, the concentrations were up to 7 times greater at MIL or JPH4 than ANZ. The concentrations of La and Th were also more abundant at MIL and JPH4 (6 to 7 times and 7 to 9 times, respectively) compared to ANZ. To put all of these data into perspective, the concentrations of TEs in the dusts extracted from snow can be compared to their corresponding concentrations in the Upper Continental Crust (UCC). Clearly, the concentrations of TEs in the dusts of the ABS region are almost always below their natural abundance in crustal materials (Fig. 8). Thus, these dusts tend to be impoverished regarding potentially toxic TEs, with respect to crustal values.
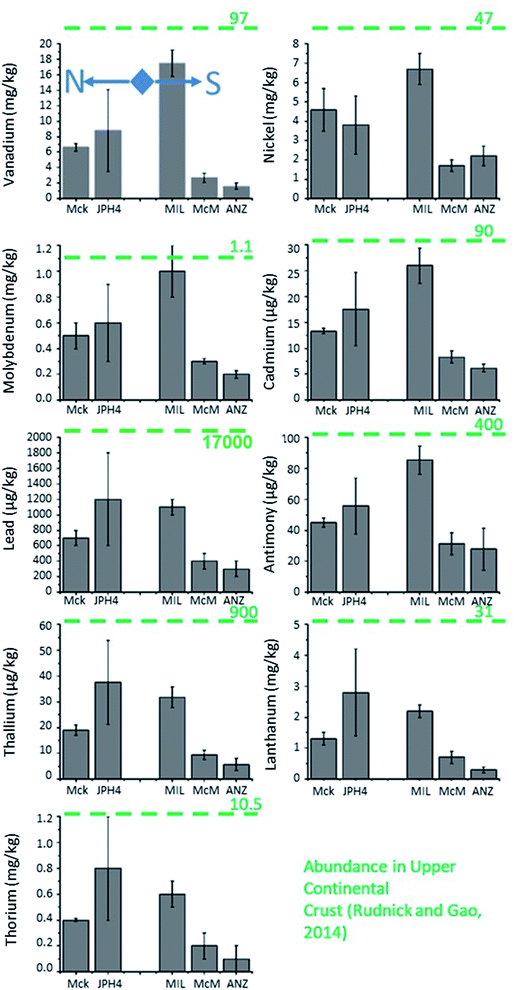 |
| Fig. 8 Trace element (TE) concentrations in dust (>0.45 μm) extracted from snow collected in 2015 from five ombrotrophic peat bogs: MIL, JPH4, McK, McM and ANZ. The green dashed lines on the graphs correspond to the abundance of TEs in the upper continental crust. The blue diamond represents the mid-point between the two central bitumen upgraders. | |
Enrichment factors of TEs in dust
To identify the relative contribution of anthropogenic versus natural sources of TEs to the atmosphere, the crustal enrichment factor (EF) is often used.64–70 The EF is defined as the concentration ratio of a given TE to that of a conservative, lithophile element such as Al, Th or Sc (or any other element which derives mainly from soil dust), normalised to the same concentration ratio in the UCC. In the current study, TE EFs were calculated using the ratio of TE/Th in the dust particles extracted from surface snow normalized to the same concentration ratio of the UCC. The concentrations of TEs in the UCC were adopted from Rudnick and Gao (2014).71 Overall, these calculations showed no TE enrichment in dust in the snow (Table S6†). In fact, the average EF of Pb was 1, which showed that the abundance of Pb in the dust particles obtained from snow is the same as that of the crustal material. For other potentially toxic TEs, the EF was ∼5 for Cd and Sb and ∼1 for Tl. For bitumen enriched TEs, the EF was <5 for V and Ni, but it was ∼15 for Mo. Thus, Mo might be a suitable tracer for monitoring the aerial deposition of TEs from industrial activities in the ABS region.
Micromorphology of dust extracted from snow
The main sources of mineral dusts in the ABS region include open-pit mines, beaches and dykes of tailings ponds, exposed overburden, and gravel roads.37,38 Using physical methods of analysis, mineral matter from these sources is difficult to distinguish from the natural background of atmospheric dusts supplied by wind erosion of soils. The dust extracted from snow contained quartz, sulfides (such as pyrite), feldspar, amphibole, zircon, and clay minerals (Appendix B†), which are the most common mineral phases in bituminous sands and other coarse-grained sedimentary rocks.72,73 Also, the dust particles in snow near industrial activities (i.e., MIL) showed a greater size, variability and abundance. At McM and ANZ, fly ash was also found (Appendix B†). A larger diversity in particle sizes was also reported in the ash fraction of Sphagnum moss sampled from bogs closer to industrial activities compared to moss sampled farther away.74
Acid soluble trace elements
The concentrations of acid soluble TEs in snow collected from three depths (15, 30 and 45 cm) are provided in Tables S5 and S7.† On average, the concentration of V was clearly greater in snow near the mining operations (i.e., at MIL), but the difference in concentrations was not statistically significant (P = 0.08) among the five bogs. The concentrations of Ni showed a similar pattern, but Mo was lower in the snow near industrial operations. The acid soluble concentrations of Cd, Pb, Sb and Tl in snow at MIL and JPH4 were greater compared to ANZ (Table S5†). Comparing the lithophile elements, the concentrations of La and Th were also greater, by 1 to 3 orders of magnitude, at MIL and JPH4 compared to ANZ. Again, these results suggest that mineral dusts are the dominant source of TEs in the snow of the region.
