DOI:
10.1039/D1SC04139H
(Edge Article)
Chem. Sci., 2022,
13, 763-774
Tunable heteroaromatic azoline thioethers (HATs) for cysteine profiling†
Received
28th July 2021
, Accepted 11th December 2021
First published on 13th December 2021
Abstract
Here we report a new series of hydrolytically stable chemotype heteroaromatic azoline thioethers (HATs) to achieve highly selective, rapid, and efficient covalent labeling of cysteine under physiological conditions. Although the resulting cysteine–azoline conjugate is stable, we highlight traceless decoupling of the conjugate to afford unmodified starting components in response to reducing conditions. We demonstrated that HAT probes reverse the reactivity of nucleophilic cysteine to electrophilic dehydroalanine (Dha) under mild basic conditions. We demonstrated the umpolung capability of HAT probes for the modification of cysteine on peptides and proteins with various nucleophiles. We demonstrated that HAT probes increase the mass sensitivity of the modified peptides and proteins by 100 fold as compared to the classical methods. Finally, we extended the application of HAT probes for specific modification of cysteines in a complex cell lysate mixture.
Introduction
Chemical modification of proteins by small molecules in a selective manner is a powerful approach for drug design, imaging, chemoproteomic studies and activity based protein profiling (ABPP).1–7 The key feature of the bioconjugation approach is the requirement of mild reaction conditions and selectivity for one particular amino acid in a plethora of all other reactive amino acids. Cysteine is the amino acid of choice for these modifications because of its low abundance (1.7%), high nucleophilicity of the side chain and important roles it plays in various biological processes including catalysis.8,9 Various electrophiles have been reported for tagging cysteines such as haloacetamides (IAA),10 epoxides,11 sulfonate esters,12 chloro- and acyloxymethyl ketones,13–15 fluorobenzene,16 aryl halides,17 Michael acceptors18,19 and heteroaromatic sulfones (Fig. 1a).20–23 However, poor hydrolytic stability of probes or resulting conjugates, low reactivity and cross-reactivity with other amino acids24–26 lead to the development of several metal-free and transition metal-mediated cysteine bioconjugation approaches by Davis, Bernardes, Pentelute, Wong, and others.25–43
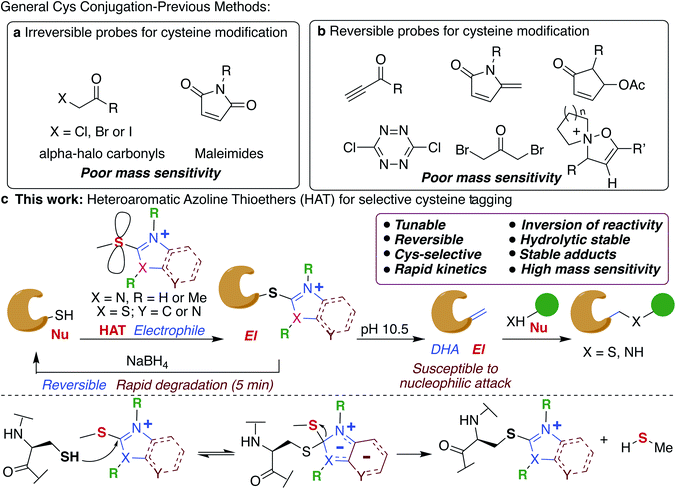 |
| Fig. 1 Reagents for selective tagging of cysteine. (a) Classical reagents for cysteine modification. (b) Reagents for cleavable cysteine modification. (c) This work: a general strategy for cysteine modification using HAT probes, cleavable under reduced conditions, umpolung capability under basic conditions and increasing the mass sensitivity of the labeled fragments. Possible mechanism of cysteine modification with HAT probes by SNAr substitution to generate stable adducts. | |
Bioconjugation reactions with cleavable linkers have recently gained considerable attention due to their wide application in many research fields such as protein immobilization, drug development,44,45 and proteomics.46,47 Only a few compounds are available for cleavable cysteine-specific modification, including Ellman's reagent,48,49 bromomaleimides,50 bromo-pyridazinediones,51 electron deficient acetylenes,52 5-methylene pyrrolones,53 4-substituted cyclopentenones54 and recently discovered isoxazoliniums (Fig. 1b).55 Despite all these advances, only ∼17% of cysteine in the entire proteome has been identified so far.56,57 Moreover, the resulting bioconjugates exhibit poor mass detection sensitivity limiting their applications in the identification of low abundant cysteine in the proteome. Therefore, there is a great need to develop new highly efficient cysteine selective modification methods with cleavable linkers using easily accessible reagents with high stability, distinct and tunable selectivity and reactivity that increases the mass detection sensitivity of the resulting conjugates that would aid in the identification of low abundant cysteines in the proteome.
In this study, we elucidate specific structural features of a new chemotype termed heteroaromatic azoline thioether (HAT) that is easily tunable, hydrolytically stable, and highly reactive and selective for Cys, and increases the mass detection sensitivity of resulting conjugates (Fig. 1c). We elucidate that HAT, a largely unexplored cysteine-reactive electrophilic group undergoes chemically triggered decoupling to generate the native unchanged coupling partners in a “traceless” manner under mild conditions (Fig. 1c). Another unique feature of HAT probes is their ability to reverse the reactivity of nucleophilic Cys into an electrophilic moiety (Fig. 1c). This inversion of reactivity enables selective modification of proteins with a variety of nucleophiles. These innovative HAT tools for probing cysteine would augment existing detection methods and will dramatically expand the toolbox for bioconjugation, proteome profiling, for targeting otherwise undruggable protein targets and identifying protein partners.
Results and discussion
Design of HAT probes
Thioethers are privileged scaffolds found in various FDA approved drugs and are the third most exemplified constituent (8.8%) of sulfur containing drugs including representative pharmaceuticals with heteroaromatic units such as cimetidine, imuran, ranitidine, azathioprine, butoconazole and many others.58 Some of the heteroaromatic thioethers such as 2-(thioalkyl)benzoxazole act as prodrugs and undergo S-oxidation during metabolism forming active sulfone drugs.59
Although thioethers are hydrolytically stable as compared to their oxidized sulfinyl and sulfone analogs, they have never been explored for protein modification due to their intrinsic low reactivity. Here we display systematic efforts to tune the reactivity and selectivity of thioethers thus expanding their utility for the selective modification of cysteine in proteins as a reversible cleavable linker and chemoproteomic profiling.
We tune the thioether probes to find a sweet spot between high reactivity, and high hydrolytic stability and selectivity for Cys. We achieved this by (i) exploring different heteroatoms on azoline-thioethers, (ii) changing the heterocyclic ring to azole, (iii) by fusing aromatic moieties to azoline-thioethers to generate heteroaromatic azoline-thioether (HAT) probes, (iv) varying the S-oxidation state, and (v) by evaluating different methylation states of azoline-thioethers and heteroaromatic azoline-thioether (HAT) probes (Fig. 2a and b). Despite various developments in the field of Cys modification, iodoacetamide (IAA) is still commonly used for the modification and profiling of Cys.60 This is mainly due to its high hydrolytic stability and easy synthesis of IAA derivatives although it exhibits slow reactivity and cross-reactivity with lysine. Throughout the manuscript, we will compare our optimized HAT probes with well-known IAA and oxidized sulfone analogs in terms of selectivity, reactivity, stability, ionization potential, and reversibility.
