DOI:
10.1039/D3RA05792E
(Paper)
RSC Adv., 2023,
13, 30548-30561
Selective catalytic synthesis of new terpenic chlorides using NaDCC as an eco-friendly and highly stable FAC agent†
Received
24th August 2023
, Accepted 9th October 2023
First published on 18th October 2023
Abstract
A simple, mild and efficient scope pathway for a selective catalytic chlorination of terpenic olefins is investigated in the presence of a highly efficient chlorination agent and a readily available Lewis acid catalyst. The sodium dichloroisocyanurate (NaDCC) used in the present work as an easy handling, sustainable and cost-effective chlorine donor due to its high free available chlorine (FAC), exhibits a high efficiency for selective catalytic chlorination. Herein, we report for the first-time the FeCl2/NaDCC combination system for the selective catalytic chlorination towards new functionalized terpenic olefins. In order to examine the general features of this catalytic reaction, the effects of pH, solvent, dilution, chlorination agent nature, stoichiometry and reaction kinetics are optimized using carvone as a model substrate. Among the studied parameters, catalyst stoichiometry was found to be determinant for highly controllable chlorination selectivity towards new allylic and vinylic chlorides. Indeed, the oxidation state, ligand and metal effects of the catalyst are examined using various Lewis acids, where the chlorinated ones (MClx), such as FeCl2, FeCl3 and SnCl2, exhibit a comprehensive approach for a controllable chlorination reaction. In addition, the homogeneous catalytic system shows good reusability with significant catalytic conversion depending on the FAC content in the reaction medium. The reaction proceeds under mild conditions with shorter reaction time and high selectivity towards new high added value allylic and vinylic chlorinated derivatives of naturally occurring terpenic olefins in good to excellent yields.
1. Introduction
Terpenes are one of the most natural compounds bearing allyl, vinyl or isopropenyl groups as a part of their structures.1 These groups are widely used as starting materials to obtain natural derived compounds or more complex atomic arrangements.2,3 Among the natural products, terpenes provide a sustainable supply of intermediates to produce flavors, perfumes and fine chemicals used in various industries and synthetic organic chemistry.4–6 For instance, the allylic chlorides are fundamental compounds in organic chemistry due to their strong electrophilic character.7 They can be transformed by functionalization, substitution, alkylation, reduction or oxidation of the double bond leading to a wide range of products such as allylic carbonates,8 allylic amines,9 allylic esters10 or β,γ-unsaturated acids.11 Thus, allylic chlorides can afford several functional groups for the synthesis of high added value natural compound derivatives.12–16 On the other hand, the vinylic chlorides are versatile chemicals that serve as valuable intermediates for the synthesis of natural product derivatives, vinyl polymers, adhesives, pharmaceuticals and agrochemical compounds.17–21 The presence of vinylic chloride in a backbone structure enhances the reactivity of the adjacent carbon–carbon double bond, enabling diverse chemical modifications such as oxidative or coupling reactions.19,22–25
Unlike their versatile importance in organic synthesis, few studies were devoted to vinylic halides preparation such as Barton synthesis from ketone-derived hydrazones,26 from ketones via vinyl phosphate intermediates,27 radical reaction of trichloromethylated substrates18 or via stereospecific isomerization of allylic halides.28 In contrast, the synthesis of allylic halides starting from allylic olefins is widely reported.29 For instance, the allyl chloride derivatives could be prepared from the corresponding allylic alcohols in presence of different reagents, e.g., titanium IV chloride,30 thionyl chloride,31 hydrochloric acid,32 chlorosilanes,33 sulfuryl chloride,34 N-chlorosuccinimide (NCS)35 or iridium catalyst.36,37 However, the allylic chloride intermediates can be synthesized from aldehydes through sequences of olefination–reduction–halogenation.7 In presence of NCS, Yb(OTf)3–TMSCl or aniline catalyst have been used to prepare allylic chlorides.38,39 Recently, nicotine has been reported as catalyst for the chlorination promoted by hypochlorous acid.40 Moreover, organoselenides have been used as catalysts for the allylic chlorination of simple and natural olefins.41–45 On the other hand, electrochemical methods are reported for the allylic chlorination of a variety of isoprenoids using sodium chloride as halogen source.46,47 While direct molecular chlorine bubbling through the reaction medium could be used for the chlorination, this method confronts various disadvantages including problems of handling chlorine gas.48 Therefore, other methods are based on the use of calcium hypochlorite49,50 or by a combination of Vilsmeier reagent and H2O2 but this excludes the use of acid-sensitive substrates.51 Besides, a combination of sodium hypochlorite and acetic acid was used for the chlorine generation with limitation to non-sensitive substrates.52
For a practical use, the replacement of Brønsted acid (e.g., acetic acid) by Lewis acid catalysts is reported as milder environment in the presence of chlorine source under biphasic system.48 Recently, we have reported the use of different Lewis acid catalysts in combination with NaClO for the chlorination of natural terpenic olefins.29 Among the reported Lewis acid catalysts, metal chlorides (MClx) such as CeCl3,48 InCl3,53 NbCl5,7 MoCl5
54 and AlCl3 or FeCl3
29 are the most reported for the catalytic chlorination of olefins. While the combination of MClx/NaClO is considered as an efficient solution for the catalytic chlorination of olefins, the use of NaClO solution as chlorinating agent faces some limitations in terms of reaction reproducibility due to the free available chlorine (FAC) stability and handling in the reaction medium. Recently, Hondo et al. have reported that the FAC of NaClO decreases by 20% due to gradual degradation and evaporation during storage.55 In this regard, the active chlorine content needs to be determined before each use for an efficient reaction reproducibility. Therefore, solid NaClO·5H2O has recently become commercially available to overcome the FAC limitations of using NaClO solution.56 However, the low melting point of solid NaClO (Tm ≈ 25 °C) makes handling the catalytic reaction difficult at room temperature.56–58 In contrast, sodium dichloroisocyanurate (NaDCC), with a high melting point of 225 °C, was reported to be favored for laboratory experiments.56,59 Hence, NaDCC is the most advantageous solid FAC donor over NaClO solution or solid NaClO·5H2O in terms of easy handling and high stability during both storage and use at room temperature. In this work, we propose the use of NaDCC as an advantageous, cost-effective and eco-friendly solid FAC donor alternative for the selective catalytic chlorination of terpenic olefins. Accordingly, the dissolution of NaDCC in water maintains an equilibrium with a slow release of hypochlorous acid (HClO) molecules (Scheme 1),55 which might be advantageous in the catalytic chlorination.
 |
| Scheme 1 Dissolution of NaDCC in aqueous medium. | |
As part of our ongoing research interest on the functionalization of natural terpenic olefins, herein we report an efficient, reliable and practical method for the selective catalytic chlorination of terpenes using a novel combination of FeCl2/NaDCC catalytic system. This catalytic chlorination system shows high selectivity and efficiency towards new allylic and vinylic terpenic derivatives. Moreover, various parameters such as effect of pH, solvent, dilution, chlorination agent nature, stoichiometry, reaction kinetics, and catalytic system reusability are studied using carvone as a model substrate. The present reported catalytic system represents an excellent high FAC tool towards new high added value functionalized natural compound derivatives under mild conditions, with reproducible quantitative yields.