After carefully reviewing the TE results, a pattern of increasing concentrations with depth was observed for sites closer to industrial activities (Table S7†). For example, for Pb at MIL, JPH4 and McM, the concentrations increased from the top to the bottom snow layers. Similar increases were observed for Ni, Mo, Cd, Sb, Tl, La and Th at least at one site. These observations suggest a metamorphism of snow that results in TE segregation and relocation within the snowpack.
Comparison with published data for TEs in snow from the ABS region
For context, the concentrations of TEs are compared to the corresponding concentrations reported in previous studies conducted in the ABS region.42–44,75 The work by Kelly et al. (2010)42 did not provide data for dissolved V and Mo in snow, even though V is the most abundant metal in bitumen.63 Comparing Ni data, our dissolved Ni values near industrial development are remarkably similar to that of reported by Kelly et al., (2010).42 Comparing the particulate TE concentrations, Pb was similar between the current study (510.8 ± 312.3 ng L−1) and the one by Kelly et al. (2010;42 660 ± 180 ng L−1). But for the other TEs, there was a great difference in the concentrations, probably due to the abundance of particulate matter. Comparing our results with other work, our average dissolved V, Ni and Mo concentrations are 3, 2 and 6 times (Table 1), respectively greater than those reported by Guéguen et al. (2016).43 Our total TE concentrations are also greater than those reported by Guéguen et al. (2016).43 Bari et al. (2014)75 also investigated the atmospheric deposition of (total recoverable) TEs in snow from the ABS region. They found 2 to 7 times greater deposition of Cd, Pb, Sb and Tl near industrial activities (at the WBEA/Mannix sampling location, 7.5 km from the midpoint between the two central upgraders) compared to a site 68 km far from the midpoint. Comparing these two sites in their study, similar differences were also found in Al deposition (7 times more near industrial activities). While these authors did not correct the differences for the abundance of mineral matter (e.g. using Al), it would appear that most of the variation in TE concentrations can be explained in terms of differences in dust deposition. Gopalapillai et al. (2019)44 conducted a temporal and spatial study for the source analysis of TEs in winter air deposition in the Athabasca oil sands region. Similar to our findings for total TEs, they found a greater abundance of 18 elements (Al, As, Ba, Be, Ce, Co, Cs, Fe, Ga, La, Mo, Pb, Rb, Ti, U, V, W, and Y) in the unfiltered snow near the industrial operations. Overall, using a hybrid source analysis, they reported a general decrease in the atmospheric loadings of trace elements over time (1978 to 2016).
Table 1 Concentrations of dissolved, acid soluble, particulate, total trace elements (TEs), and TEs in dust extracted from snow samples collected in 2015 from peat bogs in the Athabasca Bituminous Sands region (ABS)a
|
Current study |
Athabasca Bituminous Sands (ABS) region |
Dissolved, ng L−1 |
Acid soluble, μg L−1 |
Particulate, μg L−1 |
Total, μg L−1 |
Trace elements in dust, mg kg−1 |
Notes: – no data. The results for the current study are average concentrations (n = 15) ± 1 std. dev. For comparison, the results of previous studies are also included in the table (lower portion).
