DOI:
10.1039/B9PY00326F
(Paper)
Polym. Chem., 2010,
1, 480-484
Synthesis of sulfur-containing poly(thioester)s with high refractive indices and high Abbe numbers
Received
30th October 2009
, Accepted 2nd December 2009
First published on 7th January 2010
Abstract
New poly(thioester)s (PTE)s containing sulfide and alicyclic units in polymer chains with high refractive indices and high Abbe numbers have been developed as thermoplastics. The PTEs were synthesized by the polycondensation reaction of 2,5-bis(sulfanylmethyl)-1,4-dithiane (BMMD) with 1,3-adamantanedicarbonyl dichloride (ADC) or cyclohexanedicarbonyl dichloride (CDC). Tough, flexible, and transparent amorphous polymer films were obtained. All polymers showed good thermal stability such as 5% weight-loss temperatures in the range 301–307 °C under nitrogen, and glass transition temperatures (Tg) in the range 65–110 °C. Poly(BMMD/ADC) and poly(BMMD/CDC) showed high refractive indices of 1.6149 and 1.6217, Abbe numbers of 32.1 and 30.0, respectively, and high transparency in the visible region.
Introduction
Various kinds of optical polymers with high refractive indices (high n) and high Abbe numbers (νD) have been developed for micro-optic and optoelectronic applications, such as lenses, prisms, waveguides, and diffractive gratings.1–3 Polymeric materials, divided into thermoplastics and thermosets for optical application, have several advantages, such as good processability, good impact resistance, and low weights.4 Thermoplastics such as poly(methyl methacrylate), polycarbonate, and cycloolefinpolymers5 are widely used in cameras, pickups, and projector lenses made by the injection molding process. On the other hand, thermosetting polymers, such as poly[ethylene glycol bis(allylcarbonate)] (CR-39)6 and resins from episulfides,7 polythiols,8 and polyisocyanates9 are manufactured by cast molding for consumer use, such as in eyeglasses. A general approach for increasing the refractive indices of polymers is the introduction of substituents with high molar refraction, low molar volume, or high density according to the Lorentz-Lorenz equation.10 Among the various substituents, the sulfur atom, which has high atomic refraction, is one of the most effective candidates. Recently, sulfur-containing polymers such as polysulfides,11 poly(thiourethane)s,12 and poly(thiomethacrylates),13 have been reported. In addition, the Abbe number (νD), which is a key parameter for the refractive index dispersion, is also an important parameter for optical materials used in the visible region. The Abbe number is given by the following equation: | νD = (nD − 1)/(nF − nC) | (1) |
where nD, nF, and nC are the refractive indices of the material at the wavelengths of the sodium D (589.3 nm), hydrogen F (486.1 nm), and hydrogen C (656.3 nm) lines, respectively.14
There is a well-known relationship between the nD and νD values for optical materials. Generally, highly refractive materials exhibit small Abbe numbers.15 Therefore, the balance between nD and νD is very important. Thermoplastics, which have high nD and high νD, have so far not been the subject of detailed investigation, compared to thermosets.
Recently, we prepared a new poly(thioether sulfone) (poly(DSDT/DVS)), which has sulfide, sulfone, and alicyclic units in polymer chains with the highest nD (1.686) and νD (48.6), as a thermoplastic.16 However, poly(DSDT/DVS) became colored above 150 °C in air, which is probably due to the thermally unstable thioacetalgroups. Coloration at elevated temperatures imposes a limitation because injection molding cannot be applied to poly(thioether sulfone).
Quite recently, we developed new sulfur-containing poly(thioether-sulfone)s derived from 2,5-bis(sulfanylmethyl)-1,4-dithiane (BMMD) and divinylsulfone (DVS) or bis(vinylsulfonyl)methane (BVSM) without thioacetalgroups.17 The polymers exhibited no coloration even at 200 °C in air, whereas poly(DSDT/DVS) became colored at 150 °C. Although coloration was not a problem, the thermal stability, such as low Tgs in the range 42–47 °C, was not high enough for optical applications using the injection molding process.
In this study, we developed new poly(thioester)s (PTE)s derived from BMMD and ADC containing an adamantanegroup or CDC containing a cyclohexanegroup to improve thermal stability, such as the glass transition temperature (Tg), because alicyclic and ester units (as compared to thioether linkages) impart chain rigidity in polymer chains. The PTEs exhibited high refractive indices in the range of 1.6149–1.6271, relatively high Abbe numbers in the range of 30.0–32.9, colorlessness, and high optical transparency in the visible region. Moreover, the thermal properties of the polymers, such as the Tgs ranging from 65 to 110 °C, were significantly improved. The effects of chemical structure on the thermal properties, optical transparency, refractive indices, and Abbe numbers of the polymers are discussed in detail.