2. Results and discussion
The use of NaDCC as high and stable FAC agent combined with Lewis acid catalyst (FeCl2) is selective for the catalytic chlorination of carvone a as model substrate (Scheme 2).
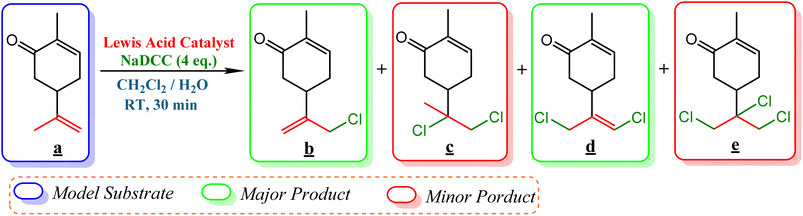 |
| Scheme 2 Catalytic chlorination of carvone a using NaDCC. | |
2.1. Optimization of FeCl2 and NaDCC amounts
First, we examined the effect of the Lewis acid and the chlorinating agent (FeCl2/NaDCC) amounts in the catalytic chlorination (Fig. 1). In absence of FeCl2, poor conversion was observed in presence of NaDCC even after 30 min of reaction. In presence of 4 eq. of NaDCC, an increase of FeCl2 amount enhances the conversion of carvone a with a variation in the selectivity of major reaction products (allylic monochloride b and vinyl allyl dichloride d) accompanied with byproducts formation (dichloride c and trichloride e). Hence, while (i) FeCl2 amount <1.75 eq. reveals a highly selective reaction towards b as major product; (ii) FeCl2 amount >1.75 eq. switches the selectivity towards the formation of d as major product with a good selectivity. Thereafter, 2.5 eq. of FeCl2 was chosen as an optimum for the formation of d and the effect of NaDCC amount on the allylic chlorination reaction was studied. While in absence of NaDCC no reaction took place, the use of 4 eq. reveals a high conversion (99%) with a maximum of selectivity (78%) towards d.
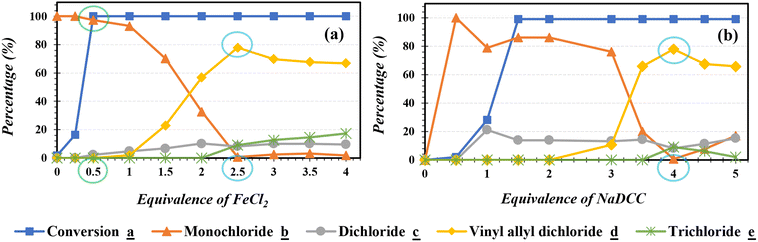 |
| Fig. 1 Effect of (a) FeCl2 and (b) NaDCC amounts on the selective catalytic chlorination reaction. | |
2.2. Influencing parameters on the selective chlorination reaction
2.2.1. Effect of pH. The pH effect on the selective catalytic chlorination reaction was investigated to understand the optimized equivalence, conversion and selectivity (Fig. 2). Hence, the amount of FeCl2 has no effect and shows a constant pH = 5 within the course of reaction (Fig. 2a). However, NaDCC has a significant effect on the pH (Fig. 2b), which affects the active chlorine content in the rection medium as well as the catalytic chlorination. While (i) an amount of NaDCC <1.5 eq. shows a fluctuation in pH from 5 to 2 within the course of reaction; (ii) the use of an amount ≥1.5 eq. maintains a stable pH = 5. Thus, the pH diagram could explain the total conversion obtained while using an amount of NaDCC ≥1.5 eq., which is reported as pH range for higher FAC.60 Accordingly, different distributions of aqueous chlorine species Cl2, HClO and ClO− are observed depending on pH.61 The diagram for chlorine/hypochlorous acid in aqueous solution shows a total predominance of dissolved Cl2 at pH < 1, HClO at 2 < pH < 6 and ClO− at pH > 9. It is well known that HClO is much faster than ClO− in terms of kinetics and reactivity.62 Therefore, the NaDCC solid reagent is acidic in solution and tends to reduce the pH of water favoring the formation of undissociated HClO. However, the chlorine loses its effectiveness through the dissociation of HClO at higher pH.63 In addition, hypochlorite (ClO−), being alkaline, tends to disadvantageously increase the pH, resulting to the HClO dissociation.64 In contrast, the capacity of NaDCC to continue significant releasing amounts of HClO allows it to operate over a wider pH range.65
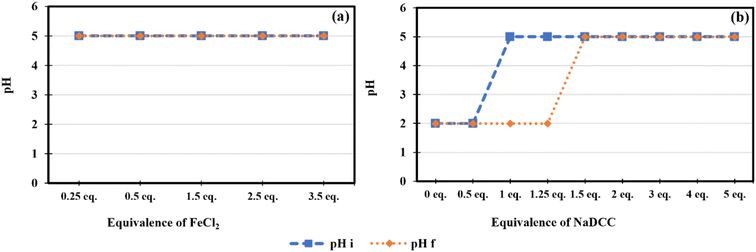 |
| Fig. 2 Effect of pH on the selective catalytic chlorination reaction while varying (a) FeCl2 and (b) NaDCC amounts. | |
2.2.2. Solvent effect. The solvent effect on the conversion and the selectivity of the catalytic chlorination reaction was studied. Under the optimized conditions leading to the major formation of d, a variety of polar, apolar, protic and aprotic solvents were examined (Table 1). The use of non-chlorinated protic and aprotic solvents leads to a complex mixture of reaction products. However, among the studied chlorinated solvents, CH2Cl2 gave the best result. While CHCl3 favored the formation of b in moderated selectivity, CCl4 shows a good conversion (79%) with a poor reaction selectivity. The obtained results can be explained by the polar character of CH2Cl2 compared to CHCl3 and CCl4, respectively. In contrast, the catalytic chlorination carried out in neat water reveals the formation of a complex mixture of reaction products with e as the major one.
Table 1 Solvent effect on the selective catalytic chlorination of carvone a a
Solvent |
Conversion a (%) |
Monochloride b (%) |
Dichloride c (%) |
Vinyl allyl dichloride d (%) |
Trichloride e (%) |
Reaction conditions: FeCl2 (2.5 eq.), NaDCC (4 eq. in 5 mL H2O), solvent/H2O (10 mL (5 : 5)), RT, 30 min. Conversion was determined by GC using an internal standard. |
CH2Cl2 |
100 |
Trace |
8 |
78 |
9 |
CHCl3 |
99 |
53 |
8 |
19 |
16 |
CCl4 |
79 |
4 |
13 |
17 |
20 |
Neat water |
100 |
5 |
4 |
Trace |
47 |
EtOH |
100 |
2 |
6 |
— |
25 |
Acetonitrile |
100 |
5 |
— |
5 |
30 |
Hexane |
58 |
2 |
5 |
4 |
34 |
The obtained results confirm that the chlorinated solvents have the best solvation effect and reveal the complexity of replacing CH2Cl2 with other alternatives in the catalytic chlorination reaction.29,48,53,54 In addition to the reported solvents in Table 1, other solvents such as carbonate ester were investigated with no significant results. Despite of the critical use of CH2Cl2, the reaction is handled at RT and the solvent is recycled under vacuum, which could decrease its environmental impact. Moreover, its unique abilities have helped to advance scientific research and improve the quality of numerous processes.66,67 Hence, it is crucial to ensure a balance between maximizing its benefits in industrial progress and minimizing its environmental and health impacts by exploring safer recovery methods and technologies.67,68
2.2.3. Effect of dilution. Next, we have studied the effect of dilution of both H2O and CH2Cl2 on the catalytic chlorination (Fig. 3). The increase of H2O volume decreased the selectivity of d due to the dilution of the FAC content in the reaction medium. 5 mL of H2O was found to be the optimum dissolution volume for the optimized equivalence of NaDCC. In contrast, CH2Cl2 volume has no effect on the selective catalytic chlorination.