|
V |
208 ± 172 |
4.16 ± 5.78 |
28.9 ± 17.2 |
29.1 ± 17.2 |
6.20 ± 6.42 |
Ni |
213 ± 103 |
3.37 ± 2.14 |
14.8 ± 6.47 |
15.0 ± 6.40 |
3.20 ± 2.40 |
Mo |
80.4 ± 58.0 |
0.10 ± 0.05 |
1.81 ± 0.66 |
1.90 ± 0.71 |
0.43 ± 0.40 |
Cd |
2.10 ± 1.60 |
0.01 ± 0.00 |
0.02 ± 0.01 |
0.02 ± 0.01 |
0.01 ± 0.01 |
Pb |
4.20 ± 1.30 |
0.41 ± 0.28 |
0.51 ± 0.31 |
0.52 ± 0.31 |
0.62 ± 0.50 |
Sb |
19.8 ± 9.60 |
0.03 ± 0.02 |
0.18 ± 0.07 |
0.20 ± 0.08 |
0.05 ± 0.02 |
Tl |
3.60 ± 2.20 |
0.01 ± 0.01 |
0.03 ± 0.02 |
0.03 ± 0.02 |
0.02 ± 0.01 |
La |
4.60 ± 3.40 |
0.50 ± 0.42 |
1.90 ± 1.60 |
1.90 ± 1.60 |
1.22 ± 1.10 |
Th |
1.80 ± 0.40 |
0.04 ± 0.05 |
0.42 ± 0.30 |
0.42 ± 0.30 |
0.40 ± 0.31 |
|
Previous studies |
Guéguen et al., 2016 43 |
Kelly et al., 2010 42 |
Shotyk et al., 2010 68 |
ABS region |
ABS region |
Southern Ontario |
Dissolved, ng L−1 |
Total, ng L−1 |
Dissolved, ng L−1 |
Particulate, ng L−1 |
Acid soluble, ng L−1 |
Johnson and Parnell |
Luther bog |
Sifton bog |
V |
64 ± 2732 |
526 ± 366 |
— |
— |
172 ± 105 |
233 ± 288 |
314 ± 135 |
Ni |
110 ± 1991 |
165 ± 1 |
240 ± 46 |
3900 ± 1900 |
336 ± 261 |
130 ± 101 |
159 ± 62 |
Mo |
16.0 |
40.6 |
— |
— |
75 ± 26 |
102 ± 72 |
124 ± 33 |
Cd |
7.0 |
17 |
56 ± 26 |
490 ± 210 |
35 ± 27 |
10 ± 8 |
17 ± 7 |
Pb |
21 |
203.3 |
750 ± 0 |
660 ± 180 |
672 ± 264 |
747 ± 726 |
798 ± 396 |
Sb |
13.0 |
13.1 |
28 ± 2 |
330 ± 68 |
31 ± 18 |
50 ± 55 |
66 ± 29 |
Tl |
1.0 |
2.5 |
32 ± 0 |
15 ± 7 |
2.5 ± 1.4 |
2.5 ± 2.6 |
3.5 ± 1.6 |
La |
— |
— |
— |
— |
— |
— |
— |
Th |
2.0 |
19 |
— |
— |
1.8 ± 0.7 |
5.5 ± 6.5 |
3.1 ± 0.9 |
Comparison of TE ratios in snow with bulk ABS, bitumen and mineral fractions
Trace element ratios in snow may be compared to their respective ratios in bulk ABS, as well as its bitumen and mineral fractions to determine potential TE sources. The TE contents in the ABS and its fractions were reported by Bicalho et al. (2017),51 and the ratios are summarized in Table S8.† The ratios of TEs that are known to be enriched in bitumen were (V/Ni, V/Mo, and Ni/Mo): 2.6, 24.1, and 9.1, respectively in bulk ABS; 2.9, 23.3, and 8.0 respectively in the bitumen fraction; and 2.3, 44.0, and 19.0, respectively in the mineral fraction. These ratios of the “dissolved” concentrations of TEs in snow (V/Ni, 0.9; V/Mo, 2.7; Ni/Mo, 3.0) are very different from the ratios in bulk ABS, bitumen and mineral fractions (Table S8†). Comparing the total and particulate concentrations, only Ni/Mo ratios in snow (7.9 and 8.2, respectively) were comparable to the ratio in bitumen (8.0). The acid soluble TE ratios in snow (V/Ni, 1.2; V/Mo, 43.9; Ni/Mo, 35.5) differ from these ratios in bulk ABS and bitumen fractions. However, compared to the mineral fraction of the ABS, similar results were found, particularly for V/Mo. Taken together, these results showed that snow near industrial activities is generally depleted in V and Ni compared to bitumen, and Mo is perhaps a better tracer of industrial activities related to bitumen mining and upgrading. The higher concentrations of Mo were also found to be associated with oil operations in Ecuador.76 Moreover, the chemical composition of the snow in the ABS region is most similar to the bulk composition of the ABS, and in particular the composition of the mineral fraction.
Ecological significance of TEs in the Athabasca River (AR)
The Peace–Athabasca Delta (PAD) is one of the largest freshwater deltas in the world and of tremendous ecological significance. There are legitimate concerns about a range of environmental impacts on the delta, including contaminants received from the AR watershed.77 Comparing the average dissolved concentrations of potentially toxic TEs (Cd, Pb, Sb and Tl) in the snow to the dissolved concentrations in the AR water reported by Shotyk et al. (2017),62 the TEs in snow were either similar e.g., Tl or lower e.g., Cd (6×), Pb (3×), and Sb (3×). Lower concentrations in the snow suggest that snowmelt does not have significant impacts on the TE dissolved loads to the AR. In the AR, using the metal-free ultraclean procedures and protocols developed for polar snow and ice, TEs in the dissolved fraction are at natural, background levels typical of uncontaminated freshwater.61,62 Moreover, the fractionation of dissolved TEs into colloidal (300 Da to 0.45 μm) and mainly ionic (<300 Da) forms shows that a significant proportion of the traditionally defined “dissolved” TE fraction is, in fact, bound with organic and inorganic colloids.60 Thus, in the lower AR watershed, these potentially toxic TEs are expected to have limited bioavailability.