Results and discussion
In order to prepare the PTEs, alicyclic monomers were synthesized according to the reported procedure.18–20 The polymerization of BMMD with ADC or CDC was carried out under reflux conditions for 12 h in chlorobenzene without a base, as shown in Scheme 1. The white-colored powders of the polymers were easily soluble in organic solvents such as chloroform and dichloromethane at room temperature. The number average and weight average molecular weights (Mn, Mw) of the poly(BMMD-ADC) and poly(BMMD-CDC) were 8000, 11900 and 22000, 34000, respectively. The polydispersity (PDI) of poly(BMMD/ADC) and poly(BMMD/CDC) was 1.49 and 1.54, respectively. The structures of the polymers were characterized by 1H NMR and FT-IR spectroscopy. The characteristic IR peaks of the polymers were observed at 1680 and 1686 cm−1, which are attributable to the carbonyl group. Fig. 1 shows the 1H NMR spectrum of the PTEs. For example, the signals of poly(BMMD/ADC) which resonated at 1.70, 1.89, 2.05, and 2.23 ppm are assignable to the adamantanegroup, and the complete absence of thiol (–SH) peaks in BMMD indicates the quantitative polyaddition of BMMD and ADC.19 The assignments of other proton signals are summarized in Fig. 1. These results indicate that new PTEs were successfully synthesized.
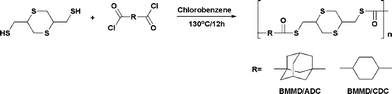 |
| Scheme 1 Synthesis of poly(BMMD/ADC) and poly(BMMD/CDC) | |
Thermal properties
The high thermal stabilities of polymers, such as high degradation temperature and high glass transition temperature, are one of the most important requirements for manufacturing optical components by injection molding. Thermally stable poly(BMMD/CHDT-DVS) without a crystallization peak was employed in our previous work.17 Although the thermal stability of poly(BMMD/CHDT-DVS) was improved without crystallization compared to poly(BMMD/DVS), the Tg was not high enough for manufacturing optical components. The thermal properties of poly(BMMD/ADC) and poly(BMMD/CDC) are summarized in Table 1. Poly(BMMD/ADC) and poly(BMMD/CDC) showed 5% weight loss temperatures (T5%) higher than 300 °C in nitrogen and air, as shown in Fig. 2. Fig. 3 shows the DSC curves of poly(BMMD/ADC) and poly(BMMD/CDC). The latter exhibited a Tg at 65 °C, which is much higher than that of poly(BMMD/DVS) in our previous work.17 In particular, poly(BMMD/ADC) showed a highly increased Tg at 110 °C because the adamantanegroup imparts the increased chain rigidity in the polymer chain. All results indicate that alicyclic PTEs are promising materials with improved thermal stability and suitable for injection molding.
Table 1 Thermal properties of the polymers
|
T
5%
/°C |
T
g
/°C |
5% weight loss temperature in nitrogen atmosphere.
Glass transition temperature.
From ref. 17.
|
Poly(BMMD/ADC) |
307 |
110 |
Poly(BMMD/CDC) |
301 |
65 |
Poly(BMMD/DVS)c |
303 |
47 |
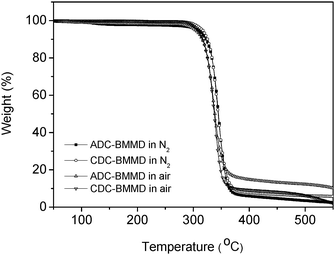 |
| Fig. 2
TGA curves of poly(BMMD/ADC) and poly(BMMD/CDC) (under nitrogen atmosphere and in air, 10 °C min−1). | |
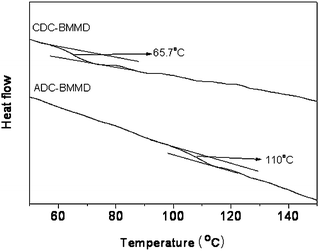 |
| Fig. 3
DSC curves of poly(BMMD/ADC) and poly(BMMD/CDC) (under a nitrogen atmosphere, 10 °C min−1). | |
Optical properties
Fig. 4 shows the UV-vis absorption spectra of the resulting polymer films with a thickness of ca. 10 μm. Both poly(BMMD/ADC) and poly(BMMD/CDC) exhibit high transparency in the visible region (λ = 400–800 nm) with transmittance over 98% at 400 nm even after thermal annealing above 150 °C (without Fresnel reflection). The high transparency may originate from the wholly aliphatic chemical structure without π-electrons. Fig. 5 shows the experimental refractive indices of poly(BMMD/ADC) and poly(BMMD/CD) at 486, 589, and 656 nm, respectively. The refractive indices and the Abbe numbers of the PTEs are summarized in Table 2.