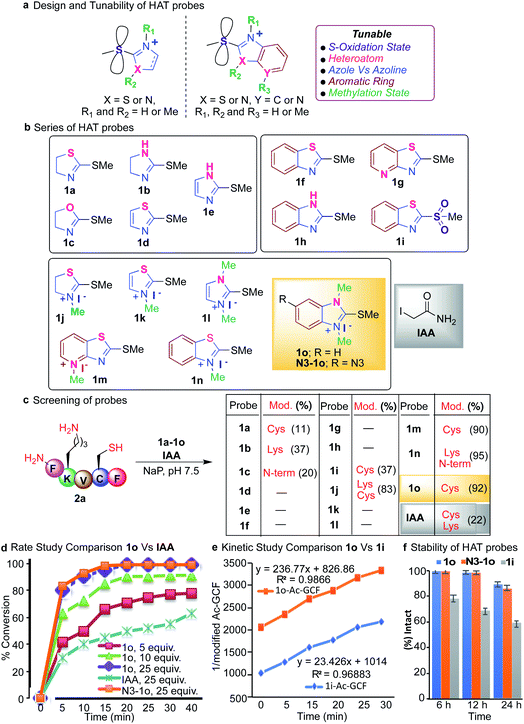 |
| Fig. 2 Tunable and stable HAT probes for targeting cysteine. (a) Design of hydrolytically stable thioether probes to tune their reactivity and selectivity for modification of cysteine. (b) Structures of a variety of HAT probes with different modifications to investigate their reactivity and selectivity for cysteine. (c) Screening of probes (40 mM, 10 equiv.) by using peptide FKVCF (4 mM, 1 equiv.) with all the nucleophilic residues and comparison with well known IAA and sulfone probe (1i) (40 mM) under physiological conditions (NaP (10 mM), pH 7.5, 25 °C) for 3 h. Probe 1o showed high selectivity and reactivity for cysteine as compared to sulfone probe 1i and IAA. (d) Observed rate of the modification of cysteine of the peptide Ac-GCF 2b (0.003 mM) with 1o (5–25 equiv.), its azide analog N3-1o (0.6 mM, 25 equiv.) and IAA (0.6 mM, 25 equiv.) in 10 mM phosphate buffer (pH 7.5, 25 °C) at different time intervals. (e) Kinetics study comparison of probe 1o (0.973 mM) and 1i for labelling peptide Ac-GCF 2b (0.973 mM) showed a 10 fold faster rate of probe 1o for cysteine modification as compared to sulfone analog 1i (0.973 mM) under physiological conditions (NaP (10 mM), pH 7.5, 25 °C). In both (d) and (e), each time point represents an average of three independent experiments. (f) High stability of probe 1o (38.75 mM) and its azide analog N3-1o (38.75 mM) as compared to sulfone probe 1i (38.75 mM) under physiological conditions (NaP (10 mM), pH 7.5, 25 °C). | |
Heteroatom core optimization
We started our initial investigation by reacting a peptide FKVCF (4 mM, 1 equiv.) containing multiple nucleophilic residues such as N-terminus, Lys and Cys with azoline-thioether probes 1 (40 mM, 10 equiv.) with various heteroatoms in the azoline ring (Synthesis of probes, ESI Fig. 1†). The reaction was conducted in phosphate buffer (NaP, pH 7.5, 10 mM) at room temperature under air for 3 h. Remarkably, the reaction with 2-methylthio thiazoline 1a labeled only the Cys residue without any modification of the N-terminus and lysine side chain due to the low electrophilicity of 1a.61 2-methylthio imidazoline 1b labeled Lys, due to the ability of the imidazole of 1b to act as a base and deprotonate the lysine side chain. 2-Methylthio oxazoline 1c labeled the N-terminus of peptide FKVCF because the pKa of the side chain of lysine ∼10.6 is higher than the pKa of the N-terminus ∼9.6 and therefore, the side chain of lysine remained protonated under the physiological conditions and labeling of lysine was not observed as determined by MS/MS (Fig. 2c and ESI Fig. 2†).61 The study showed that heteroatoms play a very important role in tuning the reactivity of azoline-thioether probes towards various nucleophiles. Replacing the sulfur atom in azoline with an oxygen or nitrogen atom completely switches the chemoselectivity as reported in our previous study.61 Conversely, substituting the heterocyclic ring azole for azoline, 2-methylthio thiazole 1d and 2-methylthio imidazole 1e eliminated the reactivity for Cys (Fig. 2c). These studies clearly showed that modulation of the heterocycle immensely influenced the selectivity and reactivity of thioethers towards various nucleophiles.
To assess the potential to tune the reactivity towards cysteine, we evaluated the reactivity and chemoselectivity of 2-methylthio benzothiazoline 1f, 2-methylthio pyridthioazoline 1g and 2-methylthio benzoimidazoline 1h where a benzene or pyridine ring is directly fused to the heterocycle thiazoline and imidazoline of thioethers (Fig. 2b, Synthesis of probes, ESI Fig. 1†). The fusion of aromatic rings drastically reduces the solubility of the azoline-thioether probes in aqueous buffer conditions and thus no reactivity was observed with peptide FKVCF in phosphate buffer at pH 7.5 (Fig. 2c). We next examined the influence of different oxidation states of sulfur on 2-methylthio benzothiazoline and the resulting sulfone analog 1i enhanced aqueous solubility and imparted Cys reactivity as confirmed by the reaction with peptide FKVCF (4 mM) followed by MS/MS analysis (37% conversion, 1i probe (40 mM, 10 equiv.) in 3 h, Fig. 2c, ESI Fig. 2†).62 However, reactive sulfones are also susceptible to degradation in aqueous solution.59
Methylation of heteroatom-core optimization
One common way to tune the reactivity of electrophilic probes is by substituting aromatic rings with electron withdrawing or electron releasing groups. The reactivity of electrophilic probes varies tremendously by the attachment of different electron withdrawing substituents on the aryl ring, with the nitro group showing very high reactivity and the fluorine group with modest reactivity as shown in a previous study.20 Therefore, probe derivatives such as affinity tags (alkynes or azides, biotin) or dye-derivatives that are obtained by the substitution at the aromatic ring showed a huge disparity in their reactivities as compared to the model probes used for the optimization studies. In fact, affinity tag-derived probes showed significantly reduced reactivity as compared to the highly reactive nitro analogs utilized for model studies.20 We hypothesized that by tuning the reactivity of the core structure of the probe, one can obtain probes with predictable reactivity and selectivity independent of the aryl or alkyl substitutions. Therefore, we carried out methylations of the heteroatoms of the core structure of heteroaromatic azoline-thioether (HAT) probes to tune their reactivity and selectivity (Fig. 2b, Synthesis of probes, ESI Fig. 1†). The methylation of HAT probes also increases their solubility in an aqueous solution. To obtain the right balance between the high reactivity of the HAT probes, their hydrolytic stability, and selectivity, a small series of thioether probes were synthesized with various methylation states (1j–1o) (Fig. 2b). Next, we screened the probes 1j–1o (10 equiv., 40 mM) with peptide FKVCF (4 mM) under physiological conditions (NaP, pH 7.5, 10 mM). Probe 1j obtained from the N-methylation of 1a modified both Cys and Lys due to the increased electrophilicity as confirmed by MS/MS (Fig. 2c and ESI Fig. 3†). Probe 1n showed modification of Lys and N-terminus because the conjugate obtained by reaction with cysteine is reversible and either reacts with lysine and N-terminus to generate a stable product or with the released methanethiol to generate the unreacted starting material63 (Fig. 2c and ESI Fig. 3†).
Interestingly both 1m and 1o showed high reactivity and selectivity for Cys (90–92% conversion, Fig. 2c and ESI Fig. 4†). Importantly, a comparison experiment performed with IAA under identical reaction conditions, showed modification of both Lys and Cys residues of peptide FKVCF (22% conversion) demonstrating the poor selectivity of IAA towards Cys (Fig. 2c and ESI Fig. 5†). These studies showed that methylations of heteroatoms increase aqueous solubility and induce huge variations in the HAT reactivity, and thus are capable of inducing selectivity for a particular target by rational tuning and provide a simple strategy to tune the core structure across the aromatic heterocyclic scaffold. Probe 1o has previously been reported for the synthesis of carbodicarbenes but has never been explored for selective peptide and protein modification.64 The optimization studies revealed that the reaction between peptide Ac-GCF (3.75 mM) and HAT probe 1o (25 equiv., 93.75 mM) selectively labels Cys and proceeds most efficiently in phosphate buffer (NaP, pH 7.5, 10 mM) at room temperature, resulting in the formation of a stable coupling product with >99% conversion in 3 h (ESI Fig. 6†).