Conclusions
A robust snow sampling, handling, processing and analytical method was developed for the reliable quantification of 40 trace elements (TEs). Except for Ag, the “dissolved” concentrations of all 39 TEs were precisely quantified at low levels (10 to 30 ng L−1). The entrapment of particulate matter to the walls of polypropylene bottles and syringes was observed, accounting for the loss of particulate matter during sample processing. Employing this method, the impact of airborne emissions from Athabasca Bituminous Sands (ABS) open pit mining and upgrading activities was studied using snow samples from 5 bogs. The dissolved fractions of Cd, Ni, Pb and V were similar at all sites, whereas Mo, Sb and Tl concentrations were greater near mining activities. The total concentrations of all the TEs including conservative lithophiles (i.e., La and Th) in snow and in the dust extracted from snow were greater near the industrial operations. However, the enrichment factors of the TEs in dust show insignificant enrichment of TEs near industrial activities (e.g., Pb EF = 1). Overall, the results show that ABS mining and upgrading activities have affected the total concentrations of TEs in snow, but not “dissolved” concentrations; in fact, the TEs are found almost exclusively in the particulate fraction. Molybdenum may be a useful tracer of atmospheric deposition from industrial activities in the region.
Conflicts of interest
There are no conflicts to declare.
Acknowledgements
We gratefully acknowledge the funding for this project provided jointly by Alberta Innovates (AI) and Canada's Oil Sands Innovation Alliance (COSIA), with special thanks to Brett Purdy and John Brogly, respectively. The SWAMP lab was created through the munificent support of the University of Alberta, the Faculty of Agricultural, Life and Environmental Sciences and Alberta Environment and Parks. The Canada Foundation for Innovation together with Alberta Enterprise and Advanced Education provided generous equipment grants. For outstanding support in the field, we are grateful to Dr Mark Donner, for administrative support to Tracy Gartner and to Rick Pelletier for GIS support (Fig. 2). We thank Fiorella Barraza for reviewing the revised version of the manuscript. We are thankful for the support from the Department of Earth and Atmospheric Sciences, U of Alberta for help in using SEM-EDX.
References
- G. Wu, X. Zhang, C. Zhang, S. Gao, Z. Li, F. Wang and W. Wang, Concentration and Composition of Dust Particles in Surface Snow at Urumqi Glacier No. 1, Eastern Tien Shan, Glob. Planet. Change, 2010, 74(1), 34–42 CrossRef.
- G. Wu, C. Zhang, X. Zhang, T. Xu, N. Yan and S. Gao, The Environmental Implications for Dust in High-Alpine Snow and Ice Cores in Asian Mountains, Glob. Planet. Change, 2015, 124, 22–29 CrossRef.
- D. S. Jeffries and W. R. Snyder, Atmospheric deposition of heavy metals in Central Ontario, Water, Air, Soil Pollut., 1981, 15(2), 127–152 CrossRef CAS.
- K. A. Morrison, E. S. Kuhn and C. J. Watras, Comparison of Three Methods of Estimating Atmospheric Mercury Deposition, Environ. Sci. Technol., 1995, 29(3), 571–576 CrossRef CAS PubMed.
- S. Azimi, A. Ludwig, D. R. Thévenot and J. L. Colin, Trace Metal Determination in Total Atmospheric Deposition in Rural and Urban Areas, Sci. Total Environ., 2003, 308(1–3), 247–256 CrossRef CAS PubMed.
- J. W. Erisman, H. Möls, P. Fonteijn, M. Geusebroek, G. Draaijers, A. Bleeker and D. van der Veen, Field intercomparison of precipitation measurements performed within the framework of the pan European intensive monitoring program of EU/ICP Forest, Environ. Pollut., 2003, 125(2), 139–155 CrossRef CAS PubMed.
- A. Avila and A. Rodrigo, Trace Metal Fluxes in Bulk Deposition, Throughfall and Stemflow at Two Evergreen Oak Stands in NE Spain Subject to Different Exposure to the Industrial Environment, Atmos. Environ., 2004, 38(2), 171–180 CrossRef CAS.
- W. Aas, L. Y. Alleman, E. Bieber, D. Gladtke, J. L. Houdret, V. Karlsson and C. Monies, Comparison of Methods for Measuring Atmospheric Deposition of Arsenic, Cadmium, Nickel and Lead, J. Environ. Monit., 2009, 11(6), 1276 RSC.
- M. Bacardit and L. Camarero, Fluxes of Al, Fe, Ti, Mn, Pb, Cd, Zn, Ni, Cu, and As in Monthly Bulk Deposition over the Pyrenees (SW Europe): The Influence of Meteorology on the Atmospheric Component of Trace Element Cycles and Its Implications for High Mountain Lakes, J. Geophys. Res.: Biogeosci., 2009, 114, G00D02 Search PubMed.
- J. Gunawardena, P. Egodawatta, G. A. Ayoko and A. Goonetilleke, Atmospheric Deposition as a Source of Heavy Metals in Urban Stormwater, Atmos. Environ., 2013, 68, 235–242 CrossRef CAS.