Table 2 Optical properties of the polymers
Polymer
|
Sulfur content (wt %) |
n
D
|
n
F
|
n
C
|
ν
D
|
Measured at 589 nm.
Measured at 486 nm.
Measured at 633 nm.
Calculated using eqn (1).
|
Poly(BMMD/ADC) |
31.9 |
1.6149 |
1.6276 |
1.6089 |
32.9 |
Poly(BMMD/CDC) |
36.6 |
1.6271 |
1.6400 |
1.6191 |
30.0 |
Poly(BMMD/DVS) |
48.5 |
1.6512 |
1.6627 |
1.6474 |
42.6 |
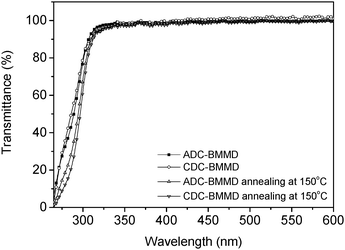 |
| Fig. 4
UV-vis spectra of poly(BMMD/ADC) and poly(BMMD/CDC) (film thickness: ∼10 μm). | |
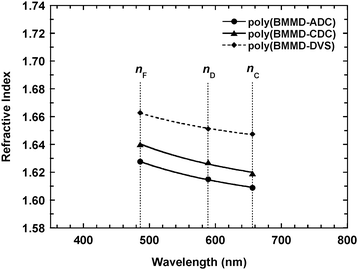 |
| Fig. 5 The experimental refractive indices of poly(BMMD/ADC) (●) and poly(BMMD/CDC) (▲). | |
The resulting polymers exhibit high refractive indices and relatively high Abbe numbers. The (nD, νD) values of poly(BMMD/ADC) and poly(BMMD/CDC) are (1.6149, 32.9) and (1.6271, 30.0), respectively. However, the refractive indices and Abbe numbers of the polymers are not as high as those of poly(BMMD/DVS), which has (nD, νD) values of (1.6512, 42.6), and which may originate from two factors. One is the sulfur content in the polymer repeating unit.
In general, the refractive index of sulfur-containing polymers depends on the sulfur content in the repeating unit. In contrast, sulfonyl groups (–SO2–), which endow low molecular dispersion as described in a previous paper,16 result in high Abbe numbers.
Fig. 6 shows the calculated absorption spectra and refractive index dispersions of the models for three polymers. The very short cutoff wavelengths at around 300 nm for all the polymers in Fig. 4 are well reproduced in the calculated spectra. Analysis of the TD-DFT calculations indicates that the one-electron transitions appearing above 200 nm are typical charge-transfer transitions with very small oscillation strengths, which are the essential cause of the high transparency. As shown in Fig. 6, the refractive indices and their dispersions for poly(BMMD/DVS), given in Table 2, are well reproduced in the calculation, whereas those of poly(BMMD/ADC) and poly(BMMD/CDC) are slightly over-estimated since the calculation does not involve the molecular packing state of the polymers, larger free volumes associated with the bulky adamantane and cyclohexanegroups in the latter two polymers lead to lower molecular packing densities, which reduces the refractive indices. Furthermore, the refractive index of poly(BMMD/ADC), which is lower than that of poly(BMMD/CDC), is also reproduced in the calculation, which indicates that the adamantane moiety possesses lower polarizability per unit volume than the cyclohexane moiety. In summary, although the refractive indices and Abbe numbers of poly(BMMD/ADC) and poly(BMMD/CDC) were not so highly improved compared to poly(BMMD/DVS) with the very high refractive index and Abbe number among the thermoplastics, the significant improvement in thermal stability at elevated temperatures of the alicyclic-derived PTEs, in particular poly(BMMD/ADC) with high Tg, is very beneficial for optical applications using injection molding.
