DOI:
10.1039/C0NR00065E
(Communication)
Nanoscale, 2010,
2, 917-919
Carbon-stabilized iron nanoparticles for environmental remediation†
Received
29th January 2010
, Accepted 18th March 2010
First published on
11th May 2010
Abstract
Ferromagnetic carbon-coated Fe nanoparticles (core size of 15 nm, saturated magnetization of Ms = 218 emu g−1 and coercivity of Hc = 62 Oe), fabricated at a mild temperature, demonstrate a strong ability to effectively remove more than 95 wt% of Cr(VI) in waste water via carbon shell physical adsorption, which is much higher than the commercially available Fe NPs.
Metallic nanoparticles (NPs) of the iron group such as Co,1 Fe2 and Ni3 are of great interest due to their unusual magnetic properties4 and chemical catalytic properties5 arising from their small size and high specific surface area. Over the past decades, magnetic materials with various shapes and sizes have demonstrated a wide range of potential applications, for example in data storage,6,7 magnetic sensors,8 bio-medical9 (i.e. drug delivery, magnetic resonance imaging and hyperthermic treatment for malignant cells) and pharmaceutical areas.10,11 However, bare metal NPs are readily oxidized upon exposure to air, or even ignite spontaneously at room temperature.12 Hence, magnetic NPs with a protective shell are a prerequisite for their potential applications and the reported shells include silica,12 polymer,13 carbon,14 and noble metals.10,15–17 Compared to other shells, carbon exhibits much higher stability in harsh environments such as acidic or basic media18,19 and better biocompatibility.14 So far, techniques to synthesize a carbon shell include magnetron and ion-beam co-sputtering,20 high temperature annealing of a mixture of carbon-based materials and metal precursors,21 catalytic chemical vapor deposition,28 and pyrolysis of organometallic compounds.22 But all these methods are costly, which limits their applications.23 An economic method for large-scale fabrication of stabilized Fe NPs is still a challenge.
Hexavalent chromium Cr(VI) is a commonly identified contaminant in soils and groundwater because of its high toxicity and mobility.24 The maximum permissible limit of total chromium in drinking water has been recommended as 0.1 mg l−1 by the US Environmental Protection Agency (EPA).25 A variety of methods have been developed to remove Cr(VI) from waste water such as solvent extraction, ion exchange, osmosis, chemical precipitation, membrane separation and adsorption.26,27 Although these methods are efficient for Cr(VI) removal, the costs are relatively high.28 Consequently, an alternative adsorption is desirable and feasible because of its low-cost and high efficiency.29,30 Besides, adsorption can effectively reduce the low concentrations of heavy metals in wastewater when compared with chemical precipitation and electrochemical methods.31,32 Activated carbon prepared from coconut wood, lignin, petroleum, and coke is one of the adsorbents being used to purify polluted water.33,34 However, activated carbon has not been able to reduce the concentration of contaminants at ppb levels.35 Sun et al.36 have reported on the removal of methyl orange by carbon-encapsulated magnetic NPs, though the study on heavy metal removal is very limited. Magnetic NPs with regular shape and narrow size distribution are necessary to maintain the dispersion stability of NPs in the wastewater. The large specific surface area of the carbon shell will enhance the heavy metal adsorption. Additionally, the mobility of magnetic NPs will enhance greatly when external magnetic fields are introduced. This will facilitate recycling NPs after treatment of the toxic heavy metals.
In this paper, a facile method utilizing a mild-temperature annealing process is developed to fabricate crystalline Fe NPs (see ESI†). Economic sodium chloride is used as the template/spacer to prevent the NPs from agglomeration. The biodegradable and water-soluble polyvinyl alcohol (PVA) is chosen as carbon precursor. The structure and magnetic properties of Fe–C NPs are investigated by TEM and 9-T physical properties measurement system (PPMS). The surface functionality of NPs is studied with FT-IR. The removal of Cr(VI) by Fe–C NPs is investigated and an adsorption mechanism is proposed. Carbon-encapsulated magnetic NPs are justified as a promising candidate for efficient removal of heavy metal ions from wastewater.