- A. M. Lai, M. M. Shafer, J. E. Dibb, C. M. Polashenski and J. J. Schauer, Elements and inorganic ions as source tracers in recent Greenland snow, Atmos. Environ., 2017, 164, 205–215 CrossRef CAS.
- M. R. Sierra-Hernández, P. Gabrielli, E. Beaudon, A. Wegner and L. G. Thompson, Atmospheric depositions of natural and anthropogenic trace elements on the Guliya ice cap (northwestern Tibetan Plateau) during the last 340 years, Atmos. Environ., 2018, 176, 91–102 CrossRef.
- T. Wei, Z. Dong, S. Kang, C. Zong, M. Rostami and Y. Shao, Atmospheric deposition and contamination of trace elements in snowpacks of mountain glaciers in the northeastern Tibetan Plateau, Sci. Total Environ., 2019, 689, 754–764 CrossRef CAS PubMed.
- Y. Li, J. Huang, Z. Li and K. Zheng, Atmospheric pollution revealed by trace elements in recent snow from the central to the northern Tibetan Plateau, Environ. Pollut., 2020, 263, 114459 CrossRef CAS PubMed.
- X. Jiao, Z. Dong, S. Kang, Y. Li, C. Jiang and M. Rostami, New insights into heavy metal elements deposition in the snowpacks of mountain glaciers in the eastern Tibetan Plateau, Ecotoxicol. Environ. Saf., 2021, 207, 111228 CrossRef CAS PubMed.
- S. C. Colbeck, A Simulation of the Enrichment of Atmospheric Pollutants in Snow Cover Runoff, Water Resour. Res., 1981, 17(5), 1383–1388 CrossRef.
- C. Zdanowicz, D. Kliza, D. Paktunc and G. F. Bonham-Carter, Physico-chemical characterization of airborne particulate impurities deposited in snow around a copper smelter, GSC Bull., 2005, 584 Search PubMed.
- C. Zdanowicz, G. Hall, J. Vaive, Y. Amelin, J. Percival, I. Girard, P. Biscaye and A. Bory, Asian dustfall in the St. Elias Mountains, Yukon, Canada, Geochim. Cosmochim. Acta, 2006a, 70, 3493–3507 Search PubMed.
- C. M. Zdanowicz, C. M. Banic, D. A. Paktunc and D. A. Kliza-Petelle, Metal emissions from a Cu smelter, Rouyn-Noranda, Québec: characterization of particles sampled in air and snow, Geochem.: Explor., Environ., Anal., 2006b, 6, 147–162 Search PubMed.
- P. W. Boyd and M. J. Ellwood, The biogeochemical cycle of iron in the ocean, Nat. Geosci., 2010, 3, 675–682 CrossRef CAS.
-
M. Viklander, Snow Quality in Urban Areas, PhD thesis, Luleå University of Technology, Luleå, Sweden, 1997.
- T. Meyer, Y. D. Lei and F. Wania, Measuring the release of organic contaminants from melting snow under controlled conditions, Environ. Sci. Technol., 2006, 40(10), 3320–3326 CrossRef CAS PubMed.
- L. A. Barrie and R. J. Vet, The concentration and deposition of acidity, major ions and trace metals in the snowpack of the Eastern Canadian shield during the winter of 1980–1981, Atmos. Environ., 1984, 18(7), 1459–1469 CrossRef CAS.
- S. K. Fortner, W. B. Lyons, A. G. Fountain, K. A. Welch and N. M. Kehrwald, Trace element and major ion concentrations and dynamics in glacier snow and melt: Eliot Glacier, Oregon Cascades, Hydrol. Processes, 2009, 23, 2987–2996 CrossRef CAS.
- A. Tovar-Sánchez, C. M. Duarte, J. C. Alonso, S. Lacorte, R. Tauler and C. Galbán-Malagón, Impacts of metals and nutrients released from melting multiyear Arctic sea ice, J. Geophys. Res., 2010, 115, C07003 Search PubMed.
- F. Cereceda-Balic, M. R. Palomo-Marín, E. Bernalte, V. Vidal, J. Christie, X. Fadic, J. L. Guevara, C. Miro and E. Pinilla Gil, Impact of Santiago de Chile urban atmospheric pollution on anthropogenic trace elements enrichment in snow precipitation at Cerro Colorado, Central Andes, Atmos. Environ., 2012, 47, 51–57 CrossRef CAS.
- H. Leivestad and I. P. Muniz, Fish kill at low pH in a Norwegian River, Nature, 1976, 259(5542), 391–392 CrossRef CAS PubMed.
- M. Johannessen and A. Hendriksen, Chemistry of snowmelt: changes in concentration during melting, Water Resour. Res., 1978, 14(4), 615–619 CrossRef CAS.
- S. A. Silvester, I. S. Lowndes and D. M. Hargreaves, A computational study of particulate emissions from an open pit quarry under neutral atmospheric conditions, Atmos. Environ., 2009, 43(40), 6415–6424 CrossRef CAS.