Under optimized conditions, IAA generated 70% conjugated product and sulfone analog 1i generated 53% conjugated product (ESI Fig. 6†). To characterize the 1o-Cys coupling product, reaction with a model compound 2-(Boc-amino)ethanethiol was carried out on a large scale under the optimized conditions. The resulting product was isolated and cysteine labeling by 1o was confirmed by NMR (1H and 13C) (ESI Fig. 7†). In contrast, no product was observed with peptide GAF-OMe (w/o Cys), thus reconfirming the high chemoselectivity of HAT probe 1o for Cys (ESI Fig. 8†).
Rate study of labeling cysteine with the HAT probe
Next, we monitored the reaction between peptide Ac-GCF 2b (3 mM) and HAT probe 1o (5–25 equiv.) after regular intervals of time and compared it to the corresponding reaction with IAA (25 equiv. 0.6 mM) under optimized conditions (NaP, pH 7.5, 10 mM) (Fig. 2d and ESI Fig. 9†). The formation of the coupling product was analyzed using HPLC and MS. The reaction proceeded with fast kinetics and more than 80% conversion to the cysteine modification product was observed in 5 min using 25 equiv. of the 1o probe (Fig. 2d and ESI Fig. 9†). The comparison experiment with IAA (25 equiv.) showed a decreased product formation (30%) in 5 min. Finally, we carried out the rate studies with the azide-derivative of 1o, (N3-1o) to determine the role of the substituent in modulating the rate of the reaction. The reaction with N3-1o (25 equiv.) showed a comparable reaction rate to 1o (Fig. 2d and ESI Fig. 9†). The similar reactivity profile of 1o and N3-1o reaffirms our initial hypothesis regarding the modulation of the core structure to obtain more predictable reactivity with various probe-derivatives. Next, the bioorthogonal reaction of N3-1o modified peptide, N3-1o-AcGCF 2b with sulfo-DBCO-Biotin using strain promoted alkyne–azide cycloaddition (SPAAC) under physiological conditions (Nap, pH 7.5, 10 mM) generated the conjugated product with full conversion as analyzed by ESI-MS (ESI Fig. 9†). This study showed the compatibility of the HAT–Cys coupling product with SPAAC thus applicable for proteome profiling and enrichment.
Next, we compared kinetics of the reaction of HAT probe 1o (0.973 mM) and its oxidized sulfone analog 1i (0.973 mM) with peptide AcGCF 2b (0.973 mM) in buffer (NaP, pH 7.5, 10 mM) at room temperature. The results showed that 1o (k = 236.77 M−1 S−1) is 10 times more reactive than 1i (k = 23.43 M−1 S−1) (Fig. 2e and ESI Fig. 10†).
Stability of HAT probes
It is usually considered that highly reactive probes exhibit poor hydrolytic stability. Therefore, we sought to determine the stability of the HAT probes 1o and N3-1o and compared them with sulfone analog 1i. These probes (38.75 mM) were incubated in aqueous phosphate buffer (pH 7.5) under ambient conditions and monitored after regular intervals of time by HPLC. Surprisingly, more reactive probes 1o and N3-1o are highly stable for 6 h without any observed degradation and the less reactive 1i probe showed 24% degradation in 6 h. Only 14–17% hydrolysis of probes 1o and N3-1o was observed in 24 h as compared to 1i that showed 40% hydrolysis in 24 h (Fig. 2f and ESI Fig. 11†). These studies showed that HAT probes 1o and N3-1o exhibit high reactivity and high selectivity towards cysteine and are hydrolytically more stable as compared to 1i.65,66 The HAT probe 1o is bench stable for 3 months as a white solid, demonstrating robust properties for long-term storage.
Protein modification with HAT
Previous studies with carbon electrophiles such as chloroacetamide and sulfonate esters showed that the solution reactivity of electrophiles with peptides is often not predictive of reactivity observed with proteins due to the unique protein environment, which modulates the pKa and reactivity of amino-acid side chains. To evaluate the selectivity and reactivity of HAT probes towards Cys with proteins, we carried out the reaction of all the HAT probes (1a–1o) with myoglobin (Mb) (w/o Cys). The modification of Mb was observed with all the reactive probes (1a–1c, 1j, 1n, and IAA) except 1i, 1m and 1o thus clearly showing their high selectivity for Cys since Mb does not have the Cys residue thus corroborating with peptide screening data (Fig. 3a and ESI Fig. 12†). Most of the other HAT probes reacted with other nucleophilic amino acids of Mb such as Lys (ESI Fig. 13†). Probes 1d–1h and 1k–1l do not show any reactivity with Mb due to their low reactivity and less water solubility similar to peptide screening data (Fig. 3a). To determine the reactivity and selectivity of 1o and 1m for proteins, we carried out the reaction with both native insulin (w/o free Cys) and reduced insulin containing six free cysteine residues (two in chain A and four in chain B, ESI Fig. 14†). We observed full modification of all the cysteine residues in both chains A and B of reduced insulin by 1o (>99% conversion) as compared to 1m (chain A – 55% and chain B – 40%) under identical conditions (50 equiv. 1m, 12 h, pH 7.5) as analyzed by MS (ESI Fig. 14†). The modification of native insulin was not observed with both 1o and 1m thus confirming high selectivity of 1o and 1m for Cys (ESI Fig. 14†).
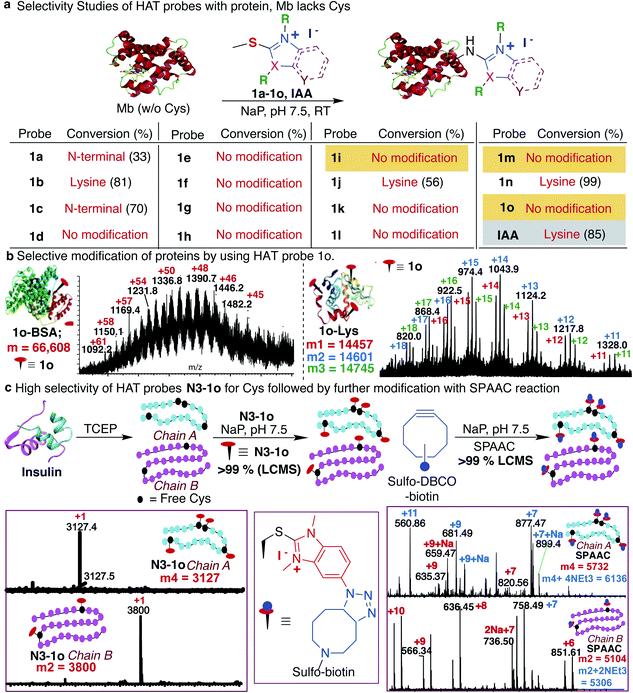 |
| Fig. 3 HAT probes for protein functionalization. (a) Conjugation of myoglobin (Mb) with various HAT probes. Mb does not have any cysteine residue and probe 1o showed no reactivity with Mb thus confirming high selectivity for cysteine. However, widely used cysteine selective probe IAA modifies the Mb. (b) Selective modification of cysteine in a protein BSA with one free cysteine and reduced lysozyme (1–3 cysteine modifications observed). (c) Modification of native and reduced insulin (0.15 mM) with HAT probe N3-1o (7.5 mM). Modification of all six free cysteines in reduced insulin and no reaction with native insulin (w/o free cysteine, disulfide form), confirms high selectivity of N3-1o towards cysteine. The strain promoted alkyne–azide cycloaddition (SPAAC) with sulfo-DBCO-Biotin further functionalized N3-1o-modified insulin chains. Reaction conditions: N3-1o-modified insulin protein (0.15 mM), sulfo-DBCO-Biotin (1.5 mM) under physiological conditions (NaP pH 7.5, 25 °C) for 4 h. | |
Next, we studied and compared the selectivity of IAA for Cys using myoglobin (Mb). Under physiological conditions (NaP, pH 7.5, 10 mM), the reaction of Mb with IAA (100 equiv.) generated the Mb-conjugate with multiple modifications (Fig. 3a and ESI Fig. 15†). This showed that IAA is not highly selective for Cys and leads to the modification of other reactive nucleophiles on Mb including Lys, and N-terminus, thus reconfirming literature reports.67–69 These studies showed that HAT probe 1o is more reactive and highly selective for cysteine conjugation as compared to widely used IAA and all other HAT probes.