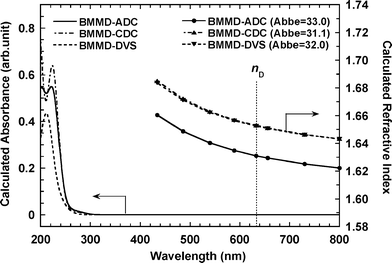 |
| Fig. 6 Calculated absorption spectra and refractive indices of model compounds, poly(BMMD/ADC), poly(BMMD/CDC), and poly(BMMD/DVS). | |
Conclusion
New thermoplastics, PTEs with high refractive indices and relatively high Abbe numbers combined with high Tgs, have been developed. The alicyclic-derived PTEs were synthesized by polycondensation reaction of BMMD with CDC or ADC in chlorobenzene. The PTEs showed high refractive indices in the range 1.6271–1.6149 and relatively high Abbe numbers in the range of 30.0–32.9, respectively. They also showed high thermal stability such as 5% weight loss temperature (T5%) in the range 307–301 °C and Tgs in the range 65–110 °C due to the introduction of alicyclic and estergroups in the polymer chains, which endow chain rigidity. The polymers, having good resistance against coloration even at 200 °C, make them good candidates for optical application using injection molding.
Experimental section
Measurements
FT-IR spectra were measured by a Horiba FT-120 Fourier transform spectrophotometer. NMR spectra were recorded on a BRUKER DPX-300S spectrometer at the resonant frequencies at 300 MHz for 1H and at 75 MHz for 13C nuclei, using CDCl3 or DMSO-d6 as a solvent. Ultraviolet-visible (UV-vis) spectra were recorded on a Hitachi U-3210 spectrophotometer in the wavelengths range 250–800 nm. Elemental analyses were performed on a Yanaco MT-6 CHN recorder elemental analysis instrument. Thermogravimetric analysis (TGA) of the PIs was performed on a Seiko TG/DTA 6300 under nitrogen atmosphere at a heating rate of 10 °C min−1. Differential scanning calorimetry (DSC) was estimated by using a Seiko DSC 6300 at a heating rate of 10 °C min−1. Number- and weight-average molecular weights (Mn and Mw) were evaluated by gel permeation chromatography (GPC) on a JASCO GULLIVER 1500 system equipped with a polystyrene gel column (Plgel 5 μm MIXED-C) eluted with CHCl3 at a flow rate of 1.0 mL min−1 calibrated by standard polystyrene samples. The refractive indices of polymer films were measured at the wavelengths of 486, 589, 656 nm by changing monochromatic filters with an Abbe refractometer (Atago, DR-M4). A halogen lamp with high brightness was used as a white light source.
Materials
2,5-Bis(sulfanylmethyl)-1,4-dithiane (BMMD), 1,3-adamantanedicarbony dilchloride (ADC), and cyclohexanedicarbonyl dichloride (CDC) was prepared according to the reported procedure.18–20Chlorobenzene was purchased from TCI, Japan and the other chemicals were used as received.
Synthesis of BMMD/ADC.
A solution of BMMD (1 mmol, 0.212 g) and ADC (1 mmol, 0.261 g) in chlorobenzene (1 mL) was refluxed at 130 °C for 12h. The resulting solution was poured into methanol. The precipitate was collected to give white powder (0.36 g, yield: 91.0%). IR (KBr, cm−1): ν = 2902 (alkyl C–H), 1678 (–C
O). 1H NMR (300 MHz, CDCl3, ppm): δ = 3.25 (d, 6H), 3.15–2.89 (m, 4H), 2.25 (s, 2H), 2.06 (s, 4H), 1.91 (s, 8H), 1.71 (s, 2H). Anal. Calcd. for C18H25O2S4: C, 53.83; H, 6.27. Found: C, 53.64; H, 5.84.
Synthesis of BMMD/CDC.
A solution of BMMD (1 mmol, 0.212 g) and CDC (1 mmol, 0.209 g) in chlorobenzene (1 mL) was refluxed at 130 °C for 12h. The resulting solution was poured into methanol. The precipitate was collected to give white powder (0.32 g, yield; 91.4%). IR (KBr, cm−1): ν = 2903 (alkyl C–H), 1685 (–C
O). 1H NMR (300 MHz, CDCl3, ppm): δ = 3.25 (d, 6H), 3.15–2.89 (m, 4H), 2.65 (s, 2H), 2.23–1.45 (m, 8H). Anal. Calcd. for C14H22O2S4: C, 47.96; H, 6.33. Found: C, 47.21; H, 5.49.