- B. Balabanova, T. Stafilov, R. Šajn and K. Bačeva, Distribution of chemical elements in attic dust as reflection of their geogenic and anthropogenic sources in the vicinity of the copper mine and flotation plant, Arch. Environ. Contam. Toxicol., 2011, 61(2), 173–184 CrossRef CAS PubMed.
- S. K. Chaulya, R. Trivedi, A. Kumar, R. K. Tiwary, R. S. Singh, P. K. Pandey and R. Kumar, Air quality modelling for prediction of dust concentrations in iron ore mines of Saranda region, Jharkhand, India, Atmos. Pollut. Res., 2019, 10(3), 675–688 CrossRef CAS.
- N. Kayet, K. Pathak, A. Chakrabarty, S. Kumar, V. M. Chowdary, C. P. Singh, S. Sahoo and S. Basumatary, Assessment of foliar dust using Hyperion and Landsat satellite imagery for mine environmental monitoring in an open cast iron ore mining areas, J. Cleaner Prod., 2019, 218, 993–1006 CrossRef.
- P. Trechera, T. Moreno, P. Córdoba, N. Moreno, X. Zhuang, B. Li, J. Li, Y. Shangguan, A. O. Dominguez, F. Kelly and X. Querol, Comprehensive evaluation of potential coal mine dust emissions in an open-pit coal mine in Northwest China, Int. J. Coal Geol., 2021, 235, 103677 CrossRef CAS.
- T. Wu, Z. Yang, A. Wang, K. Zhang and B. Wang, A study on movement characteristics and distribution law of dust particles in open-pit coal mine, Sci. Rep., 2021, 11(1), 1–10 CrossRef PubMed.
- H. B. L. Pettersson and J. Koperski, Investigation of aerial dispersion of radioactive dust from an open-pit uranium mine by passive vinyl collectors, Health Phys., 1991, 60(5), 681–690 CrossRef CAS PubMed.
- A. D. Watkinson, J. Virgl, V. S. Miller, M. A. Naeth, J. Kim, K. Serben, C. Shapka and S. Sinclair, Effects of dust deposition from diamond mining on subarctic plant communities and barren-ground caribou forage, J. Environ. Qual., 2021, 50(4), 990–1003 CrossRef CAS PubMed.
- X. Wang, J. C. Chow, S. D. Kohl, K. E. Percy, A. H. Legge and J. G. Watson, Characterization of PM 2.5 and PM 10 Fugitive Dust Source Profiles in the Athabasca Oil Sands Region, J. Air Waste Manage. Assoc., 2015, 65(12), 1421–1433 CrossRef CAS PubMed.
-
J. G. Watson, J. C. Chow, X. Wang, S. D. Kohl and L. N. R. Yatavelli, Windblown Fugitive Dust Characterization in the Athabasca Oil Sands Region, Wood Buffalo Environmental Association, Ft. McMurray, Alberta, Canada, 2014 Search PubMed.
- D. Wei, Y. Wang, C. Du, Y. Lin and B. Chang, Temporal-spatial distribution of vehicle transportation pavement dust migration in an open-pit mine, ACS Omega, 2020, 5(26), 16030–16036 CrossRef CAS PubMed.
- P. Pakbin, N. Hudda, K. L. Cheung, K. F. Moore and C. Sioutas, Spatial and temporal variability of coarse (PM10-2.5) particulate matter concentrations in the Los Angeles Area, Aerosol Sci. Technol., 2010, 44(7), 514–525 CrossRef CAS.
- V. Maus, S. Giljum, J. Gutschlhofer, D. M. da Silva, M. Probst, S. L. B. Gass, S. Luckeneder, M. Lieber and I. McCallum, A global-scale data set of mining areas, Sci. Data, 2020, 7, 289 CrossRef PubMed.
- E. N. Kelly, D. W. Schindler, P. V. Hodson, J. W. Short, R. Radmanovich and C. C. Nielsen, Oil Sands Development Contributes Elements Toxic at Low Concentrations to the Athabasca River and Its Tributaries, Proc. Natl. Acad. Sci. U. S. A., 2010, 107(37), 16178–16183 CrossRef CAS PubMed.
- C. Guéguen, C. W. Cuss and S. Cho, Snowpack deposition of trace elements in the Athabasca Oil Sands Region, Canada, Chemosphere, 2016, 153, 447–454 CrossRef PubMed.
- Y. Gopalapillai, J. L. Kirk, M. S. Landis, D. C. G. Muir, C. A. Cooke, A. Gleason, A. Ho, E. Kelly, D. Schindler, X. Wang and G. Lawson, Source Analysis of Pollutant Elements in Winter Air Deposition in the Athabasca Oil Sands Region: A Temporal and Spatial Study, ACS Earth Space Chem., 2019, 3(8), 1656–1668 CrossRef CAS.
- J. Zheng, W. Shotyk, M. Krachler and D. Fisher, 15
800 Years of Atmospheric Lead Deposition on Devon Ice Cap, Nunavut, Canada: Natural and Anthropogenic Enrichments, Isotopic Composition, and Predominant Sources, Global Biogeochem. Cycles, 2007, 21, GB2027 CrossRef.