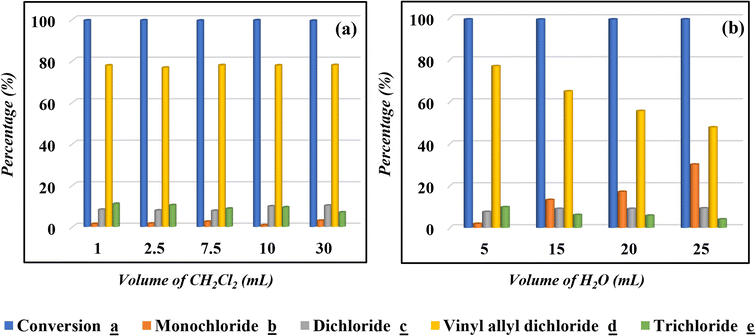 |
| Fig. 3 Effect of dilution of (a) CH2Cl2 and (b) H2O on the selective catalytic chlorination of carvone a. | |
2.2.4. Effects of chlorinating agent and FAC. To shed more light on the effects of chlorinating agent and FAC, we have carried out the catalytic chlorination reaction using NaDCC, NCS or NaClO in catalyst-free, 0.5 eq. and 2.5 eq. of FeCl2, respectively (Table 2). In presence of NCS, a slow reaction kinetics was observed with a slight catalytic effect on the reaction. However, with NaClO no reaction took place in catalyst-free condition even after a prolonged reaction time. While the use of 0.5 eq. of FeCl2 in presence of NaClO provides a very poor conversion (7%) with the only formation of b, the use of 2.5 eq. of catalyst almost leads to a total conversion with a mainly distributed selectivity between b and d (56 and 32%, respectively). The previously highlighted necessity of using a highly stable FAC chlorinating agent explains the significant results of NaDCC compared to NaClO in both reaction conditions. It has been reported that the amount of the added chlorine agents affects the reaction rate.55 Accordingly, the use of NaDCC improves the reaction time compared to the other chlorinating agents due to the formation of 2 mol of HClO while using only 1 mol of NaDCC (Scheme 1).
Table 2 Chlorinating agent and FAC effects on the selective catalytic chlorination of carvone a a,c
Chlorinating agent |
Catalyst amount (eq.) |
Time (h) |
Conversion a (%) |
Monochloride b (%) |
Dichloride c (%) |
Vinyl allyl dichloride d (%) |
Trichloride e (%) |
Reaction conditions: FeCl2 (in 5 mL H2O), chlorinating agent (4 eq. in 5 mL H2O), CH2Cl2/H2O (10 mL (5 : 5)), RT. Conversion was determined by GC using an internal standard. 5–10% FAC. NR: no reaction. |
NCS |
Catalyst-free |
0.5 |
NR |
NR |
NR |
NR |
NR |
24 |
34 |
74 |
26 |
— |
— |
0.5 |
0.5 |
NR |
NR |
NR |
NR |
NR |
24 |
52 |
46 |
54 |
— |
— |
2.5 |
0.5 |
Trace |
Trace |
— |
— |
— |
24 |
58 |
30 |
61 |
9 |
— |
NaDCC |
Catalyst-free |
0.5 |
Trace |
Trace |
— |
— |
— |
0.5 |
0.5 |
100 |
97 |
2 |
— |
— |
2.5 |
0.5 |
100 |
Trace |
8 |
78 |
9 |
NaClOb |
Catalyst-free |
0.5 |
NR |
NR |
NR |
NR |
NR |
0.5 |
0.5 |
7 |
100 |
— |
— |
— |
2.5 |
0.5 |
100 |
56 |
12 |
32 |
6 |
In a comparative purpose, both NaClO and NaDCC rely on HClO as active chlorine species with important differences in their performance. While NaClO releases all its chlorine as FAC, NaDCC releases only ≈50% of its chlorine as FAC.69 However, the balance remaining while using NaDCC is considered as ‘‘reservoir chlorine’’ in the form of chlorinated isocyanurate (Scheme 1). Thereafter, when the FAC is depleted, the equilibrium is disturbed via an immediate FAC releasing from the ‘‘reservoir’’ until the total consumption of chlorine.60 Therefore, the use of NaDCC presents the advantage of manipulating at variable pH by taking into consideration that HClO is a weak acid tending to dissociate in water at increased pH, which explains the obtained results compared to the other chlorinating agents.
2.2.5. Catalyst effects. To further enlighten the catalytic effect on the selective catalytic chlorination, a study of different types of Lewis acid catalysts, via the investigation of the oxidation state, ligand and metal effects, was carried out.
2.2.5.1. Effects of iron oxidation state and ligand. The catalytic activity of iron-based catalysts with different oxidation states on the selective catalytic chlorination has been studied with 0.5 and 2.5 eq. of catalyst (Fig. 4). While both Fe(II) and Fe(III) oxidation states reveal a good control of selectivity when varying the catalyst amount, Fe(0) shows a slow reaction kinetics with a loss in conversion and a lack of selectivity towards d due to its heterogeneous nature.
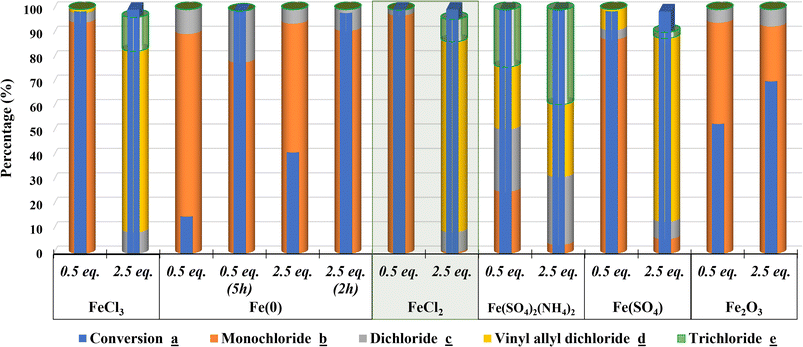 |
| Fig. 4 Effects of iron oxidation state and ligand on the selective catalytic chlorination of carvone a. | |
We have also studied the ligand effect in the presence of various iron-based Lewis acid catalysts (Fig. 4). The result indicates that chloride ligand is the most suitable for Lewis acid catalyst for a better controllable selectivity towards allylic and/or vinylic chlorides as major products. Moreover, sulfate ligand could also be suitable for a selective chlorination reaction. The slow reaction kinetics, conversion and lack in selectivity of d observed with Fe2O3 can be also due to the heterogeneous nature of the catalyst.