After a comprehensive study on the efficiency, chemoselectivity, scope, and stability of HAT probe 1o-mediated cysteine modification, we further explored its applicability for other protein bioconjugation. Bovine serum albumin (BSA) with a single free cysteine residue was utilized for bioconjugation. Treatment of BSA (0.15 mM) with HAT probe 1o (300 equiv.) in Nap 7.5 buffer at 25 °C for 8 h afforded modified protein BSA-1o in >99% conversion by LC-MS analysis (Fig. 3b and ESI Fig. 16†). For oxidized-cysteine-containing proteins, lysozyme, under the same reaction conditions, no modification was found. Reduction of lysozyme generated 8 free cysteines and reaction with 1o modified 1–3 cysteine residues in reduced lysozyme (Nap 7.5 buffer at 25 °C for 8 h, >99% conversion) (Fig. 3b and ESI Fig. 16†). The high reactivity of the probe towards particular cysteines in lysozyme is due to easy surface accessibility and the microenvironment influencing the pKa of cysteines making it more reactive. These results indicated that the HAT probe-mediated modification could be conducted with high efficiency and chemoselectivity on proteins.
Synthesis of HAT affinity tags for Cys bioconjugation
The effective bioconjugation reaction for enrichment should have the ability to attach affinity tags, thus we generated the azide functionalized HAT probe N3-1o (Synthesis of probes, ESI Fig. 1†). We then evaluated the reactivity of the azide-functionalized HAT probe N3-1o towards Mb, native insulin and reduced insulin (Fig. 3c and ESI Fig. 17†). Similar to the model probe studies with 1o, azide-HAT probe N3-1o did not modify Mb and native insulin but modified all the cysteine residues on both chains A and B of reduced insulin under optimized conditions thus showing high selectivity for Cys as confirmed by LCMS and HPLC (>99%, Fig. 3c and ESI Fig. 17†). The strain promoted alkyne–azide cycloaddition (SPAAC) with sulfo-DBCO-Biotin further functionalizes N3-1o-modified insulin chains. The reaction products were analyzed by MS and the data showed dual labeling of all the free Cys residues on reduced insulin with full conversion (>99%, Fig. 3c and ESI Fig. 17†). This study also showed the compatibility of the HAT–Cys coupling product with SPAAC thus applicable for proteome profiling and enrichment.70
Selective tagging of Cys in a complex mixture
As a further demonstration of the high selectivity of HAT probe 1o for Cys, we attempted tagging multiple proteolytic fragments in the same solution to test the potential of our method for enrichment in a complex mixture. The mixture of proteolytic fragments obtained by CNBr cleavage of Mb, Cytochrome C and reduced insulin was incubated with HAT probe 1o for 12 h under the optimized reaction conditions. The reaction was analyzed by LCMS and the data showed the tagging of only free Cys containing proteolytic fragments with 1o in the reaction mixture (ESI Fig. 18†).
Reversibility and stability of the HAT–cysteine conjugation
Next, we determined the stability of the 1o-conjugated peptide 1o-Ac-GCF 3b under different reaction conditions by monitoring with HPLC. We demonstrated that peptide conjugate 1o-Ac-GCF 3b is stable at low pH 3.5 for 48 h at both room temperature 25 °C and 40 °C and only 10% degradation was observed in 24 h at pH 7.5 (ESI Fig. 19†). Peptide conjugate 1o-Ac-GCF 3b is also stable to a strong protein disulfide reducing agent tris(2-carboxyethyl)phosphine (TCEP) for 48 h without any decomposition (ESI Fig. 19†). Even though the HAT–peptide conjugate is stable under physiologically relevant conditions, we aimed to explore if the conjugate can be decoupled in a traceless manner to avoid any limitations associated with the irreversible inhibition of proteins. One way to achieve this goal is to invert the reactivity of the nucleophilic Cys residue into electrophilic DHA, thus allowing reversibility by the attack of a nucleophile on the unchanged starting material. Indeed, exposure of the peptide conjugate 1o-Ac-GCF 3b to reduction conditions in the presence of sodium borohydride (10 equiv., 25 °C, in NaP pH 7.5) led to its rapid degradation to unchanged peptide Ac-GCF 2b (>99% conversion) in 5 min, as observed by HPLC and MS analysis (ESI Fig. 20†). Next, we applied the reversibility approach for insulin modified with 1o; we subjected 1o-modified insulin chains A and B to reducing conditions and within 5 min observed the complete reversibility to unmodified insulin chains A and B with full conversion (>99% Fig. 4a and ESI Fig. 20†). It is noteworthy that the reduction generated original protein in an unperturbed manner. The high selectivity and reactivity of the HAT probes for Cys under physiological conditions, and the ability to undergo rapid reversal in a traceless manner to regenerate unmodified protein, underscore the unique advantages of the HAT probes in bioconjugation and proteome profiling, thus also minimizing the limitations associated with producing irreversibly modified proteins.
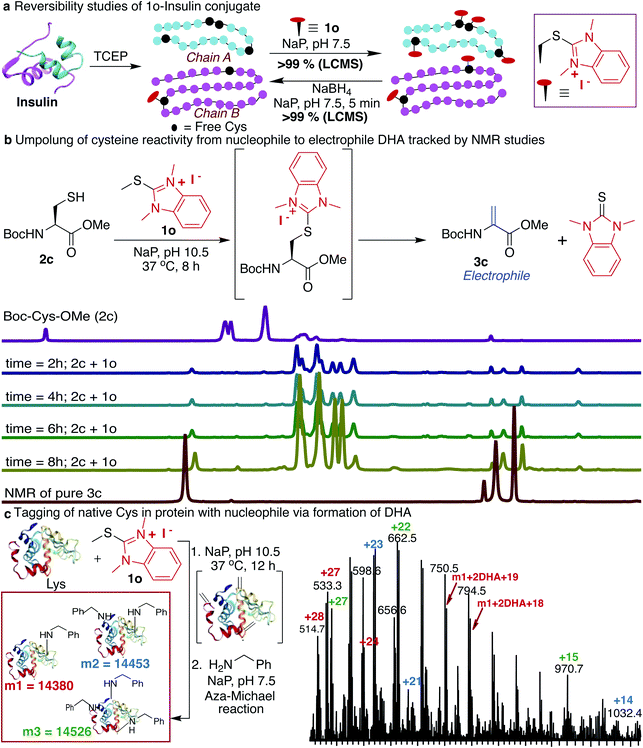 |
| Fig. 4 Reversibility and inversion of the reactivity of cysteine by HAT probe 1o. (a) Reversibility of the 1o-protein conjugate (0.15 mM) in the presence of sodium borohydride (1.5 mM) in NaP (pH 7.5) in 5 min. (b) Conversion of peptide Boc-Cys-OMe 2c directly to dehydroalanine DHA 3c under basic conditions (NaP, pH 10.5, 8 h) with >99% conversion. 1H NMR shows the formation of dehydroalanine (DHA) 3c from the HAT–Cys conjugate in a one step process. (c) Conversion of free cysteine of protein lysozyme (0.15 mM) to DHA (conversion >99%) by incubating with probe 1o (45 mM) at pH 10.5 for 12 h followed by the addition of amine (7.5 mM) by the aza-Michael reaction on DHA at pH 8.5 for 12 h to generate amine labeled protein (conversion 78%). | |
Reactivity inversion by HAT probes: reaction with nucleophiles
Many protein conjugation methods utilize the inherent nucleophilicity of Cys and carry out reactions with electrophiles. A different approach for modification at cysteine is obtained by inverting the reactivity of nucleophilic cysteine residue into electrophilic DHA, thus allowing for modification by various nucleophiles. This approach could also be applicable to capture the protein interaction partners by the formation of the covalent bond between the DHA on a peptide or protein and lysine of the interacting protein partner. We sought to achieve this goal by exposing Boc-Cys-OMe 2c to basic conditions (NaP, pH 10.5, 37 °C) and observed the formation of dehydroalanine Dha 3c directly via the 1o-Cys modified intermediate as observed by NMR and LCMS (86%, 8 h, Fig. 4b, and ESI Fig. 21†). The spontaneous elimination of the 1o-Cys conjugate under basic conditions generates a type 2 alkene, dehydroalanine Dha, which in principle serves as a handle for further conjugation with various cargoes such as polyethylene glycol (PEG) polymers, fluorophores, or affinity reagents by reaction with nucleophilic amines and thiols.71–77 By using HAT probes we converted nucleophilic Cys of a peptide Ac-GCF 2b to Ac-G(Dha)F 3d (88% conversion) followed by reactions with a variety of nucleophiles such as mercaptoethanol and benzylamine to generate thiol addition product 3e and conjugated amine 3f with >99% conversion (ESI Fig. 22†). Next, we converted the nucleophilic Cys on reduced protein lysozyme to DHA by treatment with probe 1o at pH 10.5. We observed modification of three cysteines of the reduced lysozyme to DHA with >99% conversion. Next, we carried out labeling of DHA modified lysozyme with benzylamine by the aza-Michael reaction to generate amine-modified lysozyme with 78% conversion as analyzed by LCMS (Fig. 4c and ESI Fig. 23†).