Calculation
One-electron transition energies and corresponding oscillator strengths of models for BMMD/ADC, BMMD/CDC, and BMMD/DVS were calculated using the time-dependent (TD)-DFT theory to predict the optical absorption in the UV-visible region.21,22 The wavelength-dependent refractive indices of the models were also calculated based on the Lorentz-Lorenz theory in the same way as in the previous report.23,24 The 6-311G(d) basis set was used for geometry optimizations under no constraints, and the 6-311++G(d,p) was used for calculations of linear polarizabilities, transition energies, and oscillator strengths. The three-parameter Becke-style hybrid functional (B3LYP) was adopted as a function, and all the calculations were performed using the software package of Gaussian-03 (Rev.C02 and D01). The densities of the corresponding polymers estimated from the empirical Bicerano's method were used for the calculation of refractive indices.
References
- C. Gao, B. Yang and J. Shen, J. Appl. Polym. Sci., 2000, 75, 1474 CrossRef CAS.
- T. Matsuda, Y. Funae, M. Yoshida, T. Yamamoto and T. Takaya, J. Appl. Polym. Sci., 2000, 76, 50 CrossRef CAS.
- J. G. Liu and M. Ueda, J. Mater. Chem., 2009, 19, 8907–8919 RSC.
- G. Changyou, Y. Bai and S. Jiacong, J. Appl. Polym. Sci., 2000, 75, 1474 CrossRef CAS.
- T. Kohara, Macromol. Symp., 1996, 101, 571 CAS.
- M. L. Rubin, Surv. Ophthalmol., 1986, 30, 321 CrossRef CAS.
-
H. Morishiri, S. Kobayashi, Jpn. Kokai Tokkyo Koho., 2005, JP 2005325274 Search PubMed.
-
H. Morishiri, S. Kobayashi, Jpn. Kokai Tokkyo Koho., 2006, JP 2006131724 Search PubMed.
-
R. Okada, T. Ohkubo, M. Kosaka, Eur. Pat. Appl., 1993, EP 530757 Search PubMed.
- S. Ando, T. Fujigaya and M. Ueda, Jpn. J. Appl. Phys., 2002, 41, L105–L108 CrossRef CAS.
- L. C. Lu, Z. C. Cui, Y. X. Wang, B. Yang and J. C. Shen, J. Appl. Polym. Sci., 2003, 89, 2426 CrossRef.
- A. Nebioglu, J. A. Leon and I. V. Khudyakov, Ind. Eng. Chem. Res., 2008, 47, 2155 CrossRef CAS.
- T. Matsuda, Y. Funae, M. Yoshida, T. Yamamoto and T. Takaya, J. Appl. Polym. Sci., 2000, 76, 45 CrossRef CAS.
- T. Okubo, S. Kohmoto and M. Yamamoto, J. Macromol. Sci., Part A: Pure Appl. Chem., 1998, 35, 1819 Search PubMed.
- C. J. Yang and S. A. Jenekhe, Chem. Mater., 1995, 7, 1276 CrossRef CAS.
- R. Okutsu, Y. Suzuki, A. Shinji and M. Ueda, Macromolecules, 2008, 41, 6165 CrossRef CAS.
- Y. Suzuki, T. Higashihara, S. Ando and M. Ueda, Eur. Polym. J., 2010, 46, 34–41 CrossRef CAS.
- M. D. Heagy, Q. Wang, G. A. Olah and G. K. S. Prakash, J. Org. Chem., 1995, 60, 7351 CrossRef CAS.
- T. Okubo, S. Kohmoto and M. Yamamoto, J. Appl. Polym. Sci., 1998, 68, 1791 CrossRef CAS.
- J. Liu and A. F. Yee, Macromolecules, 1998, 31, 7865 CrossRef CAS.
- S. Ando, T. Fujigaya and M. Ueda, Jpn. J. Appl. Phys., 2002, 41, L105 CrossRef CAS.
- S. Ando, T. Fujigaya and M. Ueda, J. Photopolym. Sci. Technol., 2002, 15, 559–568 CrossRef CAS.
- S. Ando, J. Photopolym. Sci. Technol., 2006, 19, 351–360 CrossRef CAS.
-
J. Bicerano, Prediction of Polymer Properties, Marcel Dekker, New York, 3rd edn, 2002 Search PubMed.
|
This journal is © The Royal Society of Chemistry 2010 |
Click here to see how this site uses Cookies. View our privacy policy here.