2.2.5.2. Metal effect. Furthermore, the selective catalytic chlorination was carried out in presence of different metal(II) chloride (MCl2, with M = Fe; Cu; Zn or Sn) under optimized conditions (Fig. 5). Accordingly, the comparative study indicates that iron is the most suitable metal for MCl2 Lewis acid catalyst for a better controllable selectivity. Additionally, SnCl2 is also revealed as suitable Lewis acid catalyst for a selective formation of d.
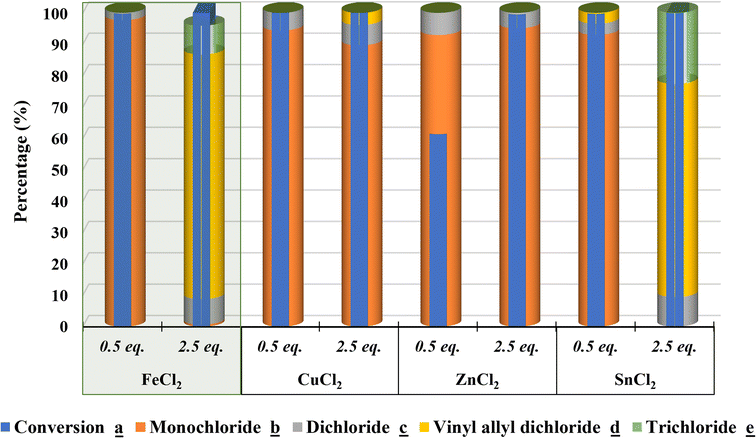 |
| Fig. 5 Metal effect on the selective catalytic chlorination of carvone a. | |
2.3. Reaction kinetics
It has been reported that 30 min represents the optimum reaction time for the catalytic chlorination.29,48,53,54 In order to gain a better insight on the reaction kinetics, the follow-up of the course of the catalytic performance under optimized conditions was investigated (Fig. 6). The reaction exhibits a significant reaction progress at t = 0 min (after the last drop of the chlorinating agent) with a high to good reaction selectivity. While the use of 0.5 eq. of FeCl2 leads to a complete reaction at about 5–10 min, the amount of 2.5 eq. exhibits a noticeable total conversion at t = 0 min. The reaction progress with a switching in selectivity started after 1 min of reaction between the two major products (b and d). In addition, the high selectivity obtained while using 2.5 eq. of FeCl2 after 5 min of reaction indicates the mechanistic pathway for the vinylic chloride formation d. Thus, the formation of d can be explained from the second allylic chlorination of b. However, the formation of byproducts (c and e) may be explained by the addition reaction of two chloride atoms. In addition to the better control of selectivity, the use of NaDCC enhances the catalytic performance in terms of reaction kinetics compared to the previous works.29,48,53,54
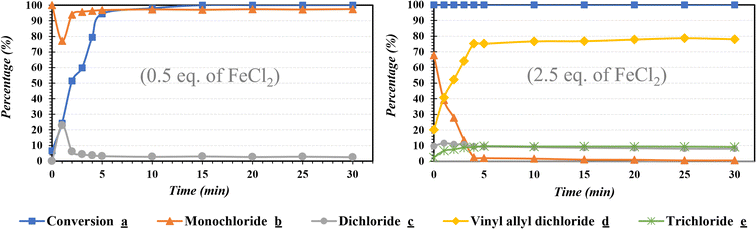 |
| Fig. 6 Reaction kinetics of the selective catalytic chlorination of carvone a using 0.5 and 2.5 eq. of FeCl2. | |
2.4. Reusability of the catalytic system
In catalysis, it is well known that the ability of a catalyst recycling is uncommon in homogenous catalysis compared to the heterogenous one, due to the complexity of the homogeneous reaction medium. However, we have studied the catalyst recycling ability of the optimized catalytic system FeCl2/NaDCC under the optimized reaction conditions (Fig. 6). Hence, the use of 0.5 eq. of FeCl2 shows a possible reusability of the catalytic system for three consecutive cycles (Fig. 7a). After each cycle, the catalytic system was recycled by removing the organic phase and reusing the aqueous one (catalytic system) for another cycle. At the third run, a poor conversion (16%) was observed, which indicates a depletion of the active chlorine (HClO) in the reaction medium that was confirmed by the addition of 1.5 eq. of NaDCC (optimized amount for a total chlorination (Fig. 1)), giving rise to a re-increased conversion. On the other hand, the use of 2.5 eq. of FeCl2 allows three consecutive cycles of reusing (Fig. 7b). At the fourth run, no reaction took place and the addition of 1.5 eq. of NaDDC confirms the depletion of the active chlorine via a re-increased conversion. Hence, the used recycling method for the studied homogeneous system allows us to evaluate both the reuse of the Lewis acid catalyst and the lifetime of the FAC during cycles. We can assume that the studied recyclability process confirms the great catalytic activity of the iron-based catalyst with a stable and continuous reusability depending on a significant presence of FAC in the reaction medium.
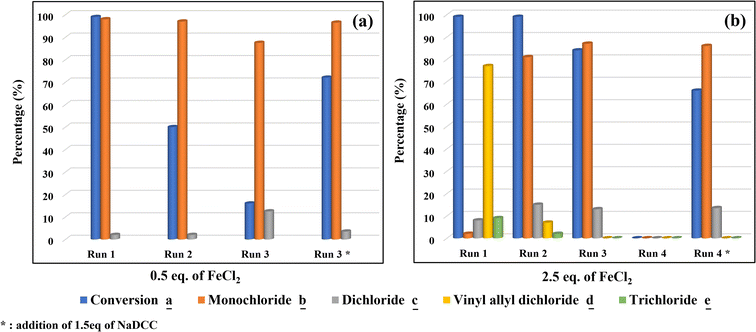 |
| Fig. 7 Reusability of the catalytic system in the selective chlorination of carvone a using (a) 0.5 and (b) 2.5 eq. of FeCl2. | |
2.5. Selective catalytic chlorination of various terpenic olefins
To enlighten the scope and limitations of the studied catalytic system FeCl2/NaDCC, the selective catalytic chlorination was extended to a much more demanding functionalized terpenic olefins such as limonene oxide (f), nootkatone (i), pulegone (m), perillyl aldehyde (p), perillyl alcohol (s) and limona ketone (t) (Table 3). The results depicted in Table 3 show that all substrates were converted to the corresponding allylic monochlorides as major products in presence of 0.5 eq. of FeCl2.29 In contrast, limona ketone (t)70 affords only the addition reaction even with 0.5 or 2.5 eq. of FeCl2, towards dichloride (u) as major product in good agreement with our previous study.29
Table 3 Selective catalytic chlorination of various terpenic olefinsa
Substrate |
Conversion (%) |
FeCl2 amount (eq.) |
TOF (s−1) |
Product/isolated yield (%) |
Reaction conditions: FeCl2 (0.5 eq. and 2.5 eq.), NaDCC (4 eq. in 10 mL H2O), CH2Cl2/H2O (30 mL (15 : 15)), RT, 30 min. Conversion was determined by GC using an internal standard. |
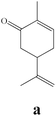 |
99% |
0.5 |
3.81 × 10−3 |
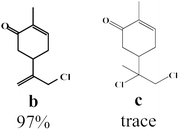 |
2.5 |
7.80 × 10−4 |
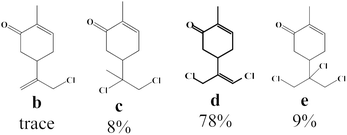 |
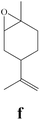 |
99% |
0.5 |
3.80 × 10−3 |
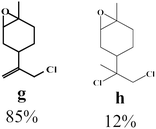 |
2.5 |
7.70 × 10−4 |
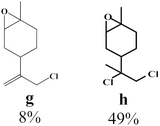 |
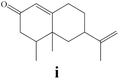 |
98% |
0.5 |
2.60 × 10−3 |
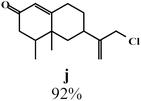 |
2.5 |
5.32 × 10−4 |
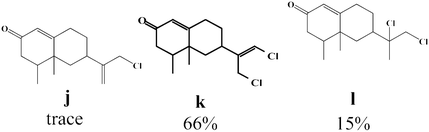 |
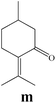 |
98% |