HAT as mass sensitivity booster
One of the limitations with current methods of selective labeling of Cys is the difficulty in the characterization of resulting bioconjugates by MS due to the poor ionization of the labeled fragments.76 This limitation is more prominent in complex mixtures leading to a major roadblock to the discovery of low abundant protein biomarkers for detection of early stage infections due to their poor mass sensitivity. Therefore methods for enhancing the detection sensitivity of labeled fragments are in critical demand.60 To determine the ionization efficiency and mass sensitivity enhancement capability of HAT probe 1o in comparison with free peptide, sulfone probe 1i and the IAA probe, we carried out MS of the mixture of 1o-labeled peptide Ac-GCF (5 μM) and 1i-labeled peptide Ac-GCF (5 μM), 1o labeled peptide Ac-GCF (5 μM) and IAA-labeled peptide Ac-GCF (5 μM) and 1o labeled peptide Ac-GCF (5 μM) and unlabeled peptide Ac-GCF (5 μM) in water in equal concentrations. The 1o tag on a peptide Ac-GCF delivered the most significant signal enhancement (1o-Ac-GCF
:
1i-Ac-GCF, 100
:
5; 1o-Ac-GCF
:
IAA-Ac-GCF, 100
:
8; and 1o-Ac-GCF
:
Ac-GCF, 100
:
1.8) (ESI Fig. 24†). Next, a concentration assay confirmed that the tagged peptide 1o-Ac-GCF is detected up to 0.5 nanomolar concentration (ESI Fig. 24†).
HAT probe N3-1o also increased the detection sensitivity of the labeled protein fragments of the reduced insulin tremendously as compared to IAA as determined by MS without any purification (Fig. 5a and ESI Fig. 25†). For unlabeled and IAA-labeled reduced insulin fragments, very poor sensitivity was observed for both chains A and B of insulin. In fact chain A was undetectable by MS. IAA labelled only one free cysteine of chain B and N3-1o labeled both the free cysteines of chain B and all four free cysteines of chain A (Fig. 5a and ESI Fig. 25†). The HAT N3-1o-labeled fragments of reduced insulin showed significantly high mass intensities of both chains A and B. This also leads to the easy detection of chain A in MS that is otherwise undetectable and thus could be of high significance in proteomics studies.
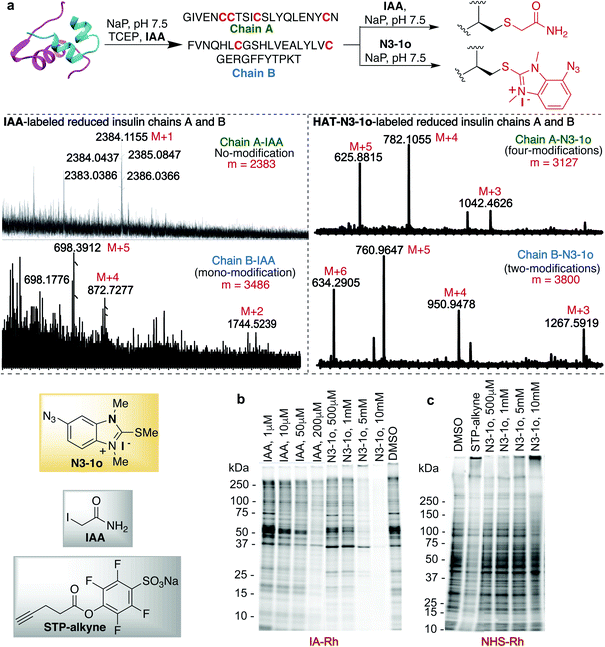 |
| Fig. 5 HAT probe 1o as mass sensitivity booster. (a) HAT probe 1o significantly increases the mass detection sensitivity of a protein as compared to IAA tagged protein. Tagging with HAT probe N3-1o improves the detection of chain A of reduced insulin significantly (right MS trace). Chain A is not modified after TCEP reduction and labeling with IAA (left MS trace). Both chains A and B of reduced insulin are visible after tagging with the HAT probe, N3-1o (right MS trace). 1o modified all 4 cysteines and 2 cysteines of chains A and B respectively. IAA modified only one cysteine of chain B under identical conditions. Reaction conditions: reduced insulin (0.15 mM), probes 1o or IAA (50 equiv.) in NaP buffer at pH 7.5, room temperature for 8 h. (b) Chemoproteomic studies of the HAT N3-1o probe by gel-based competitive activity-based protein profiling (ABPP). In-gel fluorescence analysis of the HAT N3-1o probe at different concentrations (500 μM to 10 mM) and comparison with the cysteine reactive IAA probe (1–200 μM) followed by incubation and detection with IA-Rh in HEK293T cell lysate. Fading of bands with an increase in the N3-1o concentration showed labeling with cysteine. (c) In-gel fluorescence analysis of the HAT N3-1o probe at different concentrations (500 μM to 10 mM) and comparison with the lysine reactive STP-alkyne probe (1 mM) followed by incubation and detection with NHS-Rh. No fading of bands with increasing concentration of N3-1o indicates no reactivity with lysine. | |
HAT probes for gel-based ABPP studies
Finally, we turned our attention to the use of the HAT N3-1o reagent for activity-based protein profiling (ABPP) applications, owing to its high specificity and reactivity toward cysteine, as well as the small size of the HAT group that allows access to a broad range of proteins. To assess the proteome reactivity of this class of electrophiles, we evaluated the HAT probe N3-1o by gel-based competitive ABPP with NHS-tetramethylrhodamine (NHS-Rh) and iodoacetamide-tetramethylrhodamine (IA-Rh) using HEK293T cell lysate (Fig. 5b and c and ESI Fig. 26†). Blockade of IA-Rho labeling by pre-treatment with high concentrations of N3-1o, as indicated by the decreased in-gel fluorescence signal, is consistent with cysteine-labeling by N3-1o (Fig. 5b). The kinetic study of probes showed that 1o and N3-1o are significantly more reactive than IAA (Fig. 2d), yet in the gel analysis experiment high amounts of N3-1o are required to achieve a similar labeling to IAA. This is because of the poor stability of the N3-1o cysteine conjugate products in the gel-based competitive ABPP experiments that required heating at 37 °C and 95 °C (Fig. 5b and ESI Fig. 19 and 26†). Supporting the specificity of N3-1o for cysteine labeling, N3-1o afforded no appreciable blockade of proteome labeling by the lysine-reactive probe NHS-Rh (Fig. 5c). These gel-based assays support that the high specificity of the HAT N3-1o probe observed for recombinant protein labeling extends to complex cell lysates (Fig. 5b and c and ESI Fig. 26†).
Conclusions
To close, HAT provides a unique and stable chemotype for chemoselective cysteine modification without cross reactivity with other amino acids. The reactivity of HAT is tuned by modulation of the type of aromatic ring, heteroatom on the aromatic ring, oxidation state and methylation states of the heteroatom. The resulting HAT probes are highly reactive towards cysteine and stable to hydrolysis. One of the unique features of HAT probes is their ability to be reversed easily by external stimuli to generate unmodified units in a traceless manner thus indicating the potential utility of this method in many research fields including protein immobilization, proteomics and current drug discovery efforts avoiding the permanent modification of proteins. Surprisingly, HAT probes enable reversal of the reactivity of nucleophilic cysteine to electrophilic dehydroalanine under mild basic conditions (pH 10.5) thus allowing for the modification of proteins at the cysteine site by various nucleophiles such as thiols and amines. Another unique feature about HAT probes is their ability to increase the mass sensitivity of the resulting bioconjugates by 100 fold leading to easy detection of cysteine conjugates in a complex mixture which is of high significance in proteomics studies for identification of low abundant protein fragments.