Fig. 1(a) shows the TEM microstructures of the as-prepared NPs with an average size of 15 nm. The clear lattice fringes in high-resolution TEM, Fig.1(b), indicate that the NPs consist of an Fe core surrounded by carbon. The lattice fringe with a distance of 2.1 Å, depicting a highly crystalline structure, corresponds to the (1 1 0) plane of α-Fe crystal.12,37–40 α-Fe originates from the reduction between the formed iron oxide NPs41 and carbon.39 The outer lattice fringe with a calculated d-spacing of 2.0 Å corresponds to the (0 0 2) plane of graphite.42 The shell is relatively uniform and the thickness is about 8 nm. No pore is observed in the shell from HRTEM. The BET results show an average surface area of 72.54 m2 g−1, which is much larger than that (23.5 m2 g−1) of the commercial Fe NPs provided by the QuantumSphere Inc. and that (35 m2 g−1) of the reported Fe NPs.43 This is due to the introduced carbon shell. EDAX elemental analysis does not show any trace of NaCl, indicating that NaCl does not diffuse into the Fe metal lattice during the NPs fabrication. Without NaCl, the formed products are in the bulk form. Thus, only products with sodium chloride as templates are selected for characterization and heavy metal treatment (ESI, Fig S1†).
Magnetic properties
The NPs show a tendency to be attracted to a permanent magnet and the black aqueous solution of suspended NPs turns transparent within seconds when it is placed nearby (insert of Fig. 2). This indicates that the carbon shell has protected Fe NPs from oxidation or dissolution during acid washing. Fig. 2 shows the room temperature hysteresis loop of the Fe–C NPs. The magnetization does not reach saturation even at high field. The extrapolated saturation magnetization (Ms)44 of the NPs is 218 emu g−1 (based on Fe), which is comparable to that of the bulk Fe (222 emu g−1)44–50 and higher than that of polymer or carbon coated Fe NPs.46,48 Higher Ms is desirable for most applications such as biomedical or ferrofluid areas.51
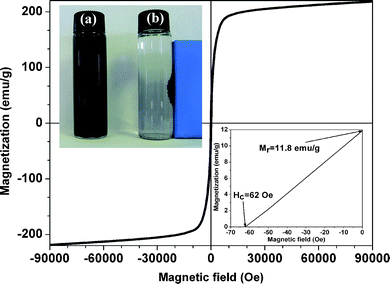 |
| Fig. 2 Magnetic hysteresis loop of Fe–C NPs. Left insert: (a) NPs redispersed in water and (b) attraction of the NPs to a permanent magnet; right inset: the enlarged partial hysteresis loop. | |
The coercivity (coercive force) is observed to be 62 Oe, which is much larger than that (5 Oe) of the bare Fe NPs with a comparable size.52 Fe NPs become magnetically harder after being coated with a carbon shell. The increased coercivity is due to the introduced carbon shell, which results in a reduced inter-particle dipolar interaction, arising from the increased nonmagnetic interparticle distance.19 The observed ferromagnetic behavior of the as-prepared Fe–C NPs is in good agreement with the reported ferromagnetic behavior of the Fe NPs with an average size of 13 nm.50
Adsorption removal of heavy metals
Fig. 3(a) shows the standard absorbance curve at 540 nm vs. the concentration of Cr(VI) by the 1,5-diphenylcarbazide (DPC) method. With the increase of the Cr(VI) concentration, the absorbance increases linearly. By correlating the UV-vis absorption of the reacted solution to the established standard absorption line, the concentration of residue Cr(VI) is determined, which will be sequentially used to calculate the removed amount of Cr(VI). Fig. 3(b) shows an absorbance of 0.086 for wastewater solution after treatment, which corresponds to a removal of 95 wt% Cr(VI) in water and is well below the limit of EPA (Cr(VI) < 100 μg L−1).25 During the reaction, no bubbles are observed in the solution, which indicates that the carbon shell effectively prohibits the Fe cores from reacting with protons. The Cr(VI) removal is found to take about 2 h and further reaction does not lead to further removal of Cr(VI). The NPs still can be attracted to a magnet after 2 h adsorption in acid solution. The adsorption capacity of Cr(VI) on the carbon-encapsulated Fe NPs is estimated to be 1.575 ml of wastewater with a lower Cr(VI) concentration of 400 μg L−1 per mg NPs, which is equal to a 630 μg Cr(VI) per g NPs and lower than the reported capacity of 84.4–109.3 mg Cr(VI) per g NPs43 for pure Fe NPs with a size of 60 nm due to the use of polluted water with a much higher Cr(VI) concentration (42.83 ± 0.52 mg L−1). As a comparison, the commericial Fe NPs with an average size of 20 nm from QuantumSphere Inc. have also been tested for heavy metal removal. With excess NPs, the Cr(VI) can only be removed by 86% and some bubbles are observed due to the redox reaction between the Fe NPs and protons.