0.5 |
3.72 × 10−3 |
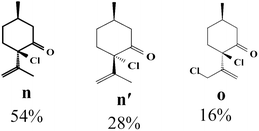 |
2.5 |
7.62 × 10−4 |
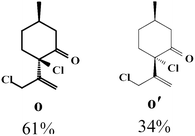 |
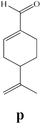 |
99% |
0.5 |
3.81 × 10−3 |
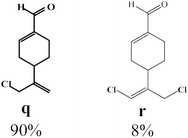 |
2.5 |
7.80 × 10−4 |
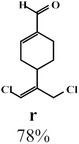 |
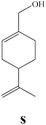 |
99% |
0.5 |
3.80 × 10−3 |
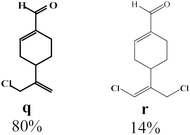 |
2.5 |
7.70 × 10−4 |
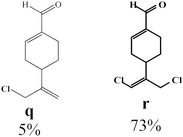 |
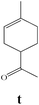 |
98% |
0.5 |
4.10 × 10−3 |
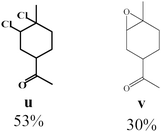 |
2.5 |
8.40 × 10−4 |
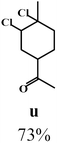 |
Due to the high interest of vinyl chlorides in organic synthesis and biological activities,19–21 the selective catalytic formation of vinylic chloride represents a good pathway for their direct one-step synthesis. The use of 2.5 eq. of FeCl2 leads to new vinyl allyl dichlorides d, k and r in good to excellent yields staring from a, i and p or s (Table 3, entries 1, 3, 5 and 6), respectively. However, f, m and t are not reactive towards vinylic chlorination. Moreover, f and t favor the addition reaction with the formation of h and u as major products, respectively. In contrast, m reveals a possible second allylic chlorination due to the presence of additional allylic reactive site. To the best of our knowledge, m leads for the first time to easily separable diastereomers (n: (R,R), n′: (R,S)) and (o: (R,R), o′: (R,S)). It is noteworthy to mention that no addition products are revealed in the catalytic chlorination of m, p and s.
2.6. Proposed mechanism for the selective catalytic chlorination reaction
To give further insight on the selective catalytic chlorination, a mechanistic pathway is proposed based on the obtained results (Scheme 3). Accordingly, the catalytic chlorination towards allylic or vinylic chlorides may be explained by a 1st and a simultaneous 2nd allylic chlorination, respectively. The chlorination reaction is probably based on the mild generation of electrophilic chloronium cation (Cl+) from the active chlorine species HClO, which further chlorinates the corresponding alkene via cationic intermediates (Scheme 3).29 While the loss of proton from the cationic intermediate in the 1st chlorination leads to the formation of the allylic chlorinated derivative, the 2nd chlorination leads to the formation of vinyl allyl dichlorinated derivative. However, a side reaction through competitive additions that form dichloride and trichloride derivatives is revealed. Based on the experimental results, we can assume that the addition products are not formed neither from the allyl nor the vinyl allyl derivatives, respectively. Furthermore, in the presence of metal ions, HClO can also generate anionic chlorine (Cl−).61,71 Hence, the addition reaction could probably be explained by the ring-opening of the cationic intermediates via the attack of the generated anionic chlorine (Scheme 3).
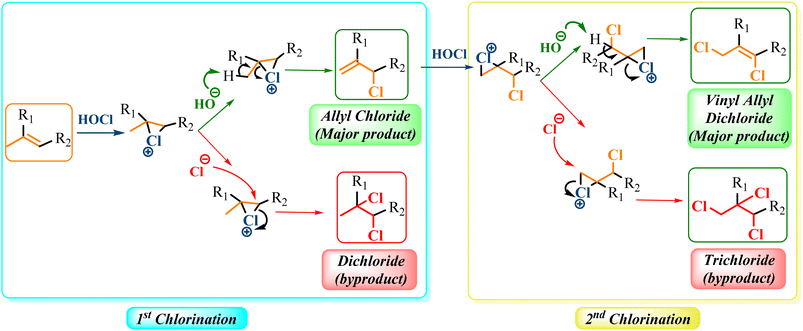 |
| Scheme 3 Proposed mechanism for the catalytic chlorination reaction. | |
3. Conclusion
In a summary, we have described a simple and efficient methodology for selective catalytic chlorination of naturally occurring terpenic olefins under mild conditions. A combination of cost-effective and highly stable FAC donor (NaDCC) with a readily available Lewis acid catalyst (FeCl2) in a biphasic system (CH2Cl2/H2O) was developed. The present reported catalytic system FeCl2/NaDCC reveals high conversion with a highly controllable selectivity towards allylic and/or novel vinylic chlorination reaction in a shorter reaction time. Various influencing parameters on the catalytic reaction (solvent, dilution, chlorination agent nature, FAC, stoichiometry, and reaction kinetics) were optimized using carvone as a model substrate. The optimization process reveals NaDCC as a mild, sustainable and cost-effective chlorine donor due to its high FAC efficiency for the selective catalytic chlorination reaction. Furthermore, the chlorinated Lewis acid catalyst (MClx), such as FeCl2, FeCl3 and SnCl2, and its stoichiometry were found to be determinant for highly controllable chlorination selectivity towards allylic or vinylic derivatives. Moreover, the chlorination of various terpenic olefins under the optimized conditions indicates that the substrates reactivity is also determinant for a better controllable selectivity. The studied homogeneous catalytic chlorination system (FeCl2/NaDCC) shows good reusability and represents an efficient tool to produce selectively and reproductively new high added value allylic and vinylic terpenic derivatives in good to excellent yields under mild conditions.
4. General procedure
In a typical procedure, terpenic olefin (0.16 mmol) in 5 mL of CH2Cl2 is added to a stirred solution of FeCl2 (0.5 or 2.5 eq.) in 5 mL of H2O at room temperature. Next, NaDCC (4 eq.) is dissolved in a minimum volume of H2O (5 mL) and added dropwise during 30 second to the mixture under vigorous stirring. After 30 min, the reaction is quenched with a saturated aqueous Na2SO3 solution and the organic layer is extracted with CH2Cl2 (3 × 10 mL) and dried over anhydrous Na2SO4. The solvent is removed and the crude is separated by silica gel column chromatography using hexane and ethyl acetate as eluents to obtain the pure chlorinated products. The isolated products were characterized by NMR (1H, 13C) and mass spectrometry (GC-MS) (ESI†).