Finally, we anticipate that the selectivity of the HAT will enable future studies aimed at identifying and pharmacologically manipulating functional cysteines in whole proteomes, as well as a starting point for therapeutic interventions by reversible covalent inhibition of the reactive cysteines. These innovative HAT tools for probing cysteine would augment existing detection methods and will dramatically expand the toolbox for bioconjugation, proteome profiling, and targeting otherwise undruggable protein targets.
Data availability
The datasets supporting this article have been uploaded as part of the ESI.†
Author contributions
M. R. and K. T. designed the project. K. T. performed all the synthetic experiments and characterized the compounds by NMR and LCMS. K. T. synthesized and purified peptides for the experiments. S. M. M. and K. B. performed proteomic studies. All authors analyzed the results and contributed to writing the manuscript.
Conflicts of interest
There are no conflicts to declare.
Acknowledgements
This research was supported by grants to M. R. from NIH (Grant No. 1R35GM133719-01) and Alfred P. Sloan Foundation.
Notes and references
- J. Singh, R. C. Petter, T. A. Baillie and A. Whitty, The resurgence of covalent drugs, Nat. Rev. Drug Discovery, 2011, 10, 307–317 CrossRef CAS PubMed
.
- D. S. Johnson, E. Weerapana and B. F. Cravatt, Strategies for discovering and derisking covalent, irreversible enzyme inhibitors, Future Med. Chem., 2010, 2, 949–964 CrossRef CAS PubMed
.
- B. F. Cravatt, A. T. Wright and J. W. Kozarich, Activity-based protein profiling: from enzyme chemistry to proteomic chemistry, Annu. Rev. Biochem., 2008, 77, 383–414 CrossRef CAS
.
- M. Fonović and M. Bogyo, Activity-based probes as a tool for functional proteomic analysis of proteases, Expert Rev. Proteomics, 2008, 5, 721–730 CrossRef
.
- L. E. Edgington, M. Verdoes and M. Bogyo, Functional imaging of proteases: recent advances in the design and application of substrate-based and activity-based probes, Curr. Opin. Chem. Biol., 2011, 15, 798–805 CrossRef CAS PubMed
.
- V. Chudasama, A. Maruani and S. Caddick, Recent advances in the construction of antibody-drug conjugates, Nat. Chem., 2016, 8, 114–119 CrossRef CAS PubMed
.
- K. Tsuchikama and Z. An, Antibody-drug conjugates: recent advances in conjugation and linker chemistries, Protein Cell, 2018, 9, 33–46 CrossRef CAS
.
- E. Weerapana, G. M. Simon and B. F. Cravatt, Disparate proteome reactivity profiles of carbon electrophiles, Nat. Chem. Biol., 2008, 4, 405–407 CrossRef CAS PubMed
.
- J. M. Chalker, G. J. Bernardes, Y. A. Lin and B. G. Davis, Chemical modification of proteins at cysteine: opportunities in chemistry and biology, Chem.–Asian J., 2009, 4, 630–640 CrossRef CAS PubMed
.
- E. Weerapana, C. Wang, G. M. Simon, F. Richter, S. Khare, M. B. Dillon, D. A. Bachovchin, K. Mowen, D. Baker and B. F. Cravatt, Quantitative reactivity profiling predicts functional cysteines in proteomes, Nature, 2010, 468, 790–795 CrossRef CAS PubMed
.
- D. Greenbaum, K. F. Medzihradszky, A. Burlingame and M. Bogyo, Epoxide electrophiles as activity-dependent cysteine protease profiling and discovery tools, Chem. Biol., 2000, 7, 569–581 CrossRef CAS PubMed
.
- G. C. Adam, B. F. Cravatt and E. J. Sorensen, Profiling the specific reactivity of the proteome with non-directed activity-based probes, Chem. Biol., 2001, 8, 81–95 CrossRef CAS
.
- L. Faleiro, R. Kobayashi, H. Fearnhead and Y. Lazebnik, Multiple species of CPP32 and Mch2 are the major active caspases present in apoptotic cells, EMBO J., 1997, 16, 2271–2281 CrossRef CAS PubMed
.
- A. B. Berger, M. D. Witte, J. B. Denault, A. M. Sadaghiani, K. M. Sexton, G. S. Salvesen and M. Bogyo, Identification of early intermediates of caspase activation using selective inhibitors and activity-based probes, Mol. Cell, 2006, 23, 509–521 CrossRef CAS
.
- K. M. Backus, B. E. Correia, K. M. Lum, S. Forli, B. D. Horning, G. E. González-Páez, S. Chatterjee, B. R. Lanning, J. R. Teijaro, A. J. Olson, D. W. Wolan and B. F. Cravatt, Proteome-wide covalent ligand discovery in native biological systems, Nature, 2016, 534, 570–574 CrossRef CAS PubMed
.
- A. M. Embaby, S. Schoffelen, C. Kofoed, M. Meldal and F. Diness, Rational Tuning of Fluorobenzene Probes for Cysteine-Selective Protein Modification, Angew. Chem., Int. Ed., 2018, 57, 8022–8026 CrossRef CAS PubMed
.
- D. A. Shannon, R. Banerjee, E. R. Webster, D. W. Bak, C. Wang and E. Weerapana, Investigating the proteome reactivity and selectivity of aryl halides, J. Am. Chem. Soc., 2014, 136, 3330–3333 CrossRef CAS
.
- H. L. Wong and D. C. Liebler, Mitochondrial protein targets of thiol-reactive electrophiles, Chem. Res.
Toxicol., 2008, 21, 796–804 Search PubMed
.
- S. G. Kathman, Z. Xu and A. V. Statsyuk, A fragment-based method to discover irreversible covalent inhibitors of cysteine proteases, J. Med. Chem., 2014, 57, 4969–4974 CrossRef CAS
.
- H. F. Motiwala, Y. H. Kuo, B. L. Stinger, B. A. Martin and B. R. Palfey, Tunable Heteroaromatic Sulfones Enhance in-Cell Cysteine Profiling, J. Am. Chem. Soc., 2020, 142, 1801–1810 CrossRef CAS
.
- C. Zambaldo, E. V. Vinogradova, X. Qi, J. Iaconelli, R. M. Iaconelli, M. Koh, K. Senkane, S. R. Chadwick, B. B. Sanchez, J. S. Chen, A. K. Chatterjee, P. Liu, P. G. Schultz, B. F. Cravatt and M. J. Bollong, 2-Sulfonylpyridines as Tunable, Cysteine-Reactive Electrophiles, J. Am. Chem. Soc., 2020, 142, 8972–8979 CrossRef CAS PubMed
.
- M. J. Evans, A. Saghatelian, E. J. Sorensen and B. F. Cravatt, Target discovery in small-molecule cell-based screens by in situ proteome reactivity profiling, Nat. Biotechnol., 2005, 23, 1303–1307 CrossRef CAS
.
- E. Weerapana, G. M. Simon and B. F. Cravatt, Disparate proteome reactivity profiles of carbon electrophiles, Nat. Chem. Biol., 2008, 4, 405–407 CrossRef CAS PubMed
.
- W. T. Lowther, D. A. McMillen, A. M. Orville and B. W. Matthews, The anti-angiogenic agent fumagillin covalently modifies a conserved active-site histidine in the Escherichia coli methionine aminopeptidase, Proc. Natl. Acad. Sci. U. S. A., 1998, 95, 12153–12157 CrossRef CAS
.
- G. J. Bernardes, J. M. Chalker, J. C. Errey and B. G. Davis, Facile conversion of cysteine and alkyl cysteines to dehydroalanine on protein surfaces: versatile and switchable access to functionalized proteins, J. Am. Chem. Soc., 2008, 130, 5052–5053 CrossRef CAS PubMed
.