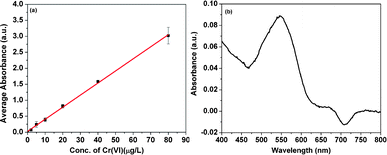 |
| Fig. 3 (a) The standard curve for DPC method quantification of Cr(VI); (b) UV-vis absorption of the solution after 2 h reaction. | |
Adsorption mechanism
Bare iron particles have been used to treat the Cr(VI) from polluted water by reduction mechanism.43 However, the Fe cores in the synthesized NPs are not accessible to Cr(VI). It is reported that carbon can greatly adsorb Cr(VI) from aqueous solutions.24,53–55 Therefore when core–shell NPs are added into the solution, the carbon shells effectively adsorb Cr(VI) onto the shell surface without sacrificing the magnetic core, which is indicated by the attraction to a magnet after excess acid and subsequent wastewater treatment. After 2 h immersion in Cr(VI) solution, the NPs are collected by a magnet and analyzed by EDAX. The result shows Cr coexisting with Fe and C, demonstrating that Cr(VI) is adsorbed onto the surface of carbon shell. The attraction to a magnet indicates a solid structure of the carbon shell, which prevents Cr(VI) going through the shell and contacting the iron core for etching. This nature of the NPs helps to recycle NPs after treatment with Cr(VI). The high adsorption removal percentage of Cr(VI) is due to the carboxylic functional group, which is consistent with the observations that the carboxylic functional groups present on the surface of carbon benefit the adsorption of heavy metals.56,57 This explains the high removal percentage of Cr(VI) with a Cr(VI) concentration of 400 μg L−1. Besides, for adsorption of Cr(VI), the contact time has a pronounced effect on the removal of pollutant species from aqueous solutions in the first 2 h, which means a rapid removal at the initial treatment stages. However, a further increase in time has a negligible effect on the removal of Cr(VI) and the removal percentage attained maximum at “equilibrium”.54 Hence, the process was conducted for 2 h.
In summary, we have demonstrated a facile method to fabricate carbon-coated iron NPs. The economic sodium chloride is found to effectively serve as a solid spacer to disperse the iron precursor and to prevent the NPs from agglomeration. The TEM result shows that the prepared NPs have microstructures of a Fe core with a diameter of around 15 nm coated with a thin carbon layer. The NPs exhibit room temperature ferromagnetic behavior with a high saturated magnetization of 218 emu g−1 and large coercivity of 62 Oe. The high saturation magnetization is desirable for various applications. These hybrid magnetic NPs have a certain amount of carboxylic functional groups on the carbon surface. The iron–carbon core–shell NPs have effectively adsorbed Cr(VI) with a removal percentage of 95 wt% without sacrificing the Fe cores.
Acknowledgements
This project is supported by the research enhancement grant (REG) from Lamar University. D. Zhang appreciates Fellowship support from Lamar University. D. P. Y. acknowledges support from the NSF under Grant No. DMR 04-49022. We are also grateful to Mr Mark Fullerton from QuantumSphere Inc for BET surface analysis.
Notes and references
- S. L. Tripp, S. V. Pusztay, A. E. Ribbe and A. Wei, J. Am. Chem. Soc., 2002, 124, 7914 CrossRef CAS.
- S.-J. Park, S. Kim, S. Lee, Z. G. Khim, K. Char and T. Hyeon, J. Am. Chem. Soc., 2000, 122, 8581 CrossRef CAS.
- Y. Hou and S. Gao, J. Mater. Chem., 2003, 13, 1510 RSC.
- P. Gambardella, S. Rusponi, M. Veronese, S. S. Dhesi, C. Grazioli, A. Dallmeyer, I. Cabria, R. Zeller, P. H. Dederichs, K. Kern, C. Carbone and H. Brune, Science, 2003, 300, 1130 CrossRef CAS.
- Y. Sun and Y. Xia, Science, 2002, 298, 2176 CrossRef CAS.
- Y. Wang, W. Wei, D. Maspoch, J. Wu, V. P. Dravid and C. A. Mirkin, Nano Lett., 2008, 8, 3761 CrossRef CAS.