Conflicts of interest
The authors declare that they have no conflicts of interest.
References
- B. M. Fraga, Natural sesquiterpenoids, Nat. Prod. Rep., 2010, 27(11), 1681–1708, 10.1039/c0np00007h.
- S. Bartel and F. Bohlmann, Total synthesis of the elmanolides (±) zempoalin A and B, Tetrahedron Lett., 1989, 30(6), 685–688 CrossRef CAS.
- A. F. Barrero, J. E. Oltra and M. Álvarez, A new strategy for the synthesis of (+)-vernolepin related compounds: an unusual sulfene elimination leads to the 2-oxa-cis-decalin skeleton, Tetrahedron Lett., 2000, 41(40), 7639–7643, DOI:10.1016/S0040-4039(00)01334-4.
- R. M. Scrowston, Chemistry of the monoterpenes. An encyclopedic handbook, parts A + B, Anal. Chim. Acta, 1986, 189, 393–394, DOI:10.1016/S0003-2670(00)83748-4.
- C. Botteghi, M. Marchetti and S. Paganelli, ChemInform Abstract: New Opportunities in Hydroformylation: Selected Syntheses of Intermediates and Fine Chemicals, Transition Met. Org. Synth., 1998, 30(11), 23–48, DOI:10.1002/chin.199911327.
- E. V. Gusevskaya, Organometallic catalysis: some contributions to organic synthesis, Quim. Nova, 2003, 26(2), 242–248, DOI:10.1590/S0100-40422003000200017.
- P. C. Ravikumar, L. Yao and F. F. Fleming, Allylic and allenic halide synthesis via NbCl5- and NbBr 5-mediated alkoxide rearrangements, J. Org. Chem., 2009, 74(19), 7294–7299, DOI:10.1021/jo901287f.
- S. El Houssame, A. A. Mekkaoui, S. Jennane, B. Boualy, N. Fdil, A. Karim and L. El Firdoussi, Palladium(0)-catalyzed allylic substitution of optically active natural, J. Mater. Environ. Sci., 2017, 8(S), 4778–4784 Search PubMed.
- B. Boualy, M. A. Harrad, S. El Houssame, L. El Firdoussi, M. A. Ali and A. Karim, Copper(II) catalyzed allylic amination of terpenic chlorides in water, Catal. Commun., 2012, 19, 46–50, DOI:10.1016/j.catcom.2011.12.019.
- S. El Houssame, L. El Firdoussi, S. Allaoud, A. Karim, Y. Castanet and A. Mortreux, Palladium-catalyzed alkoxycarbonylation of allylic natural terpenic functionalized olefins, J. Mol. Catal. A: Chem., 2001, 168(1–2), 15–23, DOI:10.1016/S1381-1169(00)00381-2.
- T. Okano, N. Okabe and J. Kiji, Palladium-Catalyzed, Atmospheric Pressure Carbonylation of Allylic Halides under the Influence of Sodium Hydroxide or Alkoxides. A Facile Synthesis of β,γ-Unsaturated Acids, Bull. Chem. Soc. Jpn., 1992, 65(10), 2589–2593, DOI:10.1246/bcsj.65.2589.
- S. El Houssame, H. Anane, L. Firdoussi and A. Karim, Palladium(0)-catalyzed amination of allylic natural terpenic functionalized olefins, Open Chem., 2008, 6(3), 470–476, DOI:10.2478/s11532-008-0042-3.
- G. Grue-Sorensen, I. M. Nielsen and C. K. Nielsen, Derivatives of 2-methylenepropane-1,3-diol as new antagonists of platelet activating factor, J. Med. Chem., 1988, 31(6), 1174–1178, DOI:10.1021/jm00401a017.
- C. J. Easton, A. J. Edwards, S. B. McNabb, M. C. Merrett, J. L. O’Connell, G. W. Simpson, J. S. Simpson and A. C. Willis, Allylic halogenation of unsaturated amino acids, Org. Biomol. Chem., 2003, 1(14), 2492–2498, 10.1039/b303719c.
- T. J. Brocksom, P. R. Zanotto and U. Brocksom, The enantioselective syntheses of bisabolane sesquiterpenes Lepistirone and Cheimonophyllon E, Tetrahedron Lett., 2005, 46(14), 2397–2398, DOI:10.1016/j.tetlet.2005.02.058.
- G. Mignani, J. P. Grass, P. Chabardes and D. Morel, Convenient synthesis of isoprenoid chlorides by a direct chlorination process, Tetrahedron Lett., 1992, 33(4), 495–498, DOI:10.1016/S0040-4039(00)93978-9.
- A. D. Asandei and V. Percec, From metal-catalyzed radical telomerization to metal-catalyzed radical polymerization of vinyl chloride: toward living radical polymerization of vinyl chloride, J. Polym. Sci., Part A: Polym. Chem., 2001, 39(19), 3392–3418, DOI:10.1002/pola.1322.
- P. Verhaeghe, P. Rathelot, S. Rault and P. Vanelle, Convenient Preparation of Original Vinylic Chlorides with Antiparasitic Potential in Quinoline Series, Lett. Org. Chem., 2006, 3(12), 891–897, DOI:10.2174/157017806779467997.
- X. Guinchard and E. Roulland, Various Entries to Vinyl Chloride Derivatives and their Applications in Total Synthesis of Natural Products, Synlett, 2011, 19, 2779–2788, DOI:10.1055/s-0031-1289862.
- S. Arimitsu, K. Terukina and T. Ishikawa, Stereoselective Synthesis of 4-Substituted 2,4-Dichloro-2-butenals by α- and γ-Regioselective Double Chlorination of Dienamine Catalysis, Synlett, 2018, 29(14), 1887–1891, DOI:10.1055/s-0037-1609559.
- T. Nishida, K. Satoh, M. Tamura, Y. Li, K. Tomishige, S. Caillol, V. Ladmiral, M. Vayer, F. Mahut, C. Sinturel and M. Kamigaito, Terpenoid-derived conjugated dienes with exo-methylene and a 6-membered ring: high cationic reactivity, regioselective living cationic polymerization, and random and block copolymerization with vinyl ethers, Polym. Chem., 2021, 12(9), 1186–1198, 10.1039/D1PY00035G.
- M. Hudlický, The Wichterle Reaction, Collect. Czech. Chem. Commun., 1993, 58(10), 2229–2244, DOI:10.1135/cccc19932229.
- S. Ma, X. Jiang, X. Cheng and H. Hou, Highly Efficient Suzuki Coupling Reaction of α-Chloroalkylidene-β-lactones and β-Lactams with Organoboronic Acids, Adv. Synth. Catal., 2006, 348(15), 2114–2124, DOI:10.1002/adsc.200600234.
- L. M. Geary and P. G. Hultin, Palladium-catalyzed modular assembly of electron-rich alkenes, dienes, trienes, and enynes from (E)-1,2-dichlorovinyl phenyl ether, J. Org. Chem., 2010, 75(19), 6354–6371, DOI:10.1021/jo1014678.