- J. M. Chalker, Y. A. Lin, O. Boutureira and B. G. Davis, Enabling olefin metathesis on proteins: chemical methods for installation of S-allyl cysteine, Chem. Commun., 2009, 3714–3716 RSC
.
- H. Y. Shiu, T. C. Chan, C. M. Ho, Y. Liu, M. K. Wong and C. M. Che, Electron-deficient alkynes as cleavable reagents for the modification of cysteine-containing peptides in aqueous medium, Chemistry, 2009, 15, 3839–3850 CrossRef CAS
.
- M. E. Smith, F. F. Schumacher, C. P. Ryan, L. M. Tedaldi, D. Papaioannou, G. Waksman, S. Caddick and J. R. Baker, Protein modification, bioconjugation, and disulfide bridging using bromomaleimides, J. Am. Chem. Soc., 2010, 132, 1960–1965 CrossRef CAS
.
- G. L. Li, K. K. Kung, L. Zou, H. C. Chong, Y. C. Leung, K. H. Wong and M. K. Wong, Multifunctional bioconjugation by Morita–Baylis–Hillman reaction in aqueous medium, Chem. Commun., 2012, 48, 3527–3529 RSC
.
- N. Toda, S. Asano and C. F. Barbas 3rd, Rapid, stable, chemoselective labeling of thiols with Julia-Kocieński-like reagents: a serum-stable alternative to maleimide-based protein conjugation, Angew. Chem., Int. Ed., 2013, 52, 12592–12596 CrossRef CAS
.
- A. M. Spokoyny, Y. Zou, J. J. Ling, H. Yu, Y. S. Lin and B. L. Pentelute, A perfluoroaryl-cysteine S(N)Ar chemistry approach to unprotected peptide stapling, J. Am. Chem. Soc., 2013, 135, 5946–5949 CrossRef CAS PubMed
.
- A. Abbas, B. Xing and T. P. Loh, Allenamides as orthogonal handles for selective modification of cysteine in peptides and proteins, Angew. Chem., Int. Ed., 2014, 53, 7491–7494 CrossRef CAS
.
- C. Zhang, M. Welborn, T. Zhu, N. J. Yang, M. S Santos, T. Van Voorhis and B. L. Pentelute, Π-Clamp-mediated cysteine conjugation, Nat. Chem., 2016, 8, 120–128 CrossRef CAS
.
- S. P. Brown and A. B. Smith 3rd, Peptide/protein stapling and unstapling: introduction of s-tetrazine,
photochemical release, and regeneration of the peptide/protein, J. Am. Chem. Soc., 2015, 137, 4034–4037 CrossRef CAS PubMed
.
- B. Bernardim, P. M. Cal, M. J. Matos, B. L. Oliveira, N. Martínez-Sáez, I. S. Albuquerque, E. Perkins, F. Corzana, A. C. Burtoloso, G. Jiménez-Osés and G. J. Bernardes, Stoichiometric and irreversible cysteine-selective protein modification using carbonylacrylic reagents, Nat. Commun., 2016, 7, 13128 CrossRef CAS PubMed
.
- Y. Zhang, X. Zhou, Y. Xie, M. M. Greenberg, Z. Xi and C. Zhou, Thiol Specific and Tracelessly Removable Bioconjugation via Michael Addition to 5-Methylene Pyrrolones, J. Am. Chem. Soc., 2017, 139, 6146–6151 CrossRef CAS PubMed
.
- S. B. Gunnoo and A. Madder, Chemical protein modification through cysteine, ChemBioChem, 2016, 17, 529–555 CrossRef CAS PubMed
.
- J. Yu, X. Yang, Y. Sun and Z. Yin, Highly Reactive and Tracelessly Cleavable Cysteine-Specific Modification of Proteins via 4-Substituted Cyclopentenone, Angew. Chem., Int. Ed., 2018, 57, 11598–11602 CrossRef CAS PubMed
.
- A. O. Chan, J. L. Tsai, V. K. Lo, G. L. Li, M. K. Wong and C. M. Che, Gold-mediated selective cysteine modification of peptides using allenes, Chem. Commun., 2013, 49, 1428–1430 RSC
.
- K. K. Kung, H. M. Ko, J. F. Cui, H. C. Chong, Y. C. Leung and M. K. Wong, Cyclometalated gold(III) complexes for chemoselective cysteine modification via ligand controlled C–S bond-forming reductive elimination, Chem. Commun., 2014, 50, 11899–11902 RSC
.
- E. V. Vinogradova, C. Zhang, A. M. Spokoyny, B. L. Pentelute and S. L. Buchwald, Organometallic palladium reagents for cysteine bioconjugation, Nature, 2015, 526, 687–691 CrossRef CAS PubMed
.
- A. J. Rojas, C. Zhang, E. V. Vinogradova, N. H. Buchwald, J. Reilly, B. L. Pentelute and S. L. Buchwald, Divergent unprotected peptide macrocyclisation by palladium-mediated cysteine arylation, Chem. Sci., 2017, 8, 4257–4263 RSC
.
- M. S. Messina, J. M. Stauber, M. A. Waddington, A. L. Rheingold, H. D. Maynard and A. M. Spokoyny, Organometallic Gold(III) Reagents for Cysteine Arylation, J. Am. Chem. Soc., 2018, 140, 7065–7069 CrossRef CAS PubMed
.
- P. M. Cal, G. J. Bernardes and P. M. Gois, Cysteine-selective reactions for antibody conjugation, Angew. Chem., Int. Ed., 2014, 53, 10585–10587 CrossRef CAS PubMed
.
- G. J. Bernardes, M. Steiner, I. Hartmann, D. Neri and G. Casi, Site-specific chemical modification of antibody fragments using traceless cleavable linkers, Nat. Protoc., 2013, 8, 2079–2089 CrossRef CAS PubMed
.
- A. Leitner, T. Walzthoeni, A. Kahraman, F. Herzog, O. Rinner, M. Beck and R. Aebersold, Probing native protein structures by chemical cross-linking, mass spectrometry, and bioinformatics, Mol. Cell. Proteomics, 2010, 9, 1634–1649 CrossRef CAS PubMed
.
- R. M. Kaake, X. Wang and L. Huang, Profiling of protein interaction networks of protein complexes using affinity purification and quantitative mass spectrometry, Mol. Cell. Proteomics, 2010, 9, 1650–1665 CrossRef CAS
.
- D. D. Roberts, S. D. Lewis, D. P. Ballou, S. T. Olson and J. A. Shafer, Reactivity of small thiolate anions and cysteine-25 in papain toward methyl methanethiosulfonate, Biochemistry, 1986, 25, 5595–5601 CrossRef CAS
.
- T. P. King, Y. Li and L. Kochoumian, Preparation of protein conjugates via intermolecular disulfide bond formation, Biochemistry, 1978, 17, 1499–1506 CrossRef CAS PubMed
.
- M. E. Smith, F. F. Schumacher, C. P. Ryan, L. M. Tedaldi, D. Papaioannou, G. Waksman, S. Caddick and J. R. Baker, Protein modification, bioconjugation, and disulfide bridging using bromomaleimides, J. Am. Chem. Soc., 2010, 132, 1960–1965 CrossRef CAS PubMed
.
- V. Chudasama, M. E. Smith, F. F. Schumacher, D. Papaioannou, G. Waksman, J. R. Baker and S. Caddick, Bromopyridazinedione-mediated protein and peptide bioconjugation, Chem. Commun., 2011, 47, 8781–8783 RSC
.
- H. Y. Shiu, T. C. Chan, C. M. Ho, Y. Liu, M. K. Wong and C. M. Che, Electron-deficient alkynes as cleavable reagents for the modification of cysteine-containing peptides in aqueous medium, Chemistry, 2009, 15, 3839–3850 CrossRef CAS
.
- Y. Zhang, X. Zhou, Y. Xie, M. M. Greenberg, Z. Xi and C. Zhou, Thiol Specific and Tracelessly Removable Bioconjugation via Michael Addition to 5-Methylene Pyrrolones, J. Am. Chem. Soc., 2017, 139, 6146–6151 CrossRef CAS PubMed
.