- W. Shotyk, J. Zheng, M. Krachler, C. Zdanowicz, R. Koerner and D. Fisher, Predominance of Industrial Pb in Recent Snow (1994-2004) and Ice (1842-1996) from Devon Island, Arctic Canada, Geophys. Res. Lett., 2005, 32(21), 1–4 CrossRef.
- A. W. Struempler, Adsorption Characteristics of Silver, Lead, Cadmium, Zinc, and Nickel on Borosilicate Glass, Polyethylene, and Polypropylene Container Surfaces, Anal. Chem., 1973, 45(13), 2251–2254 CrossRef CAS.
- W. Dyck, Adsorption of silver on borosilicate glass, Anal. Chem., 1968, 40(2), 454–455 CrossRef CAS.
- R. A. Durst and B. T. Duhart, Ion-selective electrode study of trace silver ion adsorption on selected surfaces, Anal. Chem., 1970, 42(9), 1002–1004 CrossRef CAS.
- F. K. West, P. W. West and F. A. Iddings, Adsorption of Traces of Silver on Container Surfaces, Anal. Chem., 1966, 38(11), 1566–1570 CrossRef CAS.
- B. Bicalho, I. Grant-Weaver, C. Sinn, M. W. Donner, S. Woodland, G. Pearson, S. Larter, J. Duke and W. Shotyk, Determination of Ultratrace (<0.1 mg kg−1) Elements in Athabasca Bituminous Sands Mineral and Bitumen Fractions Using Inductively Coupled Plasma Sector Field Mass Spectrometry (ICP-SFMS), Fuel, 2017, 206, 248–257 CrossRef CAS.
- W. Shotyk, R. Belland, J. Duke, H. Kempter, M. Krachler, T. Noernberg, R. Pelletier, M. A. Vile, K. Wieder, C. Zaccone and S. Zhang,
Sphagnum mosses from 21 Ombrotrophic Bogs in the Athabasca Bituminous Sands Region Show No Significant Atmospheric Contamination of “Heavy Metals”, Environ. Sci. Technol., 2014, 48(21), 12603–12611 CrossRef CAS PubMed.
- W. Shotyk, P. G. Appleby, B. Beatriz, L. J. Davies, D. Froese, I. Grant-Weaver, G. Magnan, G. Mullan-Boudreau, T. Noernberg, R. Pelletier, B. Shannon, S. van Bellen and C. Zaccone, Peat bogs document decades of declining atmospheric contamination by trace metals in the Athabasca Bituminous Sands region, Environ. Sci. Technol., 2017a, 51(11), 6237–6249 Search PubMed.
- M. B. Javed, C. W. Cuss, I. Grant-Weaver and W. Shotyk, Size-Resolved Pb Distribution in the Athabasca River Shows Snowmelt in the Bituminous Sands Region an Insignificant Source of Dissolved Pb, Sci. Rep., 2017, 7, 43622 CrossRef PubMed.
- M. B. Javed, I. Grant-Weaver and W. Shotyk, An optimized HNO3 and HBF4 digestion method for multielemental soil and sediment analysis using inductively coupled plasma quadrupole mass spectrometry, Can. J. Soil Sci., 2020a, 100, 393–407 Search PubMed.
- M. B. Javed, G. Kachanoski and T. Siddique, A Modified Sequential Extraction Method for Arsenic Fractionation in Sediments, Anal. Chim. Acta, 2013, 787, 102–110 CrossRef CAS PubMed.
- M. B. Javed and W. Shotyk, Estimating Bioaccessibility of Trace Elements in Particles Suspended in the Athabasca River Using Sequential Extraction, Environ. Pollut., 2018, 240, 466–474 CrossRef CAS PubMed.
- W. Shotyk, Trace Elements in Wild Berries from Reclaimed Lands: Biomonitors of Contamination by Atmospheric Dust, Ecol. Indic., 2020, 105960 CrossRef CAS.
- W. Shotyk, T. Noernberg and M. B. Javed, Trace elements in Labrador Tea (Rhododendron groenlandicum): how the predominant sources of trace elements to the plants affect the chemical composition of hot water extracts, Environ. Res., 2020, 183, 109272 CrossRef CAS PubMed.
- C. W. Cuss, M. W. Donner, I. Grant-Weaver, T. Noernberg, R. Pelletier, R. N. Sinnatamby and W. Shotyk, Measuring the Distribution of trace elements amongst dissolved colloidal species as a fingerprint for the contribution of tributaries to large Boreal Rivers, Sci. Total Environ., 2018, 642, 1242–1251 CrossRef CAS PubMed.
- M. B. Javed, C. W. Cuss and W. Shotyk, Dissolved versus particulate forms of trace elements in the Athabasca River, upstream and downstream of bitumen mines and upgraders, Appl. Geochem., 2020b, 122, 104706 Search PubMed.