- B. Terris and T. Thomson, J. Phys. D: Appl. Phys., 2005, 38, R199 CrossRef CAS.
- G. Li, V. Joshi, R. L. White, S. X. Wang, J. T. Kemp, C. Webb, R. W. Davis and S. Sun, J. Appl. Phys., 2003, 93, 7557 CrossRef CAS.
- J. H. Lee, Y. M. Huh, Y. W. Jun, J. W. Seo, J. T. Jang, H. T. Song, S. Kim, E. J. Cho, H. G. Yoon, J. S. Suh and J. Cheon, Nat. Med., 2007, 13, 95 CrossRef CAS.
- Z. Ban, Y. A. Barnakov, F. Li, V. O. Golub and C. J. O'Connor, J. Mater. Chem., 2005, 15, 4660 RSC.
- A. K. Gupta and M. Gupta, Biomaterials, 2005, 26, 3995 CrossRef CAS.
- N. Tang, W. Chen, W. Zhong, H. Y. Jiang, S. L. Huang and Y. W. Du, Carbon, 2006, 44, 423 CrossRef CAS.
- J. Wilson, P. Poddar, N. Frey, H. Srikanth, K. Mohomed, J. Harmon, S. Kotha and J. Wachsmuth, J. Appl. Phys., 2004, 95, 1439 CrossRef CAS.
- S. R. Rudge, T. L. Kurtz, C. R. Vessely, L. G. Catterall and D. L. Williamson, Biomaterials, 2000, 21, 1411 CrossRef CAS.
- Z. Guo, M. Moldovan, D. P. Young, L. L. Henry and E. J. Podlaha, Electrochem. Solid-State Lett., 2007, 10, E31 CrossRef CAS.
- Z. Lu, M. D. Prouty, Z. Guo, V. O. Golub, C. S. S. R. Kumar and Y. M. Lvov, Langmuir, 2005, 21, 2042 CrossRef CAS.
- Z. Guo, C. Kumar, L. Henry, E. Doomes, J. Hormes and E. J. Podlaha, J. Electrochem. Soc., 2005, 152, D1 CrossRef CAS.
- S. Xuan, L. Hao, W. Jiang, X. Gong, Y. Hu and Z. Chen, Nanotechnology, 2007, 18, 035602 CrossRef.
- X. Wei, G. Zhu and Y. Ye, Nanotechnology, 2006, 17, 4307 CrossRef CAS.
- T. Hayashi, S. Hirono, M. Tomita and S. Umemura, Nature, 1996, 381, 772 CrossRef.
- P. J. F. Harris and S. C. Tsang, Chem. Phys. Lett., 1998, 293, 53 CrossRef CAS.
- F. Ding, A. Rose'n, E. E. B. Campbell, L. K. L. Falk and K. Bolton, J. Phys. Chem. B, 2006, 110, 7666 CrossRef CAS.
- J. H. Kim, J. Kim, J. H. Park, C. K. Kim, C. S. Yoon and Y. Shon, Nanotechnology, 2007, 18, 115609 CrossRef.
- N. Hsu, S. Wang, Y. Lin, G. Sheng and J. Lee, Environ. Sci. Technol., 2009, 43, 8801 CrossRef CAS.
- Y. Xu and D. Zhao, Water Res., 2007, 41, 2101 CrossRef CAS.
- Y. Sharma, B. Singh, A. Agrawal and C. H. Weng, J. Hazard. Mater., 2008, 151, 789 CrossRef CAS.
- Y. A. Aydin and N. D. Aksoy, Chem. Eng. J., 2009, 151, 188 CrossRef CAS.
- J. Hu, G. Chen and I. M. C. Lo, Water Res., 2005, 39, 4528 CrossRef CAS.
- M. Imamoglu and O. Tekir, Desalination, 2008, 228, 108 CrossRef CAS.
- K. Kadirvelu, C. Faur-Brasquet and P. L. Cloirec, Langmuir, 2000, 16, 8404 CrossRef CAS.
- J.-K. Yang, H.-J. Park, H.-D. Lee and S.-M. Lee, Colloids Surf., A, 2009, 337, 154 CrossRef CAS.
- M. Zaini, R. Okayama and M. Machida, J. Hazard. Mater., 2009, 170, 1119 CrossRef CAS.
- S. Wang, M. Tsai, S. Lo and M. Tsai, Bioresour. Technol., 2008, 99, 7027 CrossRef CAS.