- J. Yu, X. Yang, Y. Sun and Z. Yin, Highly Reactive and Tracelessly Cleavable Cysteine-Specific Modification of Proteins via 4-Substituted Cyclopentenone, Angew. Chem., Int. Ed., 2018, 57, 11598–11602 CrossRef CAS
.
- J.-R. Deng, S.-F. Chung, A. S.-L. Leung, W.-M. Yip, B. Yang, M.-C. Choi, J.-F. Cui, K. K.-Y. Kung, Z. Zhang, K.-W. Lo, Y.-C. Leung and M.-K. Wong, Chemoselective and photocleavable cysteine modification of peptides and proteins using isoxazoliniums, Commun. Chem., 2019, 2, 93 CrossRef
.
- T. Yan, H. S. Desai, L. M. Boatner, S. L. Yen, J. Cao, M. F. Palafox, Y. Jami-Alahmadi and K. M. Backus, SP3-FAIMS Chemoproteomics for High-Coverage Profiling of the Human Cysteinome, Chembiochem, 2021, 22, 1841–1851 CrossRef CAS
.
- M. Kuljanin, D. C. Mitchell, D. K. Schweppe, A. S. Gikandi, D. P. Nusinow, N. J. Bulloch, E. V. Vinogradova, D. L. Wilson, E. T. Kool, J. D. Mancias, B. F. Cravatt and S. P. Gygi, Reimagining high-throughput profiling of reactive cysteines for cell-based screening of large electrophile libraries, Nat. Biotechnol., 2021, 39, 630–641 CrossRef CAS PubMed
.
- E. A. Ilardi, E. Vitaku and J. T. Njardarson, Data-mining for sulfur and fluorine: an evaluation of pharmaceuticals to reveal opportunities for drug design and discovery, J. Med. Chem., 2014, 57, 2832–2842 CrossRef CAS PubMed
.
- A. L. Moure, G. Narula, F. Sorrentino, A. Bojang, C. K. M. Tsui, C. Sao Emani, E. Porras-De Francisco, B. Díaz, M. J. Rebollo-López, P. A. Torres-Gómez, E. M. López-Román, I. Camino, P. Casado Castro, L. Guijarro López, F. Ortega, L. Ballell, D. Barros-Aguirre, M. Remuiñán Blanco and Y. Av-Gay, MymA Bioactivated Thioalkylbenzoxazole Prodrug Family Active against Mycobacterium tuberculosis, J. Med. Chem., 2020, 63, 4732–4748 CrossRef CAS
.
- M. Abo, C. Li and E. Weerapana, Isotopically-Labeled Iodoacetamide-Alkyne Probes for Quantitative Cysteine-Reactivity Profiling, Mol. Pharm., 2018, 15, 743–749 CrossRef CAS
.
- K. C. Tang and M. Raj, One-Step Azolation Strategy for Site- and Chemo-Selective Labeling of Proteins with Mass-Sensitive Probes, Angew. Chem., Int. Ed., 2021, 60, 1797–1805 CrossRef CAS PubMed
.
- D. Zhang, N. O. Devarie-Baez, Q. Li, J. R. Lancaster Jr and M. Xian, Methylsulfonyl benzothiazole (MSBT): a selective protein thiol blocking reagent, Org. Lett., 2012, 14, 3396–3399 CrossRef CAS PubMed
.
- K. C. Tang, J. Cao, L. M. Boatner, L. Li, J. Farhi, K. N. Houk, J. Spangle, K. M. Backus and M. Raj, Tunable Amine-Reactive Electrophiles for Selective Profiling of Lysine, Angew. Chem., Int. Ed., 2021, 60, 1797–1805 CrossRef CAS
.
- W. C. Chen, J. S. Shen, T. Jurca, C. J. Peng, Y. H. Lin, Y. P. Wang, W. C. Shih, G. P. Yap and T. G. Ong, Expanding the Ligand Framework Diversity of Carbodicarbenes and Direct Detection of Boron Activation in the Methylation of Amines with CO2, Angew. Chem., Int. Ed., 2015, 54, 15207–15212 CrossRef CAS PubMed
.
-
J. M. Walker, The Protein Protocols Handbook, Humana Press, 2007 Search PubMed
.
- D. R. Goddard and L. Michaelis, Derivative of Keratin, J. Biol. Chem., 1935, 112, 361–371 CrossRef CAS
.
- N. Lundell and T. Schreitmüller, Sample preparation for peptide mapping – A pharmaceutical quality-control perspective, Anal. Biochem., 1999, 266, 31–47 CrossRef CAS
.
- M. Abo, C. Li and E. Weerapana, Isotopically-Labeled Iodoacetamide-Alkyne Probes for Quantitative Cysteine-Reactivity Profiling, Mol. Pharm., 2018, 15, 743–749 CrossRef CAS
.
- M. L. Nielsen, M. Vermeulen, T. Bonaldi, J. Cox, L. Moroder and M. Mann, Iodoacetamide-induced artifact mimics ubiquitination in mass spectrometry, Nat. Methods, 2008, 5, 459–460 CrossRef CAS PubMed
.
- J. A. Codelli, J. M. Baskin, N. J. Agard and C. R. Bertozzi, Second-generation difluorinated cyclooctynes for copper-free click chemistry, J. Am. Chem. Soc., 2008, 130, 11486–11493 CrossRef CAS
.
- J. M. Chalker, S. B. Gunnoo, O. Boutureira, S. C. Gerstberger, M. Fernández-González, G. J. L. Bernardes, L. Griffin, H. Hailu, C. J. Schofield and B. G. Davis, Methods for converting cysteine to dehydroalanine on peptides and proteins, Chem. Sci., 2011, 2, 1666–1676 RSC
.
- Y. Zhu and W. A. van der Donk, Convergent synthesis of peptide conjugates using dehydroalanines for chemoselective ligations, Org. Lett., 2001, 3, 1189–1192 CrossRef CAS PubMed
.
- D. P. Galonić, W. A. Van Der Donk and D. Y. Gin, Oligosaccharide-peptide ligation of glycosyl thiolates with dehydropeptides: synthesis of S-linked mucin-related glycopeptide conjugates, Chemistry, 2003, 9, 5997–6006 CrossRef PubMed
.
- A. M. Freedy, M. J. Matos, O. Boutureira, F. Corzana, A. Guerreiro, P. Akkapeddi, V. J. Somovilla, T. Rodrigues, K. Nicholls, B. Xie, G. Jiménez-Osés, K. M. Brindle, A. A. Neves and G. J. L. Bernardes, Chemoselective Installation of Amine Bonds on Proteins through Aza-Michael Ligation, J. Am. Chem. Soc., 2017, 139, 18365–18375 CrossRef CAS
.
- T. H. Wright, B. J. Bower, J. M. Chalker, G. J. Bernardes, R. Wiewiora, W. L. Ng, R. Raj, S. Faulkner, M. R. Vallée, A. Phanumartwiwath, O. D. Coleman, M. L. Thézénas, M. Khan, S. R. Galan, L. Lercher, M. W. Schombs, S. Gerstberger, M. E. Palm-Espling, A. J. Baldwin, B. M. Kessler, T. D. Claridge, S. Mohammed and B. G. Davis, Posttranslational mutagenesis: A chemical strategy for exploring protein side-chain diversity, Science, 2016, 354, 597–623 CrossRef CAS
.
- A. Yang, S. Ha, J. Ahn, R. Kim, S. Kim, Y. Lee, J. Kim, D. Söll, H. Y. Lee and H. S. Park, A chemical biology route to site-specific authentic protein modifications, Science, 2016, 354, 623–626 CrossRef CAS
.
-
P. Stefanowicz, A. Kluczyk, and Z. Szewczuk, in Amino Acids, Peptides and Proteins, 2015, vol. 40 Search PubMed
.
Footnote |
† Electronic supplementary information (ESI) available: General experimental methods, spectroscopic data and supplementary figures (PDF). See DOI: 10.1039/d1sc04139h |
|
This journal is © The Royal Society of Chemistry 2022 |
Click here to see how this site uses Cookies. View our privacy policy here.