- W. Shotyk, B. Bicalho, C. W. Cuss, M. W. Donner, I. Grant-Weaver, S. Haas-Neill, M. B. Javed, M. Krachler, T. Noernberg, R. Pelletier and C. Zaccone, Trace Metals in the Dissolved Fraction (<0.45 μm) of the Lower Athabasca River: Analytical Challenges and Environmental Implications, Sci. Total Environ., 2017b, 580, 660–669 Search PubMed.
-
B. Hitchon and R. H. Filby, Trace Elements in Alberta Crude Oils, Alberta Research Council, Edmotnon, 1983 Search PubMed.
- Z. Dong, S. Kang, X. Qin, X. Li, D. Qin and J. Ren, New Insights into trace elements deposition in the snow packs at remote alpine glaciers in the northern tibetan plateau, China, Sci. Total Environ., 2015, 529, 101–113 CrossRef CAS PubMed.
- K. Kyllönen, V. Karlsson and T. Ruoho-Airola, Trace element deposition and trends during a ten year period in Finland, Sci. Total Environ., 2009, 407(7), 2260–2269 CrossRef PubMed.
- Z. Cong, S. Kang, Y. Zhang and X. Li, Atmospheric wet deposition of trace elements to Central Tibetan Plateau, Appl. Geochem., 2010, 25(9), 1415–1421 CrossRef CAS.
- J. Huang, S. Kang, Q. Zhang, J. Guo, P. Chen, G. Zhang and L. Tripathee, Atmospheric Deposition of Trace Elements Recorded in Snow from the Mt Nyainqêntanglha Region, Southern Tibetan Plateau, Chemosphere, 2013, 92(8), 871–881 CrossRef CAS PubMed.
- W. Shotyk, M. Krachler, W. Aeschbach-Hertig, S. Hillier and J. Zheng, Trace Elements in Recent Groundwater of an Artesian Flow System and Comparison with Snow: Enrichments, Depletions, and Chemical Evolution of the Water, J. Environ. Monit., 2010, 12(1), 208–217 RSC.
- K. Lee, S. D. Hur, S. Hou, S. Hong, X. Qin, J. Ren, Y. Liu, K. J. R. Rosman, C. Barbante and C. F. Boutron, Atmospheric Pollution for Trace Elements in the Remote High-Altitude Atmosphere in Central Asia as Recorded in Snow from Mt Qomolangma (Everest) of the Himalayas, Sci. Total Environ., 2008, 404(1), 171–181 CrossRef CAS PubMed.
- V. P. Shevchenko, O. S. Pokrovsky, S. N. Vorobyev, I. V. Krickov, R. M. Manasypov, N. V. Politova, S. G. Kopysov, O. M. Dara, Y. Auda, L. S. Shirokova, L. G. Kolesnichenko, V. A. Zemtsov and S. N. Kirpotin, Impact of snow deposition on major and trace element concentrations and elementary fluxes in surface waters of the western Siberian Lowland across a 1700’km Latitudinal Gradient, Hydrol. Earth Syst. Sci., 2017, 21, 5725–5746 CrossRef CAS.
-
R. L. Rudnick and S. Gao, Composition of the continental crust, ed. Holland, H., Turekian, K., in, Treatise on Geochemistry, 2014, Elsevier, pp. 1–64 Search PubMed.
-
J. E. Welton, SEM Petrology Atlas, The American Association of Petroleum Geologists, Tulsa, OK, 2003 Search PubMed.
-
J. A. Bichard, Oil Sands Composition and Behaviour Research: the Research Papers of John A. Bichard, 1957–1965, Alberta Oil Sands Technology and Research Authority, 1987 Search PubMed.
- G. Mullan-Boudreau, R. Belland, K. Devito, T. Noernberg, R. Pelletier and W. Shotyk, Sphagnum Moss as an Indicator of Contemporary Rates of Atmospheric Dust Deposition in the Athabasca Bituminous Sands Region, Environ. Sci. Technol., 2017, 51(13), 7422–7431 CrossRef CAS PubMed.
- M. A. Bari, W. B. Kindzierski and S. Cho, A Wintertime investigation of atmospheric deposition of metals and polycyclic aromatic hydrocarbons in the Athabasca Oil Sands Region, Canada, Sci. Total Environ., 2014, 485–486, 180–192 CrossRef CAS PubMed.
- F. Barraza, G. Uzu, J. L. Jaffrezo, E. Schreck, H. Budzinski, K. Le Menach, M. H. Dévier, H. Guyard, A. Calas, M. I. Perez, L. A. Villacreces and L. Maurice, Contrasts in chemical composition and oxidative potential in PM10 near flares in oil extraction and refining areas in Ecuador, Atmos. Environ., 2020, 223, 117302 CrossRef CAS.
-
K. P. Timoney, The Peace-Athabasca Delta: Portrait of a Dynamic Ecosystem, The University of Alberta Press, 2013 Search PubMed.
Footnote |
† Electronic supplementary information (ESI) available. See DOI: 10.1039/d1ea00034a |
|
This journal is © The Royal Society of Chemistry 2022 |
Click here to see how this site uses Cookies. View our privacy policy here.