- Z. Tu, Q. He, X. Chang, Z. Hu, R. Gao, L. Zhang and Z. Li, Anal. Chim. Acta, 2009, 649, 252 CrossRef CAS.
- K. Pillay, E. M. Cukrowska and N. J. Coville, J. Hazard. Mater., 2009, 166, 1067 CrossRef CAS.
- Z. H. Sun, L. F. Wang, P. P. Liu, S. C. Wang, B. Sun, D. Z. Jiang and F.-S. Xiao, Adv. Mater., 2006, 18, 1968 CrossRef CAS.
- J. N. Wang, L. Zhang, F. Yu and Z. M. Sheng, J. Phys. Chem. B, 2007, 111, 2119 CrossRef CAS.
- L. Signorini, L. Pasquini, L. Savini, R. Carboni, F. Boscherini, E. Bonetti, A. Giglia, M. Pedio, N. Mahne and S. Nannarone, Phys. Rev. B: Condens. Matter Mater. Phys., 2003, 68, 195423 CrossRef.
- Y. Xiong, J. Ye, X. Gu and Q. Chen, J. Magn. Magn. Mater., 2008, 320, 107 CrossRef CAS.
- X. Dong, Z. Zhang, Q. Xiao, X. Zhao, Y. Chuang, S. Jin, W. Sun, Z. Li, Z. Zheng and H. Yang, J. Mater. Sci., 1998, 33, 1915 CrossRef CAS.
- Z. Guo, D. Zhang, S. Wei, Z. Wang, A. Karki, Y. Li, P. Bernazzani, D. Young, J. Gomes, D. Cocke and T. C. Ho, J. Nanopart. Res., 2010 DOI:10.1007/s11051-009-9802-z.
- S. H. Park, S. M. Jo, D. Y. Kim, W. S. Lee and B. C. Kim, Synth. Met., 2005, 150, 265 CrossRef CAS.
- J. Cao and W. X. Zhang, J. Hazard. Mater., 2006, 132, 213 CrossRef.
- Z. Guo, L. L. Henry, V. Palshin and E. J. Podlaha, J. Mater. Chem., 2006, 16, 1772 RSC.
-
B. D. Cullity, Introduction to Magnetic Materials (Addison-Wiley, New York, 1972) Search PubMed.
- H. Srikanth, R. Hajndl, C. Chirinos, J. Sanders, A. Sampath and T. S. Sudarshan, Appl. Phys. Lett., 2001, 79, 3503 CrossRef CAS.
- K. Suslick, M. Fang and T. Hyeon, J. Am. Chem. Soc., 1996, 118, 11960 CrossRef CAS.
- H. Cao, G. Huang, S. Xuan, Q. Wu, F. Gu and C. Li, J. Alloys Compd., 2008, 448, 272 CrossRef CAS.
- A. Casu, M. Casula, A. Corrias, A. Falqui, D. Loche, S. Marras and C. Sangregorio, Phys. Chem. Chem. Phys., 2008, 10, 1043 RSC.
- N. Burke, H. Stoever and F. Dawson, Chem. Mater., 2002, 14, 4752 CrossRef CAS.
- J. S. Yang and Q. W. Chen, Chin. J. Chem. Phys., 2008, 21, 76 CrossRef CAS.
- Z. Guo, H. Lin, A. B. Karki, D. P. Young and T. H. Hahn, Compos. Sci. Technol., 2008, 68, 2551 CrossRef CAS.
- P. Candela, J. Martinez and R. Macia, Water Res., 1995, 29, 2174 CrossRef.
- M. Dakiky, Khamis A. Manassra and M. Mer'eb, Adv. Environ. Res., 2002, 6, 533 CrossRef CAS.
- L. Ramos, A. J. Martinez and R. M. G. Coronado, Water Sci. Technol., 1995, 30, 191.
- D. Özçimen and A. Ersoy-Meriçboyu, J. Hazard. Mater., 2009, 168, 1118 CrossRef CAS.
- O. Uchida, Shinohara, S. Ito, N. Kawasaki, T. Nakamura and S. Tanada, J. Colloid Interface Sci., 2000, 224, 347 CrossRef.
Footnote |
† Electronic supplementary information (ESI) available: Particle fabrication and specific surface area determination. See DOI: 10.1039/c0nr00065e |
|
This journal is © The Royal Society of Chemistry 2010 |
Click here to see how this site uses Cookies. View our privacy policy here.