- A. Thakur, K. Zhang and J. Louie, Suzuki-Miyaura coupling of heteroaryl boronic acids and vinyl chlorides, Chem. Commun., 2012, 48(2), 203–205, 10.1039/c1cc15990a.
- M. E. Furrow and A. G. Myers, Practical procedures for the preparation of N-tert-butyldimethylsilylhydrazones and their use in modified Wolff-Kishner reductions and in the synthesis of vinyl halides and gem-dihalides, J. Am. Chem. Soc., 2004, 126(17), 5436–5445, DOI:10.1021/ja049694s.
- K. Kamei, N. Maeda and T. Tatsuoka, A practical synthetic method for vinyl chlorides and vinyl bromides from ketones via the corresponding vinyl phosphate intermediates, Tetrahedron Lett., 2005, 46(2), 229–232, DOI:10.1016/j.tetlet.2004.11.075.
- S. Martinez-Erro, V. García-Vázquez, A. Sanz-Marco and B. Martín-Matute, Stereospecific Isomerization of Allylic Halides via Ion Pairs with Induced Noncovalent Chirality, Org. Lett., 2020, 22(11), 4123–4128, DOI:10.1021/acs.orglett.0c01200.
- A. A. Mekkaoui, M. Laayati, H. Orfi, L. El Firdoussi and S. El Houssame, Catalytic Allylic Chlorination of Natural Terpenic Olefins Using Supported and Nonsupported Lewis Acid Catalysts, J. Chem., 2020, 1–8, DOI:10.1155/2020/2563634.
- P. V. S. N. Vani, A. S. Chida, R. Srinivasan, M. Chandrasekharam and A. K. Singh, Synthesis of β-ionone, Synth. Commun., 2001, 31(2), 219–224, DOI:10.1081/SCC-100000202.
- H. Jo, J. Lee, H. Kim, S. Kim and D. Kim, Efficient construction of bicyclic systems by an internal SN2′ enolate alkylation/ring-closing metathesis (RCM) strategy: a concise synthesis of the trans-hydrindane nucleus, Tetrahedron Lett., 2003, 44(37), 7043–7044, DOI:10.1016/S0040-4039(03)01780-5.
- T. Takahashi, A. Furutani and S. Seko, Process for the preparation of halogenated compounds, PCT International Patent Application, WO200061530-A, 2000 Search PubMed.
- M. Labrouillère, C. Le Roux, H. Gaspard-Iloughmane and J. Dubac, Bismuth (III) Chloride - Catalyzed Chlorination of Alcohols by Chlorosilanes 1, Synlett, 1994, 9, 723–724, DOI:10.1055/s-1994-22985.
- M. Bulliard, G. Balme and J. Gore, Chloration allylique d’olefines de type isoprenique a l’aide du chlorure de sulfuryle, Tetrahedron Lett., 1989, 30(42), 5767–5770, DOI:10.1016/S0040-4039(00)76192-2.
- L. H. Xu and E. P. Kiindig, Efficient Synthesis of Methyl 2-(tert-Butyl)acrylate and Analogous Esters, Helv. Chim. Acta, 1994, 77(6), 1480–1484 CrossRef CAS.
- N. Ahlsten, A. Bermejo Gómez and B. Martín-Matute, Iridium-Catalyzed 1,3-Hydrogen Shift/Chlorination of Allylic Alcohols, Angew. Chem., Int. Ed., 2013, 52(24), 6273–6276, DOI:10.1002/anie.201301013.
- A. Vázquez-Romero, A. B. Gómez and B. Martín-Matute, Acid- and Iridium-Catalyzed Tandem 1,3-Transposition/3,1-Hydrogen Shift/Chlorination of Allylic Alcohols, ACS Catal., 2015, 5(2), 708–714, DOI:10.1021/cs501618h.
- M. Yamanaka, M. Arisawa, A. Nishida and M. Nakagawa, An intriguing effect of Yb(OTf)3–TMSCl in the halogenation of 1,1-disubstituted alkenes by NXS: selective synthesis of allyl halides, Tetrahedron Lett., 2002, 43(13), 2403–2406, DOI:10.1016/S0040-4039(02)00260-5.
- R. C. Samanta and H. Yamamoto, Selective Halogenation Using an Aniline Catalyst, Chem.–Eur. J., 2015, 21(34), 11976–11979, DOI:10.1002/chem.201502234.
- V. Ximenes, N. Pavan, A. de Souza, G. Barros and N. Morgon, Nicotine as a Catalyst for Chlorination Promoted by Hypochlorous Acid: Experimental and Theoretical Studies, J. Braz. Chem. Soc., 2022, 34(1), 63–68, DOI:10.21577/0103-5053.20220088.
- K. B. S. Bernard Chabaud, Selenium-Catalyzed Nonradical Chlorination, J. Org. Chem., 1979, 44(23), 4204–4208 CrossRef.
- T. Hori and K. B. Sharpless, Conversion of Allylic Phenylselenides to the Rearranged Allylic Chlorides by N-Chlorosuccinimide. Mechanism of Selenium-Catalyzed Allylic Chlorination of β-Pinene, J. Org. Chem., 1979, 44(23), 4208–4210 CrossRef CAS.
- J. A. Tunge and S. R. Mellegaard, Selective Selenocatalytic Allylic Chlorination, Org. Lett., 2004, 6(8), 1205–1207, DOI:10.1021/ol036525o.
- A. F. Barrero, J. F. Quílez del Moral, M. M. Herrador, M. Cortés, P. Arteaga, J. V. Catalán, E. M. Sánchez and J. F. Arteaga, Solid-phase selenium-catalyzed selective allylic chlorination of polyprenoids: Facile syntheses of biologically active terpenoids, J. Org. Chem., 2006, 71(15), 5811–5814, DOI:10.1021/jo060760d.
- B. Boualy, S. El Houssame, L. Sancineto, C. Santi, M. Ait Ali, H. Stoeckli-Evans and L. El Firdoussi, A mild and efficient method for the synthesis of a new optically active diallyl selenide and its catalytic activity in the allylic chlorination of natural terpenes, New J. Chem., 2016, 40(4), 3395–3399, 10.1039/C5NJ02797G.
- S. Torii, K. Uneyama, T. Nakai and T. Yasuda, An electrochemical chlorinative ene-type reaction of isoprenoids, Tetrahedron Lett., 1981, 22(24), 2291–2294, DOI:10.1016/S0040-4039(01)92913-2.
- S. Torii, H. Tanaka, N. Saitoh, T. Siroi, M. Sasaoka and J. Nokami, Chemoselective electrolytic chlorination of methyl group of 3-methyl-3-butenoate moiety of thiazoline-azetidinone homologues, Tetrahedron Lett., 1981, 22(33), 3193–3196, DOI:10.1016/S0040-4039(01)81861-X.
- F. J. Moreno-Dorado, F. M. Guerra, F. L. Manzano, F. J. Aladro, Z. D. Jorge and G. M. Massanet, CeCl3/NaClO: a safe and efficient reagent for the allylic chlorination of terminal olefins, Tetrahedron Lett., 2003, 44(35), 6691–6693, DOI:10.1016/S0040-4039(03)01630-7.
- S. G. Hegde, M. K. Vogel, J. Saddler, T. Hrinyo, N. Rockwell, R. Haynes, M. Oliver and J. Wolinsky, The Reaction of Hypochlorous Acid with Olefins. A Convenient Synthesis of Allylic Chlorides, Tetrahedron Lett., 1980, 21(5), 441–444, DOI:10.1016/S0040-4039(00)71427-4.
- G. S. Hegde and J. Wolinsky, Synthesis with Hypochlorous Acid Functionalization of an Isopropenyl Group. Syntheses of (+)-Bilobanone and the Juvabiones, J. Org. Chem., 1982, 47(16), 3148–3150 CrossRef.
- Z. Xiong, J. Yang and Y. Li, An efficient asymmetric route to eudesmane acids. Total synthesis of (+)-12-hydroxy-α-cyperone, (+)-12-oxo-α-cyperone and (+)-3-oxoeudesma-4,11(13)-dien-12-oic acid, Tetrahedron: Asymmetry, 1996, 7(9), 2607–2612, DOI:10.1016/0957-4166(96)00335-7.
- M. P. VanBrunt, R. O. Ambenge and S. M. Weinreb, A Mild, Convenient, and Inexpensive Procedure for Conversion of Vinyl Halides to α-Haloketones, J. Org. Chem., 2003, 68(8), 3323–3326, DOI:10.1021/jo020739m.
- D. S. Pisoni, D. Gamba, C. V. Fonseca, G. S. da Costa, C. L. Petzhold, E. R. de Oliveira and M. A. Ceschi, InCl3/NaClO: A reagent for allylic chlorination of terminal olefins, J. Braz. Chem. Soc., 2006, 17(2), 321–327, DOI:10.1590/S0103-50532006000200015.
- B. Boualy, L. El Firdoussi, M. A. Ali and A. Karim, Allylic chlorination of terpenic olefins using a combination of MoCl5 and NaOCl, J. Braz. Chem. Soc., 2011, 22(7), 1259–1262, DOI:10.1590/S0103-50532011000700010.
- H. Hondo, T. Saito and A. Isogai, Preparation of oxidized celluloses in a TEMPO/NaBr system using different chlorine reagents in water, Cellulose, 2019, 26(5), 3021–3030, DOI:10.1007/s10570-019-02311-5.
- M. Kirihara, T. Okada, Y. Sugiyama, M. Akiyoshi, T. Matsunaga and Y. Kimura, Sodium Hypochlorite Pentahydrate Crystals (NaOCl·5H2O): A Convenient and Environmentally Benign Oxidant for Organic Synthesis, Org. Process Res. Dev., 2017, 21(12), 1925–1937, DOI:10.1021/acs.oprd.7b00288.
- T. Okada, T. Asawa, Y. Sugiyama, M. Kirihara, T. Iwai and Y. Kimura, Sodium Hypochlorite Pentahydrate (NaOCl·5H2O) Crystals as an Extra-ordinary Oxidant for Primary and Secondary Alcohols, Synlett, 2014, 25(04), 596–598, DOI:10.1055/s-0033-1340483.
- T. Okada, T. Asawa, Y. Sugiyama, T. Iwai, M. Kirihara and Y. Kimura, Sodium hypochlorite pentahydrate (NaOCl·5H2O) crystals; An effective re-oxidant for TEMPO oxidation, Tetrahedron, 2016, 72(22), 2818–2827, DOI:10.1016/j.tet.2016.03.064.
- S. Huo, H. Chen and W. Zuo, Selective Chlorination of Methane Photochemically Mediated by Ferric Chloride at Ambient Temperature, Chin. J. Org. Chem., 2021, 41(4), 1683, DOI:10.6023/cjoc202009044.
- T. Clasen and P. Edmondson, Sodium dichloroisocyanurate (NaDCC) tablets as an alternative to sodium hypochlorite for the routine treatment of drinking water at the household level, Int. J. Hyg. Environ. Health, 2006, 209(2), 173–181, DOI:10.1016/j.ijheh.2005.11.004.
- M. Deborde and U. von Gunten, Reactions of chlorine with inorganic and organic compounds during water treatment-Kinetics and mechanisms: a critical review, Water Res., 2008, 42(1–2), 13–51, DOI:10.1016/j.watres.2007.07.025.
- B. John and H. Colin, Catalysis or Convenience? Perborate in Context, in Advances in Inorganic Chemistry, Academic Press, 2013, pp. 217–310, DOI:10.1016/B978-0-12-404582-8.00006-7.
- C. J. Hurst, Disinfection of water: drinking water, recreational water and wastewater, in Disinfection, Sterilization and Preservation, ed. S. S. Block, USA Lippincott Williams & Wilkins, Philadelphia, PA, 5th edn, 2001, pp. 1023–1047 Search PubMed.
- J. A. B. Macedo and M. M. Barra, Derivados clorados de origem organica uma solucao para o porcesso de desinfeccao de agua potavel e para desinfeccao de industrias, in VI Simposio Italo Brasileiro de Engenharia Sanitaria e Ambiental, 2002, pp. 1–5 Search PubMed.
- G. R. Dychdala, Chlorine and chlorine compounds, in Disinfection, sterilization, and preservation, ed. S. S. Block, USA Lippincott Williams & Wilkins, Philadelphia, PA, 5th edn, 2001, pp. 135–157 Search PubMed.
- P. Liu, C. Tu, P. Gong, J. Tan, Y. Song, P. Yan and X. Shen, Effect of dichloromethane on the performance and yield rate of pure green petroleum coke products, Mater. Lett., 2022, 309(2021), 131387, DOI:10.1016/j.matlet.2021.131387.
- M. Wang, M. Zhang, S. Zeng, Y. Nie, T. Li, B. Ren, Y. Bai and X. Zhang, Effective Absorption of Dichloromethane Using Carboxyl-Functionalized Ionic Liquids, Int. J. Environ. Res. Public Health, 2023, 20(10), 5787, DOI:10.3390/ijerph20105787.
- Z. Y. Kong, A. Yang, J. G. Segovia-Hernández, A. Putranto and J. Sunarso, Towards sustainable separation and recovery of dichloromethane and methanol azeotropic mixture through process design, control, and intensification, J. Chem. Technol. Biotechnol., 2023, 98(1), 213–229, DOI:10.1002/jctb.7237.
- S. F. Bloomfield and G. A. Miles, The Antibacterial Properties of Sodium Dichloroisocyanurate and Sodium Hypochlorite Formulations, J. Appl. Bacteriol., 1979, 46, 65–73 CrossRef CAS PubMed.
- A. A. Mekkaoui, H. Ben El Ayouchia, H. Anane, R. Chahboun, L. El Firdoussi and S. El Houssame, Viable route and DFT study for the synthesis of optically active limonaketone: A barely available natural feedstock in Cedrus atlantica, J. Mol. Struct., 2021, 1235, 130221, DOI:10.1016/j.molstruc.2021.130221.
- F. A. Villamena, Chemistry of Reactive Species, in Reactive Species Detection in Biology, Elsevier, 2017, pp. 13–64, DOI:10.1016/B978-0-12-420017-3.00005-0.
|
This journal is © The Royal Society of Chemistry 2023 |
Click here to see how this site uses Cookies. View our privacy policy here.